-
PDF
- Split View
-
Views
-
Cite
Cite
Qianqian Pang, Xuan Qi, Yue Chi, Ruizhi Jiajue, Li Zhang, Lijia Cui, Ou Wang, Mei Li, Xiaoping Xing, Yan Jiang, Yiyi Gong, Weibo Xia, Targeting Metabolomics in Primary Hypertrophic Osteoarthropathy: Uncovering Novel Insights into Disease Pathogenesis, The Journal of Clinical Endocrinology & Metabolism, 2024;, dgae737, https://doi.org/10.1210/clinem/dgae737
- Share Icon Share
Abstract
Primary hypertrophic osteoarthropathy (PHO) is a rare genetic disorder characterized by skeletal and skin abnormalities. Genetic defects in prostaglandin E2 (PGE2) metabolism are known to cause PHO. However, the global impact and clinical significance of eicosanoids and oxylipins beyond PGE2 remain to be elucidated.
This study aimed to investigate oxylipin networks in PHO, including the 2 subtypes, PHOAR1 and PHOAR2, and examine their associations with clinical characteristics.
We conducted a targeted metabolomic study involving 16 patients with PHO and 16 age- and sex-matched healthy controls. Serum samples were collected at the time of diagnosis. Metabolites were quantified using ultra-high–performance liquid chromatography-tandem mass spectrometry.
Laboratory analyses confirmed elevated levels of PGE2 in patients with PHO, consistent with the established pathogenesis. About 60 oxidized lipid metabolites were identified, with 19 differentially expressed in PHO. Besides the COX/PGE2 pathway, the lipoxygenase-mediated pathway was also involved in PHO. The metabolites 5-OxoETE, 15-OxoETE, 8S,15S-DiHETE, PGE2, 11β-PGE2, PGB2, LTB4, and LTE4 were significantly altered. Correlation analyses revealed associations between oxylipin metabolites and clinical features, including bone microarchitecture. Notably, the study highlighted differences in the oxylipin metabolite profiles between patients with PHOAR1 and patients with PHOAR2, suggesting distinct metabolic signatures for each subtype.
Our study indicated a significant perturbation in oxylipin metabolism among patients with PHO, with distinct metabolic signatures observed between PHOAR1 and PHOAR2. The disruption extended beyond the metabolism of PGE2. It encompassed a broader alteration across the polyunsaturated fatty acid metabolism spectrum, including various eicosanoids and oxylipins. Our work provided a comprehensive understanding of the pathogenesis of PHO, and underscored the potential for subtype-specific therapeutic interventions.
Primary hypertrophic osteoarthropathy (PHO; MIM: 167100) is a rare genetic disorder characterized by skeletal and skin abnormalities. The primary diagnostic criteria include pachydermia, finger clubbing, and periosteosis (1). Mutations in the HPGD gene encoding 15-hydroxyprostaglandin dehydrogenase, leading to primary hypertrophic osteoarthropathy autosomal recessive type 1 (PHOAR1; OMIM 259100), or mutations in the SLCO2A1 gene encoding the prostaglandin transporter, resulting in primary hypertrophic osteoarthropathy autosomal recessive type 2 (PHOAR2; OMIM 614441), have been identified as the pathogenic genes responsible for PHO (2, 3). Both HPGD and SLCO2A1 genes are involved in the prostaglandin catabolic pathway, with the former responsible for the cytoplasmic oxidation of prostaglandin E2 (PGE2) and the latter for PGE2 uptake across the plasma membrane (4, 5). Laboratory analyses in patients with PHO have consistently shown elevated circulating PGE2 levels, highlighting the crucial role of PGE2 in the pathogenesis of PHO (6, 7).
PGE2 is associated with cell proliferation and angiogenesis, which are seen in the histological changes in clubbing fingers or skin biopsies in patients with PHO (8). In addition, PGE2 is recognized for its crucial role in maintaining bone homeostasis (9), which is also reflected in the skeletal phenotype of PHO. Both osteoclast and osteoblast function could be promoted by PGE2. PGE2 had been reported to affect bone structure and bone strength in animal studies (10, 11). Our previous studies reported alterations in bone microstructure either in distal interphalangeal joints or distal tibia in patients with PHO using high-resolution peripheral quantitative computed tomography (HR-pQCT). It was also revealed that PGE2 levels were closely related to alternations in bone microstructure parameters, indicating a high level of PGE2 might be the reason for the alterations of the bone density and bone structure in patients with PHO (12, 13). However, the exact effect of prostaglandins on skeletal remains to be elucidated.
It has been well acknowledged that the biosynthesis of PGE2 from arachidonic acid (AA) involved a cascade of enzymatic reactions catalyzed by phospholipase A2 (PLA2), cyclooxygenase (COX), and prostaglandin E synthase. The primary therapeutic aim of PHO is to reduce circulating PGE2 levels (8). Nonsteroidal anti-inflammatory drugs (NSAIDs) are conventional options due to their COX-inhibiting properties, therefore reducing the PGE2 levels. Several clinical studies have confirmed NSAIDs’ efficacy in PHO (6, 14). However, the limitations and side effects of NSAIDs (such as gastrointestinal reactions) make it challenging for long-term use. Therefore, exploring alternative therapies aimed at reducing PGE2 levels is urgent and necessary. Currently, inhibitors targeting enzymes related to AA metabolic pathways are an important area of drug discovery (15). AA, an n-6 essential fatty acid, is the direct precursor of bioactive lipid metabolites of eicosanoids. It is hydrolyzed to PLA2, which is in turn further metabolized by COXs and lipoxygenases (LOXs) and cytochrome P450 (CYP) enzymes to a spectrum of bioactive mediators that include prostanoids, leukotrienes (LTs), epoxyeicosatrienoic acids, dihydroxyeicosatetraenoic acid (diHETEs), eicosatetraenoic acid, and lipoxins (16). The potential disruption of eicosanoid metabolism by the accumulation of PGE2 in PHO, whether through metabolic or transport dysfunction, remains uncertain. This uncertainty raised the possibility of competitive inhibition or feedback regulation affecting the metabolism of other mediators in the AA cascades, such as LTs or hydroxyeicosatetraenoic acids, potentially exacerbating the clinical symptoms of PHO. Elucidating these metabolic processes is essential for comprehending the clinical manifestations and underlying mechanisms of PHO and discovering novel therapeutic strategies for symptomatic relief.
Metabolomics is an emerging “omics” method that provides an in-depth investigation of the global small molecules in various biological specimens. It is an essential tool for better understanding pathogenesis, disease diagnosis, and prognosis prediction in many diseases (17, 18). Despite being a systemic metabolic disorder, PHO has a paucity of metabolomic studies. This study utilized a targeted metabolomic approach to analyze a spectrum of metabolites implicated in the prostaglandin metabolic pathway, explicitly focusing on oxylipins and eicosanoids. Through precise quantitative analysis, we could comprehensively assess the alteration in oxylipin networks resulting from the accumulation of PGE2 as an etiology of PHO. The findings of this work might provide a novel insight for understanding the pathogenesis of PHO, potentially revealing new approaches for therapeutic intervention.
Materials and Methods
Study Participants
Sixteen patients with PHO and 16 age- and sex-matched healthy controls (HCs) were enrolled in this study. All the patients with PHO were male and seen in the Department of Endocrinology at Peking Union Medical College Hospital (PUMCH), Beijing, China, from 2018 to 2021. Diagnoses of PHO were initially made based on clinical features, including digital clubbing and pachydermia, and X-ray findings, such as periosteosis, excluding secondary causes, including lung disease, cardiovascular diseases, and inflectional arthritis. Patients with a history of abnormal metabolism and malignancy were also excluded. Cases were subsequently confirmed by HPGD or SLCO2A1 analysis. All of the 16 patients with PHO collected at baseline had no medication use in the past 6 months, especially corticosteroids, NSAIDs, COX-2 inhibitors, pamidronate, and other antibone resorption medications. Sixteen age- and sex-matched HCs were recruited from the PUMCH Outpatient Clinic Physical Examination Center fulfilling the following criteria: (1) no apparent skeletal abnormalities; (2) no history of bone fractures; (3) no joint complaints; (4) no history of mineral metabolism disease, severe renal or liver diseases, gastrointestinal diseases hypertension, or malignancy; (5) no drug exposure in the year before entering the study that could affect bone metabolism (eg, bisphosphonate, teriparatide, glucocorticoid, NSAIDs, thyroxine, and thiazide diuretic) (13) and (6) volunteered to join this study. The study, which was rigorously approved by the Ethics Committee of PUMCH (reference number I-23PJ2019), ensured that informed consent documents were obtained from all participants before entering the study.
Serum Collection and Storage
Fasting whole blood samples of patients and HCs were collected at baseline and placed at room temperature for 30 minutes and then centrifuged at 3000 rpm for 10 minutes to separate serum. The serum was then stored at −80 °C until assayed.
Clinical Characteristic
Biochemistry
Twenty-four–hour urine samples of the recruited participants were collected. The blood samples were centrifuged at 3000 rpm under 4 °C for 10 minutes to obtain the serum for biochemical analysis. The following biochemical markers were assessed at the central laboratory of PUMCH: (1) high sensitivity C-reactive protein (hsCRP), erythrocyte sedimentation rate (ESR), tumor necrosis factor alpha (TNF-α), interleukin 6 (IL-6) by standard methods; (2) special markers: urinary and serum PGE2 by competitive enzyme-linked immunosorbent assay (ELISA) using standard kits (Cayman Chemicals, Ann Arbor, MI, USA, RRID:AB_2924719) following the manufacturer's protocol; (3) markers of bone metabolism: intact parathyroid hormone and C-terminal telopeptide of type I collagen (β-CTX) by an automated Roche electrochemiluminescence system (E170; Roche Diagnostics, Basel, Switzerland). sRANKL (Biomatik, Canada, RRID:AB_3661669) and osteoprotegerin (OPG) levels by sandwich ELISA (Biovendor, Brno, Czech Republic, RRID:AB_3095592) according to the manufacturer's protocol; and (4) markers of cartilage degeneration: matrix metalloproteinase-1 (MMP1) (Abcam, UK, RRID:AB_3661666) and matrix metalloproteinase-13 (MMP13) by sandwich ELISAs according the manufacturer's protocol (Abcam, UK, RRID:AB_3661667).
