-
PDF
- Split View
-
Views
-
Cite
Cite
Marketa Kovarova, Ingmar Königsrainer, Alfred Königsrainer, Fausto Machicao, Hans-Ulrich Häring, Erwin Schleicher, Andreas Peter, The Genetic Variant I148M in PNPLA3 Is Associated With Increased Hepatic Retinyl-Palmitate Storage in Humans, The Journal of Clinical Endocrinology & Metabolism, Volume 100, Issue 12, 1 December 2015, Pages E1568–E1574, https://doi.org/10.1210/jc.2015-2978
- Share Icon Share
Previous studies revealed that the common sequence variant I148M in patatin-like phospholipase domain-containing protein 3 (PNPLA3) is associated with liver fat content and liver diseases, but not with insulin resistance. Recent data suggest that the PNPLA3 I148M variant has reduced retinyl-palmitate lipase activity in hepatic stellate cells.
We hypothesized that the PNPLA3 I148M variant is associated with elevated retinyl-palmitate storage in human liver as a potential link to the clinical pathology.
Using HPLC, we quantified the retinoid metabolites in liver tissue extracts obtained from 42 human subjects, including 13 heterozygous and six homozygous carriers of the minor PNPLA3 I148M variant.
Retinyl-palmitate is elevated in human livers of homozygous PNPLA3 I148M allele carriers
The PNPLA3 I148M variant was associated with a significant increase (1.4-fold) in liver fat. The content of retinyl-palmitate was elevated and the ratio of retinol/retinyl-palmitate was reduced in liver extracts obtained from homozygous PNPLA3 I148M minor allele carriers. In a multivariate model including liver fat content, these differences remained significant independent of liver fat content. The content of the minor retinyl-fatty acid esters was similarly increased in homozygous PNPLA3 I148M carriers.
The increased content of hepatic retinyl-palmitate and the reduced ratio of retinol/retinyl-palmitate in PNPLA3 I148M minor allele carriers support in vitro findings of an altered retinyl-palmitate lipase activity. Our results indicate that the PNPLA3 I148M variant is relevant for the retinyl-palmitate content in human liver, providing a possible link to chronic liver disease.
The single nucleotide polymorphism PNPLA3 I148M (rs738409) in the patatin-like phospholipase domain-containing protein 3 (PNPLA3) has been identified as a strong determinant of liver fat content in genome-wide association studies in the Dallas Heart Study (1) and was replicated in many other populations since then (2). The variant furthermore confers an increased risk of chronic liver disease, ranging from hepatitis to hepatocellular carcinoma (3). Paradoxically, despite the increased liver fat content, which generally is a robust determinant of insulin resistance, the PNPLA3 I148M variant was not associated with insulin resistance or diabetes and seems to dissociate liver fat content from the metabolic consequences (4, 5).
Because association studies do not provide a causal explanation for these findings, several groups tried to identify the biological function of the PNPLA3 I148M variant. The observations that genetic deletion of PNPLA3 did not lead to fatty liver under a high-fat diet protocol (6, 7), whereas overexpression of the human PNPLA3 I148M variant, but not the wild-type PNPLA3, caused an increased hepatic triacylglycerol (TAG) accumulation (8) indicated a causal involvement of PNPLA3 I148M in the development of fatty liver. Subsequent studies showed a significantly reduced TAG lipase activity, but also several gains of function of the variant PNPLA3 I148M enzyme. These include lysophosphatidic acid acyltransferase activity and thioesterase activity (9). Furthermore, an altered hepatic triglyceride composition (10) and a reduced hepatic fatty acid synthesis has been described in carriers of the PNPLA3 I148M variant (10, 11). However, none of these findings can fully explain the advanced pathology in human liver disease.
