-
PDF
- Split View
-
Views
-
Cite
Cite
Mirjana Barjaktarovic, Eric A P Steegers, Vincent W V Jaddoe, Yolanda B de Rijke, Theo J Visser, Tim I M Korevaar, Robin P Peeters, The Association of Thyroid Function With Maternal and Neonatal Homocysteine Concentrations, The Journal of Clinical Endocrinology & Metabolism, Volume 102, Issue 12, 1 December 2017, Pages 4548–4556, https://doi.org/10.1210/jc.2017-01362
- Share Icon Share
Abstract
High homocysteine concentrations are associated with maternal pregnancy complications and low birth weight, jaundice, and cerebrovascular accidents in neonates. Thyroid hormone may interfere with homocysteine metabolism via stimulation of vitamin B12– and folate-dependent processes and via effects on enzymes of the remethylation pathway.
Investigating the associations of maternal and neonatal thyroid function with homocysteine during pregnancy and after delivery, respectively.
Within Generation R study, a population-based prospective cohort, we studied the associations of maternal and neonatal thyroid stimulating hormone (TSH) and free thyroxine (FT4) with homocysteine, folate, and vitamin B12 concentrations using multiple linear regression analyses.
TSH, FT4, homocysteine, folate, and vitamin B12 concentrations were determined in early pregnancy (<18 weeks; N = 1094 women without folic acid supplementation) and in cord blood of 4475 neonates.
In neonates, there was a positive association of FT4 with homocysteine and an inverse association of TSH with homocysteine. The associations attenuated after adjustment for folate and vitamin B12 concentration (β change: for FT4, 0.00559 ± 0.001, P < 0.0001, to 0.00310 ± 0.001, P = 0.015; and for TSH, –0.00165 ± 0.001, P = 0.005, to –0.00086 ± 0.001, P = 0.11). In mothers, there was a positive association of FT4 with homocysteine (P = 0.026) but no association of FT4 with folate or vitamin B12 (P ≥ 0.08).
Higher thyroid function is associated with higher homocysteine concentrations in pregnant women and in neonates. These data provide new insights into the effects of thyroid hormone on folate- and vitamin B12–dependent processes during early growth and development.
High concentrations of homocysteine, a nonprotein amino acid, are associated with adverse coronary, cerebrovascular, and maternal pregnancy-specific outcomes (1–6). In neonates, high homocysteine is associated with low birth weight, jaundice, and cerebrovascular accidents (7–9). In adults, high homocysteine may affect reproductive function and fertility (10) and is associated with neurologic disorders (11). High homocysteine concentrations may have a role in early pregnancy loss, preeclampsia, premature delivery, placental abruption, delayed embryonic development, and congenital birth defects (2–6, 12).
Homocysteine also serves as a marker of folate (vitamin B9) and vitamin B12 (cobalamin) because conversion of Homocysteine to methionine occurs through a remethylation pathway which requires folate and vitamin B12 (13). A deficiency of these vitamins negatively affects the remethylation cycle resulting in higher Homocysteine concentrations (13–16). Folate and vitamin B12 play a key role in important metabolic processes, including DNA and RNA synthesis, amino acid metabolism, cell proliferation and erythropoiesis (14, 15, 17). During fetal development and in the neonatal period, these metabolic and proliferative processes are particularly intense (18).
Thyroid hormone may affect Homocysteine metabolism via two potential mechanisms. First of all, thyroid hormone stimulates folate- and vitamin B12–dependent biochemical processes, including DNA synthesis (19, 20), amino acid metabolism (21), cell proliferation (22, 23), and erythroblast differentiation (24–26). A higher thyroid function and a consequent stimulation of these folate- and vitamin B12–consuming metabolic processes may lead to higher Homocysteine concentrations, as has been suggested previously (27, 28). Secondly, animal studies indicate that thyroid hormone affects the activity of methylenetetrahydrofolate reductase (MTHFR) and methionine synthase, enzymes required for the remethylation of Homocysteine (28). Although some studies in humans showed that thyroid dysfunction is associated with high Homocysteine concentrations (29–31), others did not (32, 33), and population-based studies are lacking.
There is a high demand for folate and vitamin B12 in the developing fetus (18). For that reason, folic acid/multivitamin supplementation is advised from the periconceptional period onwards to prevent neural tube defects (34). Still, high demands for folate and vitamin B12 continue in the neonatal period (18) and determinants of homocysteine metabolism in this period might indirectly have a role in the pathophysiology of clinical events associated with high homocysteine (8, 9). We hypothesized that thyroid function may be a determinant of homocysteine concentration during gestation and in the neonatal period.
The aim of this study was to investigate the association of thyroid function with homocysteine, folate, and vitamin B12 concentrations in pregnant women and in neonates and to explore which of the two potential pathways may play a role.
