-
PDF
- Split View
-
Views
-
Cite
Cite
Jaques A. Courtade, Agnieszka M. Klimek-Abercrombie, Yi-Chun Chen, Nirja Patel, Phoebe Y. T. Lu, Cate Speake, Paul C. Orban, Behzad Najafian, Graydon Meneilly, Carla J. Greenbaum, Garth L. Warnock, Constadina Panagiotopoulos, C. Bruce Verchere, Measurement of Pro-Islet Amyloid Polypeptide (1–48) in Diabetes and Islet Transplants, The Journal of Clinical Endocrinology & Metabolism, Volume 102, Issue 7, 1 July 2017, Pages 2595–2603, https://doi.org/10.1210/jc.2016-2773
- Share Icon Share
Abstract
Islet amyloid is a feature of β-cell failure in type 2 diabetes (T2D) and type 1 diabetes (T1D) recipients of islet transplants. Islet amyloid contains islet amyloid polypeptide (IAPP; amylin), a circulating peptide that is produced in β cells by processing of its precursor, proIAPP1-67, via an intermediate form, proIAPP1-48. Elevated proinsulin to C-peptide ratios in the plasma of persons with diabetes suggest defects in β-cell prohormone processing.
Determine whether plasma levels of precursor forms of IAPP are elevated in diabetes.
We developed an immunoassay to detect proIAPP1-48 in human plasma, and we determined the ratio of proIAPP1-48 to mature IAPP in subjects with T1D, T2D, recipients of islet transplants, and healthy controls.
The proIAPP1-48 immunoassay had a limit of detection of 0.18 ± 0.06 pM and cross-reactivity with intact proIAPP1-67 <15%. Healthy individuals had plasma concentrations of proIAPP1-48 immunoreactivity of 1.5 ± 0.2 pM and a proIAPP1-48 to total IAPP ratio of 0.28 ± 0.03. Plasma concentrations of proIAPP1-48 immunoreactivity were not significantly different in subjects with T2D but were markedly increased in T1D recipients of islet transplants. Children and adults with T1D had reduced mature IAPP levels relative to age-matched controls but an elevated ratio of proIAPP1-48 to total IAPP.
The β cells in T1D and islet transplants have impaired processing of the proIAPP1-48 intermediate. The ratio of proIAPP1-48-to-IAPP immunoreactivity may have value as a biomarker of β-cell stress and dysfunction.
Islet amyloid polypeptide (IAPP) is a hormone that is cosecreted with insulin from pancreatic β cells (1, 2). Physiologically, this molecule likely functions as a regulator of satiety and gastric emptying (3). Under conditions of β-cell stress and increased production, IAPP aggregates to form insoluble amyloid plaques that accumulate within pancreatic islets of patients with type 2 diabetes (T2D) (4, 5). Based on positive immunoreactivity against the NH2-terminal region of these forms, these deposits may contain the IAPP precursors proIAPP1-67 and proIAPP1-48 (6, 7). Both precursor forms aggregate in the presence of anionic lipid membranes (8) and may potentiate β-cell failure similarly to aggregates of the mature species (9, 10). Further work in cell lines has demonstrated that impaired processing of human proIAPP results in increased amyloidogenesis and cell death (10). In addition, rescue of human proIAPP processing in cultured mouse islets abolishes amyloid formation and β-cell apoptosis (9). Collectively, these data suggest that unprocessed forms of proIAPP may contribute to islet amyloid formation and β-cell failure in T2D.
Islet hormones are derived from inactive prohormones and undergo a series of proteolytic cleavages and posttranslational modifications before their secretion. In mouse β cells, proIAPP1-67 is cleaved at its C-terminus by prohormone convertase (PC) 1/3 to generate an N-terminally extended intermediate form, proIAPP1-48 (11). This intermediate is then cleaved in the secretory granule by PC2 to generate mature IAPP (12). Interestingly, proIAPP processing closely resembles the pathway for proteolytic conversion of proinsulin to insulin and C-peptide, involving the concerted actions of PC1/3 and PC2 (13–15). An increased ratio of proinsulin to C-peptide has been demonstrated in serum from T2D patients (16,,17), type 1 diabetes (T1D) recipients of islet transplants (16) and recent-onset T1D individuals (18), suggesting that increased circulating proinsulin levels may reflect the inability to generate bioactive insulin by stressed β cells. Akin to impairments in proinsulin processing, impairments in proIAPP processing may also be present in states of β-cell dysfunction (9).