Recently, Pirazzi et al (12) reported that PNPLA3 hydrolyzes retinyl-palmitate specifically in human hepatic stellate cells (HSC) and that this enzyme activity is markedly reduced in the PNPLA3 I148M variant. Because release of stored retinoids from HSC is a hallmark of stellate cell activation and induction of fibrosis, it is feasible to postulate that PNPLA3 I148M determines the susceptibility to chronic liver disease (eg, nonalcoholic steatohepatitis, cirrhosis, hepatocellular carcinoma) by interfering with HSC retinoid metabolism (12–14). However, previous reports showed that retinyl-palmitate may also be hydrolyzed by other enzymes such as PNPLA2 or hormone-sensitive lipase (15), indicating that the loss of retinyl-palmitate hydrolytic activity of the PNPLA3 I148M mutant may not be relevant for the increased storage of retinyl-palmitate in HSC of the PNPLA3 I148M variant.
In the present study, we aimed to evaluate the influence of the PNPLA3 I148M variant on the retinoid content in human liver samples.
Materials and Methods
Subjects
Forty-two Caucasian individuals (16 women/26 men; age, 66.2 ± 12.7 years; weight, 75 ± 16 kg; body mass index, 25.3 ± 4.5 kg/m2) undergoing liver surgery (eg, for the resection of solitary hepatic lesions) were included in the present study (Department of General, Visceral and Transplant Surgery at the University of Tübingen). Patients were fasted overnight before collection of the liver biopsies. Subjects tested negative for viral hepatitis and had no liver cirrhosis. Informed written consent was obtained from all participants, and the local medical ethics committee had approved the protocol according to the Helsinki Declaration. Liver samples were taken from normal, nondiseased tissue determined by the pathologist during surgery, immediately frozen in liquid nitrogen and stored at −80° C.
Determination of liver tissue triglyceride content
Tissue samples were homogenized in phosphate-buffered saline containing 1% Triton X-100 with a TissueLyser (Qiagen). To determine the liver fat content, TAG and total protein concentration in the homogenate was quantified using the ADVIA 1800 clinical chemistry analyzer (Siemens Healthcare Diagnostics) and the results were calculated as milligrams/100 mg tissue (%) or relative to protein content, respectively (16).
Determination of the retinol and retinyl-fatty acid esters in liver biopsies
The quantification of retinol and the retinol fatty esters was performed essentially according to Kim et al, with modifications (17). In brief, 100 μl internal standard containing 200 ng/μl retinyl-acetate in ethanol and 100 μl ethanol were added to 200 μl tissue homogenate. The samples were vortexed and 4 ml n-hexane was added. Then the samples were vortexed for 30 seconds two times and centrifuged for 3 minutes at 3000 rpm. The organic upper layer was transferred into a test tube containing 0.5 ml water and was vortexed for 30 seconds. This extraction procedure was repeated two times and the combined supernatants were evaporated under a gentle stream of nitrogen until dryness. The samples were dissolved in 100 μl mobile phase (70% acetonitrile, 15% methanol, 15% methylene-chloride vol/vol) and 20 μl sample volume were applied. The analysis was performed with an Agilent 1100 module equipped with an UV-VIS detector (Agilent). Separation was achieved using reverse-phase Ascentis C18 column (25 cm × 4.6 mm, 5 μm particle size), guarded with a C18 precolumn (2 cm × 4.0 mm, 5 μm particle size) (Sigma-Aldrich). The isocratic flow rate was 1.8 ml/min and the wavelength for detection was 325 nm. Retinol, retinyl acetate (internal standard), retinyl linoleate, retinyl oleate, retinyl palmitate, and retinyl stearate eluted at 2.5, 3.0, 14.4, 17.0, 18.1, and 24.9 minutes, respectively. The following solvents were obtained from Sigma-Aldrich: acetonitrile, methanol, ethanol, methylene chloride, n-hexane, and water (all HPLC purity). Retinol and the retinyl-fatty acid esters were from Biozol. Analytical performance of in-series precision was evaluated with human liver extracts yielding coefficients of variation of 2.6, 14.05, 11.82, and 2.5% for retinol, retinyl linoleate, retinyl oleate, and retinyl-palmitate concentrations of 41.6, 11.08, 7.63, and 179 μmol/L, respectively. The corresponding coefficients of variation for mouse liver extracts were 1.3, 1.43, 4.02, and 3.0% for retinol, retinyl linoleate, retinyl oleate, and retinyl-palmitate concentrations of 25.9, 13.1, 13.4, and 241.6 μmol/L concentrations, respectively. These values were lower than those obtained for human liver extracts because mouse liver contained more retinoid metabolites and the background was lower. To evaluate the accuracy of the entire procedure the extraction was repeated eight times from different pieces of one mouse liver. Coefficients of variation were <10% for retinyl-palmitate and retinol.
