-
PDF
- Split View
-
Views
-
Cite
Cite
Yasmina Belarbi, Niklas Mejhert, Silvia Lorente-Cebrián, Ingrid Dahlman, Peter Arner, Mikael Rydén, Agné Kulyté, MicroRNA-193b Controls Adiponectin Production in Human White Adipose Tissue, The Journal of Clinical Endocrinology & Metabolism, Volume 100, Issue 8, 1 August 2015, Pages E1084–E1088, https://doi.org/10.1210/jc.2015-1530
- Share Icon Share
MicroRNAs (miRNAs) are posttranscriptional regulators of gene expression. In white adipose tissue (WAT), recent studies suggest that miRNA levels are altered in various metabolic diseases, including obesity.
The objective of the study was to determine whether adipocyte-expressed miRNAs altered by obesity can regulate adiponectin expression/secretion in fat cells.
Eleven miRNAs previously shown to be altered in obese human WAT were overexpressed in human in vitro-differentiated adipocytes followed by assessments of adiponectin levels in conditioned media.
This was cohort study (n = 56) in an academic hospital.
Subcutaneous WAT was obtained from nonobese and obese individuals.
There were no interventions in this study.
Protein and mRNA levels of adiponectin were measured.
Of the 11 investigated miRNAs, three (miR-193b/-126/-26a) increased adiponectin secretion when overexpressed in human adipocytes. However, in human WAT only miR-193b expression correlated with adiponectin gene expression and homeostasis model assessment of insulin resistance. Moreover, quantitative PCR of miR-193b in both WAT and isolated adipocytes showed a significant association with serum adiponectin levels. Overexpression of miR-193b altered the gene expression of seven known adiponectin regulators. 3′-untranslated region reporter assays confirmed binding to cAMP-responsive element binding protein 5, nuclear receptor interacting protein 1, and nuclear transcription factor Yα. The effects of miR-193b on nuclear transcription factor Yα expression were confirmed at the protein level. Transfection with individual miRNA target protectors selective for nuclear transcription factor Yα and nuclear receptor interacting protein 1 abolished the stimulatory effect of miR-193b on adiponectin secretion.
In human adipocytes, miR-193b controls adiponectin production via pathways involving nuclear transcription factor Yα and possibly nuclear receptor interacting protein 1.
White adipose tissue (WAT) plays an important role in the development of insulin resistance (IR) and type 2 diabetes. Adipocytes present in WAT secrete a number of peptides/proteins, termed adipokines, which affect insulin sensitivity via endo-, para-, and/or autocrine mechanisms (1). In insulin-resistant obese subjects, WAT displays a chronic low-grade inflammation, which is proposed to alter insulin signaling via increased fatty acid release and production of inflammatory adipokines (2). Conversely, the levels of antiinflammatory adipokines (eg, adiponectin) are reduced in obesity (3). Although the beneficial metabolic effects of adiponectin have been intensively investigated, the molecular mechanisms regulating the expression of this adipokine are not well mapped.
MicroRNAs (miRNAs) are small noncoding RNAs that regulate gene expression posttranscriptionally (4) and influence numerous cellular processes including adipocyte function (5). They either block translation or/and initiate mRNA degradation through complementary binding in the 3′-untranslated regions (UTRs) of target genes. MicroRNAs also act indirectly, eg, by first regulating transcription factors, which, in turn, control the expression of genes (6). Recent reports suggest that several miRNAs are involved in the pathophysiological processes of obesity, diabetes, and IR (5). However, there are currently not much data available on adiponectin regulation by miRNAs. In this work, we screened 11 adipocyte-expressed miRNAs, previously shown to be perturbed in obesity (7), for their possible involvement in regulating fat cell adiponectin production.
Materials and Methods
Clinical material
Cohort 1 comprised 30 obese [body mass index (BMI) > 30 kg/m2] and 26 nonobese (BMI < 30 kg/m2) healthy women free of continuous medication. The cohort, biopsy procedures, and WAT collection have been described in detail previously (7). For in vitro studies, sc WAT was obtained from healthy men and women undergoing cosmetic liposuction. In this experimental group, there was no selection for age, sex, or BMI. The study was approved by the regional Board of Ethics, and written informed consent was obtained from all participants.