In view of the parallel processing pathways of proinsulin and proIAPP, we sought to determine if IAPP precursors may be markers of β-cell stress and dysfunction. To test this hypothesis, we developed a sandwich-type immunoassay capable of detecting proIAPP1-48 with minimal cross-reactivity to other IAPP species. We assessed proIAPP1-48 levels and the ratio of proIAPP1-48 to total IAPP in healthy individuals, subjects with T1D, T2D, and islet transplant recipients.
Materials and Methods
Immunoassay reagents
Monoclonal mouse antibodies, F002, F064, and F025 (19), raised against human proIAPP-derived peptides were provided by MedImmune (Gaithersburg, MD). Electrode-plated 96-well multiarray plates, secondary antibody, Meso Scale Discovery (MSD) SULFO-TAG labeling reagent, diluent assay buffer 41, and Read Buffer T (4X) detection reagent were purchased from MSD (Rockville, MD). Heterophilic antibody-blocking reagent-1 was purchased from Scantibodies (Santee, CA). The STELLUX Chemi Human Total Proinsulin kit was purchased from American Laboratory Products Company (Salem, NH).
Preparation of synthetic peptides and labeling of antibodies
Synthetic forms of proIAPP1-67 and proIAPP1-48 peptides were generated by Bio-Synthesis (Lewisville, TX). These peptides were dissolved in trifluoroacetic acid overnight at room temperature, lyophilized, and frozen at −80°C to maintain peptides in monomeric form. Synthetic, amidated mature IAPP was purchased from Bachem (Torrance, CA), dissolved in hexafluoroisopropanol, lyophilized as described previously, and stored at −80°C before reconstitution. F064 and F025 monoclonal antibodies were labeled using MSD SULFO-TAG labeling reagent per the manufacturer’s instructions.
Immunoassay protocol
Bare, high-affinity MSD electrode plates were coated with F002 monoclonal antibody (4 µg/mL final in phosphate-buffered saline) and incubated overnight at 4°C without agitation. Plates were blocked at room temperature with 2% bovine serum albumin before the addition of samples. For the generation of standard curves and cross-reactivity studies, synthetic peptides were diluted in assay buffer supplemented with heterophilic antibody-blocking reagent-1 and prepared at concentrations ranging from 0 to 100 pM. Plasma from humans and rodents was diluted twofold in assay buffer to counteract matrix effects. Labeled antibodies (F064 or F025, 1:1000 or 1:2000, respectively) were incubated with samples for 1 hour at room temperature. Chemiluminescent signals were generated by covering wells with Read Buffer 2× and reading plates on the Sector Imager 2400 (MSD). Spike and recovery analysis of proIAPP1-48 peptide was performed by adding peptide at three concentrations into plasma samples from three healthy individuals and comparing it to samples from the same individual without addition of peptide. Intra-assay and interassay coefficients of variation were determined by three independent operators over 2 days (three plates each day). Linearity was tested by diluting a spiked plasma sample of known concentration twofold and determining percent recovery.
Subjects
The study was approved by the Clinical Research Ethics Board at the University of British Columbia and the Research Review Committees at BC Children’s Hospital and Vancouver General Hospital. Written consent was obtained from each individual or from parents before blood collection. Children provided assent where capable. Plasma samples for proIAPP1-48 measurement in T2D and islet transplant recipients were previously used for measurement of proinsulin and C-peptide (16). Islet transplant patients were from the Vancouver Islet Transplant Program at Vancouver General Hospital. All of these patients were on tacrolimus and mycophenolate mofetil, with the exception of one individual who received tacrolimus and sirolimus. These individuals were also taking the following medications: pioglitazone and exenatide (seven patients), pioglitazone (three), or exenatide (two). Of these patients, seven were on insulin therapy, whereas five were insulin independent. Healthy age-matched individuals were used as controls. T2D individuals were not on insulin but were taking the following medications: metformin (eight patients), glyburide (five), gliclazide (two), or no medication (five). We also collected plasma from children 9 to 16 years of age that were classified as healthy (n = 29) or having long-duration T1D (n = 10, >7 years after diagnosis). Additional adult T1D and T2D samples were collected in Seattle under a protocol approved by the Benaroya Research Institute institutional review board and from kidney transplant recipients under a protocol approved by the University of Washington institutional review board.
