-
PDF
- Split View
-
Views
-
Cite
Cite
Rikke Hjortebjerg, Lars Melholt Rasmussen, Mette Faurholdt Gude, Akhmadjon Irmukhamedov, Lars P Riber, Jan Frystyk, Jo G R De Mey, Local IGF Bioactivity Associates with High PAPP-A Activity in the Pericardial Cavity of Cardiovascular Disease Patients, The Journal of Clinical Endocrinology & Metabolism, Volume 105, Issue 11, November 2020, Pages e4083–e4093, https://doi.org/10.1210/clinem/dgaa617
- Share Icon Share
Abstract
Pregnancy-associated plasma protein-A (PAPP-A) has been suggested as a proatherogenic enzyme by its ability to locally increase insulin-like growth factor (IGF) activity through proteolytic cleavage of IGF binding protein-4 (IGFBP-4). Recently, stanniocalcin-2 (STC2) was discovered as an inhibitor of PAPP-A. This study aimed to investigate IGFBP-4, PAPP-A, and STC2 as local regulators of IGF bioactivity in the cardiac microenvironment by comparing levels in the pericardial fluid with those in the circulation of patients with cardiovascular disease.
Plasma and pericardial fluid were obtained from 39 patients undergoing elective cardiothoracic surgery, hereof 15 patients with type 2 diabetes. Concentrations of IGF-I, intact and fragmented IGFBP-4, PAPP-A, and STC2 were determined by immunoassays and IGF bioactivity by a cell-based assay.
In pericardial fluid, the concentrations of total IGF-I, intact IGFBP-4, and STC2 were 72 ± 10%, 91 ± 5%, and 40 ± 24% lower than in plasma, while PAPP-A was 15 times more concentrated. The levels of the 2 IGFBP-4 fragments generated by PAPP-A and reflecting PAPP-A activity were elevated by more than 25%. IGF bioactivity was 62 ± 81% higher in the pericardial fluid than plasma. Moreover, pericardial fluid levels of both IGFBP-4 fragments correlated with the concentration of PAPP-A and with the bioactivity of IGF. All protein levels were similar in pericardial fluid from nondiabetic and diabetic subjects.
PAPP-A increases IGF bioactivity by cleavage of IGFBP-4 in the pericardial cavity of cardiovascular disease patients. This study provides evidence for a distinct local activity of the IGF system, which may promote cardiac dysfunction and coronary atherosclerosis.
Reducing the burden of coronary heart disease and cardiac dysfunction is a major clinical imperative, and a more detailed understanding of key proteins involved in the processes is of importance to develop novel treatment strategies (1). Pericardial fluid has been shown to be a biologically active compartment of the heart that communicates with the myocardial interstitium and constitutes a unique cardiac microenvironment (2, 3). This fluid contains many bioactive compounds that are considered to be products of serum ultrafiltration and leakage from the myocardium toward the pericardial cavity (2, 4). Even though the total protein concentration in pericardial fluid is lower than that of serum (3, 5), proteins that are produced by the epicardium, myocardium, or epicardial adipose tissue may be present at higher concentrations. In cardiovascular disease (CVD), the composition of the myocardial transudate may be altered, and it has been speculated that the pericardial cavity in these patients constitutes a proinflammatory and proatherogenic microenvironment primarily determined by components secreted from the heart, being distinct from the plasma (4, 6). However, our knowledge on the protein flow and potential pathophysiological consequence of the pericardial fluid is limited, and understanding the molecular mechanisms could be helpful to elucidate clinical approaches for therapeutic uses (2, 3).
The enzyme pregnancy-associated plasma protein-A (PAPP-A) is an important modulator of local insulin-like growth factor (IGF) signaling and has been shown to exert proatherogenic activity (7-9). PAPP-A is responsible for the cleavage of IGF binding protein-4 (IGFBP-4) in most tissues, hereby increasing IGF availability and potentiating its growth stimulatory effects (Fig. 1) (10, 11). In the circulation, IGFBP-4 fragment levels correlate with the protein levels and enzymatic activity of PAPP-A (12, 13). However, PAPP-A adheres to cell surfaces and the degradation of IGFBP-4 occurs primarily within tissues (14). This pericellular proteolysis in the vicinity of the IGF-I receptor (IGF-IR) seems to be an effective way of enhancing local receptor activation (15). PAPP-A and IGFBP-4 have both been shown to play important roles in atherosclerosis and circulating concentrations of PAPP-A and IGFBP-4 fragments are associated with cardiovascular risk and acute disease (12, 13, 16). Numerous observations in murine models unanimously support that PAPP-A activity favors an atherogenic milieu and accelerates plaque progression (9, 17, 18). Recently, stanniocalcin-2 (STC2) was discovered as a potent inhibitor of PAPP-A, adding yet another member to the intricate system that controls IGF activity (19). Through inhibition of PAPP-A-mediated IGFBP-4 proteolysis, STC2 overexpression decreases atherosclerotic burden by 47% in hypercholesterolemic mice (20). IGF-I, IGFBP-4, PAPP-A, and STC2 are all produced in the cardiac microenvironment (20-25), but secretory levels and their function in cardiac physiology remain unknown.
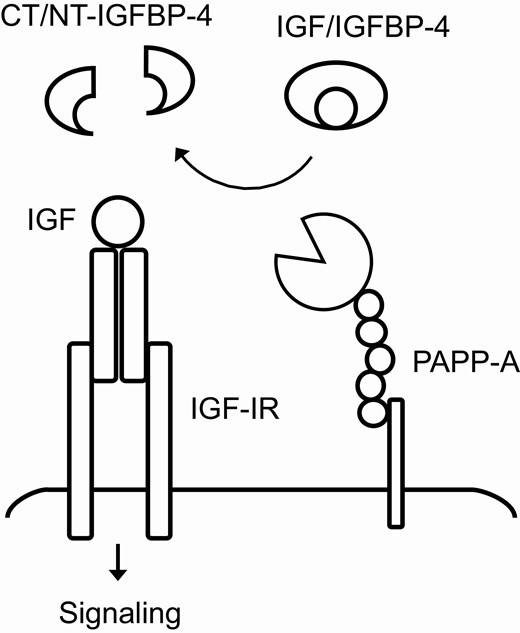
Illustration of the interaction between IGF, IGFBP-4 and PAPP-A. Cell surface-associated PAPP-A cleaves IGFBP-4 in close proximity to the IGF-IR, resulting in the release of bioactive IGF. CT, C-terminal; IGF, insulin-like growth factor; IGF-IR, IGF-I receptor; IGFBP, IGF binding protein; NT, N-terminal; PAPP-A, pregnancy-associated plasma protein-A.