Single nucleotide polymorphism genotyping
For genotyping, DNA was isolated from whole blood using a commercial DNA isolation kit (NucleoSpin, Macherey & Nagel). The PNPLA3 I148M (rs738409) mutation was genotyped using Sequenom's massARRAY System with iPLEX software (Sequenom).
Statistical analyses
Data are presented as means ± SDs. Data that were not normally distributed (Shapiro-Wilk W test, P < .05) were transformed to the natural logarithm for statistical analysis. Differences between the genotypes were assessed using the ANOVA (PANOVA), the dominant inheritance (Pdom), and the recessive inheritance model (Prec) with the statistical software package JMP 10.0 (SAS Institute Inc). Univariate associations between variables were tested using Pearson correlation analyses and correlation coefficient r and the P values are reported. To test for covariates (age, body mass index, gender), univariate linear regression analysis was performed with retinol and retinyl-fatty acid esters. Because no association was detected (P > .1 for all), these covariates were not considered for further analysis. Multivariate linear regression analyses were performed to adjust for the effects of hepatic triglyceride content as a covariate and identify independent relationships (software package JMP 10.0; SAS Institute). P < .05 was considered statistically significant.
Results
Increased TAG content in livers of carriers of PNPLA3 I148M
In the studied group of 42 subjects, 13 were heterozygous and six were homozygous carriers of the PNPLA3 I148M variant. The subjects had an average hepatic triglyceride content of 2.14 ± 2.48% (SD) covering a broad range of 0.5–10.9% (1.3–3.9%; 25th–75th percentile). Consistent with previous reports, we found a significant increase (1.4-fold) in hepatic TAG content in carriers of the PNPLA3 I148M variant (Pdom = 0.029) (Figure 1A, Table 1) (1, 5, 10, 11, 18–20).
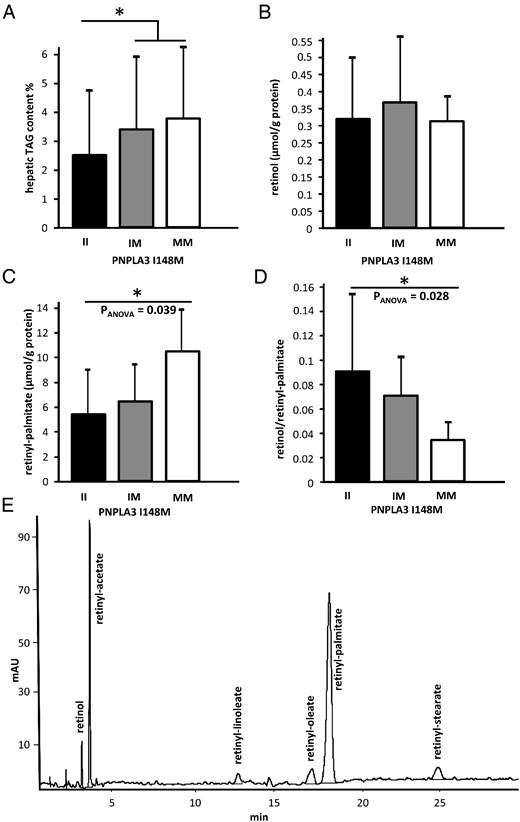
Hepatic triacylglycerol (TAG) and retinyl palmitate content is increased in patatin-like phospholipase domain-containing protein 3 (PNPLA3) I148M carriers.