Bone Mineral Density
Areal bone mineral density (aBMD) was measured by dual-energy x-ray absorptiometry (Prodigy Advance; GE Lunar Corporation, Madison, WI, USA) at the lumbar spine (L1-L4) and proximal femur, including total hip and femoral neck. The aBMD values were indicated as g/cm2 and standardized as Z-score according to the manufacturer's operating procedures for patient samples.
HR-pQCT Scanning
For further analysis of skeletal status, 16 patients with PHO underwent HR-pQCT (XtremeCT II, Scanco Medical, Switzerland), in order to assess bone microarchitecture. Therefore, scans of the nondominant distal radius and distal tibia were conducted. Each image was composed of 168 slices with an isotropic resolution of 61 μm. The scan region was positioned 9.0 mm and 22.0 mm proximal to the reference line for the distal radius and distal tibia, respectively. Imaging acquisition and analysis were according to our previous published methods (14). The following parameters were calculated directly and reported automatically: (1) total volumetric bone mineral density (Tot.vBMD), cortical volumetric bone mineral density (Ct.vBMD), and trabecular volumetric bone mineral density (Tb.vBMD), which were derived from their respective volumes and expressed in mg hydroxyapatite/cm3 (mg HA/cm3); (2) total, trabecular, and cortical bone cross-sectional area, respectively (Tot.Ar, Tb.Ar, and Ct.Ar; mm2); (3) trabecular microstructure, including trabecular bone volume fraction (Tb.BV/TV), trabecular number (Tb.N, 1/mm), trabecular thickness (Tb.Th, mm), trabecular separation (Tb.Sp, mm), and (4) cortical microstructure, including cortical porosity (Ct.Po, %), and cortical thickness (Ct.Th, mm). The patients’ HR-pQCT parameters were compared with the 50th centile of age- and sex-matched reference values provided by a previous study using the same scanner and protocol (19). The changes were calculated and presented in %.
Targeted Metabolomics
Metabolites extraction
Metabolites were extracted using solid-phase extraction columns (Oasis HLB, Waters, USA). Briefly, 100 μL of serum of each individual was mixed with 200 μL of extract solution (methanol, precooled at −40 °C, containing isotopically labeled internal standard mixture). After centrifugating at 12000 rpm for 15 minutes at 4 °C, 200 μL of supernatant of each sample was transferred and mixed with 133 μL of water. Further purification using a solid-phase extraction cartridge was conducted. All samples were eluted with 1 mL of methanol and then dried. Samples were then reconstituted in 100 μL of 30% acetonitrile solution following ultra-high–performance liquid chromatography-tandem mass spectrometry (UHPLC-MS/MS) analysis.
UHPLC-MS Analysis
An EXIONLC UHPLC system equipped with a SCIEX 6500 QTRAP + triple quadrupole mass spectrometer (Sciex) was used for metabolite analysis. A Waters ACQUITY UPLC BEH C18 column (150 × 2.1 mm, 1.7 μm, Waters) was used for sample separation. The mobile phase A for liquid chromatography was 0.01% formic acid in H2O, and the mobile phase B was 0.01% formic acid in acetonitrile. The column temperature was set at 50 °C, and the autosampler temperature was 4 °C. The injection volume was 10 μL. Ion source parameters were set as follows: curtain gas 40 psi, ionspray voltage −4500 V, temperature 500 °C, ion source gas 1 as 30 psi and ion source gas 2 as 30 psi. The standard solutions of all analytes were injected into the atmospheric pressure ionization (API) source of the mass spectrometer, and the multiple reaction monitoring (MRM) parameters of all targeted analytes were then optimized. Collision energy for each Q1/Q3 pair was optimized. The Q1/Q3 pairs with the highest sensitivity and selectivity were selected for quantitative monitoring (Supplementary Table). All MRM data acquisition and processing were performed by SCIEX Analyst Work Station Software (Version 1.6.3) and Multiquant 3.03 software (Version 20.2).
Data Processing and Analysis
Statistical analysis was performed using SPSS version 22.0. The Kolmogorov–Simirnov test was used to test the normality of continuous variables. Normally distributed data were expressed as mean ± SD. Non-normally distributed data were expressed as median (minimum, maximum). The t test and Mann–Whitney U test were used to compare the differences among patients with PHO, patients with PHOAR1, patients with PHOAR2, and HCs, as appropriate.
For the targeting metabolomics analysis, the missing values were filled by half of the minimum value. SIMCA software (V16.0.2, Sartorius Stedim Data Analytics AB, Umea, Sweden) was used for principal component analysis, multivariate statistical analysis, and orthogonal projections to latent structures discriminant analysis (OPLS-DA). The results were visualized in the form of a score plot to show the group clusters. The variable importance in the projection (VIP) plots, based on OPLS-DA analysis, were used to identify and reveal the differential metabolites accountable for the separation between the identified groups. In the univariate analysis, metabolites were identified based on a VIP threshold of 1 from the OPLS-DA model. This identification was further validated at a univariate level with adjusted P < .05, ensuring the reliability of the results. The Benjamini–Hochberg–based false discovery rate (FDR) was performed for multiple testing. Receiver operating characteristic (ROC) and multivariate exploratory ROC analysis were performed to identify and validate candidate biomarkers, achieved through SPSS version 22.0. The area under the ROC curve (AUC) value and 95% CI based on 100 cross-validation were calculated. The chi-square test was used to study the association between metabolite concentrations and clinical features (qualitative variables). Pearson correlation test explored the relationships between metabolite concentrations, clinical features (continuous variables), biochemical markers, and HR-pQCT parameters. In addition, heatmap and metabolic pathway reconstruction were achieved through MetaboAnalyst 5.0 software (http://www.metaboanalyst.ca/) and the Kyoto Encyclopedia of Genes and Genomes (KEGG) (http://www.genome.jp/kegg/)
Results
Clinical Characteristics
Basic participants characteristics
Overall, 16 patients with PHO and 16 HCs were included in the analyses. All of the 16 patients with PHO were males and from unrelated nonconsanguineous Chinese families. Five of the 16 patients with PHO were positive for HPGD mutations, and the remaining 11 patients with PHO were positive for SLCO2A1 mutations (Table 1). Detailed information on these participants’ clinical and biochemical features are depicted in Table 2. The mean chronological age of PHO and HCs was 28.8 ± 7.6 years and 28.5 ± 9.4 years, respectively (P = .345). All patients with PHO were male, with a median onset age of 15 (1, 20) years and a mean disease duration of 15.8 ± 10.1 years. The clinical phenotypes were varied, ranging from mild isolated finger clubbing to severely disabling joint diseases and other severe complications. The clinical spectrum of patients with PHO is displayed in Fig. 1A. Exception for the classic features (pachydermia, finger clubbing, and periosteosis), hyperhidrosis, seborrhea, joint swelling, and arthralgia affected over half of the patients with PHO.
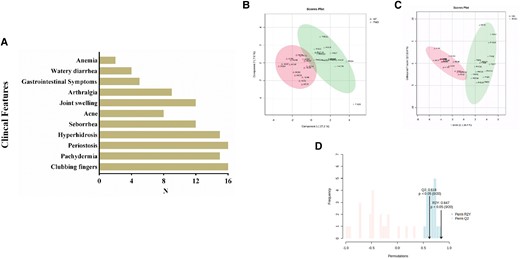
(A) The clinical spectrum of patients with PHO. (B) PLS-DA scores plot, (C) OPLS-DA scores plot, (D) and cumulative values of patients with PHO (N = 16) vs HCs (N = 16).