We hypothesized that the pericardial cavity of CVD patients, which comprises a proinflammatory coronary atherogenic microenvironment (4, 6), contains IGF-I, IGFBP-4, PAPP-A, and STC2, partly produced and secreted from the heart and mesothelium, and that the composition differs from that in the circulation. Consequently, pericardial fluid may contain increased IGF bioactivity. Thus, the study aimed to explore the activity of IGF and the IGFBP-4/PAPP-A/STC2 axis in the pericardial cavity of cardiovascular disease patients and compare them to circulating concentrations.
Material and Methods
Patient characteristics
Biological material used for the study was collected within the frame of the research center for individualized medicine in arterial diseases (CIMA) at Odense University Hospital, Denmark, between November 2015 and June 2016. Samples were collected in a systematic and standardized manner from patients undergoing elective coronary artery bypass grafting (CABG), cardiac valve replacement (VR) or both at the Department of Cardiac, Thoracic and Vascular Surgery, Odense University Hospital. As standard anticoagulant treatment, all patients received intravenous administration of heparin at initiation of surgery. On the day before operation, peripheral venous blood was drawn into pyrogen-free tubes with K2EDTA and aprotinin. Similar blood samples were collected at an early step during surgery, at the same time as pericardial fluid was obtained after median sternotomy and section of the pericardium. Blood and pericardial fluid were centrifuged at 3220g for 10 minutes at 4°C and stored at –80°C until analysis.
A total of 39 patients were included in the final cohort. To simultaneously investigate the effect of type 2 diabetes (T2D), the cohort consisted of 24 patients without and 15 patients with T2D. The 2 groups were matched for age, sex, body mass index (BMI), plasma creatinine, glycated hemoglobin (HbA1c), cholesterol, and triglyceride level. Clinical information was obtained from the patients’ medical records. Included patients were free of pericarditis, pericardial effusion, and pericardial tamponade. Patients with visible blood contamination of the pericardial fluid sample were excluded.
The study was approved by The Regional Committees on Health Research Ethics for Southern Denmark (S-20100044 and S-20140202). Investigations were conducted in accordance with the Declaration of Helsinki, and written informed consent was obtained from all patients.
Laboratory measurements
Routine plasma analyses were performed at Department of Clinical Biochemistry at Odense University Hospital using widely available automated assays.
IGF-I levels were measured using an IDS-iSYS Multi-Discipline Automated Analyzer (Immunodiagnostic Systems Nordic SA, Denmark) as previously published (26). The ability of IGF to activate the IGF-IR in vitro (bioactive IGF) was determined by an in-house kinase receptor activation assay (KIRA) as originally described (27) with slight modifications, using the commercial phospho-IGF-IR enzyme-linked immunosorbent kit from R&D systems (Cat# DYC 1770E; Abingdon, UK). The assay measures the ability of IGF to phosphorylate the IGF-IR in an in vitro based model using IGF-IR gene-transfected human embryonic kidney cells. A serial dilution of rhIGF-I (WHO 02/254) served as calibrator. The KIRA assay signal primarily reflects binding of IGF-I to the IGF-IR, but also binding of IGF-II and pro-IGF-II (cross-reactivity 12% and 2%, respectively). The cross-reactivity of proinsulin and insulin is negligible (<1%). Hence, the assay signal is referred to as IGF bioactivity. KIRA assay detection limit was <0.08 µg/L and intra- and interassay CVs were 12% and 20%, respectively.
EDTA plasma levels of intact IGFBP-4 and of the 2 PAPP-A generated fragments, C-terminal (CT)-IGFBP-4 and N-terminal (NT)-IGFBP-4, were measured in duplicate by in-house TR-IFMAs using monoclonal antibodies and recombinant human calibrators generously provided by HyTest Ltd. (Turku, Finland). The assays were performed as previously described (12, 13, 28). In each fragment assay, 1 of the antibodies specifically recognizes the proteolytic neoepitope generated upon cleavage by PAPP-A. Detection limits were 0.5 µg/L for IGFBP-4, 0.4 µg/L for CT-IGFBP-4 and 0.9 µg/L for NT-IGFBP-4. Intra- and interassay CVs were <10% and <15%, respectively.
PAPP-A and STC2 levels were determined by commercial sandwich enzyme-linked immunosorbents (PAPP-A; Cat# AL-101 and STC2; Cat# AL-143) from AnshLabs (Webster, TX, USA). Assay procedures were as described by the manufacturer, and both assays behaved linearly within the analytical range.
Total protein concentrations were determined in plasma and pericardial fluid using the Micro BCA Protein Assay Kit (Cat# 23235, ThermoFisher, MA, United States).
Heparin activity assay
Heparin has been demonstrated to elicit a rapid and transient increase in circulating concentrations of PAPP-A (29, 30). The volumes of distribution of heparin are low, reflecting minimal distribution in the extravascular space (31). However, to ensure that heparin administered on the day of surgery would not interfere with pericardial fluid PAPP-A measurements, in vitro heparin activity was determined in pericardial fluid and plasma collected intraoperatively from three randomly selected patients. The anticoagulant activity of heparin was assessed using the COAMATIC® heparin assay (Cat# 82339363, Chromogenix, Sweden) on a STA R analyzer (Stago, France) as described by the manufacturer. The biological activity of heparin resides in its ability to accelerate the inhibitory effect of antithrombin on the coagulation proteases. The amount of low molecular weight or unfractionated heparin is determined from the anti-FXa activity expressed by the complex formed by heparin and antithrombin in the sample. Thus, the prolongation of clotting time by heparin is used to indirectly determine the amount of heparin present in the blood and pericardial fluid.
Statistics
The assumption of normality was checked using quantile plots, and non-normally distributed variables were transformed using the natural logarithm prior to statistical analyses. Two-group comparisons were performed using unpaired Student’s t-test, and paired observations were analyzed using paired t-test. Variables that remained non-normally distributed upon transformation were analyzed using the nonparametric Wilcoxon matched-pairs signed-rank test or Mann Whitney U statistics as appropriate. Correlations were examined using Pearson or Spearman correlation analysis. The proteins investigated in the present study have previously shown to provide ample statistical power in cohorts of 9 to 24 subjects (10, 35, 36). Data are reported as means ± SD for normally distributed variables and medians with interquartile range for non-normally distributed variables. Level of significance was P < .05. Statistical analyses were performed using Stata 13 (StataCorp, College Station, TX, USA).