(A) Hepatic content of TAG in wild-type PNPLA3 I148M II (n = 23) and variant allele carriers (heterozygotes IM [n = 13]; homozygotes MM [n = 6]). Hepatic TAG content is higher in PNPLA3 I148M variant allele carriers (Pdom = .029). Lipids were extracted from liver homogenates and the TAG content was determined using clinical chemical methods. The retinoid metabolites in liver homogenates were separated and quantified by HPLC. (C) The content of retinyl-palmitate (PANOVA = .039, Prec = .016), but not retinol (B), was significantly elevated in livers of PNPLA3 I148M carriers. (D) Ratios of product/substrate (ie, retinol/retinyl-palmitate of PNPLA3 hydrolytic activity) is reduced in biopsies of PNPLA3 I148M carriers (PANOVA = 0.028, Prec = 0.008). Each bar represents the mean ± SD; *P < .05. (E) A representative HPLC chromatogram from a human liver biopsy extract is shown. PANOVA, P values for ANOVA; Prec, P values for the recessive inheritance model; Pdom, P values for the dominant inheritance model.
Association Between PNPLA3I148M Genotype and Hepatic Triglyceride and Retinoid Content
PNPLA3 I148M Genotype . | II . | IM . | MM . | PANOVA . | Pdom . | Prec . |
---|---|---|---|---|---|---|
n = | 23 | 13 | 6 | |||
Liver triglyceride content (%) | 2.53 ± 2.36 | 3.40 ± 2.61 | 3.77 ± 2.47 | .161 | .029 | .253 |
Retinol (μmol/g protein) | 0.319 ± 0.179 | 0.369 ± 0.206 | 0.312 ± 0.081 | .744 | .449 | .848 |
Retinyl-palmitate (μmol/g protein) | 5.51 ± 4.00 | 5.97 ± 3.57 | 10.47 ± 3.35 | .039 | .075 | .016 |
Retinyl-linoleate (μmol/g protein) | 0.259 ± 0.310 | 0.229 ± 0.158 | 0.490 ± 0.403 | .245 | .619 | .047 |
Retinyl-oleate (μmol/g protein) | 0.460 ± 0.405 | 0.435 ± 0.289 | 1.178 ± 0.448 | .008 | .335 | .001 |
Retinol/retinyl-palmitate | 0.089 ± 0.072 | 0.069 ± 0.038 | 0.033 ± 0.018 | .028 | .117 | .008 |
Retinol/retinyl-linoleate | 2.346 ± 1.754 | 2.617 ± 1.967 | 1.409 ± 1.878 | .107 | .610 | .038 |
Retinol/retinyl-oleate | 1.094 ± 0.887 | 1.126 ± 0.722 | 0.307 ± 0.151 | .003 | .357 | .0006 |
PNPLA3 I148M Genotype . | II . | IM . | MM . | PANOVA . | Pdom . | Prec . |
---|---|---|---|---|---|---|
n = | 23 | 13 | 6 | |||
Liver triglyceride content (%) | 2.53 ± 2.36 | 3.40 ± 2.61 | 3.77 ± 2.47 | .161 | .029 | .253 |
Retinol (μmol/g protein) | 0.319 ± 0.179 | 0.369 ± 0.206 | 0.312 ± 0.081 | .744 | .449 | .848 |
Retinyl-palmitate (μmol/g protein) | 5.51 ± 4.00 | 5.97 ± 3.57 | 10.47 ± 3.35 | .039 | .075 | .016 |
Retinyl-linoleate (μmol/g protein) | 0.259 ± 0.310 | 0.229 ± 0.158 | 0.490 ± 0.403 | .245 | .619 | .047 |
Retinyl-oleate (μmol/g protein) | 0.460 ± 0.405 | 0.435 ± 0.289 | 1.178 ± 0.448 | .008 | .335 | .001 |
Retinol/retinyl-palmitate | 0.089 ± 0.072 | 0.069 ± 0.038 | 0.033 ± 0.018 | .028 | .117 | .008 |
Retinol/retinyl-linoleate | 2.346 ± 1.754 | 2.617 ± 1.967 | 1.409 ± 1.878 | .107 | .610 | .038 |
Retinol/retinyl-oleate | 1.094 ± 0.887 | 1.126 ± 0.722 | 0.307 ± 0.151 | .003 | .357 | .0006 |
Data represent means ± sd. For statistical analysis, data were ln-transformed.
