-
PDF
- Split View
-
Views
-
Cite
Cite
Sanghyuk S Shin, Chawangwa Modongo, Yeonsoo Baik, Christopher Allender, Darrin Lemmer, Rebecca E Colman, David M Engelthaler, Robin M Warren, Nicola M Zetola, Mixed Mycobacterium tuberculosis–Strain Infections Are Associated With Poor Treatment Outcomes Among Patients With Newly Diagnosed Tuberculosis, Independent of Pretreatment Heteroresistance, The Journal of Infectious Diseases, Volume 218, Issue 12, 15 December 2018, Pages 1974–1982, https://doi.org/10.1093/infdis/jiy480
- Share Icon Share
Abstract
Heteroresistant Mycobacterium tuberculosis infections (defined as concomitant infection with drug-resistant and drug-susceptible strains) may explain the higher risk of poor tuberculosis treatment outcomes observed among patients with mixed-strain M. tuberculosis infections. We investigated the clinical effect of mixed-strain infections while controlling for pretreatment heteroresistance in a population-based sample of patients with tuberculosis starting first-line tuberculosis therapy in Botswana.
We performed 24-locus mycobacterial interspersed repetitive unit–variable number tandem-repeat analysis and targeted deep sequencing on baseline primary cultured isolates to detect mixed infections and heteroresistance, respectively. Drug-sensitive, micro-heteroresistant, macro-heteroresistant, and fixed-resistant infections were defined as infections in which the frequency of resistance was <0.1%, 0.1%–4%, 5%–94%, and ≥95%, respectively, in resistance-conferring domains of the inhA promoter, the katG gene, and the rpoB gene.
Of the 260 patients with tuberculosis included in the study, 25 (9.6%) had mixed infections and 30 (11.5%) had poor treatment outcomes. Micro-heteroresistance, macro-heteroresistance, and fixed resistance were found among 11 (4.2%), 2 (0.8%), and 11 (4.2%), respectively, for isoniazid and 21 (8.1%), 0 (0%), and 10 (3.8%), respectively, for rifampicin. In multivariable analysis, mixed infections but not heteroresistant infections independently predicted poor treatment outcomes.
Among patients starting first-line tuberculosis therapy in Botswana, mixed infections were associated with poor tuberculosis treatment outcomes, independent of heteroresistance.
Recent advances in molecular methods have confirmed that patients with tuberculosis often harbor heterogeneous Mycobacterium tuberculosis strains [1, 2]. Multiple M. tuberculosis strains have been detected in patients with tuberculosis (a phenomenon known as mixed M. tuberculosis infection) in specimens from separate anatomical sites, in same specimen type at different times during treatment, and within the same sample [3–8]. Patients with tuberculosis harboring mixed M. tuberculosis infections may be at higher risk for poor treatment outcomes, but the mechanisms by which mixed infections affect clinical outcomes are poorly understood [8–11].
Mixed M. tuberculosis infections can be composed of concomitant infection with drug-resistant and drug-susceptible M. tuberculosis (heteroresistant infections) [3, 12–14]. Heteroresistance can also arise when an infection with a single drug-susceptible M. tuberculosis strain develops into a mix of susceptible and resistant organisms through microevolution [12, 13]. Heteroresistance is difficult to detect with conventional molecular methods. For example, the GeneXpert MTB/RIF assay, the most widely used molecular test for tuberculosis, can only reliably detect rifampicin (RIF)–resistant tuberculosis when the resistant M. tuberculosis subpopulation composes >50% of the bacilli in the sample [2, 8, 15, 16]. The Hain Genotype MTBDR assay (Hain Lifescience, Nehren, Germany), a line probe assay, can detect heteroresistance with a resistance population composing ≥5% of the bacilli in the sample [17, 18]. Patients with undiagnosed heteroresistant infection may be provided with suboptimal treatment regimens for their disease [3, 8]. In this scenario, amplification of the drug-resistant subpopulations may occur under antibiotic pressure [14]. Undetected pretreatment heteroresistance may, therefore, explain the higher risk of poor treatment outcomes among patients with mixed infections [19].
Recently, targeted next-generation deep sequencing has been used to detect heteroresistance involving minority M. tuberculosis subpopulations [20–22]. Deep sequencing involves the repeated reading of a specific region of M. tuberculosis DNA at high levels of depth to enable quantification of the diversity of reads at that locus [20–22]. By targeting loci with variants that have been shown to be associated with drug resistance, it is possible to detect and quantify heteroresistance involving a frequency of resistance-associated variants (RAVs) within an individual patient sample that is as low as <1% [20–22]. Specially designed amplicon sequencing assays and bioinformatics algorithms have been developed to minimize the impact of sequencing errors and increase confidence in quantitation of low-frequency reads. A recent study using the single-molecule–overlapping reads assay [21] and the amplicon sequencing analysis pipeline [20] among patients with multidrug-resistant (MDR) tuberculosis found that micro-heteroresistance (defined as detection of a resistance-associated variant in <5% of the reads at a targeted locus) may explain drug-resistant phenotypes that are missed by conventional genotypic tests [22].
The goal of the present study was to determine the impact of mixed infections on treatment outcomes among patients starting first-line tuberculosis therapy, while controlling for the effect of pretreatment heteroresistance. We hypothesized that the association between mixed infections and poor treatment outcomes is primarily due to a higher prevalence of undetected heteroresistant infections among patients with mixed-strain M. tuberculosis infections.
METHODS
Study Design
We conducted a retrospective cohort study of patients with tuberculosis who were enrolled in a population-based molecular epidemiology study in Botswana (the Kopanyo Study). We used 24-locus mycobacterial interspersed repetitive-unit–variable number tandem-repeat (MIRU-VNTR) and targeted deep sequencing (involving the inhA promoter, the katG gene, and the rpoB gene) to determine mixed infections and heteroresistance, respectively [2, 20–23].