Methods
Study population
This study was embedded in Generation R, a population-based prospective cohort from early fetal life onwards, in Rotterdam, The Netherlands (35). The study was designed to identify early environmental and genetic causes leading to normal and abnormal growth, development, and health during fetal life and childhood (35). In total, 7069 mothers with expected delivery date between April 2002 and January 2006 were enrolled during early pregnancy. Thyroid stimulating hormone (TSH) and free thyroxine (FT4) were determined in the first available serum sample during early pregnancy (<18 weeks) in 5859 women, from which women with thyroid disease or thyroid (interfering) medication, twin pregnancies and/or IVF were excluded from the analysis (N = 88, N = 64, N = 24, respectively; Fig. 1). From these women, 5683 had homocysteine, folate, or vitamin B12 concentration measurements available (Fig. 1). Furthermore, a subgroup of women (N = 1094; Fig. 1) did not take folic acid supplementation during pregnancy. From the total population of women, 4968 neonates had available cord blood TSH and FT4 measurements available, from which 4475 had data on cord blood homocysteine, folate, or vitamin B12 concentration available (Fig. 1). Written informed consent was obtained from all participants. The general design, research aims and specific measurements in the Generation R study have been approved by the Medical Ethical Committee of the Erasmus Medical Center, Rotterdam.
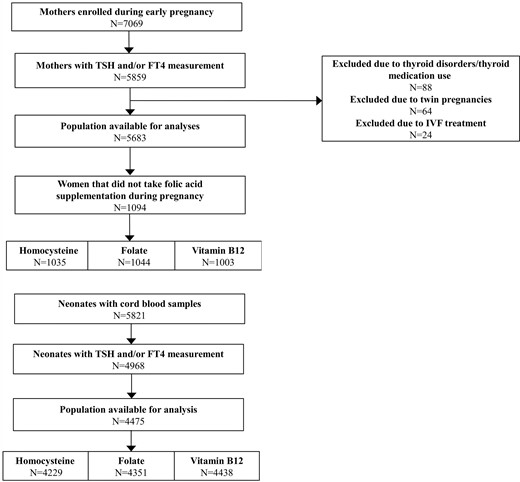
Flowchart showing selection procedure of the study population.
Thyroid function measurements
Maternal serum samples were obtained in early pregnancy [median 13.2 weeks, 95% confidence interval (CI), 9.7 to 17.6 weeks] and cord blood samples were obtained directly after birth (median gestation age at birth = 40.1 weeks, 95% CI, 36.6 to 42.3 weeks). Plain tubes were centrifuged and serum was stored at –80°C. TSH and FT4 concentrations in maternal and cord blood serum samples were determined using chemiluminiscence assays (Vitros ECI; Ortho Clinical Diagnostics). The intra-assay and interassay variation coefficients were <4.1% for TSH at a range of 3.97 to 22.7 mU/mL and <5.4% for FT4 at range of 14.3 to 25.0 pmol/L.
Homocysteine, folate, and vitamin B12 measurements
Maternal and cord blood samples were obtained at the same time as for thyroid function measurements. Total Homocysteine, folate, and total vitamin B12 concentrations were measured using a chemiluminiscence immunoassay on the Architect System (Abbott Diagnostics B.V.) with a between-run variation coefficient ranging from 1.5% to 8.9%. The analytic ranges for Homocysteine, folate, and vitamin B12 were 1 to 50 μmol/L, 1.8 to 45.3 nmol/L, and 44 to 1476 pmol/L, respectively.
Folic acid supplement intake during pregnancy
Information on folic acid supplement use (0.4 to 0.5 mg) and the time of supplement initiation was obtained by questionnaires at study enrollment. We categorized women into three groups: (1) women with no use of supplements, (2) women that started the use during first 10 weeks of pregnancy, and (3) women that started the use periconceptually. The percentage of available data for folic acid supplementation during pregnancy was 75.1%.
MTHFR single nucleotide polymorphism
DNA was extracted from white blood cells obtained from early pregnancy blood samples in mothers and neonates at birth and C677T (rs1801133) and A1298C (rs1801131) were genotyped. In mothers, genotyping was performed using a Taqman allelic discrimination assay (Applied Biosystems) and Abgene quantitative polymerase chain reaction (PCR) Rox mix (Abgene, Hamburg, Germany). The genotyping reaction was amplified by using the GeneAmp PCR system 9600 (95°C for 15 minutes), then 40 cycles of 94°C (15 seconds) and 60°C (1 minute). The fluorescence was detected on the 7900 HT fast real-time PCR system (Applied Biosystems) and individual genotypes were determined by using SDS software (version 2.3; Applied Biosystems) (36). The genotype frequencies of MTHFR C677T were 31.8% (CC), 25.1% (TC), and 5.9% (TT) and A1298C were 30.3% (AA), 26.6% (CA), and 5.9% (CC). In neonates, genotype data were extracted from an imputed genomewide association scan (HapMap phase II release 22). The genotype frequencies of MTHFR C677T were 47.0% (CC), 38.2% (TC), and 8.3% (TT) and A1298C were 47.1% (AA), 37.5% (CA), and 9.7% (CC). The percentage of missing data for MTHFR single nucleotide polymorphisms (SNPs) was 47% in the maternal data (subgroup of women that did not take folic acid supplements) and 6.4% in the neonatal data.
