-
PDF
- Split View
-
Views
-
Cite
Cite
Fernando Bril, Uche Ezeh, Mina Amiri, Sana Hatoum, Lauren Pace, Yen-Hao Chen, Fred Bertrand, Barbara Gower, Ricardo Azziz, Adipose Tissue Dysfunction in Polycystic Ovary Syndrome, The Journal of Clinical Endocrinology & Metabolism, Volume 109, Issue 1, January 2024, Pages 10–24, https://doi.org/10.1210/clinem/dgad356
- Share Icon Share
Abstract
Polycystic ovary syndrome (PCOS) is a complex genetic trait and the most common endocrine disorder of women, clinically evident in 5% to 15% of reproductive-aged women globally, with associated cardiometabolic dysfunction. Adipose tissue (AT) dysfunction appears to play an important role in the pathophysiology of PCOS even in patients who do not have excess adiposity.
We undertook a systematic review concerning AT dysfunction in PCOS, and prioritized studies that assessed AT function directly. We also explored therapies that targeted AT dysfunction for the treatment of PCOS.
Various mechanisms of AT dysfunction in PCOS were identified including dysregulation in storage capacity, hypoxia, and hyperplasia; impaired adipogenesis; impaired insulin signaling and glucose transport; dysregulated lipolysis and nonesterified free fatty acids (NEFAs) kinetics; adipokine and cytokine dysregulation and subacute inflammation; epigenetic dysregulation; and mitochondrial dysfunction and endoplasmic reticulum and oxidative stress. Decreased glucose transporter-4 expression and content in adipocytes, leading to decreased insulin-mediated glucose transport in AT, was a consistent abnormality despite no alterations in insulin binding or in IRS/PI3K/Akt signaling. Adiponectin secretion in response to cytokines/chemokines is affected in PCOS compared to controls. Interestingly, epigenetic modulation via DNA methylation and microRNA regulation appears to be important mechanisms underlying AT dysfunction in PCOS.
AT dysfunction, more than AT distribution and excess adiposity, contributes to the metabolic and inflammation abnormalities of PCOS. Nonetheless, many studies provided contradictory, unclear, or limited data, highlighting the urgent need for additional research in this important field.
Polycystic ovary syndrome (PCOS) affects 5% to 15% of women depending on the definition applied (1, 2), making it the most common endocrine-metabolic disorder in females, and a significant economic and public health burden (3). This disorder is most clearly manifested during the reproductive years and is characterized by hyperandrogenism (HA), chronic ovulatory dysfunction (OD), and polycystic ovary morphology (PCOM). The clinical presentation of PCOS can be classified phenotypically into 4 types, A through D: Phenotype A is HA + OD + PCOM; phenotype B is HA + OD; phenotype C is HA + PCOM; and phenotype D is PCOM + OD (4, 5).
Several studies have indicated that PCOS women demonstrate insulin resistance (IR) in target tissues (eg, skeletal muscle, adipose tissue [AT], liver), and more than 65% to 95% of women with PCOS also demonstrate measurable compensatory hyperinsulinemia, above and beyond that determined by their degree of obesity (6, 7). This dysfunction increases the risk for type 2 diabetes mellitus (T2DM) (8) and metabolic syndrome (9), both of which are strong risk factors for cardiovascular disease (10). Although the etiology of this disorder is incompletely understood, in the simplest of terms, PCOS appears to be the result of increased stimulation of androgen biosynthesis (ie, by hyperinsulinism and/or exaggerated pituitary luteinizing hormone [LH] secretion), when other permissive factors (eg, hyperresponsiveness of ovarian theca androgen biosynthesis) are present (Fig. 1).
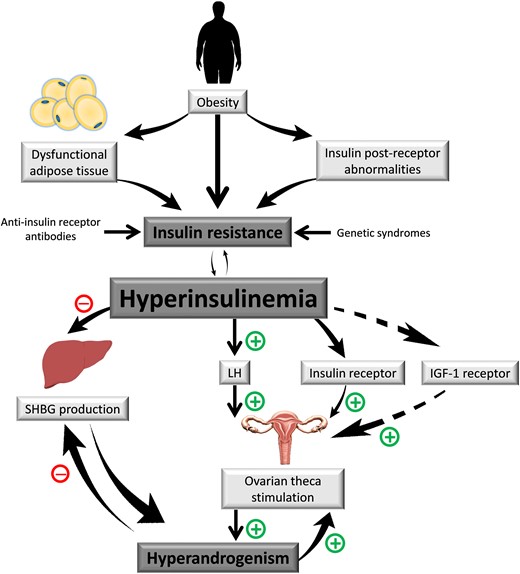
Schematic of polycystic ovary syndrome metabolic pathophysiology.
The global prevalence and burden of obesity continues to rise dramatically globally, despite the availability of novel therapeutic modalities for its treatment (11, 12). Obesity has been historically associated with PCOS. Moreover, in clinical populations, PCOS has been reported in up to 65% patients seen (13, 14). However, while it is clear that obesity exacerbates the reproductive and cardiometabolic morbidities in PCOS and other populations (8–10), recent studies indicate that the perceived increased prevalence of obesity in PCOS is, in great part, likely to be due to referral bias (13–15). Furthermore, longitudinal cross-sectional studies indicate that the increased prevalence of obesity in PCOS appears to reflect the increasing prevalence of obesity in the general population and not the PCOS phenotype itself (16).
AT plays a critical role in the maintenance of metabolic homeostasis and systemic insulin sensitivity, mediated by the secretion of adipocytokines and other secretory proteins with a wide range of endocrine and paracrine functions, via insulin-mediated glucose transport into adipocytes, and by regulating nonesterified free fatty acid (NEFA) storage, release, and circulatory levels. While both obesity and AT dysfunction are intricately linked with the development of metabolic diseases, prior work, including that of the present investigators, supports a role for AT dysfunction in the metabolic and reproductive features of PCOS, independent of excess adiposity (17–22). In this mini-review, we summarize our current understanding concerning AT dysfunction in PCOS.
Adipose Tissue Dysfunction in Polycystic Ovary Syndrome
We undertook a systematic review of the literature (see Fig. 2 and Supplementary Table S1 (23)), and prioritized studies that assessed AT function directly.
