-
PDF
- Split View
-
Views
-
Cite
Cite
Stephen R. Walsh, Zoe Moodie, Andrew J. Fiore-Gartland, Cecilia Morgan, Marissa B. Wilck, Scott M. Hammer, Susan P. Buchbinder, Spyros A. Kalams, Paul A. Goepfert, Mark J. Mulligan, Michael C. Keefer, Lindsey R. Baden, Edith M. Swann, Shannon Grant, Hasan Ahmed, Fusheng Li, Tomer Hertz, Steven G. Self, David Friedrich, Nicole Frahm, Hua-Xin Liao, David C. Montefiori, Georgia D. Tomaras, M. Juliana McElrath, John Hural, Barney S. Graham, Xia Jin, for the HVTN 083 Study Group and the NIAID HVTN, Magda Sobieszczyk, Jonathan D. Fuchs, Theresa Wagner, G. Kyle Rybczyk, Aeryn Peck, Turner Overton, C. Mhorag Hay, Catherine A. Bunce, Jon A. Gothing, Jane A. Kleinjan, Bryce Manso, Raphael Dolin, Yehuda Z. Cohen, Jennifer A. Johnson, for the HVTN 083 Study Group and the NIAID HVTN, Vaccination With Heterologous HIV-1 Envelope Sequences and Heterologous Adenovirus Vectors Increases T-Cell Responses to Conserved Regions: HVTN 083, The Journal of Infectious Diseases, Volume 213, Issue 4, 15 February 2016, Pages 541–550, https://doi.org/10.1093/infdis/jiv496
- Share Icon Share
Abstract
Background. Increasing the breadth of human immunodeficiency virus type 1 (HIV-1) vaccine-elicited immune responses or targeting conserved regions may improve coverage of circulating strains. HIV Vaccine Trials Network 083 tested whether cellular immune responses with these features are induced by prime-boost strategies, using heterologous vectors, heterologous inserts, or a combination of both.
Methods. A total of 180 participants were randomly assigned to receive combinations of adenovirus vectors (Ad5 or Ad35) and HIV-1 envelope (Env) gene inserts (clade A or B) in a prime-boost regimen.
Results. T-cell responses to heterologous and homologous insert regimens targeted a similar number of epitopes (ratio of means, 1.0; 95% confidence interval [CI], .6–1.6; P = .91), but heterologous insert regimens induced significantly more epitopes that were shared between EnvA and EnvB than homologous insert regimens (ratio of means, 2.7; 95% CI, 1.2–5.7; P = .01). Participants in the heterologous versus homologous insert groups had T-cell responses that targeted epitopes with greater evolutionary conservation (mean entropy [±SD], 0.32 ± 0.1 bits; P = .003), and epitopes recognized by responders provided higher coverage (49%; P = .035). Heterologous vector regimens had higher numbers of total, EnvA, and EnvB epitopes than homologous vector regimens (P = .02, .044, and .045, respectively).
Conclusions. These data demonstrate that vaccination with heterologous insert prime boosting increased T-cell responses to shared epitopes, while heterologous vector prime boosting increased the number of T-cell epitopes recognized.
Clinical Trials Registration. NCT01095224.
An effective vaccine against human immunodeficiency virus type 1 (HIV-1) infection remains an elusive goal, and substantial intraclade and interclade viral genetic diversity poses a remarkable challenge for immunogen design [1, 2]. Control of viral replication following HIV-1 infection has been correlated with CD8+ T-cell recognition of specific epitopes [3, 4], yet targeting of other epitopes appears to have no effect on viral replication [5]. Although vaccine-elicited T-cell responses have not been effective in preventing HIV-1 acquisition or promoting control of viremia [6–8], possibly because of an insufficient number of targeted epitopes [9], they have been found to exert selective pressure on incoming viruses [10], and increased breadth of vaccine-induced Gag-specific T cells is associated with a reduced viral load [11].
Vaccination often results in high-magnitude responses to a small number of immunodominant epitopes, with only weak responses induced against subdominant epitopes [12]. A meta-analysis of HIV-1 vaccine–elicited cellular immune responses induced by recombinant adenoviral (rAd) vectors found that T-cell epitopes clustered into immunodominant hotspots, many of which targeted highly variable regions of the viral genome [13]. Many concepts have been proposed to increase the breath (ie, number of epitopes) and the depth (ie, variant coverage within an epitope) of vaccine-elicited T-cell responses [14] to circumvent the mechanisms underlying immunodominance [15–17]. As T-cell responses directed against highly conserved epitopes may allow immune control of diverse viral isolates, vaccination with divergent immunogens has been suggested as a strategy to elicit immune responses against shared epitopes [14]. Additionally, prime-boost regimens with heterologous antigens may selectively expand cross-reactive T cells capable of recognizing >1 variant of a given epitope.