Covariates
Information on ethnicity, educational level and smoking status was obtained by questionnaires at study enrollment. Ethnicity was determined by the country of origin and was defined according to the classification of Statistics Netherlands (35). Maternal smoking was defined as no smoking, smoking until known pregnancy and continued smoking during pregnancy. Information on parity and sex of the child was obtained from hospital registries. Body mass index (BMI) was measured at study enrollment.
Statistical analyses
The association of maternal and neonatal TSH and FT4 with homocysteine, folate, and vitamin B12 concentrations was investigated using multiple linear regression analyses with restricted cubic splines utilizing three knots, to account for possible nonlinear associations. homocysteine, folate, and vitamin B12 values were logarithmically transformed to achieve normal distribution of the residuals of the regression models. Multivariable associations were graphically depicted by plots and β estimates with 95% CIs are shown in Supplemental Tables 1 and 2. To identify associations that are more likely to reflect normal biology and to exclude the effect of folic acid supplements on the associations, main analysis with maternal data was performed in the subgroup of women that did not take any folic acid supplements during pregnancy. To examine whether the association of thyroid function with homocysteine, folate, or vitamin B12 differs based on MTHFR enzyme activity, we tested for interaction by adding product terms of TSH or FT4 and SNP of MTHFR (rs1801133 and 1801131) to the models studying both mothers and neonates. The mediator role of gestational homocysteine concentration in the association of thyroid function with birth weight [described previously (37)] was investigated by performing a mediation analysis using the approach of Imai et al. (38).
All model covariates were selected based on biological plausibility, change of the effect estimate of interest or residual variability in the model. The analyses were adjusted for gestational age at blood sampling, ethnicity, maternal age, educational level, parity, and smoking status in the maternal models and for birth weight standardized to gestational age, ethnicity, maternal age, educational level, parity, and smoking in the neonatal models.
We performed multiple imputation according to Markov chain Monte Carlo method for covariates with missing data (39). Before imputation of the missing values, we performed exploratory analyses by investigating the pattern of missingness for each variable. All variables showed random missingness pattern. In the maternal data, the percentage of missing data were less than 1% for BMI and parity, 4% for ethnicity, 7.1% for education level, and 11.4% for smoking. In the neonatal data, the percentage of missing data were less than 1% for parity and maternal age, 3.6% for ethnicity, 6.7% for maternal education, 2.0% for birthweight, and 10.8% for smoking. Five imputed datasets were created and pooled for analysis. Maternal BMI, parity, educational level, ethnicity, and smoking were then added to the maternal models and maternal age, parity, smoking, education level, birth weight, and ethnicity were added to the neonatal models. Furthermore, we added TSH, FT4 concentrations as well as homocysteine, folate, and vitamin B12 concentrations as prediction variables only. There were no statistically significant differences in descriptive statistics between the original and imputed datasets. Statistical analyses were performed using Statistical Package of Social Sciences version 21.0 for Windows (SPSS Inc., Armonk, NY) and R statistical software version 3.2.1 (“rms” and “mediation” packages).
Results
After exclusions, the final study population consisted of 5683 women, of whom 1094 women did not use pre- or postconceptional folic acid supplements, and 4475 neonates (Fig. 1). Descriptive statistics are shown in Table 1 (descriptives for the total group of women are shown in Supplemental Table 3).