Study Setting and Environment
Botswana is a country with one of the highest tuberculosis incidence rates in the world, with 326 cases/100000 persons in 2016 [24]. Among patients with tuberculosis, the prevalence of human immunodeficiency virus (HIV) infection is 60%, and tuberculosis is the leading cause of death among HIV-infected persons [24]. A national survey conducted in 2007–2008 found a 2.5% and 6.6% prevalence of MDR tuberculosis among new and previously treated patients with tuberculosis, respectively, in Botswana [25]. During this study, smear microscopy was the first-line diagnostic test. Phenotypic drug-susceptibility testing (DST) was done at treatment initiation for all patients, and treatment was modified on the basis of DST results. Patients with drug-susceptible tuberculosis are treated with a standardized regimen of daily isoniazid (INH), rifampicin (RIF), pyrazinamide (PZA), and ethambutol (EMB) therapy in the 2-month intensive phase and with daily INH and RIF therapy for the remaining 4-month continuation phase. For patients receiving treatment after relapse or failure, the intensive phase consists of 2 months of daily INH, RIF, PZA, EMB, and streptomycin therapy and 1 additional month of daily INH, RIF, PZA, and EMB therapy. The continuation phase for retreatment tuberculosis consists of 5 months of daily INH, RIF, and EMB therapy. Treatment is given as fixed-dose, single-formulation tablets.
Study Population
The Kopanyo Study recruited all newly diagnosed pulmonary tuberculosis cases in the Gaborone and Ghanzi districts of Botswana during September 2012–March 2016 [26]. For the present study, we included all patients enrolled in the Kopanyo Study with mixed-strain or possibly mixed-strain infections (defined below) and a random sample of patients with single-strain infections. We used the R package dplyr to randomly select 200 participants among the 1396 with single-strain infections who were enrolled at that time. Selected participants with single-strain infections had similar distribution of demographic and clinical characteristics, compared with participants with single-strain infections who were not selected (Supplementary Table 1).
Data Source
The analysis data set for this study was derived by combining data from the Kopanyo Study, data from the electronic tuberculosis registry of the Botswana Ministry of Health, and findings of deep sequencing analysis of archived M. tuberculosis DNA preparations. Phenotypic DST and MIRU-VNTR genotyping were performed for all M. tuberculosis–positive baseline specimens [27]. Additional details about patient enrollment and sample collection can be found in a previously published article [26]. Data extracted from the Kopanyo database included patient demographic information, tuberculosis treatment history, HIV status, CD4+ T-cell counts, current antituberculosis treatment regimen, phenotypic DST results, and MIRU-VNTR results.
Main Outcome
Tuberculosis treatment outcome data were extracted from the Botswana Ministry of Health national tuberculosis registry and electronically matched to study participants. The Botswana Ministry of Health categorizes treatment outcomes on the basis of World Health Organization–recommended definitions: cured, completed, lost to follow-up, failed, and death [28]. For this analysis, patients with tuberculosis with cured or completed outcomes were categorized as having a outcome, and all other patients were categorized as having a poor outcome.
MIRU-VNTR Genotyping
Sputum samples were collected at treatment initiation and cultured in liquid or solid medium after decontamination. We extracted DNA from M. tuberculosis grown on primary culture. We first prepared M. tuberculosis bacilli by centrifuging a suspension of scraped bacterial colonies from solid culture or a 1000-µL aliquot of broth from the liquid culture. DNA was extracted from pelleted bacilli, using heat killing and GenoLyse reagent (Hain Life Sciences, Nehren, Germany). DNA preparations were shipped to GenoScreen (Lille, France) for 24-locus MIRU-VNTR genotyping [27].
We defined mixed-strain M. tuberculosis infection categories on the basis of the presence of multiple repeats at MIRU-VNTR loci, which indicates genetic heterogeneity [2]. Mixed infections were defined as the presence of ≥2 repeats at >1 locus in the same sputum sample. To account for possible genetic heterogeneity due to microevolution of a single infecting strain, the presence of >1 repeat at a single locus was defined as a possibly mixed-strain infection. Single-strain infections were defined as those with single repeat patterns in all MIRU-VNTR loci.
Deep Sequencing
After MIRU-VNTR, selected leftover M. tuberculosis DNA preparations were shipped to the Translational Genomics Research Institute (Flagstaff, AZ) for targeted sequencing using the single-molecule–overlapping-reads assay [21]. We targeted a depth of approximately 25000 reads of gene segments in the inhA promoter, the katG gene, and the rpoB gene, which are known to confer resistance to INH and RIF. Mutant sequences in these loci are responsible for the majority (>95%) of cases of first-line drug resistance [29, 30].
We defined heteroresistance on the basis of the frequency of RAVs at each targeted locus quantified by deep sequencing of overlapping amplicon reads [21, 22]. To minimize misclassification due to sequencing error, we excluded reads from amplicons with an average depth of <2000 total reads for each target. We also limited the definition of heteroresistance to at least 10 reads mapping to a RAV at ≥1 targeted locus. We adapted thresholds for genetic heteroresistance proposed by Metcalfe et al [22]. Samples with a RAV frequency at ≥1 locus of ≥95%, 5%–94%, and 0.1%–4% were defined as fixed resistant, macro-heteroresistant, and micro-heteroresistant infections, respectively. Samples with <0.1% of reads mapping to RAVs at all targeted loci were categorized as fully sensitive infections. Files containing sequencing reads were deposited in the National Institutes of Health Sequence Read Archive under BioProject PRJNA44892.
Statistical Analysis
Proportions and percentages are used to describe categorical variables, and medians and interquartile ranges (IQRs) are used to describe continuous variables. The outcome variable was poor treatment outcome (failure, death, or loss to follow-up), with a poor outcome coded as 1 and a favorable outcome coded as 0. The risk of a poor treatment outcome for a patient subgroup was calculated by dividing the number of patients with a poor treatment outcome by the total number of patients in the subgroup. Risk ratios (RRs) were calculated by dividing the risk of a poor treatment outcome in the subgroup of interest by the risk in a reference subgroup. We calculated Wald 95% confidence intervals (CIs) for RRs [31].
We used multiple logistic regression analysis to estimate the independent effects of mixed-strain infections and heteroresistance on poor outcomes. A poor outcome was specified as the dependent variable, and mixed-strain infection and heteroresistance categories were classified as primary independent variables. The covariates age and HIV status were selected for inclusion in the model, based on a priori knowledge of predictors of tuberculosis treatment outcomes, regardless of their observed statistical associations with the outcome. Phenotypic DST results were not included in the model because of high correlation with heteroresistance categories. We excluded patients with macro-heteroresistant infections from the main model because only 2 patients were in this category. For sensitivity analysis, we fitted a separate model that combined macro-heteroresistant with heteroresistant infections and another model that combined this category with fixed resistant infections. In addition, we explored the effect of different definitions for poor outcome by fitting a model that excluded patients who were lost to follow-up and another model that excluded patients lost to follow-up and death.
We used R, version 3.0.4 (R Project for Statistical Computing; available at: http://www.r-project.org), for statistical analyses. In accordance with recent statistical guidelines, we did not specify a threshold for statistical significance [32, 33].