Results
Clinical characteristics of the patients are shown in Table 1. Patients were undergoing CABG (49%), cardiac valve replacement (38%), or both (13%), indicating that 62% were suffering from ischemic heart disease due to coronary artery disease. All selected patients were male, overweight, and elderly. Most were previously diagnosed with hypertension and dyslipidemia, but due to treatments with blood pressure lowering drugs and statins, they had normal blood pressure and plasma lipid levels. Approximately half of the patients had a history of smoking, and 15 had T2D. The creatinine values indicated normal kidney function. Most patients had been prescribed combinations of low-dose aspirin, inhibitors of the renin angiotensin system and diuretics. Patients with T2D were treated with metformin (n = 11), insulin analogues (n = 4), GLP-1 receptor agonists (n = 2), or dipeptidyl peptidase-4 inhibitors (n = 1). All patients were effectively treated as judged from their normalized HbA1c levels.
Characteristics . | . |
---|---|
CABG/VR/both, n (%) | 19 (49)/15 (38)/5 (13) |
Males, n (%) | 37 (100) |
Age, years | 69 ± 6 |
BMI, kg/m2 | 28.6 ± 3.8 |
Systolic blood pressure, mmHg | 137 ± 16 |
Diastolic blood pressure, mmHg | 75 ± 11 |
Known hypertension, n (%) | 25 (64) |
Total cholesterol, mmol/L | 3.8 (3.2;4.9) |
LDL cholesterol, mmol/L | 2.1 (1.7;2.7) |
HDL cholesterol, mmol/L | 1.0 (0.9;1.2) |
Triglycerides, mmol/L | 1.4 (1.1;2.2) |
Known dyslipidemia, n (%) | 37 (95) |
Ejection fraction (%) | 55 (50;55) |
Creatinine, μmol/L | 85 ± 21 |
eGFR (mL/min/1.73 m2) | 84 ± 25 |
Type 2 diabetes, n (%) | 15 (38) |
HbA1c, mmol/mol | 40 (37;46) |
Smoking status, n (%) (current/former/never) | 8 (21)/12 (31)/19 (49) |
ACEI/ARB, n (%) | 13 (33)/10 (26) |
Aspirin, n (%) | 31 (79) |
β-blocker, n (%) | 28 (72) |
Biguanide, n (%) | 11 (28) |
Ca2+-antagonist, n (%) | 12 (31) |
Diuretic, n (%) | 8 (21) |
Statin, n (%) | 35 (90) |
Characteristics . | . |
---|---|
CABG/VR/both, n (%) | 19 (49)/15 (38)/5 (13) |
Males, n (%) | 37 (100) |
Age, years | 69 ± 6 |
BMI, kg/m2 | 28.6 ± 3.8 |
Systolic blood pressure, mmHg | 137 ± 16 |
Diastolic blood pressure, mmHg | 75 ± 11 |
Known hypertension, n (%) | 25 (64) |
Total cholesterol, mmol/L | 3.8 (3.2;4.9) |
LDL cholesterol, mmol/L | 2.1 (1.7;2.7) |
HDL cholesterol, mmol/L | 1.0 (0.9;1.2) |
Triglycerides, mmol/L | 1.4 (1.1;2.2) |
Known dyslipidemia, n (%) | 37 (95) |
Ejection fraction (%) | 55 (50;55) |
Creatinine, μmol/L | 85 ± 21 |
eGFR (mL/min/1.73 m2) | 84 ± 25 |
Type 2 diabetes, n (%) | 15 (38) |
HbA1c, mmol/mol | 40 (37;46) |
Smoking status, n (%) (current/former/never) | 8 (21)/12 (31)/19 (49) |
ACEI/ARB, n (%) | 13 (33)/10 (26) |
Aspirin, n (%) | 31 (79) |
β-blocker, n (%) | 28 (72) |
Biguanide, n (%) | 11 (28) |
Ca2+-antagonist, n (%) | 12 (31) |
Diuretic, n (%) | 8 (21) |
Statin, n (%) | 35 (90) |
Categorical variables are indicated as number (n) and as percentage (%) of all 39 patients in parenthesis. Continuous normally distributed variables are mean ± SD and non-normally distributed variables are median (25th percentile; 75th percentile).
Abbreviations: ACEI/ARB, inhibitor of angiotensin converting enzyme or antagonist of angiotensin II AT1 receptors; BMI, body mass index; CABG, coronary artery bypass surgery; eGFR, estimated glomerular filtration rate; HbA1c, glycated hemoglobin; HDL, high-density lipoprotein; LDL, low-density lipoprotein; VR, valve replacement.
*P < .05 when compared with plasma level.
Characteristics . | . |
---|---|
CABG/VR/both, n (%) | 19 (49)/15 (38)/5 (13) |
Males, n (%) | 37 (100) |
Age, years | 69 ± 6 |
BMI, kg/m2 | 28.6 ± 3.8 |
Systolic blood pressure, mmHg | 137 ± 16 |
Diastolic blood pressure, mmHg | 75 ± 11 |
Known hypertension, n (%) | 25 (64) |
Total cholesterol, mmol/L | 3.8 (3.2;4.9) |
LDL cholesterol, mmol/L | 2.1 (1.7;2.7) |
HDL cholesterol, mmol/L | 1.0 (0.9;1.2) |
Triglycerides, mmol/L | 1.4 (1.1;2.2) |
Known dyslipidemia, n (%) | 37 (95) |
Ejection fraction (%) | 55 (50;55) |
Creatinine, μmol/L | 85 ± 21 |
eGFR (mL/min/1.73 m2) | 84 ± 25 |
Type 2 diabetes, n (%) | 15 (38) |
HbA1c, mmol/mol | 40 (37;46) |
Smoking status, n (%) (current/former/never) | 8 (21)/12 (31)/19 (49) |
ACEI/ARB, n (%) | 13 (33)/10 (26) |
Aspirin, n (%) | 31 (79) |
β-blocker, n (%) | 28 (72) |
Biguanide, n (%) | 11 (28) |
Ca2+-antagonist, n (%) | 12 (31) |
Diuretic, n (%) | 8 (21) |
Statin, n (%) | 35 (90) |
Characteristics . | . |
---|---|
CABG/VR/both, n (%) | 19 (49)/15 (38)/5 (13) |
Males, n (%) | 37 (100) |
Age, years | 69 ± 6 |
BMI, kg/m2 | 28.6 ± 3.8 |
Systolic blood pressure, mmHg | 137 ± 16 |
Diastolic blood pressure, mmHg | 75 ± 11 |
Known hypertension, n (%) | 25 (64) |
Total cholesterol, mmol/L | 3.8 (3.2;4.9) |
LDL cholesterol, mmol/L | 2.1 (1.7;2.7) |
HDL cholesterol, mmol/L | 1.0 (0.9;1.2) |
Triglycerides, mmol/L | 1.4 (1.1;2.2) |
Known dyslipidemia, n (%) | 37 (95) |
Ejection fraction (%) | 55 (50;55) |
Creatinine, μmol/L | 85 ± 21 |
eGFR (mL/min/1.73 m2) | 84 ± 25 |
Type 2 diabetes, n (%) | 15 (38) |
HbA1c, mmol/mol | 40 (37;46) |
Smoking status, n (%) (current/former/never) | 8 (21)/12 (31)/19 (49) |
ACEI/ARB, n (%) | 13 (33)/10 (26) |
Aspirin, n (%) | 31 (79) |
β-blocker, n (%) | 28 (72) |
Biguanide, n (%) | 11 (28) |
Ca2+-antagonist, n (%) | 12 (31) |
Diuretic, n (%) | 8 (21) |
Statin, n (%) | 35 (90) |
Categorical variables are indicated as number (n) and as percentage (%) of all 39 patients in parenthesis. Continuous normally distributed variables are mean ± SD and non-normally distributed variables are median (25th percentile; 75th percentile).