Patient . | Gene . | Mutation type . | Mutation site . |
---|---|---|---|
P1 | SLCO2A1 | Compound heterozygous | c.1375T>C&c.850A>G |
P2 | SLCO2A1 | Compound heterozygous | c.1375T>C&c.850A>G |
P3 | SLCO2A1 | Homozygous | c.1589G>A |
P4 | SLCO2A1 | Compound heterozygous | c.940+1G>A&c.1624C>T |
P5 | SLCO2A1 | Heterozygous | c.830delT |
P6 | HPGD | Heterozygous | c.310_311delCT |
P7 | SLCO2A1 | Compound heterozygous | c.823T>G&c.1681C>T |
P8 | HPGD | Compound heterozygous | c.205C>T&C.310_311delCT |
P9 | SLCO2A1 | Homozygous | c.855delA |
P10 | SLCO2A1 | Heterozygous | c.131_134GCCA>CCTGT |
P11 | SLCO2A1 | Compound heterozygous | c.1136G>A&c.1070A>G |
P12 | SLCO2A1 | Compound heterozygous | c.941-1G>A&c.1106G>A |
P13 | SLCO2A1 | Compound heterozygous | c.611C>T&c.1624C>T |
P14 | HPGD | Homozygous | c.310_311delCT |
P15 | HPGD | Compound heterozygous | c.548C>A&IVS3:c.324+5G>A |
P16 | HPGD | Homozygous | c.310_311delCT |
Patient . | Gene . | Mutation type . | Mutation site . |
---|---|---|---|
P1 | SLCO2A1 | Compound heterozygous | c.1375T>C&c.850A>G |
P2 | SLCO2A1 | Compound heterozygous | c.1375T>C&c.850A>G |
P3 | SLCO2A1 | Homozygous | c.1589G>A |
P4 | SLCO2A1 | Compound heterozygous | c.940+1G>A&c.1624C>T |
P5 | SLCO2A1 | Heterozygous | c.830delT |
P6 | HPGD | Heterozygous | c.310_311delCT |
P7 | SLCO2A1 | Compound heterozygous | c.823T>G&c.1681C>T |
P8 | HPGD | Compound heterozygous | c.205C>T&C.310_311delCT |
P9 | SLCO2A1 | Homozygous | c.855delA |
P10 | SLCO2A1 | Heterozygous | c.131_134GCCA>CCTGT |
P11 | SLCO2A1 | Compound heterozygous | c.1136G>A&c.1070A>G |
P12 | SLCO2A1 | Compound heterozygous | c.941-1G>A&c.1106G>A |
P13 | SLCO2A1 | Compound heterozygous | c.611C>T&c.1624C>T |
P14 | HPGD | Homozygous | c.310_311delCT |
P15 | HPGD | Compound heterozygous | c.548C>A&IVS3:c.324+5G>A |
P16 | HPGD | Homozygous | c.310_311delCT |
Patient . | Gene . | Mutation type . | Mutation site . |
---|---|---|---|
P1 | SLCO2A1 | Compound heterozygous | c.1375T>C&c.850A>G |
P2 | SLCO2A1 | Compound heterozygous | c.1375T>C&c.850A>G |
P3 | SLCO2A1 | Homozygous | c.1589G>A |
P4 | SLCO2A1 | Compound heterozygous | c.940+1G>A&c.1624C>T |
P5 | SLCO2A1 | Heterozygous | c.830delT |
P6 | HPGD | Heterozygous | c.310_311delCT |
P7 | SLCO2A1 | Compound heterozygous | c.823T>G&c.1681C>T |
P8 | HPGD | Compound heterozygous | c.205C>T&C.310_311delCT |
P9 | SLCO2A1 | Homozygous | c.855delA |
P10 | SLCO2A1 | Heterozygous | c.131_134GCCA>CCTGT |
P11 | SLCO2A1 | Compound heterozygous | c.1136G>A&c.1070A>G |
P12 | SLCO2A1 | Compound heterozygous | c.941-1G>A&c.1106G>A |
P13 | SLCO2A1 | Compound heterozygous | c.611C>T&c.1624C>T |
P14 | HPGD | Homozygous | c.310_311delCT |
P15 | HPGD | Compound heterozygous | c.548C>A&IVS3:c.324+5G>A |
P16 | HPGD | Homozygous | c.310_311delCT |
Patient . | Gene . | Mutation type . | Mutation site . |
---|---|---|---|
P1 | SLCO2A1 | Compound heterozygous | c.1375T>C&c.850A>G |
P2 | SLCO2A1 | Compound heterozygous | c.1375T>C&c.850A>G |
P3 | SLCO2A1 | Homozygous | c.1589G>A |
P4 | SLCO2A1 | Compound heterozygous | c.940+1G>A&c.1624C>T |
P5 | SLCO2A1 | Heterozygous | c.830delT |
P6 | HPGD | Heterozygous | c.310_311delCT |
P7 | SLCO2A1 | Compound heterozygous | c.823T>G&c.1681C>T |
P8 | HPGD | Compound heterozygous | c.205C>T&C.310_311delCT |
P9 | SLCO2A1 | Homozygous | c.855delA |
P10 | SLCO2A1 | Heterozygous | c.131_134GCCA>CCTGT |
P11 | SLCO2A1 | Compound heterozygous | c.1136G>A&c.1070A>G |
P12 | SLCO2A1 | Compound heterozygous | c.941-1G>A&c.1106G>A |
P13 | SLCO2A1 | Compound heterozygous | c.611C>T&c.1624C>T |
P14 | HPGD | Homozygous | c.310_311delCT |
P15 | HPGD | Compound heterozygous | c.548C>A&IVS3:c.324+5G>A |
P16 | HPGD | Homozygous | c.310_311delCT |
Variables . | PHO (n = 16) . | HC (n = 16) . | P value (PHO vs HC) . | Reference value . |
---|---|---|---|---|
Age, year | 28.8 ± 7.6 | 28.5 ± 9.4 | .345 | / |
Disease duration, year | 15.8 ± 10.1 | / | / | / |
Onset age, year | 15 (1, 20) | / | / | / |
Serum PGE2, pg/mL | 383.2 (74.1, 3071.2) | / | / | 28.7∼308.2 |
Urinary PGE2, ng/mmol Cr | 289.6 (62.1, 3620.9) | / | / | 36.4∼85.5 |
RANKL/OPG | 319.4 ± 207.4 | / | / | 87.12∼178.8 |
hsCRP, mg/L | 17.7 ± 14.9 | / | / | 0∼3 |
ESR, mm/h | 17.5 ± 12.4 | / | / | 0∼15 |
iPTH, pg/mL | 36.0 ± 15.5 | / | / | 12∼68 |
β-CTX, ng/mL | 0.77 (0.54, 2.98) | / | / | 0.26∼0.512 |
MMP1, pg/mL | 1228.3 ± 741.9 | / | / | 392.3∼705.9 |
MMP13, pg/mL | 737.5 (374.6, 3251.8) | / | / | 444.21∼632.79 |
IL-6, pg/mL | 4.5 (1.3, 29.0) | / | / | 1.84∼4.04 |
TNF-α, pg/mL | 7.7 ± 2.9 | / | / | <8.2 |
Femoral neck BMD, g/cm2 | 1.0 ± 0.1 | / | / | / |
Z score of femoral neck | 0.7 ± 1.7 | / | / | / |
Total hip BMD, g/cm2 | 1.1 ± 0.2 | / | / | / |
Z score of total hip | 0.9 ± 1.7 | / | / | / |
Lumbar spine BMD, g/cm2 | 1.2 ± 0.2 | / | / | / |
Z score of lumbar spine | 1.1 ± 1.4 | / | / | / |
Variables . | PHO (n = 16) . | HC (n = 16) . | P value (PHO vs HC) . | Reference value . |
---|---|---|---|---|
Age, year | 28.8 ± 7.6 | 28.5 ± 9.4 | .345 | / |
Disease duration, year | 15.8 ± 10.1 | / | / | / |
Onset age, year | 15 (1, 20) | / | / | / |
Serum PGE2, pg/mL | 383.2 (74.1, 3071.2) | / | / | 28.7∼308.2 |
Urinary PGE2, ng/mmol Cr | 289.6 (62.1, 3620.9) | / | / | 36.4∼85.5 |
RANKL/OPG | 319.4 ± 207.4 | / | / | 87.12∼178.8 |
hsCRP, mg/L | 17.7 ± 14.9 | / | / | 0∼3 |
ESR, mm/h | 17.5 ± 12.4 | / | / | 0∼15 |
iPTH, pg/mL | 36.0 ± 15.5 | / | / | 12∼68 |
β-CTX, ng/mL | 0.77 (0.54, 2.98) | / | / | 0.26∼0.512 |
MMP1, pg/mL | 1228.3 ± 741.9 | / | / | 392.3∼705.9 |
MMP13, pg/mL | 737.5 (374.6, 3251.8) | / | / | 444.21∼632.79 |
IL-6, pg/mL | 4.5 (1.3, 29.0) | / | / | 1.84∼4.04 |
TNF-α, pg/mL | 7.7 ± 2.9 | / | / | <8.2 |
Femoral neck BMD, g/cm2 | 1.0 ± 0.1 | / | / | / |
Z score of femoral neck | 0.7 ± 1.7 | / | / | / |
Total hip BMD, g/cm2 | 1.1 ± 0.2 | / | / | / |
Z score of total hip | 0.9 ± 1.7 | / | / | / |
Lumbar spine BMD, g/cm2 | 1.2 ± 0.2 | / | / | / |
Z score of lumbar spine | 1.1 ± 1.4 | / | / | / |
Data are presented as mean ± SD or median (minimum, maximum). Abnormal results are indicated in bold. P value ≤.05 is indicated significance between the 2 groups.
Abbreviations: β-CTX, beta-C-telopeptides of type I collagen; ESR, erythrocyte sedimentation rate; hsCRP, hypersensitive C reactive protein; IL-6, interleukin-6; iPTH, intact parathyroid hormone; MMP1, metalloproteinase-1; MMP13, metalloproteinase-13; RANKL/OPG, the ratio of free soluble receptor activator of nuclear factor-kappa B Ligand to osteoprotegerin; TNF-α, tumor necrosis factor-α.
Variables . | PHO (n = 16) . | HC (n = 16) . | P value (PHO vs HC) . | Reference value . |
---|---|---|---|---|
Age, year | 28.8 ± 7.6 | 28.5 ± 9.4 | .345 | / |
Disease duration, year | 15.8 ± 10.1 | / | / | / |
Onset age, year | 15 (1, 20) | / | / | / |
Serum PGE2, pg/mL | 383.2 (74.1, 3071.2) | / | / | 28.7∼308.2 |
Urinary PGE2, ng/mmol Cr | 289.6 (62.1, 3620.9) | / | / | 36.4∼85.5 |
RANKL/OPG | 319.4 ± 207.4 | / | / | 87.12∼178.8 |
hsCRP, mg/L | 17.7 ± 14.9 | / | / | 0∼3 |
ESR, mm/h | 17.5 ± 12.4 | / | / | 0∼15 |
iPTH, pg/mL | 36.0 ± 15.5 | / | / | 12∼68 |
β-CTX, ng/mL | 0.77 (0.54, 2.98) | / | / | 0.26∼0.512 |
MMP1, pg/mL | 1228.3 ± 741.9 | / | / | 392.3∼705.9 |
MMP13, pg/mL | 737.5 (374.6, 3251.8) | / | / | 444.21∼632.79 |
IL-6, pg/mL | 4.5 (1.3, 29.0) | / | / | 1.84∼4.04 |
TNF-α, pg/mL | 7.7 ± 2.9 | / | / | <8.2 |
Femoral neck BMD, g/cm2 | 1.0 ± 0.1 | / | / | / |
Z score of femoral neck | 0.7 ± 1.7 | / | / | / |
Total hip BMD, g/cm2 | 1.1 ± 0.2 | / | / | / |
Z score of total hip | 0.9 ± 1.7 | / | / | / |
Lumbar spine BMD, g/cm2 | 1.2 ± 0.2 | / | / | / |
Z score of lumbar spine | 1.1 ± 1.4 | / | / | / |
Variables . | PHO (n = 16) . | HC (n = 16) . | P value (PHO vs HC) . | Reference value . |
---|---|---|---|---|
Age, year | 28.8 ± 7.6 | 28.5 ± 9.4 | .345 | / |
Disease duration, year | 15.8 ± 10.1 | / | / | / |
Onset age, year | 15 (1, 20) | / | / | / |
Serum PGE2, pg/mL | 383.2 (74.1, 3071.2) | / | / | 28.7∼308.2 |
Urinary PGE2, ng/mmol Cr | 289.6 (62.1, 3620.9) | / | / | 36.4∼85.5 |
RANKL/OPG | 319.4 ± 207.4 | / | / | 87.12∼178.8 |
hsCRP, mg/L | 17.7 ± 14.9 | / | / | 0∼3 |
ESR, mm/h | 17.5 ± 12.4 | / | / | 0∼15 |
iPTH, pg/mL | 36.0 ± 15.5 | / | / | 12∼68 |
β-CTX, ng/mL | 0.77 (0.54, 2.98) | / | / | 0.26∼0.512 |
MMP1, pg/mL | 1228.3 ± 741.9 | / | / | 392.3∼705.9 |
MMP13, pg/mL | 737.5 (374.6, 3251.8) | / | / | 444.21∼632.79 |
IL-6, pg/mL | 4.5 (1.3, 29.0) | / | / | 1.84∼4.04 |
TNF-α, pg/mL | 7.7 ± 2.9 | / | / | <8.2 |
Femoral neck BMD, g/cm2 | 1.0 ± 0.1 | / | / | / |
Z score of femoral neck | 0.7 ± 1.7 | / | / | / |
Total hip BMD, g/cm2 | 1.1 ± 0.2 | / | / | / |
Z score of total hip | 0.9 ± 1.7 | / | / | / |
Lumbar spine BMD, g/cm2 | 1.2 ± 0.2 | / | / | / |
Z score of lumbar spine | 1.1 ± 1.4 | / | / | / |
Data are presented as mean ± SD or median (minimum, maximum). Abnormal results are indicated in bold. P value ≤.05 is indicated significance between the 2 groups.