Viral vectors have been among the leading candidates for HIV-1 vaccines, owing to their safety, tolerability, and immunogenicity [18, 19]. Heterologous prime boosting with different viral vectors may circumvent antivector immune responses and allow boosting of responses to the vaccine immunogen [20].
We hypothesized that sequential heterologous prime-boost immunization with 2 HIV-1 envelope (env) genes derived from different clades would elicit more-robust immune responses overall and elicit T-cell responses that focused on more-conserved epitopes, compared with those elicited by homologous prime-boost vaccination, thus providing greater coverage of viral diversity. We also hypothesized that heterologous prime-boost vaccinations with distinct serotypes of adenoviruses would minimize interference by antivector responses, leading to enhanced T-cell responses versus those induced by homologous vector prime-boost regimens. We therefore performed a clinical trial in which participants were randomly assigned to one of 5 different combinations of adenovirus vectors (rAd serotypes 5 [rAd5] and 35 [rAd35]) and HIV-1 Env–derived immunogens from clades A (EnvA) and B (EnvB).
MATERIALS AND METHODS
Please see the Supplementary Materials for details of the Materials and Methods used in this study.
Vaccines
The vaccines used in this study were VRC-HIVADV038-00-VP (rAd5-EnvA), VRC-HIVADV052-00-VP (rAd5-EnvB), and VRC-HIVADV027-00-VP (rAd35-EnvA).
Study Design
Vaccines were allocated on the basis of a randomized, double-blind design and administered intramuscularly at a dose of 1 × 1010 particle units (PU) at months 0 and 3 (Table 1). All participants received vaccine, as there was no placebo group. A total of 180 participants were enrolled into the 5 groups, consisting of 30 or 45 vaccine recipients; product availability limited the Ad35-EnvA vector groups to 30 recipients. The study was approved by the local institutional review boards and institutional biosafety committees at all participating sites, and written informed consent was obtained from all participants.
Group . | Number . | Month 0 (Day 0) . | Month 3 (Day 84) . |
---|---|---|---|
A | 30 | 1 × 1010 PU rAd35 EnvA | 1 × 1010 PU rAd5 EnvA |
B | 30 | 1 × 1010 PU rAd35 EnvA | 1 × 1010 PU rAd5 EnvB |
C | 30 | 1 × 1010 PU rAd35 EnvA | 1 × 1010 PU rAd35 EnvA |
D | 45 | 1 × 1010 PU rAd5 EnvA | 1 × 1010 PU rAd5 EnvA |
E | 45 | 1 × 1010 PU rAd5 EnvA | 1 × 1010 PU rAd5 EnvB |
Total | 180 | … | … |
Group . | Number . | Month 0 (Day 0) . | Month 3 (Day 84) . |
---|---|---|---|
A | 30 | 1 × 1010 PU rAd35 EnvA | 1 × 1010 PU rAd5 EnvA |
B | 30 | 1 × 1010 PU rAd35 EnvA | 1 × 1010 PU rAd5 EnvB |
C | 30 | 1 × 1010 PU rAd35 EnvA | 1 × 1010 PU rAd35 EnvA |
D | 45 | 1 × 1010 PU rAd5 EnvA | 1 × 1010 PU rAd5 EnvA |
E | 45 | 1 × 1010 PU rAd5 EnvA | 1 × 1010 PU rAd5 EnvB |
Total | 180 | … | … |
Abbreviations: HIV, human immunodeficiency virus; PU, particle units.
Group . | Number . | Month 0 (Day 0) . | Month 3 (Day 84) . |
---|---|---|---|
A | 30 | 1 × 1010 PU rAd35 EnvA | 1 × 1010 PU rAd5 EnvA |
B | 30 | 1 × 1010 PU rAd35 EnvA | 1 × 1010 PU rAd5 EnvB |
C | 30 | 1 × 1010 PU rAd35 EnvA | 1 × 1010 PU rAd35 EnvA |
D | 45 | 1 × 1010 PU rAd5 EnvA | 1 × 1010 PU rAd5 EnvA |
E | 45 | 1 × 1010 PU rAd5 EnvA | 1 × 1010 PU rAd5 EnvB |
Total | 180 | … | … |
Group . | Number . | Month 0 (Day 0) . | Month 3 (Day 84) . |
---|---|---|---|
A | 30 | 1 × 1010 PU rAd35 EnvA | 1 × 1010 PU rAd5 EnvA |
B | 30 | 1 × 1010 PU rAd35 EnvA | 1 × 1010 PU rAd5 EnvB |
C | 30 | 1 × 1010 PU rAd35 EnvA | 1 × 1010 PU rAd35 EnvA |
D | 45 | 1 × 1010 PU rAd5 EnvA | 1 × 1010 PU rAd5 EnvA |
E | 45 | 1 × 1010 PU rAd5 EnvA | 1 × 1010 PU rAd5 EnvB |
Total | 180 | … | … |
Abbreviations: HIV, human immunodeficiency virus; PU, particle units.