Affymetrix GeneChip human gene 1.0 ST and miRNA array protocols
Transcriptional profiles have been published previously (7) and are available via GEO Profiles (http://www.ncbi.nlm.nih.gov/geoprofiles/, accession number GSE25402).
Cell culture
Isolation and culture of human adipocyte progenitor cells from sc WAT was performed as described (8). 3T3-L1 cells were handled according to the protocols from American Type Culture Collection.
MicroRNA transfection
In vitro-differentiated adipocytes (d 10–12 after the induction) were treated with 40 nM of miRIDIAN miRNA mimics or 60 nM of hairpin inhibitors (Thermo Fisher Scientific) and HiPerFect transfection reagent (QIAGEN) for 48–72 hours according to the manufacturer's protocol. To rule out unspecific effects, control cells were transfected with miRIDIAN miRNA Mimic or hairpin inhibitor nontargeting negative controls (Thermo Fisher Scientific). In some experiments, in vitro-differentiated adipocytes were cotransfected with miScript target protector [containing the miR-193b binding site on nuclear transcription factor Yα (NF-YA) and nuclear receptor interacting protein 1 (NRIP1), 500 nM; QIAGEN] and miR-193b mimics (40 nM) or control reagents (target protector negative control; QIAGEN; and miRNA mimic nontargeting negative control).
RNA isolation, cDNA synthesis, and real-time PCR
Total RNA was extracted from in vitro-differentiated adipocytes using an miRNeasy kit (QIAGEN). Synthesis of cDNA was performed using an miScript II reverse transcription kit (QIAGEN). Quantitative RT-PCR of genes was performed using commercial Taqman probes or Sybro green primers (see Supplemental Methods). Relative gene expression was calculated using the comparative cycle threshold (Ct) method, ie, 2ΔCt-target gene/2ΔCt-reference gene with 18S or LRP10 as an internal control. Expression levels of the reference genes did not differ between groups.
Enzyme-linked immunosorbent assay
Total adiponectin levels in conditioned media from in vitro-differentiated adipocytes were analyzed using an ELISA assay from Mercodia.
Luciferase reporter assay
The luciferase reporter assay was performed in 3T3-L1 cells as described before (7) using reporter vectors containing 3′UTR of cAMP-responsive element binding protein (CREB)-5, MAPK1, NF-YA, NRIP1, protein kinase D3 (PRDK3), and retinoid X receptor-α (RXRA) (GeneCopoeia).
Isolation of nuclei and Western blot
Nuclear extracts from in vitro-differentiated adipocytes transfected with miR-193b mimics were prepared as described (9). Nuclei obtained from approximately 500 000 cells were resuspended in 150 μL of radioimmunoprecipitation assay buffer (10) supplemented with 5 mM NaF, 1 mM Na3VO4, protease inhibitor cocktail set V (Calbiochem), and benzonase (Sigma). Total lysates were prepared as described (7), proteins (10–20 μg) were separated by SDS-PAGE, and Western blot was performed according to standard protocols. Primary antibodies against NF-YA (Santa Cruz Biotechnology), CREB5 (LSBio), lamin A/C (Cell Signaling Technologies), and actin (Sigma-Aldrich) were used. Secondary antibodies mouse/rabbit IgG-horseradish peroxidase were from Sigma-Aldrich. Antibody-antigen complexes were detected by chemiluminescence using an ECL Select Western blotting detection kit (GE Healthcare).
Statistical analyses
Data presented are mean ± SEM. When appropriate, the data were log transformed to obtain normal distribution. Results were analyzed with an unpaired t test or a linear regression.
Results
Screening of microRNAs identifies miR-193b as a regulator of adiponectin production in human adipocytes
To study the impact of miRNAs on adiponectin production in human adipocytes, we used samples from a transfection screen of 11 obesity-associated miRNAs previously described by us (7, 9). We presently measured the levels of adiponectin in the conditioned media from these samples. Overexpression of miR-193b, miR-126, and miR-26a resulted in a significant up-regulation of adiponectin secretion (Figure 1A), in which miR-193b had the most pronounced effect causing an approximately 25% increment.