Characteristic: Mothers . | Value . | Characteristic: Neonates . | Value . |
---|---|---|---|
TSH, median (95% CI), mU/L | 1.3 (0.03–3.96) | TSH, median (95% CI), mU/L | 9.12 (3.35–32.9) |
FT4, median (95% CI), pmol/L | 14.5 (10.2–22.6) | FT4, median (95% CI), pmol/L | 20.5 (15.4–28.5) |
Homocysteine, median, (95% CI), μmol/L | 7.4 (4.6–15.0) | Homocysteine, median (95% CI) μmol/L | 9.1 (5.2–16.5) |
Folate, median, (95% CI), nmol/L | 8.6 (4.7–21.3) | Folate, median (95% CI), nmol/L | 20.4 (10.4–38.4) |
Vitamin B12, median, (95% CI), pmol/L | 157.0 (61.1–421.3) | Vitamin B12, median (95% CI), pmol/L | 300.0 (120.0–900.0) |
Gestational age at blood sampling, median (95% CI), wk | 13.9 (9.6–17.8) | Gestational age at birth, median (95% CI), weeks | 40.1 (36.6–42.3) |
Age, mean ± SD, y | 27.8 ± 5.6 | Birth weight, mean ± SD, g | 3463.9 ± 502.9 |
BMI, median, (95% CI), kg/m2 | 23.5 (18.5–35.7) | Sex, n (%) | |
Parity, n (%) | Males | 2272 (50.8) | |
Nullipara | 499 (45.6) | Females | 2202 (49.2) |
Primipara | 334 (30.5) | Ethnicity | |
Multipara | 261 (23.9) | Dutch | 2704 (55.0) |
Smoking status, n (%) | Moroccan | 354 (7.2) | |
Nonsmokers | 756 (69.1) | Turkish | 400 (8.1) |
Stopped smokers | 77 (7.0) | Surinamese | 377 (7.7) |
Smokers | 261(23.9) | Asian | 150 (3.1) |
Educational level, n (%) | Other–European | 355 (7.2) | |
No education or primary education | 273 (25.0) | Other–non-European | 573 (11.7) |
Secondary education | 615 (56.2) | ||
Higher education | 206 (18.8) | ||
Ethnicity, n (%) | |||
Dutch | 231 (21.1) | ||
Moroccan | 163 (14.9) | ||
Turkish | 188 (17.2) | ||
Surinamese | 156 (14.3) | ||
Asian | 51 (4.7) | ||
Other–European | 82 (7.5) | ||
Other–non-European | 223 (20.4) |
Characteristic: Mothers . | Value . | Characteristic: Neonates . | Value . |
---|---|---|---|
TSH, median (95% CI), mU/L | 1.3 (0.03–3.96) | TSH, median (95% CI), mU/L | 9.12 (3.35–32.9) |
FT4, median (95% CI), pmol/L | 14.5 (10.2–22.6) | FT4, median (95% CI), pmol/L | 20.5 (15.4–28.5) |
Homocysteine, median, (95% CI), μmol/L | 7.4 (4.6–15.0) | Homocysteine, median (95% CI) μmol/L | 9.1 (5.2–16.5) |
Folate, median, (95% CI), nmol/L | 8.6 (4.7–21.3) | Folate, median (95% CI), nmol/L | 20.4 (10.4–38.4) |
Vitamin B12, median, (95% CI), pmol/L | 157.0 (61.1–421.3) | Vitamin B12, median (95% CI), pmol/L | 300.0 (120.0–900.0) |
Gestational age at blood sampling, median (95% CI), wk | 13.9 (9.6–17.8) | Gestational age at birth, median (95% CI), weeks | 40.1 (36.6–42.3) |
Age, mean ± SD, y | 27.8 ± 5.6 | Birth weight, mean ± SD, g | 3463.9 ± 502.9 |
BMI, median, (95% CI), kg/m2 | 23.5 (18.5–35.7) | Sex, n (%) | |
Parity, n (%) | Males | 2272 (50.8) | |
Nullipara | 499 (45.6) | Females | 2202 (49.2) |
Primipara | 334 (30.5) | Ethnicity | |
Multipara | 261 (23.9) | Dutch | 2704 (55.0) |
Smoking status, n (%) | Moroccan | 354 (7.2) | |
Nonsmokers | 756 (69.1) | Turkish | 400 (8.1) |
Stopped smokers | 77 (7.0) | Surinamese | 377 (7.7) |
Smokers | 261(23.9) | Asian | 150 (3.1) |
Educational level, n (%) | Other–European | 355 (7.2) | |
No education or primary education | 273 (25.0) | Other–non-European | 573 (11.7) |
Secondary education | 615 (56.2) | ||
Higher education | 206 (18.8) | ||
Ethnicity, n (%) | |||
Dutch | 231 (21.1) | ||
Moroccan | 163 (14.9) | ||
Turkish | 188 (17.2) | ||
Surinamese | 156 (14.3) | ||
Asian | 51 (4.7) | ||
Other–European | 82 (7.5) | ||
Other–non-European | 223 (20.4) |
Characteristic: Mothers . | Value . | Characteristic: Neonates . | Value . |
---|---|---|---|
TSH, median (95% CI), mU/L | 1.3 (0.03–3.96) | TSH, median (95% CI), mU/L | 9.12 (3.35–32.9) |
FT4, median (95% CI), pmol/L | 14.5 (10.2–22.6) | FT4, median (95% CI), pmol/L | 20.5 (15.4–28.5) |
Homocysteine, median, (95% CI), μmol/L | 7.4 (4.6–15.0) | Homocysteine, median (95% CI) μmol/L | 9.1 (5.2–16.5) |
Folate, median, (95% CI), nmol/L | 8.6 (4.7–21.3) | Folate, median (95% CI), nmol/L | 20.4 (10.4–38.4) |