Ethical Considerations
This study was approved by the Botswana Ministry of Health Human Research Development Committee, and the institutional review boards of the University of Pennsylvania and the University of California–Irvine. Written informed consent was obtained from all participants.
RESULTS
Overall, 299 participants were initially selected for inclusion in the present analysis; 200 had single-strain infections, 74 had possibly mixed-strain infections, and 25 had mixed-strain infections. We excluded data for 31 participants (10.4%), owing to a failure to produce sequencing reads of sufficient quality, and for 8 participants (2.7%), owing to missing data for treatment outcome. The final analysis data set contained complete data for 173, 66, and 21 patients with single, possibly mixed, and mixed-strain infections, respectively (Table 1). Compared with patients with single-strain infections, patients harboring mixed-strain and possibly mixed-strain infections were more likely to be male, older, treated with therapy for new patients (category I), and infected with HIV (Table 1). In addition, a higher proportion of patients with mixed-strain infections had drug-resistant tuberculosis, based on phenotypic testing, compared with the other categories.
Patient Characteristics, by Heterogeneity of Infecting Mycobacterium tuberculosis Strain
Characteristic . | Single Strain (n = 173) . | Possibly Mixed Strain (Repeat at 1 Locus) (n = 66) . | Mixed Strain (Repeat at ≥2 loci) (n = 21) . |
---|---|---|---|
District | |||
Gaborone | 145 (83.8) | 54 (81.8) | 17 (81) |
Ghanzi | 28 (16.2) | 12 (18.2) | 4 (19) |
Sex | |||
Male | 98 (56.6) | 44 (66.7) | 13 (61.9) |
Female | 75 (43.4) | 22 (33.3) | 8 (38.1) |
Age, y, median (IQR) | 33 (23.7–40.9) | 36.2 (30.4–44.7) | 35.3 (29.4–45.5) |
Prior history of tuberculosis | |||
No | 142 (82.1) | 59 (89.4) | 19 (90.5) |
Yes | 31 (17.9) | 7 (10.6) | 2 (9.5) |
Treatment category | |||
New | 143 (82.7) | 59 (89.4) | 21 (100) |
Retreatment | 30 (17.3) | 7 (10.6) | 0 (0) |
HIV status | |||
Negative | 81 (46.8) | 26 (39.4) | 9 (42.9) |
Positive | 90 (52.0) | 38 (57.6) | 12 (57.1) |
Unknown | 2 (1.2) | 2 (3) | 0 (0) |
Phenotypic DST result | |||
Susceptible | 149 (86.1) | 56 (84.8) | 16 (76.2) |
INH monoresistance | 6 (3.5) | 3 (4.5) | 2 (9.5) |
RIF monoresistance | 2 (1.2) | 2 (3) | 0 (0) |
Multidrug resistance | 4 (2.3) | 0 (0) | 1 (4.8) |
Unknown | 12 (6.9) | 5 (7.6) | 2 (9.5) |
INH resistance mutation frequency, % | |||
<0.1 | 159 (91.9) | 60 (90.9) | 17 (81) |
0.1–4.9 (micro-heteroresistance) | 6 (3.5) | 3 (4.5) | 2 (9.5) |
5.0–94.9 (macro-heteroresistance) | 1 (0.6) | 0 (0) | 1 (4.8) |
≥95 (fixed resistance) | 7 (4) | 3 (4.5) | 1 (4.8) |
RIF resistance mutation frequency, % | |||
<0.1 | 138 (86.8) | 56 (90.3) | 15 (78.9) |
0.1–4.9 (micro-heteroresistance) | 13 (8.2) | 5 (8.1) | 3 (15.8) |
5.0–94.9 (macro-heteroresistance) | 0 (0) | 0 (0) | 0 (0) |
≥95 (fixed resistance) | 8 (5) | 1 (1.6) | 1 (5.3) |
Treatment outcome | |||
Completed | 101 (58.4) | 43 (65.2) | 11 (52.4) |
Cured | 53 (30.6) | 17 (25.8) | 5 (23.8) |
Lost to follow-up | 4 (2.3) | 2 (3) | 1 (4.8) |
Died | 11 (6.4) | 4 (6.1) | 1 (4.8) |
Failed | 4 (2.3) | 0 (0) | 3 (14.3) |
Characteristic . | Single Strain (n = 173) . | Possibly Mixed Strain (Repeat at 1 Locus) (n = 66) . | Mixed Strain (Repeat at ≥2 loci) (n = 21) . |
---|---|---|---|
District | |||
Gaborone | 145 (83.8) | 54 (81.8) | 17 (81) |
Ghanzi | 28 (16.2) | 12 (18.2) | 4 (19) |
Sex | |||
Male | 98 (56.6) | 44 (66.7) | 13 (61.9) |
Female | 75 (43.4) | 22 (33.3) | 8 (38.1) |
Age, y, median (IQR) | 33 (23.7–40.9) | 36.2 (30.4–44.7) | 35.3 (29.4–45.5) |
Prior history of tuberculosis | |||
No | 142 (82.1) | 59 (89.4) | 19 (90.5) |
Yes | 31 (17.9) | 7 (10.6) | 2 (9.5) |
Treatment category | |||
New | 143 (82.7) | 59 (89.4) | 21 (100) |
Retreatment | 30 (17.3) | 7 (10.6) | 0 (0) |
HIV status | |||
Negative | 81 (46.8) | 26 (39.4) | 9 (42.9) |
Positive | 90 (52.0) | 38 (57.6) | 12 (57.1) |
Unknown | 2 (1.2) | 2 (3) | 0 (0) |
Phenotypic DST result | |||
Susceptible | 149 (86.1) | 56 (84.8) | 16 (76.2) |
INH monoresistance | 6 (3.5) | 3 (4.5) | 2 (9.5) |
RIF monoresistance | 2 (1.2) | 2 (3) | 0 (0) |
Multidrug resistance | 4 (2.3) | 0 (0) | 1 (4.8) |
Unknown | 12 (6.9) | 5 (7.6) | 2 (9.5) |
INH resistance mutation frequency, % | |||
<0.1 | 159 (91.9) | 60 (90.9) | 17 (81) |
0.1–4.9 (micro-heteroresistance) | 6 (3.5) | 3 (4.5) | 2 (9.5) |
5.0–94.9 (macro-heteroresistance) | 1 (0.6) | 0 (0) | 1 (4.8) |
≥95 (fixed resistance) | 7 (4) | 3 (4.5) | 1 (4.8) |
RIF resistance mutation frequency, % | |||
<0.1 | 138 (86.8) | 56 (90.3) | 15 (78.9) |
0.1–4.9 (micro-heteroresistance) | 13 (8.2) | 5 (8.1) | 3 (15.8) |
5.0–94.9 (macro-heteroresistance) | 0 (0) | 0 (0) | 0 (0) |
≥95 (fixed resistance) | 8 (5) | 1 (1.6) | 1 (5.3) |
Treatment outcome | |||
Completed | 101 (58.4) | 43 (65.2) | 11 (52.4) |
Cured | 53 (30.6) | 17 (25.8) | 5 (23.8) |
Lost to follow-up | 4 (2.3) | 2 (3) | 1 (4.8) |
Died | 11 (6.4) | 4 (6.1) | 1 (4.8) |
Failed | 4 (2.3) | 0 (0) | 3 (14.3) |
Data are no. (%) of patients, unless otherwise indicated.