T-Cell Interferon γ (IFN-γ) Enzyme-Linked Immunospot (ELISPOT) Assay
Ex vivo HIV-1–specific T-cell responses were assessed with a validated IFN-γ ELISPOT assay, using cryopreserved peripheral blood mononuclear cells stimulated overnight with synthetic peptide pools corresponding to EnvA and EnvB, as described elsewhere [21]. All immunogenicity assays were performed on samples obtained at baseline (day 0) and day 112, which corresponds to 4 weeks after the second vaccination.
Intracellular Cytokine Staining (ICS)
Flow cytometry was used to examine the CD4 and CD8 specificity of the HIV-1–specific positive ELISPOT responses, using a validated ICS assay with EnvA and EnvB peptide pools [22].
T-Cell Epitope Mapping
For each participant, we identified the epitopes that were elicited by the vaccine, based on the ELISPOT responses to individual 15mer EnvA and EnvB peptides. Response positivity was determined using the mixture models for single-cell assays (MIMOSA) method [23]; MIMOSA is a Bayesian hierarchical mixture model framework in which one component models the responders and the other component models the nonresponders [23].
Multiclade Alignment for Assessing Epitope Entropy and Coverage
To assess the potential for a given epitope or a participant's set of epitopes to provide coverage of a population of circulating HIV-1 strains, we obtained a representative set of viral sequences from the Los Alamos National Laboratory (LANL) HIV database. The entropy of amino acid frequencies was computed for each site in the representative alignment, using the Shannon entropy equation [24, 25]. Epitope coverage was defined as the fraction of viruses in the representative set whose sequence matched that of the aligned epitope sequence.
Binding and Neutralizing Antibody Assays
Serum antibodies to HIV-1 gp120/gp140 proteins and V1V2 antigens were measured by a custom binding antibody multiplex assay [26–29], and neutralizing antibody (NAb) titers were measured using a luciferase-based assay, as described previously [30].
Statistical Analyses
To address the study hypotheses, we prespecified groups of regimens for comparisons. Heterologous and homologous vector regimens were compared; heterologous and homologous insert regimens were also compared. Kruskal–Wallis nonparametric 1-way analysis of variance was used to assess continuous characteristics among the groups. Fisher exact tests were used to compare proportions between 2 groups. Wilcoxon rank sum tests were used to compare response magnitudes between groups. The Lachenbruch test statistic was used to evaluate the composite null hypothesis of equal immune response rates between 2 groups and equal response distributions among responders in those groups [31]. Poisson regression was used to model the counts of epitope responses (total, EnvA, EnvB, and shared) with robust standard errors. Two-sided 95% confidence intervals (CIs) for binomial proportions were calculated using the score test method of Agresti and Coull [32]. The Holm adjustment for multiple comparisons was used in testing for group differences in the binding antibody responses across multiple antigens. All tests were 2 sided, and P values were considered statistically significant at the .05 level.
RESULTS
Participant Characteristics, Safety and Reactogenicity, and Adverse Events
Of the 180 participants enrolled (Supplementary Table 1) at 9 sites in the United States, 77 (43%) were female, 117 (65%) were non-Hispanic and white, and the median age was 25.5 years (range, 18–50 years). Study conduct and safety assessments are detailed in the Supplementary Materials, in Supplementary Figure 2, and in Supplementary Figure 3.
T-Cell Responses Detected by IFN-γ ELISPOT at the Peptide Pool Level
ELISPOT response rates to EnvA were greater than those to EnvB (Figure 1). Within the heterologous insert groups, 65.2% and 43.3% of participants responded to the EnvA and EnvB peptide pools, respectively. The EnvB responders were essentially a subset of the EnvA responders, with only 1 participant responding to EnvB and not EnvA. Within the homologous insert group (the Ad35-EnvA/Ad5-EnvA and Ad5-EnvA/Ad5-EnvA groups combined), the response rate to EnvA and EnvB was 65.3% and 19.4%, respectively, with no participants responding only to EnvB. Although the response rates and magnitudes among responders to either peptide pool were similar in both the heterologous and homologous insert groups (P = .47, by the Lachenbruch test), we noted that the response rate to EnvB was significantly higher in the heterologous insert group, composed of the Ad35-EnvA/Ad5-EnvB and Ad5-EnvA/Ad5-EnvB groups combined (P = .003, by the Fisher exact test; Supplementary Table 2A), suggesting that regimens containing EnvB elicited greater EnvB-specific epitopes without increasing the overall number of responders. The ELISPOT response rate and magnitudes among responders to either peptide pool was significantly greater for the heterologous (74.6%) versus the homologous (63.3%) vector regimens (P = .03, by the Lachenbruch test; Supplementary Table 2B). This difference was also seen when comparing the groups with respect to the EnvA and EnvB pool responses, but it did not reach statistical significance (P = .05, by the Lachenbruch test, and P = .08, by the Fisher exact test, respectively; Supplementary Table 2B).