Abbreviations: PANOVA, P values for ANOVA; Prec, P values for the recessive inheritance model; Pdom, P values for the dominant inheritance model.
Association Between PNPLA3I148M Genotype and Hepatic Triglyceride and Retinoid Content
PNPLA3 I148M Genotype . | II . | IM . | MM . | PANOVA . | Pdom . | Prec . |
---|---|---|---|---|---|---|
n = | 23 | 13 | 6 | |||
Liver triglyceride content (%) | 2.53 ± 2.36 | 3.40 ± 2.61 | 3.77 ± 2.47 | .161 | .029 | .253 |
Retinol (μmol/g protein) | 0.319 ± 0.179 | 0.369 ± 0.206 | 0.312 ± 0.081 | .744 | .449 | .848 |
Retinyl-palmitate (μmol/g protein) | 5.51 ± 4.00 | 5.97 ± 3.57 | 10.47 ± 3.35 | .039 | .075 | .016 |
Retinyl-linoleate (μmol/g protein) | 0.259 ± 0.310 | 0.229 ± 0.158 | 0.490 ± 0.403 | .245 | .619 | .047 |
Retinyl-oleate (μmol/g protein) | 0.460 ± 0.405 | 0.435 ± 0.289 | 1.178 ± 0.448 | .008 | .335 | .001 |
Retinol/retinyl-palmitate | 0.089 ± 0.072 | 0.069 ± 0.038 | 0.033 ± 0.018 | .028 | .117 | .008 |
Retinol/retinyl-linoleate | 2.346 ± 1.754 | 2.617 ± 1.967 | 1.409 ± 1.878 | .107 | .610 | .038 |
Retinol/retinyl-oleate | 1.094 ± 0.887 | 1.126 ± 0.722 | 0.307 ± 0.151 | .003 | .357 | .0006 |
PNPLA3 I148M Genotype . | II . | IM . | MM . | PANOVA . | Pdom . | Prec . |
---|---|---|---|---|---|---|
n = | 23 | 13 | 6 | |||
Liver triglyceride content (%) | 2.53 ± 2.36 | 3.40 ± 2.61 | 3.77 ± 2.47 | .161 | .029 | .253 |
Retinol (μmol/g protein) | 0.319 ± 0.179 | 0.369 ± 0.206 | 0.312 ± 0.081 | .744 | .449 | .848 |
Retinyl-palmitate (μmol/g protein) | 5.51 ± 4.00 | 5.97 ± 3.57 | 10.47 ± 3.35 | .039 | .075 | .016 |
Retinyl-linoleate (μmol/g protein) | 0.259 ± 0.310 | 0.229 ± 0.158 | 0.490 ± 0.403 | .245 | .619 | .047 |
Retinyl-oleate (μmol/g protein) | 0.460 ± 0.405 | 0.435 ± 0.289 | 1.178 ± 0.448 | .008 | .335 | .001 |
Retinol/retinyl-palmitate | 0.089 ± 0.072 | 0.069 ± 0.038 | 0.033 ± 0.018 | .028 | .117 | .008 |
Retinol/retinyl-linoleate | 2.346 ± 1.754 | 2.617 ± 1.967 | 1.409 ± 1.878 | .107 | .610 | .038 |
Retinol/retinyl-oleate | 1.094 ± 0.887 | 1.126 ± 0.722 | 0.307 ± 0.151 | .003 | .357 | .0006 |
Data represent means ± sd. For statistical analysis, data were ln-transformed.
Abbreviations: PANOVA, P values for ANOVA; Prec, P values for the recessive inheritance model; Pdom, P values for the dominant inheritance model.