Plasma collection and hormone measurements
As detailed in Supplemental Table 1, for the majority of measurements and validation tests, blood was collected in heparin tubes in the presence of Roche Protease Inhibitor Cocktail (Laval, Quebec, Canada). Dipeptidyl peptidase IV (DPP-IV) inhibitor (Sigma-Aldrich, Oakville, Ontario, Canada) at a final concentration of 5 µM was also added to samples collected in Vancouver from healthy and adult T2D subjects. Blood was immediately centrifuged at 2000g at 4°C and the plasma layer stored at −80°C before performing enzyme-linked immunosorbent assays (ELISAs). Proinsulin measurements were repeated in samples from islet transplants recipients and T2D individuals as previously reported (16). Plasma samples from the Benaroya Institute were collected in EDTA tubes in the absence of any protease inhibitors, centrifuged within 2 hours of blood draw, aliquoted, and frozen at −80°C until shipping. Platelet-poor plasma samples from University of Washington kidney transplant recipients receiving immune suppression were collected in the absence of protease inhibitors and stored at −80°C until shipping.
DPP-IV degradation assay
Plasma from a healthy individual was collected in the absence of protease inhibitors and spiked with a 10 pM concentration of proIAPP1-48. Six separate samples were incubated for 0, 1, 2, 3, 8, or 24 hours in the presence of DPP-IV inhibitor at 37°C. Each collected sample was diluted into assay buffer and frozen before analysis.
Calculations and statistical analyses
The ratio of proIAPP1-48 to total IAPP was calculated as the concentration of proIAPP1-48 divided by the total concentration of IAPP plus proIAPP1-48. Data are expressed as mean ± standard error of the mean. Normality in subject groups was analyzed using the D’Agostino and Pearson omnibus normality test. Differences between healthy and T1D children were determined using the Mann-Whitney test. Differences between two or more groups were determined via the Kruskal-Wallis test with Dunn post hoc test for multiple comparisons. All statistical analyses were performed using GraphPad Prism 5.0. Values were considered significant at P < 0.05. The inter- and intra-assay coefficient of variation were determined using the same samples across six different plates (three per day) by three independent operators and calculated as the standard deviation divided by the mean.
Results
Development of an ELISA for proIAPP1-48-like immunoreactivity
To generate an immunoassay specific for human IAPP precursors, we used F002, F064, and F025 monoclonal antibodies raised against human proIAPP-derived peptides (Supplemental Fig. 1). For measurement of proIAPP1-48, F002 was used as a capture antibody, F064 as a detection antibody [Fig. 1(a)], and synthetic proIAPP1-48 as standard. Using this antibody pair, cross-reactivity of intact proIAPP1-67 was 15.6 ± 0.9% (relative to proIAPP1-48), whereas mature IAPP was undetectable, as expected [Fig. 1(b), Supplemental Table 2], indicating that this ELISA is relatively specific for proIAPP1-48. To determine whether the ELISA was capable of detecting proIAPP1-48 immunoreactivity synthesized by human islets, we performed serial dilutions of human islet lysates. Plotting the concentration of proIAPP1-48 vs total protein revealed a linear relationship [Fig. 1(c)]. We also measured mature IAPP using F002 as capture antibody and F025 as detection antibody. We found that the mature IAPP ELISA has no detectable cross-reactivity with proIAPP1-67 and detects <20% proIAPP1-48 immunoreactivity compared with mature IAPP (Supplemental Table 2), allowing us to better estimate mature IAPP concentrations by subtracting the contribution of the proIAPP1-48 intermediate. The limit of detection of the mature IAPP immunoassay was 0.021 ± 0.005 pM, whereas the limit of detection of the proIAPP1-48 assay was 0.18 ± 0.06 pM, as determined by three independent operators with either synthetic IAPP or proIAPP1-48 peptides (Supplemental