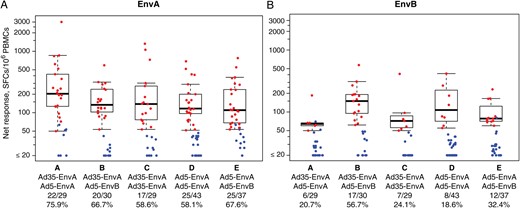
Vaccination with heterologous human immunodeficiency virus type 1 (HIV-1) env sequences and heterologous adenovirus vectors elicited T-cell responses in human volunteers. Cellular immune responses were quantified with an interferon γ enzyme-linked immunospot assay, using autologous peptide pools derived from the EnvA insert (A) and the EnvB insert (B). Positive responders are shown in red and negative responders in blue, with background responses (to medium alone) subtracted. Box plots show the distribution of responses among the positive responders only. The box indicates the median and interquartile range (IQR); the whiskers extend to 1.5 times the IQR from the upper or lower quartile. The response rates are indicated along the x-axis. Abbreviations: PBMC, peripheral blood mononuclear cell; SFC, spot-forming cell.
T-Cell Responses Detected by ICS
ICS was used to identify the phenotype of vaccine-elicited T cells in participants with a significant fraction of IFN-γ– and/or interleukin 2–expressing cells in response to EnvA or EnvB peptide pools. For all treatment groups and for both EnvA and EnvB peptide pools, CD8+ T-cell response rates tended to be higher than CD4+ T-cell response rates. Please see the Supplementary Materials, Supplementary Figure 4, and Supplementary Table 2 for details.
Epitope Mapping by IFN-γ ELISPOT
T-cell responses were measured following stimulation with individual 15mer peptides derived from vaccine-matched EnvA and EnvB sequences (Figure 2). Each vaccine recipient responded to a mean of 1.38 peptides (95% CI, 1.09–1.72; range, 0–12). The mean number of responses was greater for participants receiving the heterologous vector regimens (2.0 [95% CI, 1.3–2.9] for Ad35-EnvA/Ad5-EnvA recipients and 2.0 [95% CI, 1.2–3.3] for Ad35-EnvA/Ad5-EnvB recipients versus 1.1 [95% CI, .5–1.8] for Ad35-EnvA/Ad35-EnvA recipients, 0.8 [95% CI, .4–1.3] for Ad5-EnvA/Ad5-EnvA recipients, and 1.3 [95% CI, .76–2.09] for Ad5-EnvA/Ad5-EnvB recipients; P = .01, by Poisson regression). Owing to the 11–amino acid overlap of consecutive peptides, it was common for several overlapping 15mers to elicit responses, defining a response region that could indicate an underlying epitope. From the set of 15mers that elicited positive responses in each participant, we used computational HLA-A and HLA-B binding predictors and the extent of the overlap to determine the minimal set of underlying CD8+ T-cell epitopes that could best explain all of the 15mer responses. This analysis led to estimates of breadth (ie, number of epitopes) for each vaccine recipient, ranging from 0 to 7. The mean number of epitopes for each vaccine group was 1.43 (95% CI .93–2.07) for Ad35-EnvA/Ad5-EnvA recipients, 1.03 (95% CI, .6–1.63) for Ad35-EnvA/Ad5-EnvB recipients, 0.63 (95% CI, .3–1.03) for Ad35-EnvA/Ad35-EnvA recipients, 0.6 (95% CI, .33–.93) for Ad5-EnvA/Ad5-EnvA recipients, and 0.82 (95% CI, .49–1.33) for Ad5-EnvA/Ad5-EnvB recipients (Figure 3).

Map of CD8+ T-cell epitopes elicited by vaccination. Two sets of overlapping peptides (EnvA and EnvB) were used to map the enzyme-linked immunospot assay responses of each participant to a single 15mer peptide. The frequency of responses to each peptide were computed for each treatment group and plotted according to their start position in the human immunodeficiency virus type 1 envelope protein.