Abbreviations: DST, drug-susceptibility test; HIV, human immunodeficiency virus; INH, isoniazid; IQR, interquartile range; RIF, rifampicin.
Patient Characteristics, by Heterogeneity of Infecting Mycobacterium tuberculosis Strain
Characteristic . | Single Strain (n = 173) . | Possibly Mixed Strain (Repeat at 1 Locus) (n = 66) . | Mixed Strain (Repeat at ≥2 loci) (n = 21) . |
---|---|---|---|
District | |||
Gaborone | 145 (83.8) | 54 (81.8) | 17 (81) |
Ghanzi | 28 (16.2) | 12 (18.2) | 4 (19) |
Sex | |||
Male | 98 (56.6) | 44 (66.7) | 13 (61.9) |
Female | 75 (43.4) | 22 (33.3) | 8 (38.1) |
Age, y, median (IQR) | 33 (23.7–40.9) | 36.2 (30.4–44.7) | 35.3 (29.4–45.5) |
Prior history of tuberculosis | |||
No | 142 (82.1) | 59 (89.4) | 19 (90.5) |
Yes | 31 (17.9) | 7 (10.6) | 2 (9.5) |
Treatment category | |||
New | 143 (82.7) | 59 (89.4) | 21 (100) |
Retreatment | 30 (17.3) | 7 (10.6) | 0 (0) |
HIV status | |||
Negative | 81 (46.8) | 26 (39.4) | 9 (42.9) |
Positive | 90 (52.0) | 38 (57.6) | 12 (57.1) |
Unknown | 2 (1.2) | 2 (3) | 0 (0) |
Phenotypic DST result | |||
Susceptible | 149 (86.1) | 56 (84.8) | 16 (76.2) |
INH monoresistance | 6 (3.5) | 3 (4.5) | 2 (9.5) |
RIF monoresistance | 2 (1.2) | 2 (3) | 0 (0) |
Multidrug resistance | 4 (2.3) | 0 (0) | 1 (4.8) |
Unknown | 12 (6.9) | 5 (7.6) | 2 (9.5) |
INH resistance mutation frequency, % | |||
<0.1 | 159 (91.9) | 60 (90.9) | 17 (81) |
0.1–4.9 (micro-heteroresistance) | 6 (3.5) | 3 (4.5) | 2 (9.5) |
5.0–94.9 (macro-heteroresistance) | 1 (0.6) | 0 (0) | 1 (4.8) |
≥95 (fixed resistance) | 7 (4) | 3 (4.5) | 1 (4.8) |
RIF resistance mutation frequency, % | |||
<0.1 | 138 (86.8) | 56 (90.3) | 15 (78.9) |
0.1–4.9 (micro-heteroresistance) | 13 (8.2) | 5 (8.1) | 3 (15.8) |
5.0–94.9 (macro-heteroresistance) | 0 (0) | 0 (0) | 0 (0) |
≥95 (fixed resistance) | 8 (5) | 1 (1.6) | 1 (5.3) |
Treatment outcome | |||
Completed | 101 (58.4) | 43 (65.2) | 11 (52.4) |
Cured | 53 (30.6) | 17 (25.8) | 5 (23.8) |
Lost to follow-up | 4 (2.3) | 2 (3) | 1 (4.8) |
Died | 11 (6.4) | 4 (6.1) | 1 (4.8) |
Failed | 4 (2.3) | 0 (0) | 3 (14.3) |
Characteristic . | Single Strain (n = 173) . | Possibly Mixed Strain (Repeat at 1 Locus) (n = 66) . | Mixed Strain (Repeat at ≥2 loci) (n = 21) . |
---|---|---|---|
District | |||
Gaborone | 145 (83.8) | 54 (81.8) | 17 (81) |
Ghanzi | 28 (16.2) | 12 (18.2) | 4 (19) |
Sex | |||
Male | 98 (56.6) | 44 (66.7) | 13 (61.9) |
Female | 75 (43.4) | 22 (33.3) | 8 (38.1) |
Age, y, median (IQR) | 33 (23.7–40.9) | 36.2 (30.4–44.7) | 35.3 (29.4–45.5) |
Prior history of tuberculosis | |||
No | 142 (82.1) | 59 (89.4) | 19 (90.5) |
Yes | 31 (17.9) | 7 (10.6) | 2 (9.5) |
Treatment category | |||
New | 143 (82.7) | 59 (89.4) | 21 (100) |
Retreatment | 30 (17.3) | 7 (10.6) | 0 (0) |
HIV status | |||
Negative | 81 (46.8) | 26 (39.4) | 9 (42.9) |
Positive | 90 (52.0) | 38 (57.6) | 12 (57.1) |
Unknown | 2 (1.2) | 2 (3) | 0 (0) |
Phenotypic DST result | |||
Susceptible | 149 (86.1) | 56 (84.8) | 16 (76.2) |
INH monoresistance | 6 (3.5) | 3 (4.5) | 2 (9.5) |
RIF monoresistance | 2 (1.2) | 2 (3) | 0 (0) |
Multidrug resistance | 4 (2.3) | 0 (0) | 1 (4.8) |
Unknown | 12 (6.9) | 5 (7.6) | 2 (9.5) |
INH resistance mutation frequency, % | |||
<0.1 | 159 (91.9) | 60 (90.9) | 17 (81) |
0.1–4.9 (micro-heteroresistance) | 6 (3.5) | 3 (4.5) | 2 (9.5) |
5.0–94.9 (macro-heteroresistance) | 1 (0.6) | 0 (0) | 1 (4.8) |
≥95 (fixed resistance) | 7 (4) | 3 (4.5) | 1 (4.8) |
RIF resistance mutation frequency, % | |||
<0.1 | 138 (86.8) | 56 (90.3) | 15 (78.9) |
0.1–4.9 (micro-heteroresistance) | 13 (8.2) | 5 (8.1) | 3 (15.8) |
5.0–94.9 (macro-heteroresistance) | 0 (0) | 0 (0) | 0 (0) |
≥95 (fixed resistance) | 8 (5) | 1 (1.6) | 1 (5.3) |
Treatment outcome | |||
Completed | 101 (58.4) | 43 (65.2) | 11 (52.4) |
Cured | 53 (30.6) | 17 (25.8) | 5 (23.8) |
Lost to follow-up | 4 (2.3) | 2 (3) | 1 (4.8) |
Died | 11 (6.4) | 4 (6.1) | 1 (4.8) |
Failed | 4 (2.3) | 0 (0) | 3 (14.3) |
Data are no. (%) of patients, unless otherwise indicated.