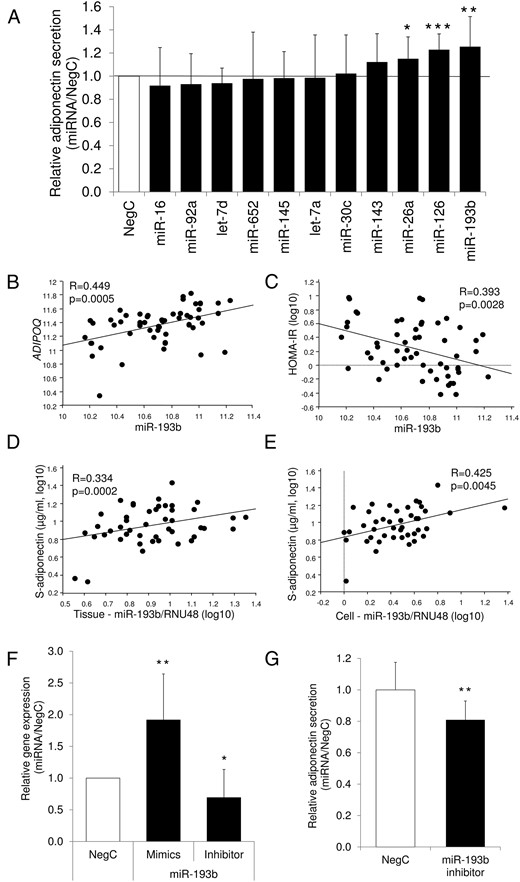
Regulation of adiponectin by miRNAs.
A, Each candidate miRNA was overexpressed in in vitro-differentiated adipocytes, and secreted levels of adiponectin were assessed. Results are representative of three to five biological/independent experiments. WAT was obtained from healthy men and women undergoing cosmetic liposuction. B and C, Association between expression of miR-193b and adiponectin (ADIPOQ) expression or IR. Expression levels of miR-193b were correlated using simple regression with ADIPOQ mRNA levels (B) or HOMAIR index (C) in the 56 subjects of cohort 1. D and E, Association between expression of miR-193b and serum adiponectin. Expression of miR-193b was measured by quantitative RT-PCR in available intact WAT (46 subjects) or isolated fat cells (43 subjects) from cohort 1 and correlated using simple regression with serum adiponectin levels. Levels of miRNA were normalized to the reference gene RNU48 and are shown as relative units. F, ADIPOQ expression levels were measured in in vitro-differentiated adipocytes after overexpression or inhibition of miR-193b. Results are representative of three to six biological/independent experiments. WAT was obtained from healthy men and women undergoing cosmetic liposuction. G, Adiponectin secretion levels were measured in in vitro-differentiated adipocytes after inhibition of miR-193b. Results are representative of five biological/independent experiments. Results in panels A, F, and G were analyzed using a t test and are presented as relative fold change ± vs negative control. To rule out unspecific effects, control cells were transfected with negative control miRNA for mimics or inhibitor. ***, P < .001; **, P < .01; *, P < .05. NegC, nontargeting negative control.
To evaluate the possible clinical significance of these three miRNAs, we determined their expression levels in sc WAT in relation to adiponectin gene expression (ADIPOQ) and in vivo insulin sensitivity [homeostasis model assessment of IR (HOMAIR)]. Only expression of miR-193b correlated with ADIPOQ mRNA and HOMAIR in the cohort of 56 individuals (Figure 1, B and C). Moreover, the expression of miR-193b in intact WAT or isolated fat cells (7) correlated with serum adiponectin levels (Figure 1, D and E). In human in vitro-differentiated adipocytes, the overexpression of miR-193b up-regulated the mRNA levels of ADIPOQ, whereas miR-193b down-regulation resulted in reciprocal effects, at both the mRNA (Figure 1F) and secretion levels (Figure 1G). The miR-193b levels after transfection with mimics or inhibitors are shown in Supplemental Figure 1. The effects of miR-193b in vitro and its association with clinical parameters prompted us to select this miRNA for further studies of adiponectin regulation in human fat cells.