Abbreviations: β-CTX, beta-C-telopeptides of type I collagen; ESR, erythrocyte sedimentation rate; hsCRP, hypersensitive C reactive protein; IL-6, interleukin-6; iPTH, intact parathyroid hormone; MMP1, metalloproteinase-1; MMP13, metalloproteinase-13; RANKL/OPG, the ratio of free soluble receptor activator of nuclear factor-kappa B Ligand to osteoprotegerin; TNF-α, tumor necrosis factor-α.
Laboratory tests revealed significantly high serum and urinary PGE2 levels in patients with PHO. Most patients with PHO showed elevated serum levels of hsCRP, ESR, β-CTX, RANKL/OPG ratio, IL-6, MMP1, and MMP13 above the reference range (Table 2).
Skeletal Status of Patients With PHO
The Z-score for aBMD values at the total hip and lumbar spine in patients with PHO were nearly normal; the mean Z-score of femoral neck, total hip, and lumbar spine were 0.7 ± 1.7, 0.9 ± 1.7 and 1.1 ± 1.4, respectively (Table 2).
Microarchitectural changes of patients with PHO at the distal and tibia are depicted in Table 3. Compared with the 50th centile of age- and sex-adjusted reference values provided by Yu et al (19), the patients’ median Tot.Ar and Tb.Ar were markedly increased (Tot.Ar radius: 69.7%, tibia: 57%; Tb.Ar radius: 84.8%, tibia: 59.7%). Tot.vBMD and Tb.vBMD were markedly reduced (Tot.vBMD radius: −32.2%, tibia: −26.2%; Tb.vBMD radius: −37.6%, tibia: −50.7%). Ct.vBMD was moderately reduced (radius: −16.8%, tibia: −21.4%). Markedly increased in Ct.Po (radius: 313.4%, tibia: 74.3%) and reduced Tb.BV/TV (radius: −40.2%, tibia: −27.3%) were found at the radius and tibia. Reduced Tb.N (−15.9%) and increased Tb.Sp (30.6%) were found in the radius, otherwise, increased Tb.N (16%) and reduced Tb.Sp (−8.8%) were found in the tibia.
Microarchitectural changes of patients with PHO at the distal radius and tibia
Variables . | Radius . | Tibia . | ||
---|---|---|---|---|
Median (%) . | IQR (%) . | Median (%) . | IQR (%) . | |
Tot.Ar (mm2) | 564.7 (+69.7) | 451.9-628.3 (54.1-58.2) | 1243.9 (+57.0) | 1012.7-1341.1 (41.9-52.1) |
Tb.Ar (mm2) | 468.1 (+84.8) | 372.3-514.9 (70.9-72.7) | 1030.9 (+59.7) | 831.8-1212.3 (48.0-63.9) |
Ct.Ar (mm2) | 85.9 (+9.3) | 74.9-132.4 (5.3-53.0) | 160.6 (+3.7) | 119.1-245.3 (14.7-44.2) |
Tot.vBMD (mg HA/cm3) | 241.6 (−32.2) | 170.9-271.6 (30.7-46.7) | 244.7 (−26.2) | 156.6-287.1 (22.7-46.8) |
Tb.vBMD (mg HA/cm3) | 114.7 (−37.6) | 96.1-163.1 (21.8-39.7) | 91.6 (−50.7) | 136.6-183.0 (13.1-15.1) |
Ct.vBMD (mg HA/cm3) | 754.1 (−16.8) | 636.3-821.9 (12.0-27.3) | 724.3 (−21.4) | 675-761.7 (19.3-24.7) |
Ct.Po (%) | 2.1 (313.4) | 1.4-3.8 (265.5-439.6) | 3.7 (+74.3) | 2.3-4.7 (50.8-59.7) |
Ct.Th (mm) | 1.23 (3.3) | 0.97-1.55 (9.6-18.8) | 1.64 (+2.3) | 1.10-2.07 (15.3-23.0) |
Tb.BV/TV | 0.17 (−40.2) | 0.13-0.24 (23.8-49.1) | 0.20 (−27.3) | 0.14-0.28 (10.4-41.8) |
Tb.N (1/mm) | 1.23 (−15.9) | 1.06-1.39 (14.1-18.7) | 1.51 (+16.0) | 1.20-1.63 (2.9-11.0) |
Tb.Th (mm) | 0.23 (−6.6) | 0.21-0.26 (0.8-13.1) | 0.23 (−14.7) | 0.21-0.27 (6.4-16.4) |
Tb.Sp (mm) | 0.82 (+30.6) | 0.70-0.95 (24.4-34.2) | 0.67 (−8.8) | 0.62-0.84 (1.3-4.8) |
Variables . | Radius . | Tibia . | ||
---|---|---|---|---|
Median (%) . | IQR (%) . | Median (%) . | IQR (%) . | |
Tot.Ar (mm2) | 564.7 (+69.7) | 451.9-628.3 (54.1-58.2) | 1243.9 (+57.0) | 1012.7-1341.1 (41.9-52.1) |
Tb.Ar (mm2) | 468.1 (+84.8) | 372.3-514.9 (70.9-72.7) | 1030.9 (+59.7) | 831.8-1212.3 (48.0-63.9) |
Ct.Ar (mm2) | 85.9 (+9.3) | 74.9-132.4 (5.3-53.0) | 160.6 (+3.7) | 119.1-245.3 (14.7-44.2) |
Tot.vBMD (mg HA/cm3) | 241.6 (−32.2) | 170.9-271.6 (30.7-46.7) | 244.7 (−26.2) | 156.6-287.1 (22.7-46.8) |
Tb.vBMD (mg HA/cm3) | 114.7 (−37.6) | 96.1-163.1 (21.8-39.7) | 91.6 (−50.7) | 136.6-183.0 (13.1-15.1) |
Ct.vBMD (mg HA/cm3) | 754.1 (−16.8) | 636.3-821.9 (12.0-27.3) | 724.3 (−21.4) | 675-761.7 (19.3-24.7) |
Ct.Po (%) | 2.1 (313.4) | 1.4-3.8 (265.5-439.6) | 3.7 (+74.3) | 2.3-4.7 (50.8-59.7) |
Ct.Th (mm) | 1.23 (3.3) | 0.97-1.55 (9.6-18.8) | 1.64 (+2.3) | 1.10-2.07 (15.3-23.0) |
Tb.BV/TV | 0.17 (−40.2) | 0.13-0.24 (23.8-49.1) | 0.20 (−27.3) | 0.14-0.28 (10.4-41.8) |
Tb.N (1/mm) | 1.23 (−15.9) | 1.06-1.39 (14.1-18.7) | 1.51 (+16.0) | 1.20-1.63 (2.9-11.0) |
Tb.Th (mm) | 0.23 (−6.6) | 0.21-0.26 (0.8-13.1) | 0.23 (−14.7) | 0.21-0.27 (6.4-16.4) |
Tb.Sp (mm) | 0.82 (+30.6) | 0.70-0.95 (24.4-34.2) | 0.67 (−8.8) | 0.62-0.84 (1.3-4.8) |
The percentage of the patients’ structural parameters compared to 50th centile of age- and sex-matched reference values from the literature in round brackets (19).
Abbreviations: Ar, area; Ct, cortical; IQR, interquartile range; N, number; Po, porosity; Sp, separation; Tb, trabecular; Tb.BV/TV, trabecular bone volume fraction; Th, thickness; vBMD, bone mineral density.