Vitamin B12, median, (95% CI), pmol/L | 157.0 (61.1–421.3) | Vitamin B12, median (95% CI), pmol/L | 300.0 (120.0–900.0) |
Gestational age at blood sampling, median (95% CI), wk | 13.9 (9.6–17.8) | Gestational age at birth, median (95% CI), weeks | 40.1 (36.6–42.3) |
Age, mean ± SD, y | 27.8 ± 5.6 | Birth weight, mean ± SD, g | 3463.9 ± 502.9 |
BMI, median, (95% CI), kg/m2 | 23.5 (18.5–35.7) | Sex, n (%) | |
Parity, n (%) | Males | 2272 (50.8) | |
Nullipara | 499 (45.6) | Females | 2202 (49.2) |
Primipara | 334 (30.5) | Ethnicity | |
Multipara | 261 (23.9) | Dutch | 2704 (55.0) |
Smoking status, n (%) | Moroccan | 354 (7.2) | |
Nonsmokers | 756 (69.1) | Turkish | 400 (8.1) |
Stopped smokers | 77 (7.0) | Surinamese | 377 (7.7) |
Smokers | 261(23.9) | Asian | 150 (3.1) |
Educational level, n (%) | Other–European | 355 (7.2) | |
No education or primary education | 273 (25.0) | Other–non-European | 573 (11.7) |
Secondary education | 615 (56.2) | ||
Higher education | 206 (18.8) | ||
Ethnicity, n (%) | |||
Dutch | 231 (21.1) | ||
Moroccan | 163 (14.9) | ||
Turkish | 188 (17.2) | ||
Surinamese | 156 (14.3) | ||
Asian | 51 (4.7) | ||
Other–European | 82 (7.5) | ||
Other–non-European | 223 (20.4) |
Characteristic: Mothers . | Value . | Characteristic: Neonates . | Value . |
---|---|---|---|
TSH, median (95% CI), mU/L | 1.3 (0.03–3.96) | TSH, median (95% CI), mU/L | 9.12 (3.35–32.9) |
FT4, median (95% CI), pmol/L | 14.5 (10.2–22.6) | FT4, median (95% CI), pmol/L | 20.5 (15.4–28.5) |
Homocysteine, median, (95% CI), μmol/L | 7.4 (4.6–15.0) | Homocysteine, median (95% CI) μmol/L | 9.1 (5.2–16.5) |
Folate, median, (95% CI), nmol/L | 8.6 (4.7–21.3) | Folate, median (95% CI), nmol/L | 20.4 (10.4–38.4) |
Vitamin B12, median, (95% CI), pmol/L | 157.0 (61.1–421.3) | Vitamin B12, median (95% CI), pmol/L | 300.0 (120.0–900.0) |
Gestational age at blood sampling, median (95% CI), wk | 13.9 (9.6–17.8) | Gestational age at birth, median (95% CI), weeks | 40.1 (36.6–42.3) |
Age, mean ± SD, y | 27.8 ± 5.6 | Birth weight, mean ± SD, g | 3463.9 ± 502.9 |
BMI, median, (95% CI), kg/m2 | 23.5 (18.5–35.7) | Sex, n (%) | |
Parity, n (%) | Males | 2272 (50.8) | |
Nullipara | 499 (45.6) | Females | 2202 (49.2) |
Primipara | 334 (30.5) | Ethnicity | |
Multipara | 261 (23.9) | Dutch | 2704 (55.0) |
Smoking status, n (%) | Moroccan | 354 (7.2) | |
Nonsmokers | 756 (69.1) | Turkish | 400 (8.1) |
Stopped smokers | 77 (7.0) | Surinamese | 377 (7.7) |
Smokers | 261(23.9) | Asian | 150 (3.1) |
Educational level, n (%) | Other–European | 355 (7.2) | |
No education or primary education | 273 (25.0) | Other–non-European | 573 (11.7) |
Secondary education | 615 (56.2) | ||
Higher education | 206 (18.8) | ||
Ethnicity, n (%) | |||
Dutch | 231 (21.1) | ||
Moroccan | 163 (14.9) | ||
Turkish | 188 (17.2) | ||
Surinamese | 156 (14.3) | ||
Asian | 51 (4.7) | ||
Other–European | 82 (7.5) | ||
Other–non-European | 223 (20.4) |
Mothers (nonsupplemented)
There was no statistically significant association of TSH with homocysteine [Fig. 2(A), P = 0.06], whereas there was a positive association of FT4 with homocysteine [Fig. 2(B), P = 0.026]. There was an inverse association of TSH with folate [Fig. 2(C), P = 0.004], whereas there was no association of FT4 with folate [Fig. 2(D), P = 0.30]. There was a positive association of TSH with vitamin B12 [Fig. 2(E), P = 0.033], whereas there was no statistically significant association of FT4 with vitamin B12 [Fig. 2(F), P = 0.08]. The association of thyroid function with homocysteine did not change after adjustment for folate and vitamin B12 concentrations (data not shown). The results remained similar after adjustment for thyroid peroxidase (TPO) antibodies or human chorionic gonadotropin concentrations and did not differ according to MTHFR SNPs (Supplemental Table 4). The associations of TSH or FT4 with homocysteine, folate, and vitamin B12 for the total group of women, irrespective of folic acid supplementation status, are shown in Supplemental Fig. 1.