Table 3). Plasma samples spiked with known concentrations of proIAPP1-48 were run on two consecutive days to assess reproducibility (Supplemental Table 4). Inter- and intra-assay coefficient of variation values were <12% and <11%, respectively (Supplemental Table 5). To assess assay linearity, we performed twofold serial dilutions with a sample containing a known concentration of proIAPP1-48 immunoreactivity. This analysis revealed that, at most, a fourfold dilution of plasma was appropriate for measurement of this intermediate (Supplemental Table 6). Because proIAPP1-48 has a putative DPP-IV cleavage site at its NH2-terminus, we performed spike and recovery experiments in the presence of a DPP-IV inhibitor. At 37°C and in the absence of protease inhibitors, proIAPP1-48 immunoreactivity was reduced to <60% after 2 hours, whereas treatment of plasma samples from the same individual with a DPP-IV inhibitor was able to completely preserve the stability of the proIAPP1-48 peptide for at least 3 hours (Supplemental Fig. 2). Treatment of samples with DDP-IV inhibitor resulted in close to 100% percent recovery of the protein intermediate (Supplemental Table 7). To determine whether DPP-IV inhibitor and/or the addition of a protease inhibitor cocktail was important for preserving proIAPP1-48 in samples stored at −80°C, we measured proIAPP1-48 immunoreactivity in samples from four healthy subjects, collected with or without these inhibitors. We found no substantial differences in proIAPP1-48 immunoreactivity regardless of whether these samples were stored in the absence or presence of a protease inhibitor cocktail or DPP-IV inhibitor [Supplemental Fig. 3(A)]. Further, when plasma samples underwent up to three freeze thaws in the absence of any protease or DPP-IV inhibitors, levels of proIAPP1-48 immunoreactivity in plasma samples were not significantly affected [Supplemental Fig. 3(B)]. We determined a proIAPP1-48 concentration of 1.5 ± 0.2 pM and a proIAPP1-48 to total IAPP ratio of 0.28 ± 0.03 in the circulation of healthy individuals.
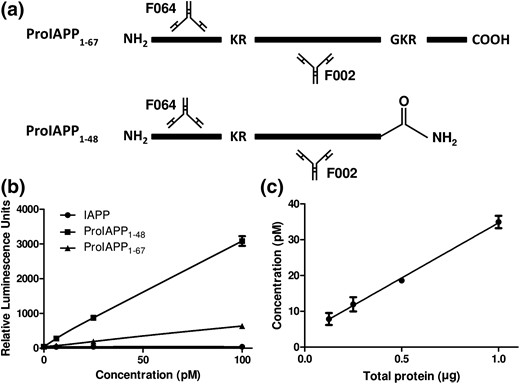
Development of an immunoassay to detect proIAPP1-48-like immunoreactivity. (a) Sandwich-immunoassay for detection of human IAPP precursors. F002 was used as capture antibody and F064 as detection antibody labeled with an MSD SULFO-TAG. (b) Standard curves for synthetic proIAPP1-67, proIAPP1-48, and mature IAPP using F002 as capture and F064 as detection. (c) Detection of proIAPP1-48 in twofold serial dilutions of healthy human islet lysate. Data represented as mean ± standard error of the mean of three technical replicates.
ProIAPP1-48 immunoreactivity in T2D
We assessed proIAPP1-48 immunoreactivity in plasma samples from adults with T2D, which we previously reported to have an increased ratio of total proinsulin to C-peptide (16) (Supplemental Table 7). As previously reported (16), this cohort of patients with T2D tended to be older (70.1 ± 1.2 years), have higher fasting blood glucose (7.0 ± 0.3 mmol/L), and increased hemoglobin A1c (6.4 ± 0.2%) relative to healthy controls (Supplemental Table 7). Patients with T2D had plasma levels of mature IAPP [Fig. 2(a)] and proIAPP1-48 immunoreactivity that were not significantly different than those of controls without diabetes [Fig. 2(b)]. These results were also reflected in the ratio of proIAPP1-48 to total IAPP, which was similar between T2D patients and healthy individuals. Despite displaying no differences in levels of proIAPP1-48-like immunoreactivity, samples from T2D patients had increased total proinsulin levels, as previously reported (16) [Fig. 2(d)]. To verify our findings, we performed these measurements in a separate cohort of patients with T2D from the Benaroya Research Institute. As was observed in the first cohort, we found no substantial differences in IAPP, proIAPP1-48, or the ratio of proIAPP1-48 to total IAPP in T2D subjects compared with healthy controls in this group [Supplemental Fig. 3(A–C)].