![Epitope conservation analyses. For each participant, the epitopes underlying the observed responses were determined using 2 sets of criteria, one based on the entire overlapping region of 15mer responses and the second based on predicted HLA binding. A, An example of the 2 criteria shows the mean number of observed responses or underlying epitopes for participants in the Ad35-EnvA/Ad5-EnvB group (± standard error of the mean [SEM]). Responses were to either an EnvA (blue) or EnvB (red) 15mer peptide, whereas epitopes could additionally be attributed to overlapping EnvA and EnvB peptides (“shared”). The number of amino acid mismatches (MM) between the overlapping peptides is indicated by the color of the stacked bars. B, Comparison of epitopes determined using the HLA criteria for participants in each treatment group (± SEM). Regimens using both clades induced a greater proportion of epitopes that are shared between the 2 clades.](https://oup.silverchair-cdn.com/oup/backfile/Content_public/Journal/jid/213/4/10.1093_infdis_jiv496/2/m_jiv49603.jpeg?Expires=1747921142&Signature=lMjtjfdNMgA-~IGtTPrRhdGIHSisDJ9g-GKAOQpQiJE2DqNh-kUP41FmVx1IhVJDl1zN9ewnMSxCMshFk29CnzZAJ68TQ0aUoD2HjHASXDtRBFaYbH58LsK7EfLQaDQ~1YNdGBb4qWm2scN7nQ3kCL54oTcmy0Cld5qxuSfNMItNhAXLICRX2oykASuoBjIyzyZ3Wgz-wBbFNf~BHmlonFGoHjtoLQJeO7lYF-Fkocrn3qvYy8ycam2N9Fw31ffM8wnQzGrqwT8KgfCW3eYi6tu0LZE9IFX~mlEpIrhgdaV0gtcD15fWDC~7AQDIbiHLSz1dJtgX2mg-kwwRNtXL-g__&Key-Pair-Id=APKAIE5G5CRDK6RD3PGA)
Epitope conservation analyses. For each participant, the epitopes underlying the observed responses were determined using 2 sets of criteria, one based on the entire overlapping region of 15mer responses and the second based on predicted HLA binding. A, An example of the 2 criteria shows the mean number of observed responses or underlying epitopes for participants in the Ad35-EnvA/Ad5-EnvB group (± standard error of the mean [SEM]). Responses were to either an EnvA (blue) or EnvB (red) 15mer peptide, whereas epitopes could additionally be attributed to overlapping EnvA and EnvB peptides (“shared”). The number of amino acid mismatches (MM) between the overlapping peptides is indicated by the color of the stacked bars. B, Comparison of epitopes determined using the HLA criteria for participants in each treatment group (± SEM). Regimens using both clades induced a greater proportion of epitopes that are shared between the 2 clades.
Shared responses were defined as responses to regions that were conserved (defined as ≤2 amino acid mismatches) between EnvA and EnvB (Figure 3). Heterologous vector regimens had higher numbers of EnvA, EnvB, and total epitopes than homologous vector regimens (P = .044, .045, .02, respectively; Figure 4A and Supplementary Table 2); responses to shared epitopes were also higher but not significantly so (P = .07, by Poisson regression). Heterologous and homologous insert regimens elicited responses to similar total numbers of epitopes (ratio of means, 1.0; 95% CI, .6–1.6; P = .91, by Poisson regression), but heterologous insert regimens targeted a greater number of epitopes that were shared between EnvA and EnvB, compared with homologous insert regimens (ratio of means, 2.7; 95% CI, 1.2–5.7; P = .01, by Poisson regression; Figure 4B).
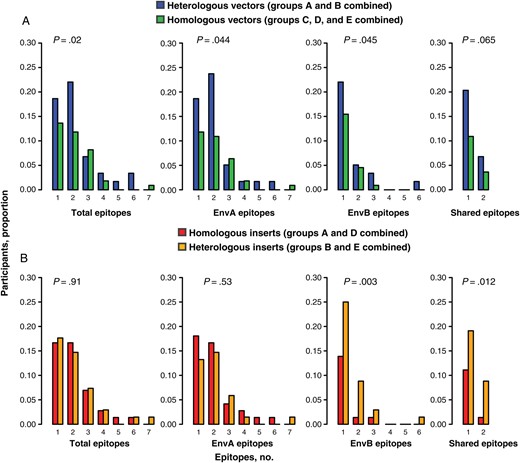
Comparison of the number of epitopes recognized by heterologous versus homologous vector regimens and by heterologous versus homologous insert regimens. A, Heterologous vector regimens induced significantly higher numbers of total, EnvA, and EnvB epitopes than homologous vector regimens. B, Heterologous insert regimens induced significantly higher numbers of EnvB and shared epitopes than homologous insert regimens.
Epitope Entropy and Coverage
The degree to which a vaccine-elicited epitope is conserved may be critical, as highly conserved epitopes may be more likely to cover a broader set of HIV-1 isolates and possibly less vulnerable to viral escape. Participants who received an EnvB insert targeted a greater number of epitopes that were shared between EnvA and EnvB, despite only small differences in the total number of epitopes targeted. While this finding may indicate a T-cell response with broader specificities, it could also suggest that the response was focused on more-conserved regions of Env. To distinguish these possibilities, we assessed the evolutionary conservation and viral coverage of the epitopes elicited by using a representative set of 1569 HIV-1 clade A and clade B envelope sequences that were added to the LANL database in 2012. The level of conservation of each epitope was assessed by averaging the per-site entropy over the alignment for every site spanned by the epitope (higher conservation means lower entropy; Figure 5A). To ensure that the conservation of larger repertoires was not artifactually lower, we defined repertoire conservation as that of the single most conserved epitope and found that epitope conservation was significantly higher for participants who responded to the heterologous insert regimen (mean entropy [±SD], 0.32 ± 0.05 bits) as compared to those who responded to the homologous insert regimen (mean entropy [±SD], 0.68 ± 0.1 bits; P = .003). However, because this is an analysis of participants with at least 1 epitope recognized (due to exclusion of nonresponders), there may be postrandomization selection bias. No difference in epitope conservation was observed in comparisons of participants in the heterologous versus homologous vector groups (P = .86).