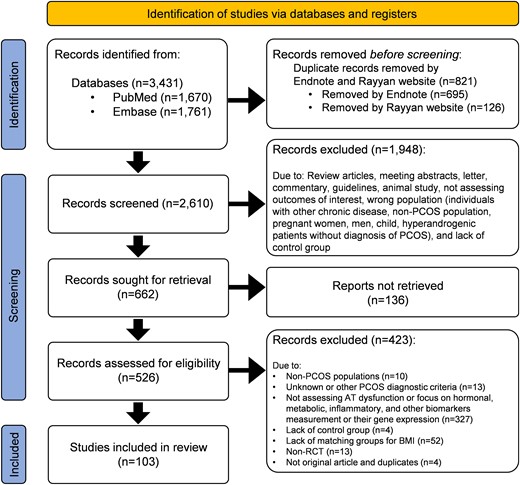
PRISMA (Preferred Reporting Items for Systematic Review and Meta-Analysis) flow diagram of systematic review. PubMed and Embase were systematically searched to retrieve human studies assessing adipose tissue dysfunction in patients with polycystic ovary syndrome (PCOS) published until November 2022 without language limitations. The search strategy for each database can be found in Supplementary Table S1 (23). The references of all included studies were also reviewed for any additional publications missed with the original search. Observational and interventional studies were eligible for inclusion if they assessed adipose tissue function in PCOS patients diagnosed by the 1990 National Institutes of Health, the 2003 Rotterdam, and/or the 2006 Androgen Excess & PCOS Society (AE-PCOS) criteria and compared results against body mass index (BMI)-matched controls without PCOS. Studies with other designs such as reviews, meta-analyses, or letters, animal studies, studies including hyperandrogenic or amenorrheic women without defined PCOS, and those without matched-BMI controls were excluded from the study. Search strategy and study identification were performed by 2 reviewers (M.A. and S.H.) using a standardized protocol. Any disagreement in the study selection was resolved by other reviewers (F.B. and R.A.). All investigators extracted data from relevant articles. Out of 3431 records retrieved from searching databases, 103 original articles were selected for the review.
What Is Adipose Tissue Dysfunction?
AT is a dynamic endocrine organ that has a key effect on glucose and lipid metabolism. In addition to adipocytes, AT is also composed of macrophages and other stromal cells, which play an important role in inflammation and cytokine release. Therefore, when AT becomes dysfunctional, a cascade of metabolic derangements is triggered, with systemic repercussions (24). The exact mechanisms that initiate AT dysfunction have not been well established. Indeed, these mechanisms may be considerably different depending on whether AT dysfunction is being promoted by genetic, epigenetic, and/or environmental factors.
While a wide variety of insults have been identified as responsible for promoting AT dysfunction, regardless of the initial insult, AT inflammation and decreased insulin sensitivity appear to be common final pathways. These, in turn, result in adipokine dysregulation and increased release of proinflammatory cytokines and NEFAs, which play a key role in the associated systemic manifestations. Therefore, in broad terms, we defined AT dysfunction as any change in AT anatomy, morphology (cellular size or number), histology, or physiology that led to decreased insulin response, dysregulation of adipokine secretion, and/or increased inflammation. Fig. 3 summarizes how different processes/pathways in AT may contribute to its dysfunction in women with PCOS.
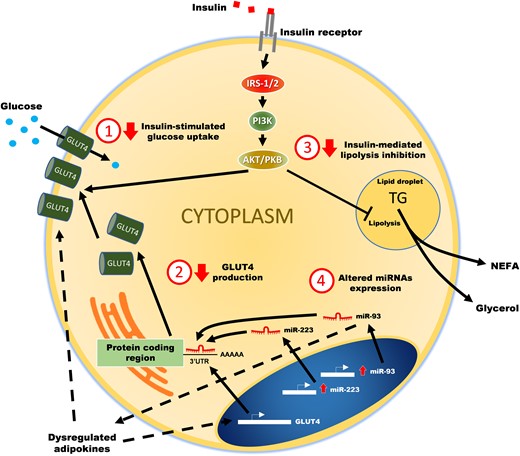
Potential mechanisms leading to adipose tissue dysfunction in polycystic ovary syndrome.
Subcutaneous vs Visceral Adipose Tissue
The distribution of adiposity is at least as important as total body fat, which likely stems from the fact that, depending on its location, AT has different functions and metabolic effects. Visceral AT (VAT) is defined as the intra-abdominal AT bound by parietal peritoneum or transversalis fascia, which surrounds organs such as the stomach, bowels, and liver. VAT is more metabolically active, has higher rates of lipolysis, and is more insulin resistant than subcutaneous AT (SAT) (25). In line with this, VAT volume is considered to correlate better than SAT volume with metabolic derangements and cardiovascular disease, although the difference is less significant in women (26). Of note, the stronger metabolic effect of VAT occurs despite the fact that it represents only 5% to 8% of total adiposity in women (27). In a study by Dumesic et al (21), nonobese PCOS patients had increased VAT, and this finding correlated with their androgen levels and the degree of IR. However, because VAT expands in the setting of a complex metabolic disbalance leading to concomitant ectopic fat accumulation in other organs, such as the liver, skeletal muscle, and pancreas, it is difficult to determine if VAT per se is a driver of the metabolic abnormalities or just a confounding factor.
More recently, the relative importance of VAT vs SAT in mediating metabolic function has been questioned. Using frequently sampled IV glucose tolerance tests (FSIVGTTs) and single-slice computed tomography (CT) of the abdomen, we observed that insulin sensitivity in PCOS was primarily associated with SAT and not VAT (17). Indeed, other investigators comparing VAT to abdominal SAT have reported that both correlated similarly (and independently) to insulin sensitivity measured by euglycemic hyperinsulinemic clamp (28, 29). Additionally, Barber et al (30) found that after matching for body mass index (BMI) and fat mass, fat distribution measured by magnetic resonance imaging was similar in patients with and without PCOS, despite higher IR in the PCOS group. Mannerås-Holm et al (31) also observed that, despite higher waist-to-hip ratio, no differences were seen in abdominal/visceral fat by magnetic resonance imaging between PCOS and controls. Studies assessing the relationship between hyperandrogenemia and VAT have also reported equivocal results (32, 33). These data support the concept that AT dysfunction, more than AT distribution, contributes to the metabolic abnormalities in PCOS.