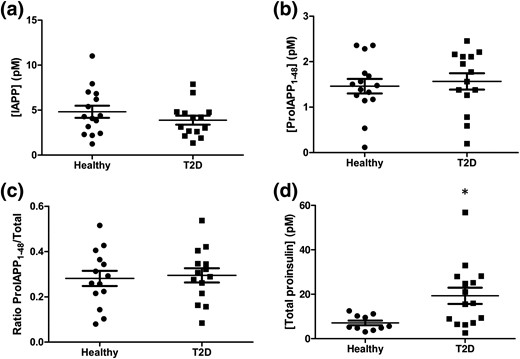
IAPP, proIAPP1-48, and ratio of proIAPP1-48 to total IAPP levels in T2D patients. Plasma concentrations of (a) mature IAPP, (b) proIAPP1-48, (c) the ratio of proIAPP1-48 to total IAPP, and (d) total proinsulin in healthy individuals and T2D patients. Data are expressed as mean ± standard error of the mean of 14 to 15 individuals per group. *P < 0.05.
ProIAPP1-48 immunoreactivity in islet transplant recipients
We next evaluated proIAPP1-48 levels in T1D subjects that had received an islet allograft, a state characterized by increased secretory demand on transplanted β cells (20). Plasma samples from recipients of islet transplants had similar levels of circulating mature IAPP [Fig. 3(a)] and significantly higher levels of proIAPP1-48 immunoreactivity compared with healthy individuals [Fig. 3(b)]. Thus, compared with healthy controls, transplant recipients had an increased ratio of proIAPP1-48 to total IAPP species [Fig. 3(c)], supporting the idea that transplanted β cells may have impaired prohormone processing (16). Because islet allograft recipients receive immune suppression agents that may directly impact β-cell function (21), we also measured proIAPP immunoreactive forms in plasma samples from a Seattle cohort of nondiabetic recipients of kidney transplants who had received similar immunosuppressive regimens, specifically tacrolimus and mycophenolate mofetil. These subjects had modestly lower levels of mature plasma IAPP (3.6 ± 0.9 pM); the ratio of proIAPP1-48 to total IAPP immunoreactivity in these subjects (0.38 ± 0.05) was somewhat, but not markedly, higher than our healthy Vancouver controls (0.28 ± 0.03), and not as high as in islet allograft recipients. Stratification of islet transplant recipients into insulin-dependent and insulin-independent groups revealed elevated levels of IAPP [Supplemental Fig. 5(A)] and proIAPP1-48 immunoreactivity [Supplemental Fig. 5(B)] in the insulin-independent group, but no difference in the ratio of proIAPP1-48 to total IAPP [Supplemental Fig. 3(C)]. It is possible that exogenous insulin treatment in insulin-dependent islet transplant recipients promotes β-cell rest and improved proIAPP processing. As we previously reported, total circulating proinsulin levels were not significantly elevated in this cohort of islet transplant recipients [Fig. 3(d)], although such patients have been demonstrated to have an increased ratio of proinsulin to C-peptide ratio relative to healthy controls (16).
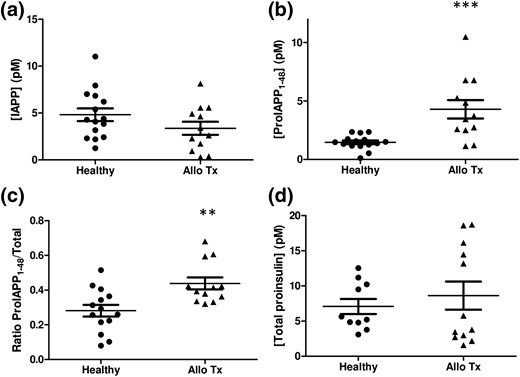
IAPP, proIAPP1-48, and ratios of proIAPP1-48 to total IAPP levels in islet transplant recipients. (a) Mature IAPP concentrations, (b) proIAPP1-48 concentrations, (c) the ratio of proIAPP1-48 to total IAPP, and (d) total proinsulin concentrations in healthy individuals and islet transplant recipients. Data are expressed as mean ± standard error of the mean of 12 to 15 individuals per group. **P < 0.01, ***P < 0.001.