MicroRNA-193b controls adiponectin production via NF-YA and possibly NRIP1
The fact that miRNAs generally down-regulate their predicted targets combined with the finding that miR-193b overexpression up-regulated adiponectin secretion indicated that the mechanisms linking this miRNA to adiponectin were indirect and probably mediated via effects on upstream negative regulators. This hypothesis was supported by bioinformatic analyses, in which ADIPOQ was not a direct target of miR-193b. To identify possible miR-193b regulatory pathways controlling adiponectin production, we searched for predicted miR-193b targets among genes known to affect adiponectin production (Supplemental Table 1). This approach identified 11 negative regulators: adaptor-related protein complex 1, β1 subunit, CREB5 and cAMP-responsive element binding protein-3-like 2, IL-1 receptor-associated kinase 1 (IRAK1), MAPK1, NF-YA, NRIP1, phosphatidylinositol-4-phosphate 3-kinase, catalytic subunit type 2α, protein kinase Cα, PRDK3, and RXRA.
Even if the above-mentioned genes were predicted targets of miR-193b in at least three databases, they have not been validated experimentally. We therefore measured the mRNA expression of the genes in adipocytes with altered levels of miR-193b. Overexpression of miR-193b significantly decreased the levels of CREB5, MAPK1, NF-YA, NRIP1, PRKD3, and RXRA, suggesting that the genes are potentially true targets of miR-193b (Figure 2A). Expression of IRAK1 was up-regulated, suggesting a more complex interaction, which is difficult to evaluate experimentally. Therefore, IRAK1 was excluded from further investigations. Luciferase reporter assays confirmed that miR-193b interacted directly with the 3′ UTR region of CREB5, NF-YA, and NRIP1 but not MAPK1, PRDK3, or RXRA (Figure 2B). The effect of miR-193b on NF-YA was confirmed at the protein level (Figure 2C), whereas CREB5 protein, in contrast to the findings at the mRNA level, was increased (Supplemental Figure 2). All tested primary antibodies detecting NRIP1 were unspecific in human adipocytes, thereby precluding analyses of protein expression. Although the effects of miR-193b were confirmed only at the protein level for NF-YA, simultaneous transfection of miR-193b and target protectors corresponding to the miR-193b target sequence in the 3′UTR regions of NF-YA and NRIP1 abolished the effect of miR-193b on adiponectin secretion (Figure 2D).
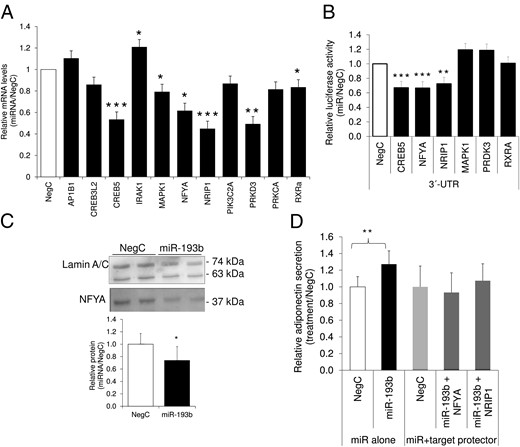
MicroRNA-193b controls adiponectin via negative regulators NF-YA and NRIP1.
A, Expression levels of candidate genes were determined after miR-193b overexpression in human adipocytes differentiated in vitro. Results are based on three to five biological/independent experiments. B, A subset of reporter constructs were transfected with miR-193 mimics, and alterations of luciferase activity were monitored. Results are based on three to four independent experiments. C, MicroRNA-193b was overexpressed in human differentiated adipocytes and nuclear lysates were analyzed by Western blot. Results are representative of three biological/independent experiments, and representative combined blots are shown. D, Interaction of miR-193b with NF-YA and NRIP1 was studied by cotransfecting human in vitro-differentiated adipocytes with miR-193b mimics and target protectors corresponding to the binding site of miR-193b on the 3′UTR of NF-YA or NRIP1 followed by the evaluation of adiponectin secretion by ELISA. To rule out unspecific effects, cells were transfected in parallel with appropriate negative control (nontargeting negative control for miRNA mimics and negative control for target protector). Cells in panels A–D were isolated and differentiated from WAT obtained as described in the legend to Figure 1. Data were analyzed using a t test and are presented as fold change vs negative control ± SEM. ***, P < .001; **, P < .01; *, P < .05. NegC, negative control.