Mechanisms of Adipose Tissue Dysfunction
Dysregulation in adipose tissue storage capacity and adipose tissue hypoxia
Adipocyte hypertrophy occurs as an adaptative response to caloric excess, helping to prevent ectopic fat accumulation and lipotoxicity. In support of this concept, lean individuals with smaller adipocytes have a worse metabolic response and ectopic lipid accumulation in response to overfeeding relative to those with larger adipocytes (34). Based on this “AT expandability” hypothesis, AT dysfunction occurs once AT capacity for fat storage reaches its limit (35). Because this “capacity” varies from individual to individual, metabolic dysfunction can occur with different levels of adiposity, thus explaining why not all obese individuals are metabolically challenged.
In animal and humans, adipocyte hypertrophy correlates with metabolic abnormalities (36). In women with PCOS, subcutaneous adipocytes were larger than in BMI-matched controls and adipocyte volume showed the strongest negative correlation with insulin sensitivity, even after adjustment for other important factors, such as waist circumference, BMI, androgens, and adiponectin (31). Other studies assessing adipocyte volume also found larger cells in patients with PCOS compared to BMI-matched controls, which correlated with the degree of HA (32).
The mechanisms leading from adipocyte hypertrophy to cellular dysfunction are unknown. One widespread hypothesis is that adipocyte hypertrophy triggers cellular hypoxia by increasing the distance that oxygen must diffuse through and by inducing a relative reduction in blood flow in the AT, as vasculature is unable to expand proportionally to the adipocyte expansion (37). Indeed, studies in humans have confirmed lower oxygen pressures in AT of overweight/obese patients compared to lean individuals, as well as lower capillary density in abdominal SAT (38). Nevertheless, when IR and insulin-sensitive obese individuals (based on results of FSIVGTT) were compared, oxygen pressure in abdominal SAT obtained by open incision was not significantly different in the 2 groups (39). Despite similar oxygenation, hypoxia-induced genes, such as HIF-1α and VEGF-A, were upregulated in patients with IR (39). Alternatively, no differences were observed in hypoxia target genes in a different study using AT obtained by liposuction and comparing overweight/obese patients vs lean controls (38).
In summary, it is still not clear if differences in AT oxygen pressure are responsible for AT dysfunction or just related to total adiposity. Moreover, specific studies on the role of tissue hypoxia in PCOS women are lacking and its contribution to chronic inflammation in this disorder is currently unknown.
Adipose tissue hyperplasia and impaired adipogenesis
In addition to adipocyte size and hypertrophy, the total number of adipocytes and the capacity to recruit more adipocytes (ie, hyperplasia) also appear to play an important role in AT homeostasis (40). In simple terms, hyperplasia counterregulates hypertrophy (ie, if there are more adipocytes available to store fat, this results in smaller and healthier adipocytes). In mice, Kim et al (41) measured adipogenesis by infusing stable isotopes and found a decreased capacity of adipogenesis in obese animals. In a PCOS ovine model, prenatal androgen excess was associated with reduced adipogenesis in adolescence, which in turn was associated with metabolic abnormalities typical of PCOS (42). In primary human adipocyte cultures obtained from surgical biopsies, androgens inhibit adipose stem cell commitment to preadipocytes (43), as well as preadipocyte differentiation into adipocytes (44). Moreover, in a small study comparing PCOS women with age- and BMI-matched controls, newly differentiated adipocytes showed higher lipid content in PCOS women, which correlated with testosterone levels, although this relationship was affected by insulin levels (45).
While reduced adipogenesis is associated with metabolic abnormalities, the opposite is also true. Measuring insulin sensitivity by FSIVGTT, Dumesic et al (46) reported that increased adipogenesis was associated with increased insulin sensitivity in PCOS women. In obese women undergoing bariatric surgery, AT hyperplasia (both VAT and abdominal SAT) was associated with better insulin sensitivity and lower insulin levels, despite similar BMI, total adiposity, and fat distribution (47). In summary, data suggest that metabolic dysfunction in PCOS may be associated with decreased adipogenic capacity which, in turn, may be linked to HA.
Impaired insulin signaling and glucose transport in adipose tissue
In simple terms, insulin binding to its receptor (InsR) leads to tyrosine phosphorylation of InsR and other endogenous substrates, including insulin receptor substrates (IRS)-1 and IRS-2, which in turn activate the phosphatidyl-inositol 3-kinase and protein kinase B (Akt) cascade (the IRS/PI3K/Akt pathway) that eventually leads to glucose transporter-4 (GLUT-4) translocation and enhanced glucose uptake (see Fig. 3). While tyrosine phosphorylation activates InsR and the subsequent signaling pathway, simultaneous serine phosphorylation serves to dampen InsR and IRS activation and subsequent signaling, essentially bringing the activation brought on by the binding of insulin to the InsR to a close.
Activation of the IRS/PI3K/Akt signaling pathway also results in other important metabolic consequences in the AT, such as lipogenesis, inhibition of lipolysis, and glycogen synthesis (48). In women with PCOS, several studies have shown defects in insulin receptor activation (49) and signaling (50, 51) in the fibroblasts and skeletal muscle. However, this has not been the case in the AT, for which studies have observed no differences in InsR phosphorylation or proteins (52) or messenger RNA (53) in the IRS/PI3-K/Akt pathway. Despite the absence of differences in the IRS/PI3-K/Akt pathway, differences in GLUT-4 expression and protein levels have been reported in PCOS (16, 18, 54, 55).
When we assessed the IRS/PI3-K/Akt pathway signaling components in the abdominal SAT of 21 PCOS and 20 controls (55), only GLUT-4 expression was significantly lower in PCOS patients and in control individuals with IR. We also did not observe any difference in InsR serine or tyrosine phosphorylation at baseline or after activation, as had been reported previously in skin fibroblasts of PCOS women (49) (Fig. 4). As no changes have been reported in the IRS/PI3-K/Akt pathway in AT of PCOS women, changes in GLUT-4 expression are likely determined by other mechanisms (eg, see role of epigenetic factors discussed later). It is also possible that the IRS/PI3-K/Akt pathway may undergo posttranslational regulation, which would not affect protein or messenger RNA levels. This mechanism of regulation has indeed been observed in patients with PCOS, for example, in the rate of phosphorylation of glycogen synthase kinase-3β (56).