ProIAPP1-48 levels in T1D
We next measured proIAPP1-48 and IAPP levels in a cohort of children with established T1D (>7 years following diagnosis). Subjects had a mean age of 14.0 ± 0.7 years compared with 12.3 ± 0.5 years in controls without diabetes (Table 1). T1D individuals had a mean hemoglobin A1c level of 9.2 ± 0.3% and average nonfasting blood glucose level of 14.0 ± 0.5 mmol/L (Supplemental Table 8). The average diabetes duration was 9.2 ± 0.6 years (Supplemental Table 8). As expected, children with T1D had reduced plasma concentrations of mature IAPP [Fig. 4(a)] compared with healthy age-matched controls, whereas proIAPP1-48 immunoreactivity tended to be lower in T1D subjects [Fig. 4(b)], although this difference was not statistically significant. Notably, the ratio of proIAPP1-48 relative to total IAPP species was markedly higher in patients with T1D (0.613 ± 0.069%) than in healthy controls (0.176 ± 0.020%) [Fig. 4(c)]. Eight individuals with T1D were not included in this ratio calculation because of a lack of detectable IAPP immunoreactivity in the mature IAPP ELISA.
. | Healthy . | T1D . |
---|---|---|
N | 29 | 18 |
Sex (male/female) | 15/14 | 10/8 |
Age (y) | 12.3 ± 0.5 | 14.0 ± 0.7 |
Fasting blood glucose (mmol/L) | 5.3 ± 0.1 | Not measured |
. | Healthy . | T1D . |
---|---|---|
N | 29 | 18 |
Sex (male/female) | 15/14 | 10/8 |
Age (y) | 12.3 ± 0.5 | 14.0 ± 0.7 |
Fasting blood glucose (mmol/L) | 5.3 ± 0.1 | Not measured |
Data are expressed as mean ± standard error of the mean.
. | Healthy . | T1D . |
---|---|---|
N | 29 | 18 |
Sex (male/female) | 15/14 | 10/8 |
Age (y) | 12.3 ± 0.5 | 14.0 ± 0.7 |
Fasting blood glucose (mmol/L) | 5.3 ± 0.1 | Not measured |
. | Healthy . | T1D . |
---|---|---|
N | 29 | 18 |
Sex (male/female) | 15/14 | 10/8 |
Age (y) | 12.3 ± 0.5 | 14.0 ± 0.7 |
Fasting blood glucose (mmol/L) | 5.3 ± 0.1 | Not measured |
Data are expressed as mean ± standard error of the mean.
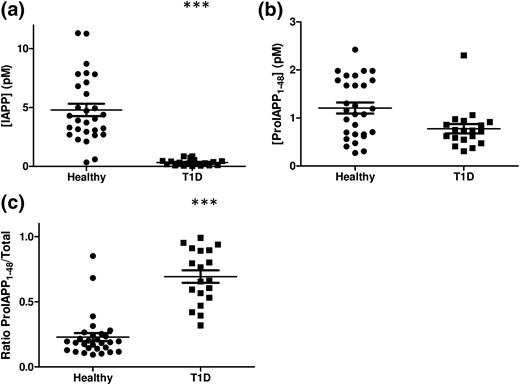
IAPP, proIAPP1-48, and ratios of proIAPP1-48 to total IAPP levels in T1D patients. (a) Mature IAPP concentrations, (b) proIAPP1-48 concentrations, and (c) the ratio of proIAPP1-48 to total IAPP in healthy children and children with T1D. Data are expressed as mean ± standard error of the mean of 18 to 29 individuals per group. ***P < 0.001.
To confirm this finding, we also measured proIAPP1-48 and mature IAPP in a cohort of adult subjects with T1D. Within this group, six of 15 plasma samples had nondetectable levels of mature IAPP (but detectable levels of proIAPP1-48) and were therefore excluded from the analysis. As we observed in T1D children, adults with T1D had reduced IAPP and proIAPP1-48 immunoreactivity relative to healthy controls and tended to have an increased ratio of proIAPP1-48 to total IAPP [Supplemental Fig. 6(A–C)]. To enable inclusion of samples below the level of detectability in the IAPP assay, we assigned a mature IAPP value to these subjects as the limit of detection of the assay divided by the square root of 2 (22). This analysis revealed that the ratio of proIAPP1-48 to total IAPP in these individuals is likely higher than in other T1D subjects [Supplemental Fig. 6(D)], and suggests that, if anything, we may be underestimating the true proIAPP1-48 to IAPP ratio in T1D subjects. As expected, subjects with T1D had undetectable levels of proinsulin and low circulating levels of C-peptide (Supplemental Fig. 7).