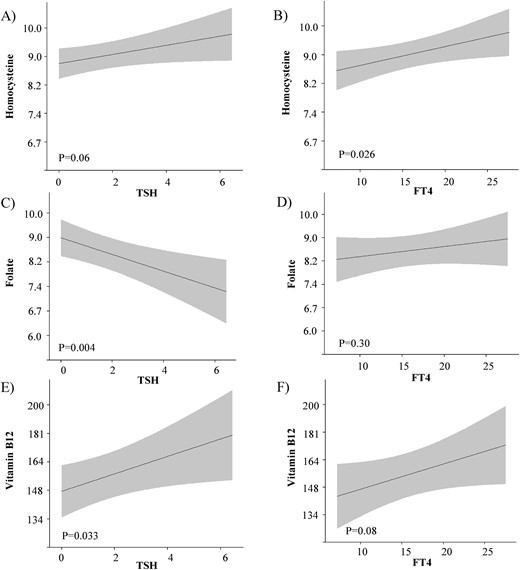
The association of maternal thyroid function with homocysteine, folate, and vitamin B12 concentrations. Plots show the linear regression models for the association of TSH and FT4 with folic acid, vitamin B12, and homocysteine concentrations, as predicted mean with 95% CI in the group of mothers that did not take folic acid supplements anytime during pregnancy. Analyses were adjusted for gestational age at blood sampling, fetal sex, ethnicity, maternal age, educational level, parity, and smoking status.
Neonates
There was an inverse association of TSH with homocysteine [Fig. 3(A), P = 0.005] and a positive association of FT4 with homocysteine [Fig. 3(B), P < 0.0001]. There was a positive association of TSH with folate [Fig. 3(C), P < 0.0001] and an inverse association of FT4 with folate [Fig. 3(D), P = 0.003]. There was no association of TSH with vitamin B12 [Fig. 3(E), P = 0.43], and there was an inverse association of FT4 with vitamin B12 [Fig. 3(F), P < 0.0001].
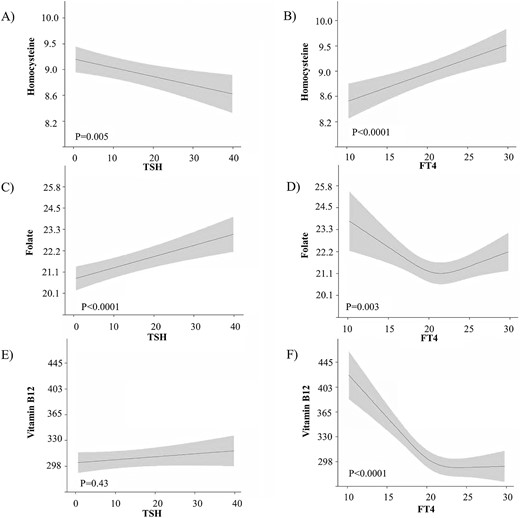
The association of neonatal thyroid function with homocysteine, folate, and vitamin B12 concentrations. Plots show the linear regression models for the association of TSH and FT4 with folic acid, vitamin B12, and homocysteine concentrations, as predicted mean with 95% CI in the neonates. Analyses were adjusted for birth weight standardized for gestational age, fetal sex, ethnicity, maternal age, educational level, parity, and smoking status.
The association of TSH with homocysteine attenuated after adjustment for folate and vitamin B12 [β estimate change: –0.00165 ± 0.00058 (P = 0.005) to –0.00086 ± 0.00054 (P = 0.11)]. Similarly, the association of FT4 with homocysteine attenuated after adjustment for folate and vitamin B12 [β estimate change: 0.00559 ± 0.00138 (P < 0.0001) to 0.00310 ± 0.00127 (P = 0.015)]. Taken together, this indicates that in neonates, roughly 48% to 55% of the association of thyroid function with homocysteine is mediated through changes in folate and vitamin B12.