Abbreviations: ACEI/ARB, inhibitor of angiotensin converting enzyme or antagonist of angiotensin II AT1 receptors; BMI, body mass index; CABG, coronary artery bypass surgery; eGFR, estimated glomerular filtration rate; HbA1c, glycated hemoglobin; HDL, high-density lipoprotein; LDL, low-density lipoprotein; VR, valve replacement.
*P < .05 when compared with plasma level.
IGF levels in the circulation
When comparing protein levels in plasma samples obtained before and during surgery, we observed a diminution in several target protein concentrations (Table 2). Levels were reduced by 17 ± 11% for IGF-I, 19 ± 9% for IGFBP-4, 18 ± 9% for CT-IGFBP-4, 17 ± 8% for NT-IGFBP-4, and 16 ± 12% for STC2. Hemodilution is common during cardiac surgery, due to intravenous fluid administration after anesthesia. However, IGF bioactivity in plasma was similar before and during surgery. Total PAPP-A in the circulation prior to operation was similar to levels reported in healthy, nonpregnant individuals (approximately 0.8-1 ng/mL) (28-30, 32), whereas intraoperative plasma levels were significantly elevated by 65-fold. Unless otherwise stated, levels of IGF-I, IGF bioactivity, IGFBP-4, PAPP-A, and STC2 in plasma from the day before surgery are compared with the pericardial fluid at initiation of surgery.
IGF protein levels in pericardial fluid and plasma prior to and during operation of cardiovascular disease patients
Protein (ng/mL) . | Plasma before . | Plasma during . | P plasma . | Pericardial fluid . | P before . | P during . |
---|---|---|---|---|---|---|
IGF-I | 136 ± 35 | 108 ± 28 | <.001 | 36 ± 8 | <.001 | <.001 |
IGF bioactivity | 0.78 ± 32 | 0.85 ± 0.33 | .241 | 1.25 ± 0.57 | <.001 | <.001 |
IGFBP-4 | 247 ± 56 | 210 ± 50 | <.001 | 23 ± 12 | <.001 | <.001 |
CT-IGFBP-4 | 51 ± 12 | 42 ± 9 | <.001 | 75 ± 18 | <.001 | <.001 |
NT-IGFBP-4 | 151 ± 19 | 128 ± 20 | <.001 | 187 ± 34 | <.001 | <.001 |
PAPP-A | 0.84 (0.75;1.17) | 61 (60;63) | <.001 | 14.6 (12.0;18.4) | <.001 | <.001 |
STC2 | 27.7 ± 6.9 | 23.1 ± 5.2 | <.001 | 16.9 ± 6.2 | <.001 | <.001 |
Protein (ng/mL) . | Plasma before . | Plasma during . | P plasma . | Pericardial fluid . | P before . | P during . |
---|---|---|---|---|---|---|
IGF-I | 136 ± 35 | 108 ± 28 | <.001 | 36 ± 8 | <.001 | <.001 |
IGF bioactivity | 0.78 ± 32 | 0.85 ± 0.33 | .241 | 1.25 ± 0.57 | <.001 | <.001 |
IGFBP-4 | 247 ± 56 | 210 ± 50 | <.001 | 23 ± 12 | <.001 | <.001 |
CT-IGFBP-4 | 51 ± 12 | 42 ± 9 | <.001 | 75 ± 18 | <.001 | <.001 |
NT-IGFBP-4 | 151 ± 19 | 128 ± 20 | <.001 | 187 ± 34 | <.001 | <.001 |
PAPP-A | 0.84 (0.75;1.17) | 61 (60;63) | <.001 | 14.6 (12.0;18.4) | <.001 | <.001 |
STC2 | 27.7 ± 6.9 | 23.1 ± 5.2 | <.001 | 16.9 ± 6.2 | <.001 | <.001 |
Continuous normally distributed variables are mean ± SD and non-normally distributed variables are median (25th percentile; 75th percentile). P values represent comparison between plasma before and during operation (P plasma) or between pericardial fluid and plasma before and during operation, respectively.
Abbreviations: CT, C-terminal; IGF, insulin-like growth factor; IGFBP, IGF binding protein; NT, N-terminal; PAPP-A, pregnancy-associated plasma protein-A; STC2, stanniocalcin-2
IGF protein levels in pericardial fluid and plasma prior to and during operation of cardiovascular disease patients
Protein (ng/mL) . | Plasma before . | Plasma during . | P plasma . | Pericardial fluid . | P before . | P during . |
---|---|---|---|---|---|---|
IGF-I | 136 ± 35 | 108 ± 28 | <.001 | 36 ± 8 | <.001 | <.001 |
IGF bioactivity | 0.78 ± 32 | 0.85 ± 0.33 | .241 | 1.25 ± 0.57 | <.001 | <.001 |
IGFBP-4 | 247 ± 56 | 210 ± 50 | <.001 | 23 ± 12 | <.001 | <.001 |
CT-IGFBP-4 | 51 ± 12 | 42 ± 9 | <.001 | 75 ± 18 | <.001 | <.001 |
NT-IGFBP-4 | 151 ± 19 | 128 ± 20 | <.001 | 187 ± 34 | <.001 | <.001 |
PAPP-A | 0.84 (0.75;1.17) | 61 (60;63) | <.001 | 14.6 (12.0;18.4) | <.001 | <.001 |
STC2 | 27.7 ± 6.9 | 23.1 ± 5.2 | <.001 | 16.9 ± 6.2 | <.001 | <.001 |
Protein (ng/mL) . | Plasma before . | Plasma during . | P plasma . | Pericardial fluid . | P before . | P during . |
---|---|---|---|---|---|---|
IGF-I | 136 ± 35 | 108 ± 28 | <.001 | 36 ± 8 | <.001 | <.001 |
IGF bioactivity | 0.78 ± 32 | 0.85 ± 0.33 | .241 | 1.25 ± 0.57 | <.001 | <.001 |
IGFBP-4 | 247 ± 56 | 210 ± 50 | <.001 | 23 ± 12 | <.001 | <.001 |
CT-IGFBP-4 | 51 ± 12 | 42 ± 9 | <.001 | 75 ± 18 | <.001 | <.001 |
NT-IGFBP-4 | 151 ± 19 | 128 ± 20 | <.001 | 187 ± 34 | <.001 | <.001 |
PAPP-A | 0.84 (0.75;1.17) | 61 (60;63) | <.001 | 14.6 (12.0;18.4) | <.001 | <.001 |
STC2 | 27.7 ± 6.9 | 23.1 ± 5.2 | <.001 | 16.9 ± 6.2 | <.001 | <.001 |
Continuous normally distributed variables are mean ± SD and non-normally distributed variables are median (25th percentile; 75th percentile). P values represent comparison between plasma before and during operation (P plasma) or between pericardial fluid and plasma before and during operation, respectively.