Discussion
We developed an ELISA to measure circulating levels of the IAPP precursor intermediate species proIAPP1-48, created by PC1/3 cleavage of proIAPP1-67 (11). Fasting concentrations of proIAPP1-48 averaged 1.5 pM in healthy individuals. The ratio of proIAPP1-48 to total IAPP in circulation was higher than reported values for the ratio of proinsulin to insulin (23, 24). Using this ELISA and a mature IAPP ELISA, we determined plasma levels of IAPP, proIAPP1-48 immunoreactivity, and the ratio of proIAPP1-48 to total IAPP in several patient populations, including T1D, T2D, and islet transplant recipients (Supplemental Table 9). Measurement of this intermediate in circulation suggests that proIAPP1-48, and in particular the ratio of proIAPP1-48 to total IAPP, holds potential as markers of β-cell stress.
Patients with T1D have a drastic reduction in the number of β cells compared with healthy individuals and secrete low levels of insulin and C-peptide. We found that patients with T1D similarly have reduced levels of both proIAPP1-48 and mature IAPP, but a ratio of the proIAPP1-48 intermediate to mature IAPP that is approximately threefold higher than in healthy individuals, suggesting that these individuals have profound defects in proIAPP processing. In agreement with our findings, prediabetic NOD mice (25) and recent-onset T1D patients (18) similarly have elevated circulating proinsulin concentrations, suggesting that β-cell dysfunction in T1D is associated with impairments in the β-cell prohormone processing machinery. β cells in NOD mice and T1D individuals may secrete increased proportions of these prohormone precursors for several reasons, including changes in PC enzyme expression or activity, endoplasmic reticulum (ER) stress (20), or increased β-cell secretory stress in the face of reduced β-cell mass. Whether IAPP precursor levels are elevated in pre-T1D as was previously reported for proinsulin (26) and whether this may have prognostic value has not yet been determined.
Because of the limited availability of human islets, T1D patients who receive islet transplants typically receive a suboptimal mass of islet tissue and often require multiple islet donors. Even with recent improvements in transplant outcomes, fewer than half of patients remain insulin independent at 3 years posttransplant (27). Transplanted human islets can develop extensive amyloid deposition (28, 29), associated with graft dysfunction. Because proIAPP1-48 may be a component of islet amyloid (30), it is possible that the elevated levels of this intermediate form in the circulation of islet transplant recipients contributes to its aggregation and amyloid formation. Under these circumstances, it is plausible that the measurement of precursor species of IAPP in circulation may reflect the progression of amyloidogenesis at the level of the pancreatic islet. Because it is difficult to evaluate whether an islet transplant will fail based on parameters such as the age of the donor or recipients and quality of the islet preparation, having improved biomarkers of impending graft failure would be of considerable value. The elevated proportions of proIAPP1-48 in islet transplant recipients and T1D patients that we observed suggest that it is worthy of further investigation as a biomarker of β-cell dysfunction before graft failure or diabetes onset. One limitation of our study is that, unlike islet transplant recipients, our control subjects were not taking any antirejection medications. Because immune suppressive agents such as glucocorticoids have been shown to affect β-cell function and impair proinsulin processing (31), and other agents such as tacrolimus can directly impair β-cell function (21), we cannot exclude the possibility that such medications are partly responsible for the elevated ratios of proIAPP1-48 to total IAPP that we observed in T1D recipients of islet transplants. Our finding that the proIAPP1-48 to total IAPP immunoreactivity ratio was not markedly elevated in nondiabetic kidney transplant recipients undergoing treatment with tacrolimus and MMF supports the conclusion that, although immune suppressive drugs may contribute, impaired proIAPP processing is a common characteristic of islet transplant recipients.