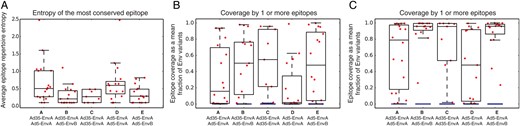
Sequential boosting with heterologous inserts improves targeting of conserved regions of human immunodeficiency virus type 1 envelope protein. A, Entropy and coverage of vaccine-elicited epitopes. Repertoire entropy was defined to be the single most-conserved epitope for each responder, where entropy of each epitope was computed as the mean site-wise entropies, given the amino acid distributions in the Los Alamos National Laboratory (LANL)–derived set of 1569 sequences uploaded in 2012. B, Repertoire coverage for each responder is defined as the fraction of sequences in the LANL-derived set that match exactly at least 1 of their epitopes. C, Relaxed definition of repertoire coverage tolerates 1 amino acid mismatch between an epitope and a sequence for it to be considered covered. For each regimen, the box indicates the interquartile range (IQR) and the median, with whiskers extending to the lowest and highest observation within 1.5 × IQR.
We also performed an epitope coverage analysis under the hypothesis that a cellular immune response can only be effective against strains of HIV-1 that match the vaccine-induced epitope. We defined the coverage of a participant's repertoire to be the fraction of viruses in the LANL-derived set whose aligned sequence matched that of at least 1 of the epitopes in the repertoire. As there were many more clade B (n = 1361) than clade A (n = 208) viruses in the LANL-derived set, we weighted each sequence according to the inverse frequency of its clade in the set. Overall, epitope coverage was heterogeneous, with some epitopes matching 100% and others matching <0.1% of sequences in the set. We considered treatment group differences in repertoire coverage by imputing 0% coverage to the nonresponders in each group. Although the heterologous insert regimens elicited higher coverage repertoires (20%; Figure 5B) than the homologous insert regimens (12%), the difference was not significant (P = .25). Repertoire coverage was significantly greater for participants who received a heterologous vector regimen (23%), compared with a homologous vector regimen (13%; P = .02).
While an analysis of all participants provides an unbiased comparison of the randomized treatment arms, an analysis of responders may help separate the coverage analysis from the observed differences in response rates among the groups. Among responders, the epitope coverage was significantly greater for those who received a heterologous (49%) versus a homologous (28%) insert regimen (P = .035). However, among responders, differences in the epitope coverage provided by the heterologous versus homologous vector regimens were not significant (P = .90). This suggests that, while overall coverage is greater for heterologous vector regimens, the effect may be driven by a higher response rate and greater number of epitopes, as opposed to a change in the composition of the epitopes that were targeted.
Relaxing the definition of a matching epitope to allow for at least 1 mismatched amino acid exaggerated the comparison of the heterologous versus homologous insert regimens. By this definition there was a significant difference (P = .01) in coverage between participants who received a heterologous (37%) versus a homologous (24%) insert. This definition also exaggerated the difference that was observed in the response-conditioned analysis (88% vs 55%; P = .003; Figure 5C).
Humoral Immune Responses
Binding antibody responses were assessed to determine whether heterologous vector-insert regimens would increase the frequency or breadth of responses (Figure 6 and Supplementary Figure 5). For antigens A1.con.env03 140 CF, B.con.env03 140 CF, C.con.env03 140 CF, Con S gp140 CFI, and gp41, the overall response rates were very high, generally exceeding 80%. We did not observe any significant differences in the magnitudes or response rates among the responders when comparing participants receiving heterologous versus homologous vector regimens (P > .13 for all comparisons, after the Holm adjustment for multiplicity; Supplementary Table 3A). However, binding antibody responses were considerably lower for participants who received the EnvB insert as part of a heterologous insert regimen, likely owing to the excision of the V2 region from the EnvB insert [33]. Participants receiving the EnvB insert had lower responses (P < .001 for all comparisons, after the Holm adjustment for multiplicity) for all antigens except gp41 (P = .407; Supplementary Table 3B).