Abbreviations: CT, C-terminal; IGF, insulin-like growth factor; IGFBP, IGF binding protein; NT, N-terminal; PAPP-A, pregnancy-associated plasma protein-A; STC2, stanniocalcin-2
Pericardial fluid IGF levels
In pericardial fluid, the concentrations of IGF-I, IGFBP-4, and STC2 were significantly lower than in preoperative plasma, being reduced by 72 ± 10%, 91 ± 5%, and 40 ± 24%, respectively (Table 2 and Fig. 2A). IGF bioactivity was increased by 62 ± 81% in pericardial fluid as compared with plasma. When compared with preoperative plasma, pericardial fluid CT-IGFBP-4 and NT-IGFBP-4 levels were increased by 52 ± 46% and 25 ± 26%, respectively, and the increase was higher (86 ± 57% and 49 ± 33%, respectively) than intraoperative plasma levels. PAPP-A was also significantly higher in pericardial fluid than preoperative plasma, being increased by more than 15-fold (16 ± 6 times). To investigate if heparin administered at initiation of surgery could interfere with pericardial fluid PAPP-A measurements, heparin activity was determined in pericardial fluid and intraoperative plasma using a coagulation assay. In plasma samples obtained during surgery, heparin activity was high, whereas pericardial fluid heparin activities were below limit of detection (data not shown). Based on these results, heparin appears to be distributed solely in the circulating volume and not to be present in pericardial fluid. Thus, high PAPP-A levels in pericardial fluid did not result from the heparin administration, and in the subsequent analyses, we consider it justified to compare plasma from the day before surgery with the pericardial fluid at initiation of surgery.
![(A) Ratios of pericardial fluid-to-plasma level ([PF]/[circulation]) of IGF protein levels and (B) ratios of these proteins in the pericardial fluid and plasma ([PF]/[circulation] adjusted for total protein. Ratio >1 reflects higher PF level and ratio <1 higher level in circulation. Horizontal line shows level at which PF = plasma. Ratios are mean ± SD on log scale. CT, C-terminal; IGF, insulin-like growth factor; IGFBP, IGF binding protein; NT, N-terminal; PAPP-A, pregnancy-associated plasma protein-A; PF, pericardial fluid; STC2, stanniocalcin-2.](https://oup.silverchair-cdn.com/oup/backfile/Content_public/Journal/jcem/105/11/10.1210_clinem_dgaa617/3/m_dgaa617_fig2.jpeg?Expires=1750311378&Signature=kzc8x1Eo1T9JDy-FumQwuWkqQvmSU1DI2FtS9-D7CxnRX-Ad5knGHYePIBCOwn-tgvQ8ucynmMJsssk5yxBGHP4EnEaaJ8cpDHbZDQVQVGbgi~6W9CHXyGf~j8wARwjKKwZITDc-NKpOzKfaEmw952PjYsE3T2NndnQT5N2k53zgQAOqrNc5bNd5uFuoGLXy2DBMzMgTTKTWdO1Co0jlWFN2lm3slIzNWCoZVKmNbTGxtcn-ZSBLblSY6SWyXaTNRuAd7HKorIgn21TRefxuKxF~fniS3PZ9~n9NbpnnH43DRv3He~WYi0gGB1VyajlGgUyAX0tV~lPCdxle0l8NYQ__&Key-Pair-Id=APKAIE5G5CRDK6RD3PGA)
(A) Ratios of pericardial fluid-to-plasma level ([PF]/[circulation]) of IGF protein levels and (B) ratios of these proteins in the pericardial fluid and plasma ([PF]/[circulation] adjusted for total protein. Ratio >1 reflects higher PF level and ratio <1 higher level in circulation. Horizontal line shows level at which PF = plasma. Ratios are mean ± SD on log scale. CT, C-terminal; IGF, insulin-like growth factor; IGFBP, IGF binding protein; NT, N-terminal; PAPP-A, pregnancy-associated plasma protein-A; PF, pericardial fluid; STC2, stanniocalcin-2.
The total protein concentration in pericardial fluid differed significantly from that in plasma, with a fluid-to-plasma protein ratio in the same patient of 0.36 (0.30; 0.54), P < 0.05. When calculating the target protein concentrations as a fraction of the total protein concentration of each compartment ([pericardial fluid]/[plasma]total protein), IGF-I appeared to be of similar abundance with a ratio of 0.96 ± 0.25, P = .052 (Fig. 2B). However, IGF bioactivity was significantly elevated in the pericardial cavity, being more than 4-fold higher (4.5 ± 2.2, P < .001). IGFBP-4 remained to constitute a smaller fraction of the total protein concentration in pericardial fluid compared to plasma with a ratio of 0.26 ± 0.14 (P < .001), whereas CT-IGFBP-4 and NT-IGFBP-4 were 4.2 ± 1.3 and 3.5 ± 0.7 times enriched in the pericardial cavity (P < .001). PAPP-A constituted a significantly larger fraction of the total protein concentration in the local microenvironment around the heart than in the circulation, being more than 40-fold higher (43 ± 16, P < .001). STC2 was 1.7 ± 0.7 times locally more abundant (P < .001). All IGF protein levels in plasma and pericardial fluid were similar in patients undergoing CABG, VR, or both.