Abbreviations: DST, drug-susceptibility test; HIV, human immunodeficiency virus; INH, isoniazid; IQR, interquartile range; RIF, rifampicin.
Frequencies of reads corresponding to targeted RAVs are presented in Figure 1. We found fixed resistance (RAV frequency, ≥95% at at least 1 locus) for INH and RIF in 11 patients (4.2%) and 10 patients (3.8%), respectively (Table 1). We found 2 patients (0.8%) harboring macro-heteroresistant infections for INH (both for katG315-AGC→ACC mutations) and none with RIF macro-heteroresistance (Table 1). Micro-heteroresistance to INH and RIF were found in samples from 11 patients (4.2%) and 21 patients (8.1%), respectively. Mutations leading to micro-heteroresistance were katG315-AGC->ACC for INH (n = 11) and rpoB526-CAC->AAC (n = 19), and rpoB531-TCG->TTG (n = 2). A higher proportion of patients with mixed-strain M. tuberculosis infections had INH and RIF micro-heteroresistant infections, compared to the other categories (Table 1).
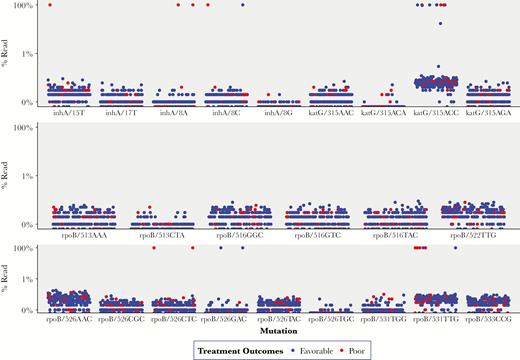
Frequency of resistance-associated mutations for isoniazid and rifampicin and treatment outcomes among 260 patients with tuberculosis from Botswana.
Overall, 30 (11.5%) patients had poor tuberculosis treatment outcomes (16 who died, 7 for whom treatment failed, and 7 who were lost to follow-up; Table 1). In unadjusted analysis (Table 2), the risk of poor treatment outcomes was higher among HIV-infected patients as compared to HIV-uninfected patients (RR, 3.98; 95% CI, 1.57–10.10), patients with MDR tuberculosis as compared to those with phenotypically INH and RIF susceptible tuberculosis (RR, 8.42; 95% CI, 4.63–15.31), and patients harboring organisms with fixed resistant RAVs as compared to those with fully genotypic susceptible infections (RR, 4.74; 95% CI, 2.38–9.44). One of 32 patients (3.2%) harboring micro-heteroresistant infections had poor outcomes, and the 2 patients with macro-heteroresistant infections had favorable outcomes. The risk of poor outcomes for single-strain, possibly mixed-strain, and mixed-strain infections was 11.0%, 9.1%, and 23.8%, respectively (Table 2). The unadjusted RR for poor outcomes among patients harboring mixed-strain infections was 2.17 (95% CI, .90–5.20), compared with single-strain infections.
Characteristic . | Poor Outcome, % (Proportion) . | Risk Ratio (95% CI) . |
---|---|---|
Sex | ||
Male | 9.7 (15/155) | 1.00 |
Female | 14.3 (15/105) | 1.48 (.75–2.89) |
Age, y | ||
<21 | 3.8 (1/26) | 1.00 |
21–30 | 5.5 (4/73) | 1.42 (.17–12.17) |
31–40 | 15.5 (13/84) | 4.02 (.55–29.31) |
41–50 | 17.1 (7/41) | 4.44 (.58–34.03) |
≥51 | 14.7 (5/34) | 3.82 (.48–30.77) |
Prior history of tuberculosis | ||
No | 10.9 (24/220) | 1.00 |
Yes | 15.0 (6/40) | 1.38 (.60–3.15) |
Treatment category | ||
New | 11.7 (26/223) | 1.00 |
Retreatment | 10.8 (4/37) | 0.93 (.34–2.50) |
HIV status | ||
Negative | 4.3 (5/116) | 1.00 |
Positive | 17.1 (24/140) | 3.98 (1.57–10.10) |
Phenotypic DST result | ||
INH and RIF susceptible | 9.5 (21/221) | 1.00 |
INH monoresistance | 27.3 (3/11) | 2.87 (1.01–8.18) |
RIF monoresistance | 25.0 (1/4) | 2.63 (.46–15.07) |
Multidrug resistance | 80.0 (4/5) | 8.42 (4.63–15.31) |
Resistance mutation frequency, % | ||
<0.1 | 9.8 (19/193) | 1.00 |
0.1–4.9 (micro-heteroresistance) | 3.2 (1/31) | 0.33 (.05–2.36) |
5.0–94.9 (macro-heteroresistance) | 0.0 (0/2) | 0.00 (Undefined) |
≥95 (fixed resistance) | 46.7 (7/15) | 4.74 (2.38–9.44) |
Strain heterogeneity | ||
Single strain | 11.0 (19/173) | 1.00 |
Possible mixed strains | 9.1 (6/66) | 0.83 (.35–1.98) |
Mixed strains | 23.8 (5/21) | 2.17 (.90–5.20) |
Characteristic . | Poor Outcome, % (Proportion) . | Risk Ratio (95% CI) . |
---|---|---|
Sex | ||
Male | 9.7 (15/155) | 1.00 |
Female | 14.3 (15/105) | 1.48 (.75–2.89) |
Age, y | ||
<21 | 3.8 (1/26) | 1.00 |
21–30 | 5.5 (4/73) | 1.42 (.17–12.17) |
31–40 | 15.5 (13/84) | 4.02 (.55–29.31) |
41–50 | 17.1 (7/41) | 4.44 (.58–34.03) |