Given the elevated proinsulin levels in patients with T2D (16, 17), we expected to see elevated circulating proIAPP1-48 concentrations in our T2D patients. Surprisingly, T2D adults and healthy controls had comparable circulating levels of proIAPP1-48 and similar ratios of proIAPP1-48 to total IAPP species. We do not believe that this can be explained by improper sample storage, because proIAPP1-48 immunoreactivity was not affected in samples that underwent multiple freeze thaws, although those experiments were performed over a short period. Further study is needed to assess possible loss of proIAPP immunoreactive forms during longer storage in the presence and absence of protease inhibitors. Moreover, we replicated our findings in an independent cohort of T2D individuals from the Benaroya Research Institute in Seattle. It is possible that IAPP precursors are secreted in higher proportions earlier during the disease course or before disease onset, but not once the disease is fully developed. Although we do not know the circulating half-life of proIAPP1-48, a second possibility is that this intermediate species is more rapidly cleared from circulation in T2D than proinsulin, which has a circulating half-life of 25.6 minutes (32). Finally, because the ELISA detects primarily proIAPP1-48 and not intact proIAPP1-67, it is still possible that proIAPP1-67 (but not proIAPP1-48) is disproportionately elevated in T2D. Elevated proIAPP1-67 levels would be indicative of a processing impairment at the C-terminal end of the peptide, possibly at the level of PC1/3 cleavage (11), and would suggest impairments earlier in the proIAPP processing pathway. Although we are currently developing a proIAPP1-67 ELISA, it is not yet sensitive enough to enable proIAPP1-67 measurement in fasted human plasma. If indeed proIAPP1-67 circulates in plasma, our measurement of total IAPP immunoreactivity (mature plus proIAPP forms) may underestimate true total IAPP immunoreactivity, and, if so, our calculation of the ratio of proIAPP1-48 to total IAPP immunoreactivity may be an overestimate. Moreover, if proIAPP1-67 is altered in states of β-cell dysfunction, it could also affect the ratio of proIAPP1-48 to total IAPP immunoreactivity in diabetes. It will thus be of value to determine whether different disease states are characterized by differences in the proportion of proIAPP1-67 and proIAPP1-48.
In summary, we demonstrate here that proIAPP1-48, an intermediate form produced during β-cell processing of proIAPP to mature IAPP, is detectable in the circulation of humans and is disproportionately elevated relative to mature IAPP in certain conditions of β-cell stress and dysfunction, including T1D and islet transplantation. Our finding that changes in proIAPP1-48 levels do not always parallel those of proinsulin suggests differences in their processing in disease states and raises the possibility that measurement of IAPP precursors may complement those of proinsulin and provide additional insight into β-cell function in disease states.
Abbreviations:
- DPP-IV
dipeptidyl peptidase IV
- ELISA
enzyme-linked immunosorbent assay
- IAPP
islet amyloid polypeptide
- PC
prohormone convertase
- T1D
type 1 diabetes
- T2D
type 2 diabetes.
Acknowledgments
We thank Peter Wunderli and Martin Blankfard from American Laboratory Products Company for their suggestions in enzyme-linked immunosorbent assay development. We also thank Heather Denroche, Paul Yen, Evan Wang, Charles Nieh, and Sophia Lee for assistance in performing immunoassays.
This work was supported by the Canucks for Kids Fund Childhood Diabetes Laboratories for core support. C.B.V. was supported by the Canadian Institutes of Health Research (CIHR; Grant MOP-14682) and JDRF (Grant 3-SRA-2014-39-Q-R), an investigator award from BC Children’s Hospital (BCCH), and the Irving K. Barber Chair in Diabetes Research. C.P. received funding from a Child & Family Research Institute Clinical Research Capacity Building Award from BCCH. P.Y.T.L was supported by a Frederick Banting and Charles Best Canada Graduate Scholarship. J.A.C. was supported by a trainee award from the CIHR Transplant Training Program.
Author contributions: J.A.C., A.M.K.-A., P.C.O., C.P., and C.B.V. contributed to the design and conceptualized the experiments described. N.P. and P.Y.T.L. provided expertise in enzyme-linked immunosorbent optimization and assisted in validation. C.P. and G.L.W. provided clinical samples from subjects. All authors performed a critical analysis of the manuscript and approved the final version to be published. C.B.V. is the guarantor of this work.
Disclosure Summary: The authors have nothing to disclose.
References
Author notes
Address all correspondence and requests for reprints to: C. Bruce Verchere, PhD, Departments of Surgery and Pathology & Laboratory Medicine, BC Children’s Hospital, University of British Columbia, 950 West 28th Avenue, Vancouver, BC V5Z 4H4, Canada. E-mail: [email protected].