Relations between IGF protein levels
In uncorrected Pearson correlation analysis, IGF-I levels were strongly correlated in plasma obtained before and during surgery (r = 0.89, P < .001). The same pattern was observed for IGF bioactivity (r = 0.51, P = .007), IGFBP-4 (r = 0.84, P < .001), CT-IGFBP-4 (r = 0.87, P < .001), NT-IGFBP-4 (r = 0.71, P < .001), and STC2 (r = 0.83, P < .001). Following Bonferroni correction, all associations apart from IGF bioactivity remained statistically significant. Significant correlations between plasma and pericardial fluid concentrations were not observed for any of the proteins investigated.
IGF-I concentration in the circulation was positively associated with IGF bioactivity and negatively associated with the levels of both PAPP-A and STC2 (Table 3). In pericardial fluid, IGF-I and IGF bioactivity were correlated. Relationships between IGFBP-4 proteins were found in both compartments, with IGFBP-4, CT-IGFBP-4, and NT-IGFBP-4 plasma levels all being positively interrelated. However, in pericardial fluid, only CT-IGFBP-4 and NT-IGFBP-4 were associated. In pericardial fluid, both fragments were also positively related to total IGF-I, IGF-I bioactivity, and PAPP-A concentration (Fig. 3). Pericardial STC2 was positively associated with IGF-I, IGF bioactivity, IGFBP-4 fragment, and PAPP-A levels (Fig. 4). Overall, more statistically significant correlations were observed in the pericardial cavity than in plasma (Table 3).
Relationships between the concentrations of IGF proteins in pericardial fluid and plasma
. | IGF-I . | IGF bioactivity . | IGFBP-4 . | CT-IGFBP-4 . | NT-IGFBP-4 . | PAPP-A . | STC2 . |
---|---|---|---|---|---|---|---|
IGF-I | .364 | –.392 | –.443 | ||||
.032 | .039 | .018 | |||||
IGF bioactivity | .680 | ||||||
.000 | |||||||
IGFBP-4 | .444 | .541 | |||||
.009 | .001 | ||||||
CT-IGFBP-4 | .566 | .464 | .362 | ||||
.000 | .003 | .038 | |||||
NT-IGFBP-4 | .547 | .414 | .760 | ||||
.000 | .010 | .000 | |||||
PAPP-A | .571 | .378 | .349 | ||||
.000 | .018 | .045 | |||||
STC2 | .704 | .407 | .633 | .618 | .410 | ||
.000 | .011 | .000 | .000 | .009 |
. | IGF-I . | IGF bioactivity . | IGFBP-4 . | CT-IGFBP-4 . | NT-IGFBP-4 . | PAPP-A . | STC2 . |
---|---|---|---|---|---|---|---|
IGF-I | .364 | –.392 | –.443 | ||||
.032 | .039 | .018 | |||||
IGF bioactivity | .680 | ||||||
.000 | |||||||
IGFBP-4 | .444 | .541 | |||||
.009 | .001 | ||||||
CT-IGFBP-4 | .566 | .464 | .362 | ||||
.000 | .003 | .038 | |||||
NT-IGFBP-4 | .547 | .414 | .760 | ||||
.000 | .010 | .000 | |||||
PAPP-A | .571 | .378 | .349 | ||||
.000 | .018 | .045 | |||||
STC2 | .704 | .407 | .633 | .618 | .410 | ||
.000 | .011 | .000 | .000 | .009 |
Correlation analyses of IGF proteins in pericardial fluid (italics) and plasma in cardiovascular disease patients. Data are expressed as unadjusted correlation coefficient (Pearson’s correlation) r values (top) and P values (bottom). Only statistically significant correlations (P < .05) are reported.
Abbreviations: CT, C-terminal; IGF, insulin-like growth factor; IGFBP, IGF binding protein; NT, N-terminal; PAPP-A, pregnancy-associated plasma protein-A; STC2, stanniocalcin-2.
Relationships between the concentrations of IGF proteins in pericardial fluid and plasma
. | IGF-I . | IGF bioactivity . | IGFBP-4 . | CT-IGFBP-4 . | NT-IGFBP-4 . | PAPP-A . | STC2 . |
---|---|---|---|---|---|---|---|
IGF-I | .364 | –.392 | –.443 | ||||
.032 | .039 | .018 | |||||
IGF bioactivity | .680 | ||||||
.000 | |||||||
IGFBP-4 | .444 | .541 | |||||
.009 | .001 | ||||||
CT-IGFBP-4 | .566 | .464 | .362 | ||||
.000 | .003 | .038 | |||||
NT-IGFBP-4 | .547 | .414 | .760 | ||||
.000 | .010 | .000 | |||||
PAPP-A | .571 | .378 | .349 | ||||
.000 | .018 | .045 | |||||
STC2 | .704 | .407 | .633 | .618 | .410 | ||
.000 | .011 | .000 | .000 | .009 |
. | IGF-I . | IGF bioactivity . | IGFBP-4 . | CT-IGFBP-4 . | NT-IGFBP-4 . | PAPP-A . | STC2 . |
---|---|---|---|---|---|---|---|
IGF-I | .364 | –.392 | –.443 | ||||
.032 | .039 | .018 | |||||
IGF bioactivity | .680 | ||||||
.000 | |||||||
IGFBP-4 | .444 | .541 | |||||
.009 | .001 | ||||||
CT-IGFBP-4 | .566 | .464 | .362 | ||||
.000 | .003 | .038 | |||||
NT-IGFBP-4 | .547 | .414 | .760 | ||||
.000 | .010 | .000 | |||||
PAPP-A | .571 | .378 | .349 | ||||
.000 | .018 | .045 | |||||
STC2 | .704 | .407 | .633 | .618 | .410 | ||
.000 | .011 | .000 | .000 | .009 |
Correlation analyses of IGF proteins in pericardial fluid (italics) and plasma in cardiovascular disease patients. Data are expressed as unadjusted correlation coefficient (Pearson’s correlation) r values (top) and P values (bottom). Only statistically significant correlations (P < .05) are reported.
Abbreviations: CT, C-terminal; IGF, insulin-like growth factor; IGFBP, IGF binding protein; NT, N-terminal; PAPP-A, pregnancy-associated plasma protein-A; STC2, stanniocalcin-2.
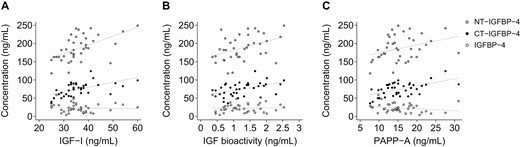
Associations of pericardial fluid IGFBP-4, CT-IGFBP-4 and NT-IGFBP-4 with IGF-I (A), IGF bioactivity (B), and PAPP-A (C). CT, C-terminal; IGF, insulin-like growth factor; IGFBP, IGF binding protein; NT, N-terminal; PAPP-A, pregnancy-associated plasma protein-A.