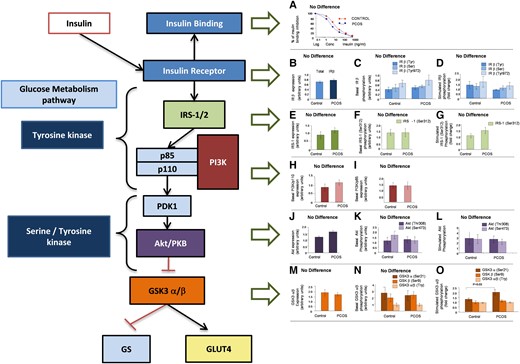
PI3K/Akt insulin signaling cascade. No obvious defect in insulin binding, or insulin receptor (InsR) or PI3K/AKT insulin-signaling pathway activity was observed in the adipose tissue (AT) of polycystic ovary syndrome (PCOS) women (based on data from Chen et al (55)).
Regardless of the specific mechanisms leading to impaired insulin action, there is consensus that AT of women with PCOS demonstrates impaired insulin-mediated glucose uptake due to decreased GLUT-4 and increased rates of lipolysis, compared to non-PCOS patients (18, 19), as also seen in patients with T2DM and nonalcoholic fatty liver disease. Reduced GLUT-4 expression in AT is associated with IR in patients with or without PCOS (20), which is in line with observations from adipocyte-specific knock-down GLUT-4 mice (57).
Because AT accounts for only approximately 10% of glucose uptake in the body, the association between decreased GLUT-4 expression and whole-body IR appears to be via indirect mechanisms. For example, GLUT-4 expression in AT affects the expression of an AT-specific isoform of carbohydrate-responsive element binding protein (ChREBP)-β. This enzyme activates de novo lipogenesis (DNL) in AT, and in humans has been associated with enhanced glucose tolerance and insulin sensitivity (58). While the exact mechanisms linking ChREBP in AT to insulin sensitivity are unclear, the relationship appears to be dependent on the DNL rate (58). It is possible that specific NEFAs derived from AT DNL have important signaling functions that affect other metabolic processes, such as the recently described branched fatty acid esters of hydroxy-fatty acids (FAHFAs) (59) (see lipolysis section).
In addition to enhanced glucose transport and inhibited lipolysis, the interaction of insulin with its receptor stimulates cell growth and differentiation through the mitogen-activated protein kinase (MAPK)-ERK1/2 pathway, independently of the of IRS/PI3K/Akt cascade (48). Interestingly, IR can selectively affect one of these pathways without affecting the other one (60). Indeed, despite decreased GLUT-4, patients with PCOS seem to exhibit constitutively activated MAPK-ERK1/2 pathway in skeletal muscle (61) and AT (62) compared to patients without PCOS. Interestingly, MAPK-ERK1/2 activation has multiple downstream targets, one of which is the serine phosphorylation of IRS-1, leading to a reduction in the activity of the IRS/PI3K/Akt pathway. This appears to be a common occurrence in all IR states, including also obesity and T2DM (60).
In summary, it appears that PCOS is associated with decreased GLUT-4 expression and content in adipocytes, leading to decreased insulin-mediated glucose transport in AT. This abnormality does not seem to be directly related to alterations in insulin binding or in the IRS/PI3K/Akt signaling pathway in AT. Additionally, increased MAPK-ERK1/2 activity may contribute to some of the systemic hypertrophic effects (acanthosis nigricans, ovarian thecal hyperplasia, etc) and the reduced glucose transport observed in the disorder.
Dysregulated lipolysis
Increased lipolysis in AT plays a key role in the crosstalk between AT dysfunction and the rest of the body. Excess supply of NEFAs to other organs results in ectopic fat accumulation and lipotoxicity (63). The term lipotoxicity was originally introduced to describe the effects of fat accumulation in pancreatic β cells; however, it has lately been used to refer to any deleterious effects resulting from abnormal fat accumulation in ectopic tissues, such as the liver, skeletal and myocardial muscle, pancreas, and kidney. AT dysfunction leads to ectopic fat accumulation in different tissues, which in turn become IR, further contributing to the metabolic abnormalities seen.
Evidence of increased lipolysis in PCOS is not uniform. Using well-established surrogate markers of IR in AT in the fasting (ie, Adipo-IR: fasting insulin × NEFAs) and postprandial (ie, NEFA nadir during an FSIVGTT) states, we observed that patients with PCOS demonstrate worse AT IR compared to BMI-matched controls (20). However, in a different cohort of individuals, fasting NEFA was actually lower and calculated maximal rates of lipolysis similar in PCOS compared to controls (19). Other investigators noted that whole-body fasting lipolysis, measured by [2H5]-glycerol turnover, was lower in obese girls with PCOS compared to non-PCOS patients, despite significantly reduced suppression of lipolysis during insulin infusion in patients with PCOS compared to controls (64). Using plasma glycerol as a biomarker of lipolysis, Mannerås-Holm et al (31) found no difference between adult women with and without PCOS. Moreover, nonobese PCOS patients without IR demonstrated reduced glycerol levels compared to non-PCOS individuals (65). These conflicting data regarding the rates of lipolysis in PCOS is likely due to the multifactorial regulation of AT lipolysis. Moreover, data from patients with PCOS suggest that abdominal SAT and VAT regulate lipolysis differently (66).
We should note that increased NEFAs in isolation is likely not enough to induce ectopic fat accumulation, as hyperinsulinemia appears to be a crucial factor. For example, plasma insulin levels have been shown to be tightly correlated to the amount of liver fat accumulation in patients with or without PCOS (67, 68). In nonobese PCOS patients with relatively normal insulin sensitivity and more prominent HA, the effect of androgens on SAT is likely to prevail, impairing catecholamine-stimulated lipolysis through reduced levels of β2-adrenergic receptor, hormone-sensitive lipase, and protein kinase A regulatory-IIβ component (PKA-RegIIβ) (65). Alternatively, in overweight/obese PCOS, where IR predominates and where VAT may be more prominent, lipolysis is increased by the impaired suppression of lipolysis by insulin, the higher lipolytic rates of VAT, and the relative resistance of VAT to the action of androgens (66). In summary, while data regarding AT lipolysis in PCOS are still limited, current evidence suggests that women with the disorder have defective NEFA kinetics, in part related to hyperinsulinism, HA, and body fat distribution.