Although there was a positive association of maternal homocysteine concentrations with neonatal homocysteine concentrations (data not shown), the results remained similar after adjustment for maternal homocysteine, folate, or vitamin B12 concentrations, maternal folate supplementation status and did not differ according to differences in neonatal MTHFR SNPs (Supplemental Table 4). Of all covariates in the models, gestational age at blood measurement and ethnicity had the largest effect on the association studied (up to 36% and 27% change in the effect estimate, respectively).
The mediator role of homocysteine in the association of maternal thyroid function with birth weight
Mediation analysis showed that the effect of FT4 on birth weight was in part mediated through effects of FT4 on homocysteine concentrations (13.3% of mediated effect, P ≤ 0.001; Supplemental Table 5).
Discussion
Our data show an association of thyroid function with homocysteine concentrations in pregnant women and in neonates. To our knowledge, this is the first study that investigates the associations of both maternal and neonatal thyroid function with homocysteine, folate, and vitamin B12 concentrations in a population-based setting. In pregnant women, higher FT4 concentrations were associated with higher homocysteine. In neonates, higher FT4 and lower TSH were associated with higher homocysteine concentrations and this was mediated by the effects of thyroid function on folate and vitamin B12 concentrations.
There is a paucity of population-based studies investigating the association of thyroid function with homocysteine. Some clinical studies suggest that hypothyroid patients have higher homocysteine concentration compared with euthyroid controls (29, 30). Conversely, in patients with thyrotoxicosis, a positive association of FT4 with homocysteine is reported (31). However, due to differences in determinants of homocysteine as well as potential pathological influences in diseased and/or elderly populations, the previous findings are ungeneralizable to women in pregnancy or to children.
We demonstrate that in neonates, higher FT4 and lower TSH concentrations are associated with higher homocysteine concentrations. This suggests that a thyroid hormone-mediated stimulation of metabolic effects may lead to lower availability and/or activity of factors necessary for homocysteine conversion or degradation. In neonates, higher thyroid function was also associated with a lower folate and vitamin B12 concentration, in line with the notion of depleting effects of higher thyroid function on folate and vitamin B12 concentrations (27, 28, 31). This hypothesis is further supported by the attenuation of the association of thyroid function with homocysteine by 48% to 55% after adjustment for folate and vitamin B12 concentration. The growth of fetal tissues, especially in the last trimester, requires intense cell proliferation that is dependent on folate and vitamin B12 concentrations (18). The most important processes that depend on folate and vitamin B12 consumption include DNA synthesis, amino acid metabolism and erythroblast differentiation (19–26). Animal data show that thyroid hormone is essential for adequate erythropoiesis especially at birth (40), in line with a progressive increase in erythrocyte content throughout gestation and the highest values at birth (41). This is in line with the timing of our thyroid function measurement and might in part explain the observed differences between maternal and neonatal analyses.
Animal studies show that hyperthyroidism leads to higher homocysteine concentrations (42–44) and thyroid hormone dependent effects on methionine synthase activity have been suggested (28). In turn, this enzyme is vitamin B12 dependent (28). As such, higher thyroid hormone concentrations may affect the remethylation cycle via lowering of vitamin B12 and or/folate availability, as well as via direct interference with the enzymes of the remethylation cycle. However, our analyses regarding genetic variance in the MTHFR gene did not suggest that MTHFR enzyme is implicated in such mechanisms. Future studies are required to further elucidate pathways through which thyroid hormone affects the metabolic cycle of homocysteine.