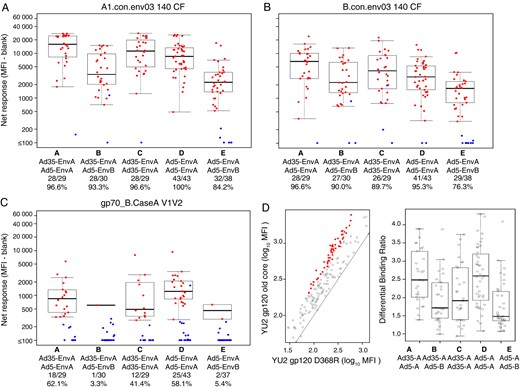
Prime-boost using heterologous env sequences does not augment antibody responses. Binding antibody responses were measured by an human immunodeficiency virus type 1 (HIV-1) envelope binding antibody multiplex assay using a consensus clade A envelope (A), a consensus clade B envelope (B), and the V1V2 variable region (C). Antibodies specific for the CD4 binding site were assessed by differential binding to the clade B isolate YU2 gp120 old core and the D368R mutant (D). Positive responders are shown in red and negative responders in blue (A, B, and C), with background responses (to medium alone) subtracted. Boxplots show the distribution of responses among the positive responders only. The box indicates the median and interquartile range (IQR); the whiskers extend to 1.5 times the IQR from the upper or lower quartile. The response rates are indicated along the x-axis. Abbreviation: MFI, mean fluorescence intensity.
Binding immunoglobulin A (IgA) antibody responses and NAb responses were assessed to determine whether heterologous vector/insert regimens would increase the frequency or breadth of responses. IgA binding responses were generally low but highest to Con S gp140 CFI. NAb responses were very weak overall and almost exclusively targeted the highly sensitive clade C MW965.26 virus. Please see the Supplementary Materials for details.
DISCUSSION
Our study tested the hypothesis that T-cell coverage could be improved by prime-boost injections with heterologous vectors and/or heterologous inserts. We found that both the T-cell response rate and the number of epitopes elicited were influenced by heterologous boosting and that the resulting coverage of viral diversity was higher for both heterologous vector and heterologous insert regimens. Our data therefore suggest that vaccination strategies directed at improving T-cell breadth and coverage should use heterologous vectors and inserts.
The heterologous insert groups (EnvA-EnvB) had response rates similar to those of the EnvA-EnvA homologous insert groups, although their rate of response to the EnvB peptide pool was greater. More importantly, however, when ELISPOT responses were mapped to individual epitopes, heterologous insert prime boosting increased T-cell responses to shared epitopes. The epitopes targeted by heterologous insert regimens were more conserved and provided a greater depth of coverage of viral diversity, as computed using a representative set of clade A and B sequences. Heterologous vector regimens (Ad35-Ad5) also elicited epitope repertoires with greater coverage than homologous vector regimens (Ad5-Ad5 or Ad35-Ad35), although our results suggest that this effect was mediated by higher response rates and a greater number of total epitopes. This is supported by the lack of difference in epitope conservation and in epitope coverage if only responders were analyzed.
To assess epitope conservation for each participant, we assumed that only their single most-conserved epitope would be relevant to the effectiveness of their T-cell response [34–36]. This assumption reduced the risk of underestimating conservation if a participant had 1 highly conserved epitope but several additional nonconserved epitopes. For heterologous insert regimens, the increased recognition of highly conserved epitopes led to improved coverage of the representative set of clade A and clade B viruses. Increased T-cell responses to conserved epitopes may ultimately translate into a greater depth of coverage of globally circulating viruses across other clades. Furthermore, as epitopes shared between the clade A– and clade B–derived inserts are generally conserved across clades, sequential prime boosting with heterologous immunogens may be an effective strategy for focusing the CD8+ T-cell response on evolutionarily conserved epitopes that are functionally constrained.
Although it is likely that responses directed toward a highly conserved epitope could provide deeper coverage of circulating viruses, we estimated coverage directly by comparing the EnvA and EnvB sequences of vaccine-elicited epitopes to clade A and clade B sequences in the LANL database. In many cases, an epitope and a mismatched sequence will only differ at a single amino acid residue; if a conservative amino acid substitution has minimal impact on HLA or T-cell receptor binding, it is possible that the mismatched sequence could still be recognized. Our initial calculations could underestimate epitope coverage, and we therefore examined a relaxed definition of matching to tolerate 1 amino acid mismatch. Although our proportional weighting of clade A and clade B sequences does not represent any actual geographical locale, it provides an unbiased way to test the theoretical hypothesis that is implicit in a trial of a EnvA/EnvB heterologous insert regimen. We also observed qualitatively similar results when we evaluated epitope coverage against only the clade A sequences in the LANL database (data not shown), supporting the hypothesis that differences in epitope coverage can be attributed to differences in epitope conservation.
While we found that EnvA priming (either with an Ad5 or Ad35 vector) followed by EnvB boosting (with an Ad5 vector) elicited an increased frequency of shared epitopes, the absolute number of targeted epitopes was low, and the absolute increase in the number of shared epitopes elicited by the heterologous insert regimens was quite modest. Several strategies to increase the breadth and depth of cellular immune responses have been proposed, including computationally derived mosaic antigens [37, 38] and conserved sequence antigens [39, 40], and early phase clinical trials of these concepts have begun [41].