Adipokine dysregulation
AT is responsible for the secretion of a number of cytokines and adipokines with key local and distant physiologic functions (24). Adiponectin, resistin, leptin, lipocalin 2, tumor necrosis factor-α (TNF-α), interleukin-6 (IL-6), angiopoietin-like protein 2, visfatin, and retinol-binding protein 4, are likely among the best characterized adipokines. Our systematic review indicated that most of the studies focusing on AT function in PCOS assessed AT function indirectly by measuring one or more of these adipokines in the circulation. Of note, many of these cytokines are not exclusively secreted by AT (69), and therefore their circulating levels could be substantially influenced by other tissues. Nonetheless, there is evidence that patients with PCOS have dysregulated adipokine and cytokine secretion compared to BMI-matched controls (69, 70).
Adiponectin has been extensively studied in patients with PCOS, and while a relationship is undeniable, we still do not completely understand the nature or magnitude of this relationship. Polymorphisms in the ADIPOQ gene were associated with risk of PCOS in a meta-analysis, suggesting a causal relationship (71). However, adiponectin levels were found to depend more on obesity than on the presence of PCOS in a group of 120 patients (72). Among lean patients (ie, BMI <25), adiponectin levels were similar in patients with or without PCOS (73, 74). These results were in agreement with some (75, 76), but not all (77), studies assessing AT adiponectin expression. A meta-analysis that included 30 studies with a total of 2565 samples found significantly lower levels of adiponectin in nonobese PCOS patients when compared to controls, despite significant heterogeneity among studies (78).
It is also possible that the response of this adipokine to regulatory factors may differ. In one study using AT samples obtained by open biopsy (Fig. 5), we observed that while the baseline secretion of adiponectin by isolated adipocytes did not differ between PCOS and control samples, the net change in adiponectin secretion in response to IL-6, monocyte chemoattractant protein-1 (MCP-1), and TNF-α differed between PCOS (decreasing) and control (increasing) adipocytes, although the difference reached significance only for TNF-α (70). Coculture of isolated adipocytes and AT macrophages (ATMs) resulted in a decrease in adiponectin secretion in PCOS but not control adipocytes, and the difference between the net change in adiponectin secretion in PCOS vs control samples was significant. In addition to adiponectin levels, adiponectin receptor levels also appear to be distinctively regulated in AT of women with PCOS. While sex steroids appear to upregulate adiponectin receptors 1 and 2 (AdipoR1 and AdipoR2) in AT (79), the opposite effect has been described for hyperinsulinemia in nonobese patients with PCOS (80).
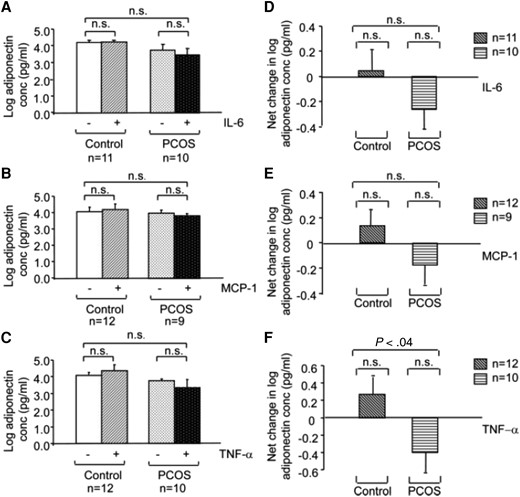
Effect of interleukin-6 (IL-6), monocyte chemoattractant protein 1 (MCP-1), and tumor necrosis factor-α (TNF-α) on adiponectin secretion in adipose tissue of polycystic ovary syndrome (PCOS) and control women. The graphs depict the log scale means of the absolute adiponectin levels in picograms per milliliter (panels A to C) and the net changes in log adiponectin concentration (panels D to F) secreted by PCOS and control adipocytes in response to incubation with 10 ng/mL IL-6 (A and D), MCP-1 (B and E), and TNF-α (C and F). All values were log-transformed before analysis. Sample numbers (n) per group are indicated for each experiment. Reproduced from Chazenbalk G, et al Regulation of adiponectin secretion by adipocytes in the polycystic ovary syndrome: role of tumor necrosis factor-{alpha}. J Clin Endocrinol Metab. 2010 Feb; 95(2):935-42 with permission from Oxford University Press (70).
In summary, results suggested that adiponectin secretion by adipocytes in response to cytokines/chemokines, and most notably in response to coculturing with ATMs, differs between PCOS and controls, favoring greater suppression of adiponectin in PCOS. Whether adiponectin dysregulation in PCOS is inherent or the result of other factors, such as excess adiposity or IR remains unclear. Despite numerous studies, these have had conflicting results and heterogeneity is high. The same can be said about most, if not all, of the other adipokines studied. In the meta-analysis mentioned earlier (78), the investigators reported increased levels of leptin, chemerin, visfatin, and resistin among nonobese PCOS patients compared to controls, but no association for vaspin, omentin, irisin, or apelin. A comprehensive review on the available data for each individual adipokine can be found elsewhere (81).
Cytokine dysregulation and subacute inflammation in adipose tissue
AT dysfunction in PCOS is also characterized by abnormal cytokine release, primarily by ATMs, contributing to systemic subclinical inflammation. In turn, subclinical inflammation in PCOS appears to be related to IR and HA (69). While a large number of studies have compared inflammatory markers in the circulation in patients with PCOS against BMI-matched controls, this approach cannot estimate the real contribution to circulatory cytokines from AT as compared to that of other tissues. When focusing on AT, Huang et al (82) observed increased inflammatory ATMs in SAT based on detection of CD11c and α5 (ie, an integrin present in ATMs) in women with PCOS. They also demonstrated higher crown-like structures (ie, ATMs engulfing adipocytes). The contribution to AT inflammation by PCOS per se, independently of obesity and IR, remains unclear, with some studies suggesting that the observed changes are driven primarily by obesity and not PCOS (83).
The direction of the relationship between AT dysfunction and inflammation is another area of extensive debate: Do inflammatory changes induce IR or does IR induce tissue inflammation? A potential mechanism linking all these processes could start with AT hypertrophy leading to tissular hypoxia as previously discussed. This would eventually lead to the activation of nuclear factor κB, which promotes expression of inflammatory mediators, such as TNF-α, ILs, VCAM1, MCP-1, and transforming growth factor-β (69). These, in turn, would contribute to IR. For example, TNF-α has been found to induce serine phosphorylation of IRS-1 with a consequent decrease in GLUT-4 expression, which in turn could lead to IR (84). However, the opposite is also possible, as excess NEFAs, observed in IR states, can lead to the generation of reactive oxygen species (ROS) (through incomplete β-oxidation or lipid intermediates) and trigger inflammation. Overall, the mechanisms underlying the subacute inflammatory state of PCOS, including the contribution of AT and ATMs to this, independent of other factors such as obesity, remain unclear.