≥51 | 14.7 (5/34) | 3.82 (.48–30.77) |
Prior history of tuberculosis | ||
No | 10.9 (24/220) | 1.00 |
Yes | 15.0 (6/40) | 1.38 (.60–3.15) |
Treatment category | ||
New | 11.7 (26/223) | 1.00 |
Retreatment | 10.8 (4/37) | 0.93 (.34–2.50) |
HIV status | ||
Negative | 4.3 (5/116) | 1.00 |
Positive | 17.1 (24/140) | 3.98 (1.57–10.10) |
Phenotypic DST result | ||
INH and RIF susceptible | 9.5 (21/221) | 1.00 |
INH monoresistance | 27.3 (3/11) | 2.87 (1.01–8.18) |
RIF monoresistance | 25.0 (1/4) | 2.63 (.46–15.07) |
Multidrug resistance | 80.0 (4/5) | 8.42 (4.63–15.31) |
Resistance mutation frequency, % | ||
<0.1 | 9.8 (19/193) | 1.00 |
0.1–4.9 (micro-heteroresistance) | 3.2 (1/31) | 0.33 (.05–2.36) |
5.0–94.9 (macro-heteroresistance) | 0.0 (0/2) | 0.00 (Undefined) |
≥95 (fixed resistance) | 46.7 (7/15) | 4.74 (2.38–9.44) |
Strain heterogeneity | ||
Single strain | 11.0 (19/173) | 1.00 |
Possible mixed strains | 9.1 (6/66) | 0.83 (.35–1.98) |
Mixed strains | 23.8 (5/21) | 2.17 (.90–5.20) |
Abbreviations: CI, confidence interval; DST, drug susceptibility test; HIV, human immunodeficiency virus; INH, isoniazid; RIF, rifampicin.
Characteristic . | Poor Outcome, % (Proportion) . | Risk Ratio (95% CI) . |
---|---|---|
Sex | ||
Male | 9.7 (15/155) | 1.00 |
Female | 14.3 (15/105) | 1.48 (.75–2.89) |
Age, y | ||
<21 | 3.8 (1/26) | 1.00 |
21–30 | 5.5 (4/73) | 1.42 (.17–12.17) |
31–40 | 15.5 (13/84) | 4.02 (.55–29.31) |
41–50 | 17.1 (7/41) | 4.44 (.58–34.03) |
≥51 | 14.7 (5/34) | 3.82 (.48–30.77) |
Prior history of tuberculosis | ||
No | 10.9 (24/220) | 1.00 |
Yes | 15.0 (6/40) | 1.38 (.60–3.15) |
Treatment category | ||
New | 11.7 (26/223) | 1.00 |
Retreatment | 10.8 (4/37) | 0.93 (.34–2.50) |
HIV status | ||
Negative | 4.3 (5/116) | 1.00 |
Positive | 17.1 (24/140) | 3.98 (1.57–10.10) |
Phenotypic DST result | ||
INH and RIF susceptible | 9.5 (21/221) | 1.00 |
INH monoresistance | 27.3 (3/11) | 2.87 (1.01–8.18) |
RIF monoresistance | 25.0 (1/4) | 2.63 (.46–15.07) |
Multidrug resistance | 80.0 (4/5) | 8.42 (4.63–15.31) |
Resistance mutation frequency, % | ||
<0.1 | 9.8 (19/193) | 1.00 |
0.1–4.9 (micro-heteroresistance) | 3.2 (1/31) | 0.33 (.05–2.36) |
5.0–94.9 (macro-heteroresistance) | 0.0 (0/2) | 0.00 (Undefined) |
≥95 (fixed resistance) | 46.7 (7/15) | 4.74 (2.38–9.44) |
Strain heterogeneity | ||
Single strain | 11.0 (19/173) | 1.00 |
Possible mixed strains | 9.1 (6/66) | 0.83 (.35–1.98) |
Mixed strains | 23.8 (5/21) | 2.17 (.90–5.20) |
Characteristic . | Poor Outcome, % (Proportion) . | Risk Ratio (95% CI) . |
---|---|---|
Sex | ||
Male | 9.7 (15/155) | 1.00 |
Female | 14.3 (15/105) | 1.48 (.75–2.89) |
Age, y | ||
<21 | 3.8 (1/26) | 1.00 |
21–30 | 5.5 (4/73) | 1.42 (.17–12.17) |
31–40 | 15.5 (13/84) | 4.02 (.55–29.31) |
41–50 | 17.1 (7/41) | 4.44 (.58–34.03) |
≥51 | 14.7 (5/34) | 3.82 (.48–30.77) |
Prior history of tuberculosis | ||
No | 10.9 (24/220) | 1.00 |
Yes | 15.0 (6/40) | 1.38 (.60–3.15) |
Treatment category | ||
New | 11.7 (26/223) | 1.00 |
Retreatment | 10.8 (4/37) | 0.93 (.34–2.50) |
HIV status | ||
Negative | 4.3 (5/116) | 1.00 |
Positive | 17.1 (24/140) | 3.98 (1.57–10.10) |
Phenotypic DST result | ||
INH and RIF susceptible | 9.5 (21/221) | 1.00 |
INH monoresistance | 27.3 (3/11) | 2.87 (1.01–8.18) |
RIF monoresistance | 25.0 (1/4) | 2.63 (.46–15.07) |
Multidrug resistance | 80.0 (4/5) | 8.42 (4.63–15.31) |
Resistance mutation frequency, % | ||
<0.1 | 9.8 (19/193) | 1.00 |
0.1–4.9 (micro-heteroresistance) | 3.2 (1/31) | 0.33 (.05–2.36) |
5.0–94.9 (macro-heteroresistance) | 0.0 (0/2) | 0.00 (Undefined) |
≥95 (fixed resistance) | 46.7 (7/15) | 4.74 (2.38–9.44) |
Strain heterogeneity | ||
Single strain | 11.0 (19/173) | 1.00 |
Possible mixed strains | 9.1 (6/66) | 0.83 (.35–1.98) |
Mixed strains | 23.8 (5/21) | 2.17 (.90–5.20) |
Abbreviations: CI, confidence interval; DST, drug susceptibility test; HIV, human immunodeficiency virus; INH, isoniazid; RIF, rifampicin.