In this study, we measured the antibody responses that were elicited by the various prime-boost regimens. Response rates were high against clade A, clade B, clade C, and group M consensus envelopes, as well as against gp41, for all regimens. CD4 binding site (CD4BS) antibodies were induced with the highest levels seen in the Ad35-EnvA/Ad5-EnvA and Ad5-EnvA/Ad5-EnvA homologous insert groups (48.3% and 51.2%, respectively). However, CD4BS immunoglobulin G (IgG) binding antibodies with specificity similar to the broad NAb VRC01 [42] (RSC/RSCΔ371 differential binding) were not significantly induced. We also examined antibody responses to V1V2 antigens since V1V2-specific IgG levels correlated with a decreased risk of HIV-1 infection in the RV144 trial [26, 28]. Similar to the CD4BS antibody responses, V1V2 IgG levels were highest in the homologous EnvA insert groups. The heterologous insert regimens had the lowest levels of V1V2 IgG responses, likely because of the deletion of the V2 loop in the EnvB immunogen [33, 43].
Our findings therefore highlight 4 key concepts. First, prime-boost regimens of adenovirus-vectored HIV-1 envelope, including heterologous Ad5/Ad35 vectors and EnvA/EnvB inserts, are well tolerated and immunogenic in people. Heterologous vector pairs were associated with higher T-cell response rates and induced significantly higher numbers of total T-cell epitopes, compared with homologous vector pairs. Heterologous insert pairs induced significantly higher numbers of shared epitopes than homologous insert pairs. Finally, both a greater number of epitopes and a greater number of shared epitopes could potentially lead to broader and deeper coverage of circulating strains of HIV-1. These candidate regimens exhibited limited T-cell breadth overall, and, given the potential risks of Ad5-vectored HIV vaccines observed in efficacy trials [44, 45], it is unlikely that these vaccines will be advanced. However, our findings provide guiding principles about prime-boost strategies that will be valuable in the design of future vaccine strategies.
STUDY GROUP MEMBERS
In addition to the authors of this article, members of the HVTN 083 study group consist of Magda Sobieszczyk, Jonathan D. Fuchs, Theresa Wagner, the Bridge HIV Vaccine Team, G. Kyle Rybczyk, Aeryn Peck, Turner Overton, C. Mhorag Hay, Catherine A. Bunce, Jon A. Gothing, Jane A. Kleinjan, Bryce Manso, Raphael Dolin, Yehuda Z. Cohen, and Jennifer A. Johnson.
Notes
Acknowledgments. We thank Adam Sherwat, Elizabeth Noonan, Laura Novik, Cynthia S. Hendel, Eva A. Chung, Marcel Curlin, Stephen DeRosa, Vicki Ashley, and Judith Lucas, for their invaluable assistance in this study; Dr John R. Mascola (VRC) and Dr Barton F. Haynes (Duke University), for generously providing Env antigens for our antibody assays; Dr Marcella Sarzotti-Kelsoe, for quality assurance and quality control oversight; Dr Lawrence Corey, for helpful comments on the manuscript; the study participants who volunteered to participate in this study, for their contribution; and the dedicated staff at the HVTN clinical sites and affiliated laboratories who made the study possible.
Disclaimer. This article was written by some authors (E. M. S. and B. S. G.) in their capacity as employees of the National Institutes of Health (NIH), but the views expressed herein do not necessarily represent those of the NIH.
Financial support. This work was supported by the National Institute of Allergy and Infectious Diseases and the National Center for Advancing Translational Sciences (US Public Health Service grants UM1 AI068614 [to HVTN Core FHCRC], UM1 AI068635 [to SCHARP], UM1 AI068618 [to HVTN Laboratory program FHCRC], UM1 AI069452 [to UAB], UM1AI069418 [to Emory], UM1 AI069511 [to Rochester], UM 1AI069412 and UL 1RR025758 [to Harvard], UM1 AI069470 [to Columbia], UM1 AI069439 and UL1 TR000445 [to Vanderbilt], and UM1 AI069496 [to SFDPH]).
Potential conflicts of interest. P. A. G. has received a research grant from Hemispherx Biopharma to conduct a clinical trial with an influenza vaccine. All other authors report no potential conflicts. All authors have submitted the ICMJE Form for Disclosure of Potential Conflicts of Interest. Conflicts that the editors consider relevant to the content of the manuscript have been disclosed.
References
Author notes
Presented in part at: AIDS Vaccine 2013, Barcelona, Spain, 7–10 October 2013. Abstract P04.39 LB.
Present affiliations: Division of Infectious Diseases, University of Pennsylvania, Philadelphia (M. B. W.); Chinese Institute for Cancer and Infectious Diseases, Shenzhen (F. L.), Department of Microbiology, Immunology, and Genetics, Ben-Gurion University of the Negev, Beer-Sheva, Israel and Viral Disease (T. H.); and Vaccine Translational Research Unit, Institut Pasteur of Shanghai, Chinese Academy of Sciences, Shanghai, China (X. J.).