Epigenetic dysregulation of adipose tissue function
DNA methylation, an epigenetic mechanism that regulates gene expression, has been assessed in the AT of patients with PCOS (85). In a pilot study we observed that the percentage of global methylation of peripheral blood DNA was not significantly altered in 20 women with PCOS compared with 20 controls (86). In another study, we hypothesized that perturbation of the epigenome, through altered DNA methylation, was one of the mechanisms whereby prenatal androgenization (PA) reprograms rhesus monkeys to develop PCOS-like features (87). Our results indicated that PA may modify DNA methylation patterns both in infant and adult VAT, suggesting that excess fetal androgen exposure in female nonhuman primates may predispose to PCOS via alteration of the epigenome.
We also measured DNA methylation and gene expression in SAT biopsies to identify PCOS-specific alterations and observed reduced methylation in the LHCGR locus and increased methylation in the INSR locus, changes that are concordant with the altered gene expression profiles observed (88). Complex patterns of methylation quantitative trait loci and expression quantitative trait loci were identified in these loci, suggesting that local genetic variation plays an important role in gene regulation. These data suggested that nonobese PCOS women possess significant alterations in LH receptor expression, which drives excess androgen secretion from the ovary. Alternatively, obese women with PCOS possess alterations in InsR expression, with underexpression in metabolic tissues and overexpression in the ovary, resulting in peripheral IR and excess ovarian androgen production.
Consistent with these findings, a genome-wide DNA methylation study in AT of patients with PCOS indicated that a significant number of DNA sites were distinctively methylated compared to controls (85). Moreover, these changes in methylation correlated with a differential transcriptional regulation of genes involved in inflammation, adipogenesis, and metabolism. Interestingly, methylation in AT was found to be reduced 2 years after bariatric surgery compared to AT from BMI-matched, never-obese individuals (89). Changes in AT DNA methylation have also been reported after electroacupuncture in PCOS (90), although these changes did not remain statistically significant after adjusting for multiple comparisons.
Epigenetic regulation of AT can also occur via microRNAs (miRNAs), short, noncoding RNAs involved in posttranscriptional regulation of gene expression, and that are known to influence many cellular functions, including glucose and lipid metabolism. IR adipocytes are known to contain a differentially expressed miRNA profile (91). In women with PCOS, multiple miRNAs were found to be differentially expressed in AT compared to BMI-matched controls (55), although these results have not been uniform in all studies (92).
We observed that miR-93 was overexpressed in AT of patients with PCOS, as well as in control patients with IR (55). In vitro studies in human adipocytes confirmed that miR-93 targets the GLUT-4 gene, and that its levels were significantly associated with decreased expression of GLUT-4 and whole-body IR. Patients with PCOS and IR also overexpressed miR-133 and miR-223, although these miRNAs failed to show an association with GLUT-4 levels (55). Alternatively, miR-223 has been shown to participate in AT differentiation in studies with adipocyte cell lines (93). It is important to emphasize that in addition to autocrine functions, AT is an important source of circulating exosomal miRNAs and AT-derived exosomal miRNAs regulate expression in other tissues (94). The role of serum (as well as other tissue-specific) miRNAs in PCOS has been reviewed elsewhere (95). Overall, epigenetic modulation via DNA methylation and miRNA regulation appears to be an important mechanism for the control of AT function and its role in the pathophysiology of PCOS.
Mitochondrial dysfunction and endoplasmic reticulum and oxidative stress
Mutations in mitochondrial DNA extracted from peripheral blood samples have been associated with the presence of PCOS (96). However, few studies have focused on assessing mitochondrial content or function specifically in AT from PCOS individuals and their role in the development of AT dysfunction remains unclear (97). Lionett and colleagues (98) measured mitochondrial respiration (ie, oxygen consumption) from abdominal and gluteal SAT of women with PCOS and controls using a respirometer. In this small study, patients with PCOS had lower oxygen flux in SAT, although these differences reached statistical significance only for abdominal fat. However, patients with PCOS had higher BMI in this study, and this could have confounded the results, as obesity is associated with abnormal mitochondrial function in humans (99). Of note, 16 weeks of semi-supervised exercise training did not produce any changes in mitochondrial function in patients with PCOS (98).
There is in vitro evidence that ROS are associated with fat accumulation and adipocyte dysfunction (100). ROS have been associated with downregulation of GLUT-4 in adipocytes and the development of IR (101). However, whether ROS are the result, or the cause, of adipocyte dysfunction remains to be determined. Moreover, studies looking at oxidative stress specifically in AT of women with PCOS are lacking. In addition, while several studies have explored the role of ER stress and activation of the unfolded protein response at the level of granulosa cells in PCOS (102), studies focusing on AT are also lacking. Evidence that ER stress may play a role in AT dysfunction arise mainly from studies on obesity and T2DM (103).
Targeting Adipose Tissue Dysfunction to Treat Polycystic Ovary Syndrome
Lifestyle Modification
It is beyond the scope of this review to assess in depth all evidence of different lifestyle interventions for the treatment of PCOS. Overall, a 2019 Cochrane systematic review found that when compared to minimal treatment, lifestyle intervention resulted in a significant weight reduction, lower waist circumference, improvement of HA (ie, lower testosterone, higher sex hormone–binding globulin (SHBG), lower free androgen index, and lower Ferriman-Gallwey score), and a reduction in insulin levels (104). The review concluded that there were insufficient data to draw any conclusions regarding fertility (ie, pregnancy, live births, or miscarriages) and ovulation (this latter due to inappropriate reporting for a meta-analysis).