Retinyl-palmitate and minor retinyl-fatty acid esters are increased in livers of PNPLA3 I148M carriers
Then we determined the amounts of retinol and retinyl-fatty acid esters in the hepatic tissue lysates. The median amount of retinyl-palmitate per gram of liver tissue detected in our samples was 498 nmol/g wet tissue weight (305–658 nmol/g, 25th–75th percentile), which is well within the previously reported ranges (18). For further analysis, retinoids were normalized to protein concentrations in the liver homogenates.
Retinol content was not different between the genotypes (Figure 1B, Table 1). The content of the storage form retinyl-palmitate, however, was significantly higher in homozygous carriers of the minor allele (II, 5.51 ± 4.00; IM, 5.97 ± 3.57; MM, 10.47 ± 3.35 μmol/g protein; PANOVA = .039) (Figure 1C, Table 1). The most prominent difference occurred between homozygous carriers of the PNPLA3 I148M variant and carriers of one or two wild-type alleles (Prec = 0.016), whereas only a trend was detectable in the dominant model (Pdom = .075). Because the substrate retinyl-palmitate will be hydrolyzed by the activity of PNPLA3 forming the products retinol and palmitate or other fatty acids, we evaluated also the product/substrate ratio. The ratio of retinol and the storage form retinyl-palmitate was lower in the carriers of the PNPLA3 I148M variant (II, 0.089 ± 0.072 ; IM, 0.069 ± 0.038; MM, 0.033 ± 0.018; PANOVA = .028; Prec = .008) (Figure 1D, Table 1).
Apart from retinyl-palmitate, which is the major storage form of retinoids in the liver, we also quantified the retinyl-fatty acid esters of oleate and linoleate, which comprise about 7.5 and 4% of retinyl-palmitate content, respectively (Figure 1E). The retinyl stearate content was low and variable and therefore not further considered. The amounts of retinyl oleate and retinyl linoleate were closely correlated with those of retinyl-palmitate (r = 0.86, P < .0001; r = 0.65, P < .0001, respectively; data not shown). In analogy to retinyl-palmitate, retinyl oleate was present in higher concentrations in homozygous carriers of PNPLA3 I148M (II, 0.460 ± 0.405; IM, 0.435 ± 0.289; MM, 1.178 ± 0.448 μmol/g protein; PANOVA = .008; Prec = .001) and the ratio of retinol/retinyl oleate was lower (II, 1.094 ± 0.887; IM, 1.126 ± 0.722; MM, 0.307 ± 0.151; PANOVA = .003; Prec = .0006). For retinyl linoleate, similar trends were observed; however, with larger variations (II, 0.259 ± 0.310; IM, 0.229 ± 0.158; MM, 0.490 ± 0.403 μmol/g protein; PANOVA = .245; Prec = .047 for retinyl linoleate, and II, 2.346 ± 1.754; IM 2.617 ± 1.967; MM, 1.409 ± 1.878; PANOVA = .107; Padd = .038 for the retinol/retinyl linoleate ratio). These data indicate that the hydrolytic activity of PNPLA3 for retinyl-fatty acid esters is not limited to retinyl-palmitate esters, but also extends to other retinyl-esters with fatty acids like oleate and linoleate, with similar effects of the PNPLA3 I148M variant.
Not retinol, but retinyl-palmitate, as well as the retinol/retinyl-palmitate ratio were significantly associated with hepatic triglyceride content (r = 0.013, P = .938, r = 0.32, P = .041, and r = −0.40, P = .009, respectively) (Figure 2A–C). Therefore, we next tested if the association of hepatic retinoid content with PNPLA3 I148M genotype is independent of hepatic triglyceride content using a multivariate model. After adjusting for hepatic triglyceride content, both the relationships between retinyl-palmitate (II, 5.58 ± 1.66; IM, 5.91 ± 2.15; MM, 10.34 ± 3.18 μmol/g protein (mean ± 95% confidence interval [CI]) Prec = 0.038), as well as the ratio of retinol and retinyl-palmitate (II, 0.087 ± 0.024; IM, 0.072 ± 0.032; MM, 0.038 ± 0.047 [mean ± 95%CI], Prec = .020) were significantly associated with PNPLA3 I148M genotype in homozygous allele carriers (Figure 2D, E). This indicates that the increase of hepatic retinyl-palmitate depends on altered PNPLA3 I148M function independent of increased hepatic triglyceride storage.