In multiple logistic regression analysis controlling for age, HIV status, and genetic resistance categories, mixed-strain infections were found to be independently associated with poor outcomes as compared to single-strain infections (odds ratio [OR], 5.42; 95% CI, 1.42–20.63; Table 3). HIV positivity, age, and fixed resistance–associated mutations were also associated with poor outcomes. We observed a lower odds of poor outcomes among patients who had micro-heteroresistant infections, but the 95% CIs for the OR overlapped 1.0 (OR, 0.17; 95% CI, .02–1.52). We excluded patients with macro-heteroresistant infections from this model, owing to the small cell size in this group (n = 2). Sensitivity analyses that combined this group with the micro-heteroresistant category or with the fixed mutations category did not change our findings (Supplementary Table 2). Sensitivity analysis with different definitions of a poor outcome produced imprecise estimates owing to the small sample size, but the patterns of associations were consistent with our primary findings (Supplementary Table 3).
Adjusted Odds Ratios (ORs) for Poor Treatment Outcome, Based on Multiple Logistic Regression Modeling
Characteristic . | Adjusted OR (95% CI) . |
---|---|
Age (per 1-y increase) | 1.03 (.99–1.06) |
HIV status | |
Negative | 1.00 |
Positive | 5.31 (1.69–16.74) |
Resistance mutation frequency (INH or RIF), %a | |
<0.1 | 1.00 |
0.1–4.9 | 0.17 (.02–1.52) |
≥95 | 9.45 (2.73–32.76) |
Strain heterogeneity | |
Single strain | 1.00 |
Possible mixed strains | 0.62 (.2–1.96) |
Mixed strains | 5.42 (1.42–20.63) |
Characteristic . | Adjusted OR (95% CI) . |
---|---|
Age (per 1-y increase) | 1.03 (.99–1.06) |
HIV status | |
Negative | 1.00 |
Positive | 5.31 (1.69–16.74) |
Resistance mutation frequency (INH or RIF), %a | |
<0.1 | 1.00 |
0.1–4.9 | 0.17 (.02–1.52) |
≥95 | 9.45 (2.73–32.76) |
Strain heterogeneity | |
Single strain | 1.00 |
Possible mixed strains | 0.62 (.2–1.96) |
Mixed strains | 5.42 (1.42–20.63) |
Abbreviations: CI, confidence interval; HIV, human immunodeficiency virus; INH, isoniazid; RIF, rifampicin
aPatients with macro-heteroresistance (5.0%–94.9% resistance-associated mutations in at least 1 allele) were excluded from the model because of a small cell size in that category (n = 2).
Adjusted Odds Ratios (ORs) for Poor Treatment Outcome, Based on Multiple Logistic Regression Modeling
Characteristic . | Adjusted OR (95% CI) . |
---|---|
Age (per 1-y increase) | 1.03 (.99–1.06) |
HIV status | |
Negative | 1.00 |
Positive | 5.31 (1.69–16.74) |
Resistance mutation frequency (INH or RIF), %a | |
<0.1 | 1.00 |
0.1–4.9 | 0.17 (.02–1.52) |
≥95 | 9.45 (2.73–32.76) |
Strain heterogeneity | |
Single strain | 1.00 |
Possible mixed strains | 0.62 (.2–1.96) |
Mixed strains | 5.42 (1.42–20.63) |
Characteristic . | Adjusted OR (95% CI) . |
---|---|
Age (per 1-y increase) | 1.03 (.99–1.06) |
HIV status | |
Negative | 1.00 |
Positive | 5.31 (1.69–16.74) |
Resistance mutation frequency (INH or RIF), %a | |
<0.1 | 1.00 |
0.1–4.9 | 0.17 (.02–1.52) |
≥95 | 9.45 (2.73–32.76) |
Strain heterogeneity | |
Single strain | 1.00 |
Possible mixed strains | 0.62 (.2–1.96) |
Mixed strains | 5.42 (1.42–20.63) |
Abbreviations: CI, confidence interval; HIV, human immunodeficiency virus; INH, isoniazid; RIF, rifampicin
aPatients with macro-heteroresistance (5.0%–94.9% resistance-associated mutations in at least 1 allele) were excluded from the model because of a small cell size in that category (n = 2).
DISCUSSION
In our analysis of a population-based sample of patients with tuberculosis, we found that mixed-strain M. tuberculosis infections were associated with increased odds for poor tuberculosis treatment outcomes among patients receiving first-line tuberculosis therapy. Contrary to our expectations, heteroresistance among mixed-strain M. tuberculosis infections did not explain the association between mixed-strain infections and poor outcomes.
Numerous case series have demonstrated that mixed-strain infections involving heteroresistant infections can adversely affect treatment outcomes [3, 9, 14, 34–37]. Heteroresistance may lead to missed detection of the drug-resistant strain, and treating such cases with first-line anti-tuberculosis therapy could lead to the selection and amplification of the drug-resistant strain in the host [3, 8, 14, 34, 35, 37]. A previous study of patients with tuberculosis in Iran found a high risk of poor outcomes among patients harboring mixed-strain infections and heteroresistance defined by phenotypic DST [11]. However, that study did not report an analysis of the independent effects of mixed-strain infections and heteroresistance [11]. Heteroresistant infections may also lead to poor outcomes among patients with MDR tuberculosis, owing to the inefficient treatment of the drug-susceptible strain during second-line MDR tuberculosis therapy [9]. Our present study sought to build on these findings by determining the extent to which undetected genetic heteroresistance affects outcomes among patients with newly diagnosed tuberculosis who are starting first-line therapy in real-life clinical settings. Our findings suggest that, in settings with low levels of resistance to first-line drugs (ie, INH and RIF) among strains circulating in the community, overall heteroresistance prevalence is low, and undetected heteroresistant infections at initial diagnosis may not play a prominent role in driving the negative treatment outcomes associated with mixed-strain M. tuberculosis infections.
Our primary finding that mixed-strain infections were independently associated with an increased risk of poor outcomes is consistent with results of 2 previous studies conducted in Botswana and South Africa [8, 10]. Notably, our previous study of patients with tuberculosis who were evaluated for MDR strains in Botswana found an increased odds of poor tuberculosis treatment outcomes among patients with mixed-strain infections (adjusted OR, 6.5; 95% CI, 2.1–20.5), even after controlling for false-negative results of Xpert testing for RIF resistance [8]. Consistent with the present study, findings from that study suggest that mixed-strain M. tuberculosis infections may affect treatment outcomes through a mechanism independent of missed detection of heteroresistant infections. In contrast, a study of patients with tuberculosis in Uganda found similar rates of treatment response (ie, culture negativity at 2 and 5 months of treatment) between patients with mixed-strain infections and those with single-strain infections [38]. However, that study only included 8 patients with mixed-strain infections, which limited its ability to detect differences in treatment response.