Diet
When associated with weight loss, hypocaloric diets (independently of the strategy used) appear to be associated with significant improvements in overweight/obese women with PCOS (ie, improved insulin sensitivity, decrease androgen levels, and improved ovarian function and fertility) (105, 106). While weight loss has been associated with numerous beneficial changes in AT in obese patients, less is known about the specific mechanisms in women with PCOS. In an elegant study in obese individuals (107), approximately 5% weight loss through diet was enough to improve body composition and to maximally improve insulin-mediated suppression of lipolytic activity in AT. Moreover, there was a progressive upregulation of lipid flux pathways and a downregulation of lipid synthesis, oxidative stress, and extracellular matrix remodeling pathways proportional to the amount of weight loss. However, approximately 5% weight loss was not associated with any improvements in SAT markers of inflammation (ie, gene expression of IL-6, TNF, MCP-1, CD68). Indeed, a more pronounced weight loss of 11% to 16% was needed to observe significant changes in AT markers of inflammation. Whether these observations are the same in patients with PCOS remains unknown.
In addition to hypocaloric diets inducing weight loss, other studies have focused on dietary composition for the treatment of PCOS. Different diets have been tested, including Mediterranean, ketogenic, DASH (Dietary Approaches to Stop Hypertension), low carbohydrate or low glycemic index, and pulse-based diets (108). Despite no significant changes in weight, we demonstrated that a short-term, eucaloric, low-carbohydrate diet (16 days) significantly reduced insulin levels compared to standard and high-monounsaturated fatty acid diets in PCOS (109). A longer intervention (ie, 8 weeks) of the eucaloric, low-carbohydrate diet also demonstrated improvements in body composition, testosterone levels, and IR, despite no changes in weight (110).
Ketogenic and Mediterranean diets have also been found to improve hormonal profiles and decrease IR in PCOS (111). However, studies with these types of diets have usually been associated with concomitant weight loss, making it difficult to determine whether the composition of these diets themselves play any role in the improvement in PCOS. Notably, among overweight women with PCOS, high- vs low-protein diets led to similar metabolic and reproductive improvements primarily driven by associated weight loss (112). A meta-analysis indicated that diets aimed at reducing weight were of clinical benefit in PCOS, irrespective of dietary composition (113).
Alternatively, reduction in dietary advanced glycation end products (AGEs) has been observed to improve insulin sensitivity and hormonal profile in PCOS women even in the absence of weight loss (114). AGEs are naturally present in uncooked animal-derived foods, and cooking results in the formation of new AGEs within these foods. In particular, grilling, broiling, roasting, searing, and frying propagate and accelerate new AGE formation (115). Consequently, modern diets are largely heat-processed and as a result contain high levels of AGEs.
Overall, it appears that weight loss (irrespectively of diet composition) plays a key role in the treatment of overweight and obese women with PCOS. Furthermore, there also appears to be a role for dietary composition (eg, hypoglycemic or low AGEs) in improving metabolic dysfunction in PCOS, independent of weight loss. However, the impact of dietary intervention on AT function in PCOS remains to be determined and large, randomized controlled trials (RCTs) assessing these strategies are still needed.
Exercise
Results from RCTs assessing exercise for the treatment of PCOS have reported conflicting results (116), which are likely due to a variety of reasons, including type of exercise, duration of the intervention, amount of weight loss achieved, and compliance of patients with exercise, among others. In a meta-analysis assessing different types and intensities of exercise in PCOS, investigators found the largest improvement in peak oxygen consumption (VO2peak) after moderate and vigorous intensity aerobic exercise (116). Changes in weight were only minimal with exercise-only interventions, although a significant reduction in IR (ie, homeostasis model assessment of IR [HOMA-IR]) was still observed. While moderate and vigorous intensity aerobic exercise did not result in improvement of HA, resistance training was associated with the greatest improvement in free androgen index (116). This effect of resistance training has been confirmed in other studies (117).
When added to a hypocaloric diet in overweight and obese PCOS women, aerobic exercise improved body composition (ie, greater reduction in fat mass) compared to diet alone, but without changes in overall weight, or hormonal or reproductive outcomes (118). High-intensity interval training (HIIT) has also been assessed in women with PCOS with conflicting results. HIIT after 10 weeks was associated with a reduction of HOMA-IR compared to controls and to strength training, despite similar changes in body composition between HIIT and strength training (119). In a 16-week study, the nonexercise group had a more significant weight loss than the HIIT group (98). Moreover, while the non-PCOS group showed an improvement in whole-body fat oxidation, this was not observed in PCOS patients, suggesting that exercise response may be different in women with PCOS. When looking specifically at AT, HIIT induced no changes in miRNA expression, mitochondrial respiration, or adipocyte size (98). While scarce data are available in women with PCOS, even in the obesity literature the effect of exercise on AT GLUT-4 expression is unclear (120).
In summary, there is insufficient evidence that improvements in PCOS due to exercise are related to a direct effect on AT function. Nonetheless, there is a consensus that the hormonal and phenotypic manifestations of PCOS are improved with regular physical activity, particularly in obese patients, likely related to changes in skeletal muscle and overall adiposity.
Bariatric or Metabolic Surgery
Surgical interventions to reduce adiposity, commonly referred to as bariatric surgery, also appear to affect PCOS phenotype and hormonal profile. Roux-en-Y bypass has been found to improve menstrual regularity, improve or resolve hirsutism, and improve metabolic and hormonal profiles in PCOS women (121, 122). Sleeve gastrectomy also improves menses and hormone profiles, though data on the magnitude of its hormonal effect are mixed (123, 124). Of note, pregnancy and fertility rates in patients with PCOS after bariatric surgery are similar to those of non-PCOS controls undergoing bariatric surgery (125).
The significant degree of weight loss achieved after bariatric surgery appears to induce important changes in AT function (126–128). While these studies were not performed specifically in PCOS patients, it is likely that they can be extrapolated to obese PCOS patients. After bariatric surgery, AT demonstrated improvement in inflammatory profile (126), normalization of insulin-induced suppression of lipolysis (127), and improved mitochondrial respiration (128). Of note, improvements in mitochondrial respiration were not related to increased mitochondrial content, but to increased number of adipocytes per milligram of tissue (ie, lower cellular size). It is possible that changes in AT function after substantial weight loss are, at least in part, responsible for the metabolic and hormonal improvements observed in patients with PCOS following bariatric surgery. However, further research is needed to better understand this relationship.