Microarchitectural changes of patients with PHO at the distal radius and tibia
Variables . | Radius . | Tibia . | ||
---|---|---|---|---|
Median (%) . | IQR (%) . | Median (%) . | IQR (%) . | |
Tot.Ar (mm2) | 564.7 (+69.7) | 451.9-628.3 (54.1-58.2) | 1243.9 (+57.0) | 1012.7-1341.1 (41.9-52.1) |
Tb.Ar (mm2) | 468.1 (+84.8) | 372.3-514.9 (70.9-72.7) | 1030.9 (+59.7) | 831.8-1212.3 (48.0-63.9) |
Ct.Ar (mm2) | 85.9 (+9.3) | 74.9-132.4 (5.3-53.0) | 160.6 (+3.7) | 119.1-245.3 (14.7-44.2) |
Tot.vBMD (mg HA/cm3) | 241.6 (−32.2) | 170.9-271.6 (30.7-46.7) | 244.7 (−26.2) | 156.6-287.1 (22.7-46.8) |
Tb.vBMD (mg HA/cm3) | 114.7 (−37.6) | 96.1-163.1 (21.8-39.7) | 91.6 (−50.7) | 136.6-183.0 (13.1-15.1) |
Ct.vBMD (mg HA/cm3) | 754.1 (−16.8) | 636.3-821.9 (12.0-27.3) | 724.3 (−21.4) | 675-761.7 (19.3-24.7) |
Ct.Po (%) | 2.1 (313.4) | 1.4-3.8 (265.5-439.6) | 3.7 (+74.3) | 2.3-4.7 (50.8-59.7) |
Ct.Th (mm) | 1.23 (3.3) | 0.97-1.55 (9.6-18.8) | 1.64 (+2.3) | 1.10-2.07 (15.3-23.0) |
Tb.BV/TV | 0.17 (−40.2) | 0.13-0.24 (23.8-49.1) | 0.20 (−27.3) | 0.14-0.28 (10.4-41.8) |
Tb.N (1/mm) | 1.23 (−15.9) | 1.06-1.39 (14.1-18.7) | 1.51 (+16.0) | 1.20-1.63 (2.9-11.0) |
Tb.Th (mm) | 0.23 (−6.6) | 0.21-0.26 (0.8-13.1) | 0.23 (−14.7) | 0.21-0.27 (6.4-16.4) |
Tb.Sp (mm) | 0.82 (+30.6) | 0.70-0.95 (24.4-34.2) | 0.67 (−8.8) | 0.62-0.84 (1.3-4.8) |
Variables . | Radius . | Tibia . | ||
---|---|---|---|---|
Median (%) . | IQR (%) . | Median (%) . | IQR (%) . | |
Tot.Ar (mm2) | 564.7 (+69.7) | 451.9-628.3 (54.1-58.2) | 1243.9 (+57.0) | 1012.7-1341.1 (41.9-52.1) |
Tb.Ar (mm2) | 468.1 (+84.8) | 372.3-514.9 (70.9-72.7) | 1030.9 (+59.7) | 831.8-1212.3 (48.0-63.9) |
Ct.Ar (mm2) | 85.9 (+9.3) | 74.9-132.4 (5.3-53.0) | 160.6 (+3.7) | 119.1-245.3 (14.7-44.2) |
Tot.vBMD (mg HA/cm3) | 241.6 (−32.2) | 170.9-271.6 (30.7-46.7) | 244.7 (−26.2) | 156.6-287.1 (22.7-46.8) |
Tb.vBMD (mg HA/cm3) | 114.7 (−37.6) | 96.1-163.1 (21.8-39.7) | 91.6 (−50.7) | 136.6-183.0 (13.1-15.1) |
Ct.vBMD (mg HA/cm3) | 754.1 (−16.8) | 636.3-821.9 (12.0-27.3) | 724.3 (−21.4) | 675-761.7 (19.3-24.7) |
Ct.Po (%) | 2.1 (313.4) | 1.4-3.8 (265.5-439.6) | 3.7 (+74.3) | 2.3-4.7 (50.8-59.7) |
Ct.Th (mm) | 1.23 (3.3) | 0.97-1.55 (9.6-18.8) | 1.64 (+2.3) | 1.10-2.07 (15.3-23.0) |
Tb.BV/TV | 0.17 (−40.2) | 0.13-0.24 (23.8-49.1) | 0.20 (−27.3) | 0.14-0.28 (10.4-41.8) |
Tb.N (1/mm) | 1.23 (−15.9) | 1.06-1.39 (14.1-18.7) | 1.51 (+16.0) | 1.20-1.63 (2.9-11.0) |
Tb.Th (mm) | 0.23 (−6.6) | 0.21-0.26 (0.8-13.1) | 0.23 (−14.7) | 0.21-0.27 (6.4-16.4) |
Tb.Sp (mm) | 0.82 (+30.6) | 0.70-0.95 (24.4-34.2) | 0.67 (−8.8) | 0.62-0.84 (1.3-4.8) |
The percentage of the patients’ structural parameters compared to 50th centile of age- and sex-matched reference values from the literature in round brackets (19).
Abbreviations: Ar, area; Ct, cortical; IQR, interquartile range; N, number; Po, porosity; Sp, separation; Tb, trabecular; Tb.BV/TV, trabecular bone volume fraction; Th, thickness; vBMD, bone mineral density.
Targeted Metabolomics of Oxylipins in PHO
Multivariate analysis for metabolic profiling
Using the LC-MS/MS approach, the quantification of 105 oxylipins within patients with PHO and HCs was conducted. Analysis Detail information of analyses was provided elsewhere (Tables S1-S4) (20), including MRM ion pairs, calibration, relative standard deviation, and recovery. After relative standard deviation denoising, 59 metabolites were identified in serum samples. The final datasets, including sample names and concentrations for identified metabolites, were imported into MetaboAnalyst 5.0 for a meticulous multivariate analysis. The OPLS-DA, a reliable and robust model, was carried out to characterize the differential profiling between PHO and HCs and the 2 subtypes of patients with PHO (PHOAR1 and PHOAR2). As shown in Fig. 1B-1D, the OPLS-DA between patients with PHO and HCs showed cumulative values of R2Y = 0.847, and Q2 = 0.618, P < .05, suggesting that OPLS-DA models efficiently separated the patients with PHO and HCs. A similar plot pattern was observed between PHOAR1 and patients with PHOAR2, with a cumulative value of R2Y = 0.989, and Q2 = 0.063 (Fig. S1A-S1C (21)).
Identification of Key Metabolic Signatures in PHO
The metabolites that significantly contributed to the difference between patients with PHO and HCs were identified by the S-plot of the OPLS-DA analysis. The features, with VIP > 1, were screened and conducted further hierarchical clustering (Table S5 (20)). Twenty-six targeted metabolites were filtered and comparatively analyzed between with patients with PHO, including subtypes PHOAR1 and PHOAR2, and HCs. As shown in Fig. 2A-2C, in patients with PHO and HCs, patients with PHOAR1 and HCs, and patients with PHOAR2 and HCs, most samples of each group divided into 2 differentiated clusters, though sample clusters of each group overlapped slightly. The metabolites cluster in PHOAR1 overlapped with the cluster in patients with PHOAR2 (Fig. 2D), suggesting that changes between PHOAR1 and PHOAR2 were inconspicuous. To further identify candidate biomarkers capable of classifying PHO and HCs, the study set a stringent threshold, based on statistical significance and biological relevance, to screen and identify differentially expressed metabolites. Nineteen metabolites, with FDR < 0.05 in the univariate analysis, were detected between patients with PHO and HCs. Compared with HCs, patients with PHO were characterized by higher levels of 5-oxoeicosatetraenoic acid (5-OxoETE), 15-oxoeicosatetraenoic acid (15-OxoETE), (8R,15S)-dihydroxyeicosatetraenoic acid (8S,15S-DiHETE), PGE2, 11β-PGE2, PGB2, 9S-hydroxyoctadecadienoic acid (9S-HODE), 13S-hydroxyoctadecadienoic acid (13S-HODE), 5S-hydroxyeicosatrienoic acid (5S-HETrE), 8S-hydroxyeicosatrienoic acid (8S-HETrE), 15S-hydroxyeicosatrienoic acid (15S-HETrE), ±13-hydroxydocosahexaenoic acid (±13-HDoHE), ±8-hydroxydocosahexaenoic acid (±8-HDoHE), ±19,20-epoxy docosapentaenoic acid (±19,20-EpDPE), 5S-hydroxyeicosapentaenoic acid (5S-HEPE), 15S-hydroxyeicosapentaenoic acid (15S-HEPE), and 13-(S)-hydroxyoctadecatrienoic acid (13(S)-HOTrE(γ)), as well as lower levels of LTB4 and LTE4 (Table 4 and Fig. 3). Likewise, to further investigate the impact of pathogenic genes on oxylipin metabolism, we analyzed the statistical significance of these 19 differentially expressed metabolites across various groups, encompassing the different subtypes of PHO. The results, of significant importance, revealed that all 18 metabolites, except for 15S-HEPE, had significant differences between PHOAR2 and HCs. Similarly, in patients with PHOAR1, 14 metabolites showed significant differences between PHOAR1 and HCs. However, 15-OxoETE, LTB4, 15S-HETrE, 13(S)-HOTrE(γ), ±13-HDoHE showed no significant differences between PHOAR1 and HCs (Table 5).
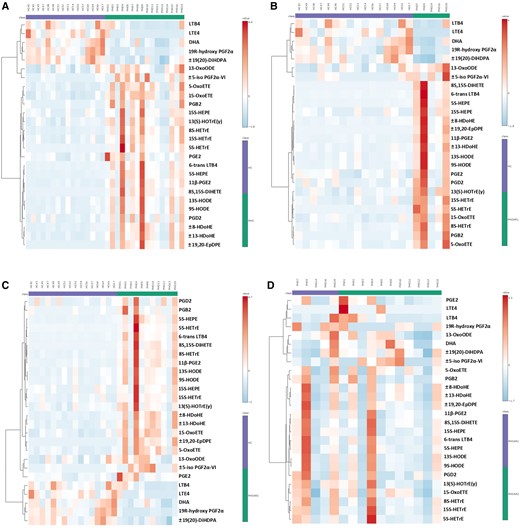
(A-D) Clustering heatmaps showing the metabolic differences of each group of oxylipins (VIP > 1) among patients with PHO, patients with PHOAR1, patients with PHOAR2, and HCs. (A) Metabolic differences of oxylipins between patients with PHO and HCs. (B) Metabolic differences of oxylipins between patients with PHOAR1 and HCs. (C) Metabolic differences of oxylipins between patients with PHOAR2 and HCs. (D) Metabolic differences of oxylipins between patients with PHOAR1 and patients with PHOAR2.
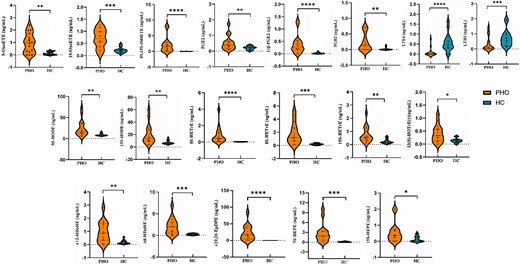
Violin diagram showing the concentration of 19 metabolite profiles in the quantitative analysis of patients with PHO and HCs. The significance between the HC and PHO groups was determined using the Mann–Whitney U test. *P < .05, **P < .01, ***P < .001, ****P < .0001.