Associations of pericardial fluid STC2 with IGF-I (A), IGF bioactivity (B), IGFBP-4 fragments (C) and PAPP-A (D). CT, C-terminal; IGF, insulin-like growth factor; IGFBP, IGF binding protein; NT, N-terminal; PAPP-A, pregnancy-associated plasma protein-A; STC2, stanniocalcin-2.
Influence of diabetes on the IGF axis
T2D is associated with a marked increase in the risk of CVD compared with nondiabetic subjects. To assess potential distortions in the IGF system in diabetic cardiovascular patients, protein levels were compared between the 15 patients with T2D and the 24 nondiabetic patients. Circulating IGF-I levels were lower in the patients with T2D (108 ± 31 vs 151 ± 26 ng/mL, P < .007), whereas the IGF bioactivity was similar in nondiabetic and diabetic subjects (0.66 ± 0.23 vs 0.85 ± 0.35, P = .126). Neither plasma IGFBP-4, IGFBP-4 fragments, PAPP-A, nor STC2 were affected by diabetes status. All protein levels were similar in pericardial fluid from nondiabetic and diabetic subjects.
Discussion
In pericardial fluid of CVD patients, the activity of IGF and the IGFBP-4/PAPP-A/STC2 axis differs notably from that of the circulation, indicating that this regulatory IGF system is secreted in part by the heart. PAPP-A levels were increased by more than 15-fold in pericardial fluid than in the circulation, suggesting an important function of this IGFBP protease in the local cardiac compartment. A high PAPP-A activity was further substantiated by measurements of the PAPP-A generated IGFBP-4 fragments. IGF-I bioactivity was also markedly increased in pericardial fluid, despite total IGF-I levels being lower than those in the circulation. Importantly, both IGFBP-4 fragments were associated with PAPP-A and IGF bioactivity in the pericardial fluid, emphasizing that IGFBP-4 is a main substrate of PAPP-A and that high IGFBP-4 proteolytic degradation results in increased IGF-IR activation. Thus, our results substantiate that PAPP-A serves to increase IGF bioactivity in the local cardiac environment through cleavage of IGFBP-4.
STC2 associated positively with pericardial IGF-I, IGF bioactivity, both IGFBP-4 fragments, and PAPP-A (Fig. 4). This is in apparent conflict with the role of STC2 as an inhibitor of PAPP-A and hence IGF signaling. However, STC2 has primarily been shown to inhibit PAPP-A and reduce atherosclerotic burden when overexpressed in mice (20). Thus, the studies show that lesion development can be inhibited in an experimental model by artificially driving the balance towards inhibited PAPP-A. However, it is notable that multiple articles link upregulation of STC2 to human cancer, which similarly is not in line with its growth inhibitory effects (33). No clear picture can be drawn at this time, but it is tempting to speculate that PAPP-A and STC2 are part of the same regulatory system in tissues where they may be coexpressed.
The function of the IGF system in cardiac dysfunction and CVD has been a subject of much controversy and reports conflict whether the IGF system is fundamentally exerting proatherogenic or atheroprotective functions (14). However, overwhelming evidence supports the theory that high PAPP-A activity favors an atherogenic milieu. Transgenic overexpression of PAPP-A in mice accelerates plaque progression, whereas absence of PAPP-A causes an 80% reduction in plaque development (7, 9, 17, 18). In addition, atherogenesis driven by PAPP-A is directly related to its proteolysis of IGFBP-4 in close proximity to cell surface IGF-IR (7). Finally, STC2 was shown to be present in mouse intimal and medial smooth muscle during atheroma formation (20).
In humans, studies have primarily focused on the circulating IGF system (32, 34-39) and have not addressed extravascular fluids with respect to cardiac dysfunction and CVD. Pericardial fluid isolates the heart from the adjacent tissues and is considered to be reflective of the heart’s physiological status (4). The epicardium or the serous visceral pericardium covers the outside of the heart and consists of a monolayer of mesothelial cells lying on a basal membrane. The pericardial fluid is considered to be drained by the lymphatic capillary bed, but it may also arise from myocardial interstitial fluid traversing the epicardium (2, 40). For proteins to move from the intravascular space to the pericardial cavity, it must cross connective tissues and the mesothelial barrier of the epicardium. Thus, for most proteins, levels in pericardial fluid are lower than in plasma, and the influx shows a strong relationship with molecular weight, with a high concentration for small molecules and a lower concentration for high molecular weight proteins. Molecules up to 40 kDa can easily diffuse into the pericardial cavity, whereas transport of larger macromolecules is generally prevented (41, 42). However, proteins that are produced by the myocardium and surrounding tissue may be more abundant in the pericardium. In this study, the total protein concentration in pericardial fluid was only 36% of that in plasma, which is similar to concentrations found in other studies (5). However, local and systemic disorders may perturb the balance between synthesis and discharge of the fluid and cause its aberrant accumulation in the pericardial cavity (40). Furthermore, the protein transport properties of the epicardial barrier during chronic cardiac disease may be very different. Studies indicate that the pericardial cavity of CVD patients is a proinflammatory microenvironment determined by components derived from the heart and the mesothelium rather than the circulation or paracardial tissues (4, 6). In this study, the pericardial concentration of IGF-I matched reports by others (43), and the fluid-to-plasma ratio of 35% was similar to the ratio for total protein. The molecular weight of free IGF-I is 7.6 kDa (44), which should not complicate the diffusion from the circulation. Thus, the ratio suggests that an equilibrium between plasma and pericardial fluid IGF-I has been established. However, the lack of correlation between plasma and pericardial IGF-I suggests that pericardial IGF-I may not originate entirely from the circulation. Interestingly, PAPP-A was highly enriched in pericardial fluid. Enzymatically active PAPP-A is a homodimer of 2 identical subunits of each 200 kDa (45). The high molecular weight of PAPP-A implies that the paracellular route would not be an access way to the pericardial cavity, strongly indicating cardiac production. STC2 concentrations were lower in pericardial fluid than plasma, but when adjusting for total protein level, STC2 was more abundant. STC2 is a glycosylated 45 kDa protein, which is present in the circulation as a 90 kDa disulfide-linked dimeric complex (19). Thus, completely unrestricted transport into the pericardium seems unlikely. Furthermore, covalent disulfide linkage between 2 STC2 molecules and 2 PAPP-A molecules results in a high molecular mass complex of 500 kDa (19). Traversing of this complex from the circulation into the pericardium is not feasible, further supporting the notion that the myocardium or epicardium is the primary source of pericardial PAPP-A. IGFBP-4 exists in a nonglycosylated (26 kDa) and glycosylated (28 kDa) form. Following proteolytic cleavage, 18 kDa NT-IGFBP-4 and 13 kDa CT-IGFBP-4 fragments are generated (14). Thus, all 3 proteins may diffuse freely. Interestingly, the observed levels of IGFBP-4 and its fragments did, however, not correlate with levels in the circulation.