In mothers, higher FT4 concentrations were also associated with higher homocysteine concentrations. However, opposite to the results in neonates, this association could not be explained by the relation of FT4 with folate and vitamin B12. This difference between pregnant women and neonates could be explained by various factors. Firstly, diet and vitamin supplements during pregnancy may interfere with homocysteine metabolism (13). To minimize the latter effect, we focused only on women that were not taking folic acid supplements. However, no data were available on vitamin B12 supplementation or other vitamin supplementation. Furthermore, it is likely that some women would use combined (pre)conceptional multivitamin formulas and the availability of our data on supplement usage leaves the possibility of data misclassification. In contrast, the associations in neonates might potentially be less affected by such factors and might represent a better model for studying this association. Secondly, beside the remethylation to methionine, a significant portion of homocysteine is actually converted to cystathionine via vitamin B6-dependent process (13). The positive association of FT4 with homocysteine in mothers could potentially be due to a greater effect of thyroid hormone on homocysteine conversion to cystathionine than on remethylation to methionine. In line with this, lower vitamin B6 concentrations were observed in hyperthyroidism compared with euthyroid state (45). Unfortunately, we do not have vitamin B6 concentrations available to further investigate this hypothesis. In addition, women with a higher general susceptibility to autoimmune disease may have both TPO antibodies and gastric autoantibodies, and the latter could affect vitamin B12 concentrations (46). Therefore, we adjusted all models for TPO antibodies, which did not result in a change in the effect estimates. This suggests that the observed association is unlikely to be confounded by or mediated via higher susceptibility to autoimmunity in general. Finally, hemodilution and hormonal changes in pregnancy might complicate the interpretation of the studied associations with homocysteine (47, 48). Nonetheless, the positive association of maternal thyroid hormone with homocysteine concentration is worth of further investigation, especially considering its potential mediating role in the association of high thyroid hormone with lower birth weight we observed. Therefore, due to potential harmful effects of high homocysteine concentrations that may contribute to adverse pregnancy outcomes, clinical determinants of homocysteine concentrations may be helpful in predicting the risk of pregnancy complications. Future studies should confirm our findings regarding the association of high gestational thyroid hormone with high homocysteine concentrations and further investigate the extent of this effect and its potential role in pregnancy complications. Our analyses showed that gestational age at blood sampling and ethnicity were the most important confounding variables, which indicates that future studies should take into account gestational age-related changes as well as potential genetic differences in thyroid function and homocysteine concentration.
A potential limitation of our study is the availability of a single TSH, FT4, and homocysteine measurement only. This did not enable us to investigate whether the observed associations change in time, particularly in later stages of gestation. However, during pregnancy, both thyroid function (49) and homocysteine concentrations (48) are subject to a relatively low intraindividual change, indicating that no large changes in the current associations can be expected. Another potential limitation of the current study is the fact that a large proportion of pregnant women (47%) had missing data on MTHFR SNPs. Therefore, analyses might have been underpowered to detect potential relevant MTHFR-dependent differences in the association of thyroid function with homocysteine. However, MTHFR-dependent differences were not observed in neonates either, for which data on MTHFR SNPs were available in 93.6%. In addition, we cannot exclude the possibility that the difference in results between mothers and neonates is due to the lack of power to detect similar effects in our subset of women nonsupplemented with folic acid. Finally, the observational nature of this study does not allow for inference of causality and does not exclude the existence of residual confounding.
In conclusion, our study demonstrates that higher thyroid function is associated with higher homocysteine concentration in pregnant women and in neonates. In neonates, the underlying mechanism of this association is likely to involve thyroid hormone mediated stimulation of metabolic processes that require folate and vitamin B12. In pregnant women, different mechanisms seem to underlie the effects of thyroid function on homocysteine metabolism. These data provide new insights into the effects of thyroid hormone on folate and vitamin B12–dependent biochemical processes during early growth and development. Further research is required to investigate the clinical significance of thyroid hormone dependent folate and/or vitamin B12 utilization, and to further elucidate the potential role of thyroid function on vitamin B6 dependent pathway of homocysteine catabolism.
Abbreviations:
- BMI
body mass index
- CI
confidence interval
- FT4
free thyroxine
- MTHFR
methylenetetrahydrofolate reductase
- PCR
quantitative polymerase chain reaction
- SNP
single nucleotide polymorphism
- TPO
thyroid peroxidase
- TSH
thyroid stimulating hormone.
Acknowledgments
The contribution of the endocrine laboratory technicians is highly appreciated. We gratefully acknowledge the contribution of children and parents, general practitioners, hospitals, midwives and pharmacies in Rotterdam. The Generation R study is conducted by the Erasmus Medical Center (Rotterdam) in close collaboration with the School of Law and faculty of Social Sciences of the Erasmus University Rotterdam; the Municipal Health Service Rotterdam area, Rotterdam; the Rotterdam Homecare Foundation, Rotterdam; and the Stichting Trombosedienst and Artsenlaboratorium Rijnmond, Rotterdam. The general design of the Generation R study is made possible by financial support from the Erasmus Medical Center, Rotterdam; the Erasmus University Rotterdam; The Netherlands Organization for Health Research and Development; The Netherlands Organization for Scientific Research; the Ministry of Health, Welfare, and Sport; and the Ministry of Youth and Families. The general design, all research aims and the specific measurements in the Generation R study have been approved by the Medical Ethical Committee of the Erasmus Medical Center, Rotterdam. Written informed consent was obtained from all participants.
Financial Support: This work was supported by a fellowship from ERAWEB, a project funded by the European Commission (to M.B.) and by clinical fellowship from The Netherlands Organization for Health Research and Development (ZonMw), Project 90700412 (to R.P.P.).
Disclosure Summary: The authors have nothing to disclose.
References