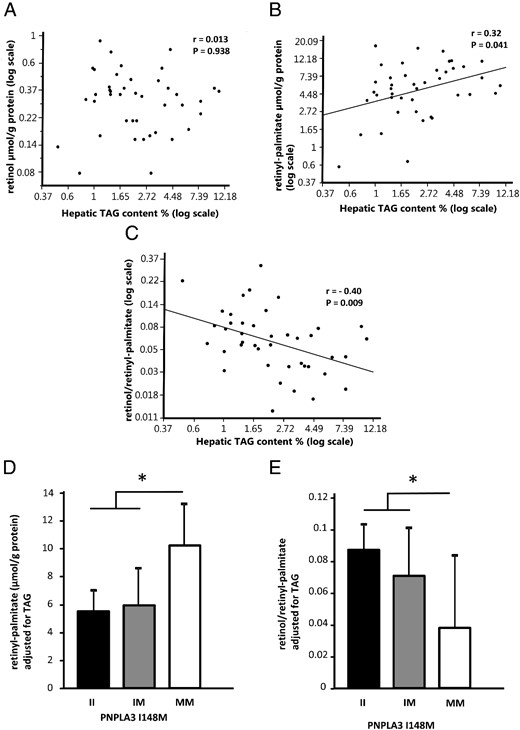
Hepatic retinyl-palmitate content is associated with liver fat content independent of patatin-like phospholipase domain-containing protein 3 (PNPLA3) I148M genotype.
(A) Hepatic triacylglycerol (TAG) content was not correlated with hepatic retinol content in liver tissue samples (r = 0.013, P = .938). (B) The major storage form of hepatic retinoids, retinyl-palmitate showed a positive correlation with liver fat content (r = 0.32, P = .041). (C) The product/substrate ratio of retinol/retinyl-palmitate of PNPLA3 hydrolytic activity was negatively associated with liver fat content (r = −0.40, P = .009). (D) In a multivariate model, taking PNPLA3 I148M genotype as well as liver fat content into account, PNPLA3 I148M was significantly associated with hepatic content of retinyl-palmitate as well as the retinol/retinyl-palmitate ratio (E), independent of liver fat content (Prec = .038, and Prec = .020, respectively). Each bar represents the mean + 95%confidence interval; *P < .05.
Discussion
In the present study, we tested the hypothesis that hepatic retinyl-palmitate storage is affected by the PNPLA3 I148M genotype in humans. Our data show that the homozygous carriers of the PNPLA3 I148M variant have significantly elevated hepatic retinyl-palmitate, retinyl linoleate, and retinyl oleate concentrations and a reduced ratio of free retinol to the storage forms retinyl-palmitate, retinyl-linoleate, and retinyl-oleate. The effect on retinyl-palmitate storage is most prominent in homozygous and does not display strong effects in heterozygous PNPLA3 I148M variant allele carriers (12). Interestingly, the increased risk to develop hepatocellular carcinoma also seems to be limited to the homozygous PNPLA3 I148M allele carriers (21). This is clearly different from other altered enzyme activities of PNPLA3 I148M, particularly the increased hepatic triglyceride storage that is largely present even with one variant allele. Hepatic stellate cells that are located in the space of Disse and comprise only 1% of total liver mass but play an essential role in liver fibrosis (22). Besides “retinal cells,” HSC are the main storage site of retinol; HSC account for 70–80% of total retinol storage the human body. Activation of HSC changes their phenotype to myofibroblast-like cells with an increased production of extracellular matrix and loss of retinol-containing cellular lipid droplets. The involvement of retinoids in this process is supported by several lines of evidence (14). However, the functional mechanism is not fully understood and some observations seem controversial. On the one hand, the concentration of retinoids in liver but not in plasma samples of subjects with nonalcoholic fatty liver disease is increased (23), and excessive amounts of retinoids are associated with the development of hepatic fibrosis. On the other hand, the loss of intracellular retinoid stores is a hallmark of stellate cell activation. Thus the reduced capacity of liberating retinol from retinyl-esters may be protective; however, most the literature on this topic describes that high retinoid stores in stellate cells are an indicator for future liver disease (eg, fibrosis) (14). Furthermore, the biological function of retinol and whether the loss of retinol stores is an early event in HSC activation or a secondary consequence of activation is therefore still unclear. The effect of the PNPLA3 I148M genotype on hepatic retinyl-palmitate storage is independent of liver fat content, which is a determinant of retinyl-palmitate storage in the previous study, as well as ours. We can therefore exclude that the genotype effect is only mediated via the increased hepatic triglyceride content in PNPLA3 I148M carriers.