Pharmacologic Therapy
Metformin
One of the most commonly used medications for the treatment of PCOS, metformin has been extensively studied for its effect on endocrine profiles, AT function, inflammation, and fertility outcomes. Studies demonstrate positive effects on menstrual regularity and hirsutism as well as reduction in circulating androgens and inflammatory markers, increased SHBG, and decreased IR (129). Of note, these benefits of metformin occur even in the absence of changes in BMI (130). In a multicenter RCT of 320 women with PCOS and anovulatory infertility, the pregnancy rate increased 1.6 times with the addition of metformin to standard infertility treatment (131). Although AT does not appear to be the primary target of metformin (132), its use in patients with PCOS is associated with indirect changes in AT function, as evidenced by increased adiponectin and decreased leptin and vaspin (133, 134). Also, the effect of metformin on miRNAs is an emerging area of interest, as the drug may decrease expression of certain miRNAs associated with PCOS and IR in AT (135).
Thiazolidinediones
There are currently 2 thiazolidinediones (TZDs) available: pioglitazone and rosiglitazone, which share the same main mechanism of action (ie, PPAR-γ agonism), although pioglitazone has been found to have an overall better metabolic profile. The main target of TZDs is AT, where they induce significant metabolic changes, including increased adipogenesis, increased adiponectin, reduced lipolysis and release of NEFAs, and increased GLUT-4 expression (136). TZDs result in improvements in anovulation, hyperandrogenemia, hirsutism, and infertility in PCOS, reinforcing the importance of AT dysfunction in the pathophysiology of the disorder (137, 138). In PCOS, TZDs appear to be as effective as metformin, with additional benefits when both are combined (139). Of note, metabolic and reproductive improvements with TZDs are also observed in lean patients with PCOS (140). Nonetheless, despite their important benefits, TZDs should not be considered first-line therapies for metabolic dysfunction in PCOS. We should also note that TZDs cross the placenta and are contraindicated in pregnancy, thus limiting their use in fertility-seeking PCOS women.
Glucagon-like peptide-1 receptor agonists
Glucagon-like peptide-1 receptor (GLP-1R) agonists have grown in popularity for the treatment of diabetes and obesity, prompting research into their efficacy in treating PCOS. Adipocytes express GLP-1R and in vitro studies have shown that GLP-1R agonists have direct effects on AT metabolic function (141). The use of GLP-1R agonists in patients with PCOS has been associated with improvements in metabolic and reproductive end points (142). These improvements include reductions in testosterone levels and free androgen index, increased SHBG levels, increased insulin sensitivity and adiponectin levels, and increased ovulation/menstrual frequency and pregnancy rates during in vitro fertilization (143–145). A combination of GLP-1R agonist with metformin was reported to be associated with further improvements when compared to each medication by itself (143). Nonetheless, existing data seem to indicate that the efficacy of GLP-1R agonists in PCOS is largely due to their effect on weight loss (142). Whether these results translate to lean patients with PCOS remains to be determined.
Antiandrogens
Flutamide is an androgen receptor antagonist that is US Food and Drug Administration approved for the treatment of prostate cancer. Some studies have shown that it is effective in the treatment of PCOS (146). In addition to improvements in hirsutism, androgen levels, and ovulation (146, 147), a recent RCT demonstrated significant changes in AT after 6 months of low-dose flutamide. Specifically, normal-weight PCOS patients treated with low-dose flutamide experienced a reduction in android fat mass and in lipid accumulation in newly formed subcutaneous abdominal adipocytes compared to placebo (148). These results are in line with our current understanding of the effects of HA in PCOS women, which have been shown to be associated with increased intra-abdominal fat mass and increased lipid accumulation in newly formed adipocytes in vitro (46). However, the clinical utility of flutamide in AT dysfunction in PCOS may be limited by the rare potential for hepatotoxicity and teratogenesis.
Novel Therapies and Future Directions
Given the role of AT dysfunction in PCOS pathogenesis, the idea of altering this tissue composition has given rise to a number of potential novel therapeutic targets. For example, the transplantation of brown AT in rats was found to improve menstrual regularity, insulin sensitivity, and fertility (149). Heat therapy has also been shown to improve AT inflammation and insulin signaling, ultimately improving metabolic profiles and decreasing total testosterone (150). The role of anti–TNF-α therapy in PCOS treatment has also been suggested, with promising data in murine models (151). When considering future directions for research in PCOS treatment, particularly as it relates to AT dysfunction, it is worth noting that while the majority of studies focus on an overweight or obese model of PCOS, the majority of these patients in the population are not obese (13, 15), and AT dysfunction has been observed even in lean PCOS individuals (55).
Funding
This work was supported by the National Institutes of Health (grant Nos. 1-K24-HD01346, R01-DK073632, and R01-HD29364), an endowment from the Helping Hand of Los Angeles, Inc, and the Foundation for Research and Education Excellence, Inc (to Ricardo Azziz).
Disclosures
Uche Ezeh is an investor in Concentric Analgesics, Inc. Ricardo Azziz serves as a consultant for Spruce Bioscience, Core Access Surgical Technology, Rani Therapeutics, and Fortress Biotech, an advisor for Aurora Forge, and an investor in Martin Imaging. The other authors have nothing to disclose.
Data Availability
Data are available on request to the corresponding author.
References
Abbreviations
- AGE
advanced glycation end product
- Akt
protein kinase B
- AT
adipose tissue
- ATM
adipose tissue macrophage
- BMI
body mass index
- CT
computed tomography
- DNL
de novo lipogenesis
- ER
endoplasmic reticulum
- FSIVGTT
frequently sampled intravenous glucose tolerance test
- GLP-1R
glucagon-like peptide-1 receptor
- GLUT-4
glucose transporter-4
- HA
hyperandrogenism
- HIIT
high-intensity interval training
- HOMA-IR
homeostasis model assessment of insulin resistance
- IL
interleukin
- InsR
insulin receptor
- IR
insulin resistance
- IRS
insulin receptor substrate
- LH
luteinizing hormone
- MAPK
mitogen-activated protein kinase
- MCP-1
monocyte chemoattractant protein-1
- miRNA
microRNA
- NEFA
nonesterified free fatty acid
- OD
ovulatory dysfunction
- PCOM
polycystic ovary morphology
- PCOS
polycystic ovary syndrome
- RCT
randomized controlled trial
- ROS
reactive oxygen species
- SAT
subcutaneous adipose tissue
- SHBG
sex hormone–binding globulin
- T2DM
type 2 diabetes mellitus
- TNF-α
tumor necrosis factor-α
- TZD
thiazolidinedione
- VAT
visceral adipose tissue