Discussion
The present work demonstrates that miR-193b regulates adiponectin secretion and mRNA expression in human adipocytes. This appears to be mediated through a negative regulatory cascade involving NF-YA and possibly NRIP1. Studies on the role of miRNAs affecting adiponectin have received modest attention so far. Research in the field describes the regulation of miRNAs by adiponectin (11) or the regulation of adiponectin receptor expression by miRNAs (12, 13). In murine cells, miR-21 and miR-378 have been reported to regulate adiponectin expression (14, 15). Our results thus add an additional regulatory layer, namely the regulation of adiponectin itself by miRNA in human adipocytes.
In this study, we linked miR-193b to previously described negative regulators of adiponectin. This set of factors included NRIP1, a corepressor that regulates adiponectin secretion via effects on the nuclear export (16, 17) and NF-YA (a subunit of the transcription factor, nuclear transcription factor Y), which is central for transcriptional regulation of one-third of all eukaryote genes as reviewed (18). NF-YA has not been well characterized in human adipocytes, but in murine cells, the overexpression of NF-YA decreased the basal promoter activity of adiponectin with up to 20% (19). The observation that target protectors specific for these two factors attenuated the effect of miR-193b provides further support to the notion that adiponectin production is controlled via NF-YA and NRIP1. The link between miR-193b, CREB5, and adiponectin production in human adipocytes could not be defined in the present study. Although miR-193b bound to the 3′UTR of CREB5 and attenuated its gene expression, the protein levels were in fact increased. The reasons for these discrepancies remain to be elucidated in further investigations.
It is well established that adiponectin expression is subjected to negative regulation by proinflammatory factors, such as TNFα and IL-6. We recently reported that miR-193b overexpression decreased chemokine ligand-2 and TNFα production in human adipocytes (7, 9), and we could confirm these effects in the samples used in the present study (results not shown). Thus, we cannot exclude that in addition to the regulatory circuits proposed in this study, miR-193b may control adiponectin secretion via its effects on the inflammatory environment.
We observed more pronounced effects on adiponectin mRNA compared with protein in adipocytes with altered miR-193b expression. This may be due to technical limitations of the ELISA kit or the extensive posttranslational modifications that are required for efficient adiponectin multimerization and secretion. Relevant for the latter process, we could not identify any candidates with established roles in posttranslational modifications of adiponectin (eg, endoplasmic reticulum membrane-associated oxireductase-Lα, its associated protein estrogen receptor-p44, or disulfide-bond A oxidoreductase-like protein) as potential miR-193b targets.
In summary, this study identifies a clinically relevant miRNA, miR-193b, which is altered in obese WAT and regulates adiponectin production through a regulatory circuit involving NF-YA and possibly NRIP1. It is tempting to speculate that due to its properties in reducing the secretion of proinflammatory adipokines while increasing insulin sensitizing adiponectin, miR-193 could have a therapeutic potential in the treatment of metabolic complications such as IR. However, adipose-specific miRNA targeting has not yet been tested in clinical trials, and many issues such as side effects and cellular context should be taken into account in designing miRNA therapy.
Acknowledgments
The excellent technical assistance of Gaby Åström, Elisabeth Dungner, Kerstin Wåhlen, and Eva Sjölin is greatly appreciated.
This work was supported by several grants from the Swedish Research Council, the Swedish Diabetes Foundation, Diabetes Wellness, the Diabetes Program, and Center for Innovative Medicine Senior Investigator grants at Karolinska Institutet, the Tore Nilsson Foundation, the Foundation for Gamla Tjänarinnor, the Åke Wiberg Foundation, the European Foundation for the Study of Diabetes/Lilly Program, and the Novo Nordisk Foundation.
Disclosure Summary: The authors have nothing to disclose.
Abbreviations
- BMI
body mass index
- CREB
cAMP-responsive element binding protein
- Ct
cycle threshold
- HOMAIR
homeostasis model assessment of IR
- IR
insulin resistance
- IRAK1
IL-1 receptor-associated kinase 1
- miRNA
microRNA
- NF-YA
nuclear transcription factor Yα
- NRIP1
nuclear receptor interacting protein 1
- RXRA
retinoid X receptor-α
- UTR
untranslated region
- WAT
white adipose tissue.