Previous studies showed that hormone sensitive lipase exerts retinyl-fatty acid esterase activity. However, this enzyme is highly expressed in adipocytes but not in HSC and is responsible for the retinyl-ester hydrolysis in fat tissue (15). Furthermore, the adipocyte TAG lipase PNPLA2, which is also expressed in HSC, has retinyl-palmitate hydrolytic activity and may therefore be involved in regulation of hepatic retinoid metabolism (15). However, deletion of PNPLA2 in mice neither caused retinyl-palmitate accumulation in the liver nor affected retinol plasma levels indicating no important role of PNPLA2 in retinyl-palmitate storage (15). Our results obtained with human liver biopsies clearly show an increased retinyl-fatty acid ester amount in carriers of the PNPLA3 variant, indicating that PNPLA3 is the relevant enzyme for the regulation of retinoid metabolism in human liver.
The study has several limitations: The study population is small and consists of only Caucasian individuals. Furthermore, the individuals were undergoing liver surgery and were not primarily selected to study hepatic lipid or glucose metabolism. Further studies in metabolically phenotyped individuals with and without nonalcoholic fatty liver disease are necessary to confirm the effects of genetic variant I148M in PNPLA3 on hepatic retinoid metabolism and rule out other metabolic influences on the retinyl-palmitate lipase activity of PNPLA3.
Although numerous enzyme activities for PNPLA3 and its variant have been described, so far, few studies are available reporting on the effects of the PNPLA3 I148M variant in humans. These are 1) the TAG hydrolytic activity is markedly reduced in carriers of the allele leading to increased TAG content in human liver (8, 9); 2) the fatty acid composition of the TAG is changed in carriers of the variant (10), however the molecular mechanism for this observation is unknown; and 3) the finding of this report showing that homozygous carriers of the PNPLA3 I148M variant have increased retinyl-fatty acid esters in livers corresponding to the markedly reduced retinyl-palmitate hydrolytic activity shown in a human liver cell line (12).
In summary, we found an increased retinyl-palmitate content in liver biopsies in carriers of the PNPLA3 I148M variant. This finding supports the suggestion that the PNPLA3 I148M variant is relevant for the retinyl-palmitate content in human HSC, providing evidence for a potential link between the PNPLA3 variant, human hepatic retinoid metabolism, and chronic liver disease. Further studies in different cohorts are needed to confirm the general relevance of our finding.
Acknowledgments
This work was supported by Grant GRK 1302/2 from the German Research Foundation. Part of this work was supported by a grant from the German Federal Ministry of Education and Research to the German Centre for Diabetes Research.
Author Contributions: All authors provided substantial contributions to conception and design, acquisition of data or analysis and interpretation of data, drafting the article, or revising it critically for important intellectual content and final approval of the version to be published. A.P. and E.S. designed the study, researched data, and wrote the manuscript. M.K., I.K., F.M., and A.K. researched data and edited the manuscript. H.U.H. contributed to the discussion and reviewed the manuscript.
Disclosure Summary: The authors have nothing to disclose.
Abbreviations
- HSC
hepatic stellate cells
- PNPLA3
patatin-like phospholipase domain-containing protein 3
- TAG
triacylglycerol.