Our findings underscore the importance of improving the understanding of the relationship between mixed-strain infections and poor tuberculosis treatment outcomes. Explanations for this association that do not involve pretreatment heteroresistance include the possibility that mixed-strain infections may be a marker of disease severity and/or pathogen burden. Previous studies have found a higher prevalence of hemoptysis and cavitary tuberculosis among patients with mixed-strain infections at the time of tuberculosis diagnosis [39, 40]. However, inclusion of hemoptysis as a covariate in our final model did not alter our findings (data not shown). Mixed infections may also be a marker of host susceptibility to M. tuberculosis infection and pathology [41]. In fact, our previous study showed a strong association between HIV-associated immune suppression and prevalence of mixed-strain infections [7]. Additional research is needed to unravel the host-related factors associated with mixed-strain infections. Residual confounding may also be responsible for the observed association. For instance, socioeconomic status may increase the risk of repeated exposure to M. tuberculosis leading to mixed-strain infections and also increase the risk of poor outcomes [42]. Alternatively, interactions between distinct M. tuberculosis strains within the host may increase the risk for poor outcomes. For example, it has been shown that mixed-strain infections can be caused by a new infection that triggers the reactivation of a previously acquired infection [43]. At present, little is known regarding mechanisms involving interactions between distinct strains that may lead to a decreased response to tuberculosis medication.
We found a low prevalence of heteroresistance in our study population and, therefore, could not fully examine their impact on treatment outcomes. In particular, only 2 patients (0.8%) were found to harbor macro-heteroresistant infections (RAVs frequency, 5%–94% of reads). While this finding was expected among patients with newly diagnosed tuberculosis due to single-strain infections, we had anticipated higher prevalence of heteroresistance among patients with tuberculosis due to mixed-strain infections, owing to concomitant infection with drug-resistant and drug-susceptible strains [8, 19]. The low prevalence of heteroresistance in our study suggests that baseline heteroresistance may not play a significant role in tuberculosis outcomes among patients with newly diagnosed tuberculosis at the population level in Botswana. Future studies should examine these associations in populations with higher rates of primary transmission of drug-resistant tuberculosis, where the prevalence of pretreatment heteroresistance may be higher.
Strengths of our study include the composition of the sample population, which was drawn from a population-based study of patients with culture-confirmed tuberculosis who were recruited from routine clinical settings [26]. Our data consisted of patients with mixed-strain infections and genetic heteroresistance, determined using 2 genotyping methods (MIRU-VNTR and next-generation targeted deep sequencing, respectively), allowing us to evaluate the extent to which each of these genotypes affects tuberculosis outcomes. We used a state-of-the-art sequencing and bioinformatics approach that has been validated for quantifying low-frequency minority RAVs [20, 21]. Our findings were robust to sensitivity analyses for analyzing macro-heteroresistant infections and varying definitions of treatment outcome.
Our findings should be interpreted with consideration of the following limitations. Our study focused on resistance to INH and RIF, as they are the cornerstone of first-line tuberculosis therapy. However, we were not able to determine the clinical effect of undetected heteroresistance to other first-line drugs. Likewise, previous studies focused on the importance of heteroresistance occurring during treatment of patients with MDR tuberculosis or extensively drug-resistant tuberculosis [20–22, 44]. It is likely that heteroresistance plays a more important role in patients with MDR tuberculosis who are already receiving second-line and tertiary therapies. Our study involved MIRU-VNTR and deep sequencing performed on M. tuberculosis DNA extracted from primary cultures. Previous studies have shown that the competitive growth inherent to the culture process affects the composition of the infecting M. tuberculosis population, which could have led to missed detection of heteroresistance and mixed-strain infections [44–46].
Our study population consisted of patients with tuberculosis who initiated first-line tuberculosis therapy, and treatment regimens were modified for some patients according to the baseline DST results. Therefore, different definitions were used for treatment success, based on the regimen administered to the patient. We also did not have data on the cause of death. While we used the conventional definition of defining death as death due to any cause during treatment, some of the deaths in our study may not have been directly related to tuberculosis. Finally, while the sequencing and bioinformatics approach used in this study has been designed to accurately detect very-low-frequency (<0.1%) minority RAVs, sequencing error leading to misclassification of micro-heteroresistant infections cannot be ruled out [47].
In conclusion, we present data from a population-based molecular epidemiologic study that demonstrate an association between mixed-strain M. tuberculosis infections and poor tuberculosis treatment outcomes. We did not find evidence that heteroresistant infections were responsible for this observed association. Future studies should be done to replicate our findings in settings where drug-resistant tuberculosis is more prevalent. Furthermore, additional studies that examine the clinical impact of heteroresistance determined from M. tuberculosis DNA extracted directly from clinical specimens could provide further insights into the clinical impact of heteroresistant infections.
Supplementary Data
Supplementary materials are available at The Journal of Infectious Diseases online. Consisting of data provided by the authors to benefit the reader, the posted materials are not copyedited and are the sole responsibility of the authors, so questions or comments should be addressed to the corresponding author.
Notes
Acknowledgments. We thank the study participants and their families; the Kopanyo team, including Alyssa Finlay, Eleanor S. Click, Patrick K. Moonan, John E. Oeltmann, Matsiri Ogopotse, Othusitse Fane, Mbatshi Dima, Rosanna Boyd, Tsaone Tamuhla, and Joyce Basotli; and Eric Lehmkuhl and Kristin Wiggins, for their valuable technical support in the genomics laboratory.
Disclaimer. The funders had no role in study design, data collection and analysis, decision to publish, or preparation of the manuscript. The content is solely the responsibility of the authors and does not necessarily represent the official views of the National Institutes of Health.
Financial support. This work was supported by the National Institutes of Health (grants R01AI097045, P30AI45008, K01AI118559, and R21AI120838).
Potential conflicts of interest. All authors: No reported conflicts of interest. All authors have submitted the ICMJE Form for Disclosure of Potential Conflicts of Interest. Conflicts that the editors consider relevant to the content of the manuscript have been disclosed.