Metabolite name . | Category . | P value . | FC (PHO/HC) . | FDR (PHO/HC) . | Type . | AUC . | 95% CI . |
---|---|---|---|---|---|---|---|
5-OxoETE | AA | .002 | 7.518 | 0.009 | Upregulated | 0.824 | 0.662-0.987 |
15-OxoETE | AA | .0003 | 3.155 | 0.002 | Upregulated | 0.871 | 0.730-1.000 |
8S,15S-DiHETE | AA | .0001 | 422.139 | 0.006 | Upregulated | 0.867 | 0.729-1.000 |
PGE2 | AA | .010 | 2.668 | 0.020 | Upregulated | 0.766 | 0.635-0.965 |
11β-PGE2 | AA | .0001 | 16.757 | 0.006 | Upregulated | 0.898 | 0.767-1.000 |
PGB2 | AA | .01 | 11.723 | 0.029 | Upregulated | 0.732 | 0.552-0.913 |
LTB4 | AA | .007 | 0.450 | 0.020 | Downregulated | 0.783 | 0.606-0.961 |
LTE4 | AA | .0001 | 0.127 | 0.006 | Downregulated | 0.871 | 0.737-1.000 |
9S-HODE | LA | .002 | 2.681 | 0.009 | Upregulated | 0.820 | 0.656-0.984 |
13S-HODE | LA | .002 | 2.778 | 0.009 | Upregulated | 0.816 | 0.654-0.979 |
5S-HETrE | DGLA | .0001 | 30.580 | 0.006 | Upregulated | 0.910 | 0.799-1.000 |
8S-HETrE | DGLA | .0003 | 9.459 | 0.002 | Upregulated | 0.875 | 0.738-1.000 |
15S-HETrE | DGLA | .002 | 3.157 | 0.009 | Upregulated | 0.824 | 0.677-0.972 |
13(S)-HOTrE(γ) | ALA | .014 | 2.998 | 0.034 | Upregulated | 0.754 | 0.571-0.937 |
±13-HDoHE | DHA | .003 | 5.459 | 0.010 | Upregulated | 0.805 | 0.624-0.985 |
±8-HDoHE | DHA | .001 | 8.631 | 0.006 | Upregulated | 0.836 | 0.671-1.000 |
±19,20-EpDPE | DHA | .0001 | 843.542 | 0.006 | Upregulated | 0.889 | 0.759-1.000 |
5S-HEPE | EPA | .001 | 24.159 | 0.006 | Upregulated | 0.848 | 0.687-1.000 |
15S-HEPE | EPA | .013 | 4.098 | 0.033 | Upregulated | 0.750 | 0.576-0.924 |
Metabolite name . | Category . | P value . | FC (PHO/HC) . | FDR (PHO/HC) . | Type . | AUC . | 95% CI . |
---|---|---|---|---|---|---|---|
5-OxoETE | AA | .002 | 7.518 | 0.009 | Upregulated | 0.824 | 0.662-0.987 |
15-OxoETE | AA | .0003 | 3.155 | 0.002 | Upregulated | 0.871 | 0.730-1.000 |
8S,15S-DiHETE | AA | .0001 | 422.139 | 0.006 | Upregulated | 0.867 | 0.729-1.000 |
PGE2 | AA | .010 | 2.668 | 0.020 | Upregulated | 0.766 | 0.635-0.965 |
11β-PGE2 | AA | .0001 | 16.757 | 0.006 | Upregulated | 0.898 | 0.767-1.000 |
PGB2 | AA | .01 | 11.723 | 0.029 | Upregulated | 0.732 | 0.552-0.913 |
LTB4 | AA | .007 | 0.450 | 0.020 | Downregulated | 0.783 | 0.606-0.961 |
LTE4 | AA | .0001 | 0.127 | 0.006 | Downregulated | 0.871 | 0.737-1.000 |
9S-HODE | LA | .002 | 2.681 | 0.009 | Upregulated | 0.820 | 0.656-0.984 |
13S-HODE | LA | .002 | 2.778 | 0.009 | Upregulated | 0.816 | 0.654-0.979 |
5S-HETrE | DGLA | .0001 | 30.580 | 0.006 | Upregulated | 0.910 | 0.799-1.000 |
8S-HETrE | DGLA | .0003 | 9.459 | 0.002 | Upregulated | 0.875 | 0.738-1.000 |
15S-HETrE | DGLA | .002 | 3.157 | 0.009 | Upregulated | 0.824 | 0.677-0.972 |
13(S)-HOTrE(γ) | ALA | .014 | 2.998 | 0.034 | Upregulated | 0.754 | 0.571-0.937 |
±13-HDoHE | DHA | .003 | 5.459 | 0.010 | Upregulated | 0.805 | 0.624-0.985 |
±8-HDoHE | DHA | .001 | 8.631 | 0.006 | Upregulated | 0.836 | 0.671-1.000 |
±19,20-EpDPE | DHA | .0001 | 843.542 | 0.006 | Upregulated | 0.889 | 0.759-1.000 |
5S-HEPE | EPA | .001 | 24.159 | 0.006 | Upregulated | 0.848 | 0.687-1.000 |
15S-HEPE | EPA | .013 | 4.098 | 0.033 | Upregulated | 0.750 | 0.576-0.924 |
Abbreviations: 8S,15S-DiHETE, (8R,15S)-dihydroxyeicosatetraenoic acid; AA, arachidonic acid; AUC, area under curve; DHA, docosahexenoic acid; EPA, timnodonic acid; EpDPE, epoxy docosapentaenoic acid; FC, fold change; FDR, false discovery rate; HC, healthy control; HDoHE, hydroxydocosahexaenoic acid; HEPE, hydroxyeicosapentaenoic acid; HETE, hydroxyeicosatetraenoic acid; HETrE, hydroxyeicosatrienoic acid; HHTrE, hydroxyheptadecatrienoic acid; HODE, hydroxyoctadecadienoic acid; LA, linoleic acid; LTB4, leukotriene B4; OxoETE, oxoeicosatetraenoic acid; PHO, primary hypertrophic osteoarthropathy.
Metabolite name . | Category . | P value . | FC (PHO/HC) . | FDR (PHO/HC) . | Type . | AUC . | 95% CI . |
---|---|---|---|---|---|---|---|
5-OxoETE | AA | .002 | 7.518 | 0.009 | Upregulated | 0.824 | 0.662-0.987 |
15-OxoETE | AA | .0003 | 3.155 | 0.002 | Upregulated | 0.871 | 0.730-1.000 |
8S,15S-DiHETE | AA | .0001 | 422.139 | 0.006 | Upregulated | 0.867 | 0.729-1.000 |
PGE2 | AA | .010 | 2.668 | 0.020 | Upregulated | 0.766 | 0.635-0.965 |
11β-PGE2 | AA | .0001 | 16.757 | 0.006 | Upregulated | 0.898 | 0.767-1.000 |
PGB2 | AA | .01 | 11.723 | 0.029 | Upregulated | 0.732 | 0.552-0.913 |
LTB4 | AA | .007 | 0.450 | 0.020 | Downregulated | 0.783 | 0.606-0.961 |
LTE4 | AA | .0001 | 0.127 | 0.006 | Downregulated | 0.871 | 0.737-1.000 |
9S-HODE | LA | .002 | 2.681 | 0.009 | Upregulated | 0.820 | 0.656-0.984 |
13S-HODE | LA | .002 | 2.778 | 0.009 | Upregulated | 0.816 | 0.654-0.979 |
5S-HETrE | DGLA | .0001 | 30.580 | 0.006 | Upregulated | 0.910 | 0.799-1.000 |
8S-HETrE | DGLA | .0003 | 9.459 | 0.002 | Upregulated | 0.875 | 0.738-1.000 |
15S-HETrE | DGLA | .002 | 3.157 | 0.009 | Upregulated | 0.824 | 0.677-0.972 |
13(S)-HOTrE(γ) | ALA | .014 | 2.998 | 0.034 | Upregulated | 0.754 | 0.571-0.937 |
±13-HDoHE | DHA | .003 | 5.459 | 0.010 | Upregulated | 0.805 | 0.624-0.985 |
±8-HDoHE | DHA | .001 | 8.631 | 0.006 | Upregulated | 0.836 | 0.671-1.000 |
±19,20-EpDPE | DHA | .0001 | 843.542 | 0.006 | Upregulated | 0.889 | 0.759-1.000 |
5S-HEPE | EPA | .001 | 24.159 | 0.006 | Upregulated | 0.848 | 0.687-1.000 |
15S-HEPE | EPA | .013 | 4.098 | 0.033 | Upregulated | 0.750 | 0.576-0.924 |
Metabolite name . | Category . | P value . | FC (PHO/HC) . | FDR (PHO/HC) . | Type . | AUC . | 95% CI . |
---|---|---|---|---|---|---|---|
5-OxoETE | AA | .002 | 7.518 | 0.009 | Upregulated | 0.824 | 0.662-0.987 |
15-OxoETE | AA | .0003 | 3.155 | 0.002 | Upregulated | 0.871 | 0.730-1.000 |
8S,15S-DiHETE | AA | .0001 | 422.139 | 0.006 | Upregulated | 0.867 | 0.729-1.000 |
PGE2 | AA | .010 | 2.668 | 0.020 | Upregulated | 0.766 | 0.635-0.965 |
11β-PGE2 | AA | .0001 | 16.757 | 0.006 | Upregulated | 0.898 | 0.767-1.000 |
PGB2 | AA | .01 | 11.723 | 0.029 | Upregulated | 0.732 | 0.552-0.913 |
LTB4 | AA | .007 | 0.450 | 0.020 | Downregulated | 0.783 | 0.606-0.961 |
LTE4 | AA | .0001 | 0.127 | 0.006 | Downregulated | 0.871 | 0.737-1.000 |
9S-HODE | LA | .002 | 2.681 | 0.009 | Upregulated | 0.820 | 0.656-0.984 |
13S-HODE | LA | .002 | 2.778 | 0.009 | Upregulated | 0.816 | 0.654-0.979 |
5S-HETrE | DGLA | .0001 | 30.580 | 0.006 | Upregulated | 0.910 | 0.799-1.000 |
8S-HETrE | DGLA | .0003 | 9.459 | 0.002 | Upregulated | 0.875 | 0.738-1.000 |
15S-HETrE | DGLA | .002 | 3.157 | 0.009 | Upregulated | 0.824 | 0.677-0.972 |
13(S)-HOTrE(γ) | ALA | .014 | 2.998 | 0.034 | Upregulated | 0.754 | 0.571-0.937 |
±13-HDoHE | DHA | .003 | 5.459 | 0.010 | Upregulated | 0.805 | 0.624-0.985 |
±8-HDoHE | DHA | .001 | 8.631 | 0.006 | Upregulated | 0.836 | 0.671-1.000 |
±19,20-EpDPE | DHA | .0001 | 843.542 | 0.006 | Upregulated | 0.889 | 0.759-1.000 |
5S-HEPE | EPA | .001 | 24.159 | 0.006 | Upregulated | 0.848 | 0.687-1.000 |
15S-HEPE | EPA | .013 | 4.098 | 0.033 | Upregulated | 0.750 | 0.576-0.924 |
Abbreviations: 8S,15S-DiHETE, (8R,15S)-dihydroxyeicosatetraenoic acid; AA, arachidonic acid; AUC, area under curve; DHA, docosahexenoic acid; EPA, timnodonic acid; EpDPE, epoxy docosapentaenoic acid; FC, fold change; FDR, false discovery rate; HC, healthy control; HDoHE, hydroxydocosahexaenoic acid; HEPE, hydroxyeicosapentaenoic acid; HETE, hydroxyeicosatetraenoic acid; HETrE, hydroxyeicosatrienoic acid; HHTrE, hydroxyheptadecatrienoic acid; HODE, hydroxyoctadecadienoic acid; LA, linoleic acid; LTB4, leukotriene B4; OxoETE, oxoeicosatetraenoic acid; PHO, primary hypertrophic osteoarthropathy.