Another possible source of pericardial fluid proteins is the fat surrounding the heart. Epicardial adipose tissue is a visceral fat deposit accumulated between the pericardium and the myocardium, sharing many pathophysiological characteristics with other visceral fat depots (21). It may also cause local inflammation and likely has direct effects on coronary atherosclerosis. A recent study by Conover et al. has shown that PAPP-A is produced in large amount by preadipocytes from human epicardial fat and regulates IGF-I signaling in cardiomyocytes (21). Thus, the authors hypothesize that bioactive products from epicardial fat are disseminated and interact locally with the coronary arteries and heart through the microcirculation, herby accelerating atherosclerotic processes. These findings are in agreement with the present study.
People with T2D are disproportionately affected by heart disease and cardiovascular dysfunction than nondiabetic subjects (46). Several mechanisms may be contributing, including disturbed myocardial energy metabolism, vascular changes, structural changes in collagen, and increased myocardial and epicardial fat fibrosis (47). However, in this study, only few differences in serum IGF protein levels were observed between diabetic and nondiabetic patients, and all protein levels were similar in the pericardial fluid. Thus, with regard to the IGF system, the myocardial transudate does not seem to be altered in T2D and does not explain why these patients have increased risk of diabetic cardiomyopathy among others. However, it should be noted that all patients were medically well treated.
The reductions of approximately 17% in most protein concentrations during surgery were expected, as hemodilution is common during cardiac surgical procedures due to intravenous fluid administration during anesthesia (48, 49). Moreover, approximately 75% of all CABG procedures are performed in a conventional fashion with cardiopulmonary bypass, and priming of the extracorporeal circuit using asanguineous fluid is also a contributor to intraoperative hemodilution (48, 49). The use of preoperational plasma levels in our analyses is justified, as it is more likely to reflect normal conditions. Of note, the level of IGF bioactivity was not affected by hemodilution. IGF bioactivity measurements are known to be relatively independent of the dilution of a sample in vitro, and, thus, this is also likely to be the case when dilution occurs during surgery. The phenomenon is well acknowledged; the buffering capacity of the IGFBPs ensures that IGFs are liberated during dilution to maintain equilibrium (27, 50).
As previously demonstrated (29, 30), high PAPP-A levels were observed in intraoperative plasma sampled from patients following intravenous administration of heparin. A heparin sulfate binding site is located at the C-terminus end of PAPP-A, which likely allows heparin to facilitate the detachment of PAPP-A from the vascular wall (45). Thus, heparin does not result in an increased de novo synthesis of PAPP-A. Quantification of the PAPP-A generated fragments of IGFBP-4 can be used to reflect PAPP-A enzymatic activity, as the IGFBP-4 fragment levels are unaffected by the heparin treatment (30, 13). Importantly, we were able to verify that heparin was not present in pericardial fluid, hence not being the reason for elevated pericardial PAPP-A levels. This finding was anticipated, as the volumes of distribution of heparin are low (31). Thus, heparin is largely confined to the intravascular space and does not enter or reside in the pericardial fluid.
This study has several limitations. It was a cross-sectional, observational investigation and does not provide evidence for causality or mechanistic details at the level of single cells of how IGFBP-4, PAPP-A and STC2 may function together. The patients with T2D were optimally treated and do not represent poorly controlled T2D. For ethical reasons, pericardial fluid could not be obtained from healthy volunteers. However, the unique study material has, despite a limited number of samples, allowed a detailed picture of the IGF system in pericardial fluid versus the circulation.
In conclusion, this study provides evidence that the pericardial cavity of CVD patients contains IGF-I, IGFBP-4, PAPP-A, and STC2, and that the composition, including 15-fold increased PAPP-A level, is likely to augment local IGF activity. Furthermore, the data strengthen the theory that the pericardial cavity is a distinct microenvironment of the heart. It has been speculated that the IGFBP-4/PAPP-A/STC2 axis may possess potential as a target of therapeutic intervention in CVD (9, 51, 52). Indeed, studies into novel driving forces of cardiac dysfunction and atherosclerosis and a more detailed understanding of the effector molecules are necessary to develop future treatment strategies. Thus, the present results substantiate the role of IGFBP-4 and PAPP-A in CVD and encourage further investigation.
Abbreviations
- BMI
body mass index
- CABG
coronary artery bypass grafting
- CT
C-terminal
- CVD
cardiovascular disease
- HbA1c
glycated hemoglobin
- IGF
insulin-like growth factor
- IGFBP-4
IGF binding protein-4
- KIRA
kinase receptor activation assay
- NT
N-terminal
- PAPP-A
pregnancy-associated plasma protein-A
- STC2
stanniocalcin-2
- T2D
type 2 diabetes
- VR
valve replacement
Acknowledgments
The authors are grateful to HyTest Ltd for providing monoclonal antibodies and calibrators for the IGFBP-4 immunoassays. The authors want to thank the patients who participated in this study.
Financial Support: The work was supported by the Odense University Hospital, the University of Southern Denmark, Steno Diabetes Center Odense, the Danish Council for Independent Research (DFF-4092-00098), and the Direktør Kurt Bønnelycke og hustru fru Grethe Bønnelyckes Fond. It was conducted within the frame of the elite research center for individualized medicine in arterial (CIMA) diseases at Odense University Hospital, Denmark.
Author Contributions: R.H., J.F., and J.G.R.D.M. conceived and designed the study. L.M.R., A.I., L.P.R., and J.G.R.D.M. were responsible for patient recruitment and sample collection. R.H., L.M.R., J.F., and J.G.R.D.M. performed the experiments and acquired the data. Data interpretation and statistical analyses were performed by R.H. R.H. drafted the manuscript, which was revised and approved by all authors. R.H. had full access to all data in the study and takes responsibility for the integrity of the data and the accuracy of the data analysis.
Additional Information
Disclosure Summary: The authors declare that they have no conflicts of interest.
Data Availability
The datasets generated during and/or analyzed during the current study are not publicly available but are available from the corresponding author on reasonable request.
References