Analysis of differential metabolites among PHOAR1 (n = 5), PHOAR2 (n = 11), and HCs (n = 16)
Metabolite name . | FC (PHOAR1/HCs) . | FDR (PHOAR1/HCs) . | FC (PHOAR2/HCs) . | FDR (PHOAR2/HCs) . | FC (PHOAR1/PHOAR2) . | FDR (PHOAR1/PHOAR2) . |
---|---|---|---|---|---|---|
5-OxoETE | 8.770 | 0.044* | 6.949 | 0.009** | 0.792 | 0.776 |
15-OxoETE | 3.518 | 0.062 | 2.991 | 0.003** | 0.850 | 0.462 |
8S,15S-DiHETE | 552.502 | 0.026* | 362.883 | 0.003** | 0.657 | 0.493 |
PGE2 | 2.615 | 0.026* | 2.691 | 0.018* | 1.029 | 0.777 |
11β-PGE2 | 23.139 | 0.026* | 13.856 | 0.006** | 0.599 | 0.335 |
PGB2 | 17.731 | 0.043* | 8.992 | 0.030* | 0.507 | 0.375 |
LTB4 | 0.562 | 0.112 | 0.409 | 0.018* | 0.729 | 0.794 |
LTE4 | 0.001 | 0.026* | 0.184 | 0.006** | 216.756 | 0.325 |
9S-HODE | 3.439 | 0.026* | 2.336 | 0.025* | 0.679 | 0.396 |
13S-HODE | 3.560 | 0.026* | 2.423 | 0.025* | 0.681 | 0.462 |
5S-HETrE | 37.949 | 0.046* | 27.230 | 0.003** | 0.718 | 0.396 |
8S-HETrE | 12.569 | 0.046* | 8.045 | 0.004** | 0.640 | 0.533 |
15S-HETrE | 3.860 | 0.062 | 2.838 | 0.009** | 0.735 | 0.533 |
13(S)-HOTrE(γ) | 4.075 | 0.059 | 2.509 | 0.050* | 0.616 | 0.174 |
±13-HDoHE | 6.582 | 0.078 | 4.949 | 0.011* | 0.752 | 0.865 |
±8-HDoHE | 10.839 | 0.038* | 7.627 | 0.011* | 0.704 | 0.533 |
±19,20-EpDPE | 1165.340 | 0.026* | 697.270 | 0.003** | 0.598 | 0.532 |
5S-HEPE | 34.598 | 0.043* | 19.414 | 0.006** | 0.561 | 0.233 |
15S-HEPE | 6.099 | 0.038* | 3.189 | 0.073 | 0.523 | 0.171 |
Metabolite name . | FC (PHOAR1/HCs) . | FDR (PHOAR1/HCs) . | FC (PHOAR2/HCs) . | FDR (PHOAR2/HCs) . | FC (PHOAR1/PHOAR2) . | FDR (PHOAR1/PHOAR2) . |
---|---|---|---|---|---|---|
5-OxoETE | 8.770 | 0.044* | 6.949 | 0.009** | 0.792 | 0.776 |
15-OxoETE | 3.518 | 0.062 | 2.991 | 0.003** | 0.850 | 0.462 |
8S,15S-DiHETE | 552.502 | 0.026* | 362.883 | 0.003** | 0.657 | 0.493 |
PGE2 | 2.615 | 0.026* | 2.691 | 0.018* | 1.029 | 0.777 |
11β-PGE2 | 23.139 | 0.026* | 13.856 | 0.006** | 0.599 | 0.335 |
PGB2 | 17.731 | 0.043* | 8.992 | 0.030* | 0.507 | 0.375 |
LTB4 | 0.562 | 0.112 | 0.409 | 0.018* | 0.729 | 0.794 |
LTE4 | 0.001 | 0.026* | 0.184 | 0.006** | 216.756 | 0.325 |
9S-HODE | 3.439 | 0.026* | 2.336 | 0.025* | 0.679 | 0.396 |
13S-HODE | 3.560 | 0.026* | 2.423 | 0.025* | 0.681 | 0.462 |
5S-HETrE | 37.949 | 0.046* | 27.230 | 0.003** | 0.718 | 0.396 |
8S-HETrE | 12.569 | 0.046* | 8.045 | 0.004** | 0.640 | 0.533 |
15S-HETrE | 3.860 | 0.062 | 2.838 | 0.009** | 0.735 | 0.533 |
13(S)-HOTrE(γ) | 4.075 | 0.059 | 2.509 | 0.050* | 0.616 | 0.174 |
±13-HDoHE | 6.582 | 0.078 | 4.949 | 0.011* | 0.752 | 0.865 |
±8-HDoHE | 10.839 | 0.038* | 7.627 | 0.011* | 0.704 | 0.533 |
±19,20-EpDPE | 1165.340 | 0.026* | 697.270 | 0.003** | 0.598 | 0.532 |
5S-HEPE | 34.598 | 0.043* | 19.414 | 0.006** | 0.561 | 0.233 |
15S-HEPE | 6.099 | 0.038* | 3.189 | 0.073 | 0.523 | 0.171 |
Abnormal results are indicated in bold.
Abbreviations: 8S,15S-DiHETE, (8R,15S)-dihydroxyeicosatetraenoic acid; AA, arachidonic acid; DHA, docosahexenoic acid; EPA, timnodonic acid; EpDPE, epoxy docosapentaenoic acid; FC, fold change; FDR, false discovery rate; HC, healthy control; HDoHE, hydroxydocosahexaenoic acid; HEPE, hydroxyeicosapentaenoic acid; HETE, hydroxyeicosatetraenoic acid; HETrE, hydroxyeicosatrienoic acid; HHTrE, hydroxyheptadecatrienoic acid; HODE, hydroxyoctadecadienoic acid; LA, linoleic acid; LTB4, leukotriene B4; OxoETE, oxoeicosatetraenoic acid; PHO, primary hypertrophic osteoarthropathy.
*P < .05; **P < .01.
Analysis of differential metabolites among PHOAR1 (n = 5), PHOAR2 (n = 11), and HCs (n = 16)
Metabolite name . | FC (PHOAR1/HCs) . | FDR (PHOAR1/HCs) . | FC (PHOAR2/HCs) . | FDR (PHOAR2/HCs) . | FC (PHOAR1/PHOAR2) . | FDR (PHOAR1/PHOAR2) . |
---|---|---|---|---|---|---|
5-OxoETE | 8.770 | 0.044* | 6.949 | 0.009** | 0.792 | 0.776 |
15-OxoETE | 3.518 | 0.062 | 2.991 | 0.003** | 0.850 | 0.462 |
8S,15S-DiHETE | 552.502 | 0.026* | 362.883 | 0.003** | 0.657 | 0.493 |
PGE2 | 2.615 | 0.026* | 2.691 | 0.018* | 1.029 | 0.777 |
11β-PGE2 | 23.139 | 0.026* | 13.856 | 0.006** | 0.599 | 0.335 |
PGB2 | 17.731 | 0.043* | 8.992 | 0.030* | 0.507 | 0.375 |
LTB4 | 0.562 | 0.112 | 0.409 | 0.018* | 0.729 | 0.794 |
LTE4 | 0.001 | 0.026* | 0.184 | 0.006** | 216.756 | 0.325 |
9S-HODE | 3.439 | 0.026* | 2.336 | 0.025* | 0.679 | 0.396 |
13S-HODE | 3.560 | 0.026* | 2.423 | 0.025* | 0.681 | 0.462 |
5S-HETrE | 37.949 | 0.046* | 27.230 | 0.003** | 0.718 | 0.396 |
8S-HETrE | 12.569 | 0.046* | 8.045 | 0.004** | 0.640 | 0.533 |
15S-HETrE | 3.860 | 0.062 | 2.838 | 0.009** | 0.735 | 0.533 |
13(S)-HOTrE(γ) | 4.075 | 0.059 | 2.509 | 0.050* | 0.616 | 0.174 |
±13-HDoHE | 6.582 | 0.078 | 4.949 | 0.011* | 0.752 | 0.865 |
±8-HDoHE | 10.839 | 0.038* | 7.627 | 0.011* | 0.704 | 0.533 |
±19,20-EpDPE | 1165.340 | 0.026* | 697.270 | 0.003** | 0.598 | 0.532 |
5S-HEPE | 34.598 | 0.043* | 19.414 | 0.006** | 0.561 | 0.233 |
15S-HEPE | 6.099 | 0.038* | 3.189 | 0.073 | 0.523 | 0.171 |
Metabolite name . | FC (PHOAR1/HCs) . | FDR (PHOAR1/HCs) . | FC (PHOAR2/HCs) . | FDR (PHOAR2/HCs) . | FC (PHOAR1/PHOAR2) . | FDR (PHOAR1/PHOAR2) . |
---|---|---|---|---|---|---|
5-OxoETE | 8.770 | 0.044* | 6.949 | 0.009** | 0.792 | 0.776 |
15-OxoETE | 3.518 | 0.062 | 2.991 | 0.003** | 0.850 | 0.462 |
8S,15S-DiHETE | 552.502 | 0.026* | 362.883 | 0.003** | 0.657 | 0.493 |
PGE2 | 2.615 | 0.026* | 2.691 | 0.018* | 1.029 | 0.777 |
11β-PGE2 | 23.139 | 0.026* | 13.856 | 0.006** | 0.599 | 0.335 |
PGB2 | 17.731 | 0.043* | 8.992 | 0.030* | 0.507 | 0.375 |
LTB4 | 0.562 | 0.112 | 0.409 | 0.018* | 0.729 | 0.794 |
LTE4 | 0.001 | 0.026* | 0.184 | 0.006** | 216.756 | 0.325 |
9S-HODE | 3.439 | 0.026* | 2.336 | 0.025* | 0.679 | 0.396 |
13S-HODE | 3.560 | 0.026* | 2.423 | 0.025* | 0.681 | 0.462 |
5S-HETrE | 37.949 | 0.046* | 27.230 | 0.003** | 0.718 | 0.396 |
8S-HETrE | 12.569 | 0.046* | 8.045 | 0.004** | 0.640 | 0.533 |
15S-HETrE | 3.860 | 0.062 | 2.838 | 0.009** | 0.735 | 0.533 |
13(S)-HOTrE(γ) | 4.075 | 0.059 | 2.509 | 0.050* | 0.616 | 0.174 |
±13-HDoHE | 6.582 | 0.078 | 4.949 | 0.011* | 0.752 | 0.865 |
±8-HDoHE | 10.839 | 0.038* | 7.627 | 0.011* | 0.704 | 0.533 |
±19,20-EpDPE | 1165.340 | 0.026* | 697.270 | 0.003** | 0.598 | 0.532 |
5S-HEPE | 34.598 | 0.043* | 19.414 | 0.006** | 0.561 | 0.233 |
15S-HEPE | 6.099 | 0.038* | 3.189 | 0.073 | 0.523 | 0.171 |
Abnormal results are indicated in bold.
Abbreviations: 8S,15S-DiHETE, (8R,15S)-dihydroxyeicosatetraenoic acid; AA, arachidonic acid; DHA, docosahexenoic acid; EPA, timnodonic acid; EpDPE, epoxy docosapentaenoic acid; FC, fold change; FDR, false discovery rate; HC, healthy control; HDoHE, hydroxydocosahexaenoic acid; HEPE, hydroxyeicosapentaenoic acid; HETE, hydroxyeicosatetraenoic acid; HETrE, hydroxyeicosatrienoic acid; HHTrE, hydroxyheptadecatrienoic acid; HODE, hydroxyoctadecadienoic acid; LA, linoleic acid; LTB4, leukotriene B4; OxoETE, oxoeicosatetraenoic acid; PHO, primary hypertrophic osteoarthropathy.
*P < .05; **P < .01.
Further ROC analysis was conducted to evaluate the performance of these potential indicators. There were 18 metabolites with AUC > 0.75, identified as potential discriminators to reflect the PHO disease (Table 4).
Correlation Analysis of Between Metabolomics and Related Parameters in Patients With PHO, PHOAR1, and PHOAR2
In order to address the significance of changed metabolic biomarker, Pearson correlation analysis between different major metabolites and HR-pQCT parameters at the distal radius, HR-pQCT parameters at the distal tibia, BMD, major clinical features, and biochemical markers in patients with PHO were conducted. At the distal radius, the concentration of 5S-HETrE was found to be positively correlated with Ct.Po, and negatively correlated with Tb.Ar. The concentration of LTE4 was significantly positively correlated with Tot.Ar and Tb.Ar at the distal radius, as well as positively correlated with Ct.Ar at the distal tibia. At the distal tibia, PGE2 was found to be positively correlated with Tot.Ar and Tb.Ar, and LTB4 was found to be positively correlated with Ct.vBMD. Correlation analysis among clinical markers and oxylipin metabolites found that the level of MMP13 positively correlated with 15S-HEPE, 5S-HEPE, ±19,20-EpDPE, ±13-HDoHE, ±8-HDoHE, 9S-HODE, 13S-HODE, 11β-PGE2 and 8S,15S-DiHETE (Fig. 4A-4C). No significant associations were found among BMD and oxylipin metabolites.
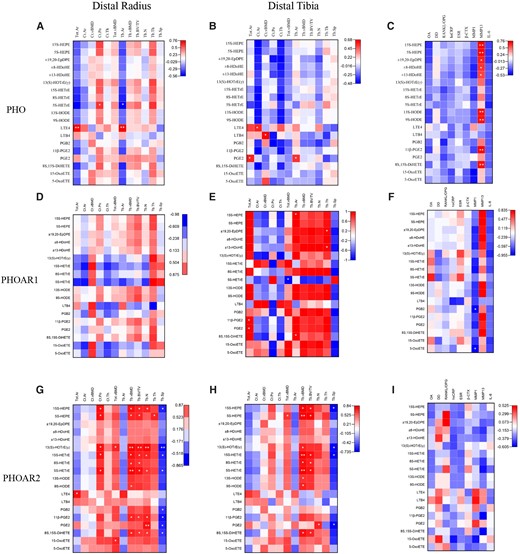
(A-C) Pearson r correlation matrix representing the relationship among the 19 metabolites with HR-pQCT parameters of the distal radius, HR-pQCT parameters of the distal tabia, and selected biochemical markers in patients with PHO, respectively. (D-F) Pearson r correlation matrix representing the relationship among the 19 metabolites with HR-pQCT parameters of the distal radius, HR-pQCT parameters of the distal tabia, and selected biochemical markers in patients with PHOAR1, respectively. (G-I) Pearson r correlation matrix representing the relationship among the 19 metabolites with HR-pQCT parameters of the distal radius, HR-pQCT parameters of the distal tabia, and selected biochemical markers in patients with PHOAR2, respectively. *P < .05, **P < .01.
To further detect whether a relationship existed between pathogenic genes and differential metabolites, correlation analysis between the different oxylipin metabolites and HR-pQCT parameters at the distal radius, HR-pQCT parameters at the distal tibia, BMD, and significant clinical features, and biochemical markers in patients with PHOAR1 and PHOAR2 were conducted, respectively. Results found that, at the distal radius, most of the metabolites were significantly associated with HR-pQCT parameters in patients with PHOAR2. Specifically, the concentration of 15S-HEPE, 13(S)-HOTrE(γ), 15S-HETrE, 5S-HETrE, 11β-PGE2, and 8S,15S-DiHETE were positively correlated with Tb.vBMD, Tb.N, and Tb.BV/TV, and negatively correlated with Tb.Sp. However, in patients with PHOAR1, no associations were found between metabolites and HR-pQCT parameters of the distal radius. Similarly, correlations between different metabolites and HR-pQCT parameters of the distal tibia in patients with PHOAR1 and PHOAR2 differed. In patients with PHOAR1, PGE2 and 11β-PGE2 were positively correlated with Tot.Ar, but the associations disappeared in patients with PHOAR2. The concentration of 15S-HEPE, 5S-HEPE, 15S-HETrE, 8S-HETrE, 5S-HETrE, and 8S,15S-DiHETE were found to be positively correlated with Tb.vBMD and Tb.BV/TV. Moreover, 15S-HEPE and 15S-HETrE were significantly negatively correlated with Tb.Sp. PGE2 in patients with PHOAR2 at distal radius or distal tibia, was positively correlated with Tb.N and negatively with Tb.Sp (Fig. 4D-4I).
Accordingly, significant associations between metabolites and BMD were only observed in patients with PHOAR2. The BMD values of the femoral neck and total hip were positively correlated with 15S-HEPE, 5S-HEPE, 11β-PGE2, 9S-HODE, 13S-HODE, 15S-HETrE, 8S-HETrE, 13(S)-HOTrE(γ), 5S-HETrE, and 8S,15S-DiHETE. All correlation data are detailed elsewhere (Tables S6-S15 (20)).
Discussion
Oxylipins are vital lipid intermediaries synthesized from polyunsaturated fatty acids (PUFAs). Omega-6 polyunsaturated fatty acid (n-6 PUFAs): AA, linolenic acid (LA), and omega 3 PUFAs (n-3 PUFAs): EPA and DHA, were the main precursors of oxylipins (22). Previous studies have suggested that oxylipins participated in nearly all physiological functions of the body and were closely related to the occurrence and development of a variety of diseases, including tumors (23), cardiovascular disease (24), diabetes (25), osteoarthritis (26, 27), etc. Lipidomics efficiently understood the pathophysiology changes linked to disease progression and new targets. Elevated levels of PGE2 were the pathogenesis of PHO. PGE2 was synthesized through the action of the rate-limiting enzyme COX2 from AA (28). Although PGE2 and COX2 have been reported to be elevated in PHO, little was known about the relationship between oxylipins and the occurrence and development of PHO. Therefore, we conducted a UHPLC-MS analysis of serum oxylipin metabolites in patients with PHO. Our targeted quantitative analysis, which primarily focused on the AA and LA metabolism pathways (as confirmed by the KEGG pathway enrichment in Table S16 (20)), had led to some intriguing findings. We have categorized and depicted the pathway distribution in this study according to the KEGG database (Fig. 5). Our results have unveiled a novel discovery, 19 oxylipin metabolites, including 15-OxoETE, 5-OxoETE, 8S,15S-DiHETE, PGE2, 11β-PGE2, PGB2, LTB4, LTE4, 9S-HODE, 13S-HODE, 13(S)-HOTrE(γ), 5S-HETrE, 15S-HETrE, 8S-HETrE, ±19,20-EpDPE, ±13-HDoHE, ±8-HDoHE, 5S-HEPE, and 15-HEPE, were associated with PHO (Fig. 5). These results, for the first time, revealed alterations of oxylipin metabolites during the development of PHO, independent of the pathogenic genes of PHO. Our study also evaluated the potential influences of oxylipin metabolites on bone microstructure and clinical features in patients with PHO. The findings might provide a novel insight into understanding the pathogenesis of PHO.

KEGG metabolic pathway for arachidonic acid. The circle and triangle represent differentially expressed metabolites in the oxylipin metabolic pathway. The circle indicate increased metabolites in patients with PHO, while the triangle indicate reduced metabolites in patients with PHO. KEGG, Kyoto Encyclopedia of Genes and Genomes.
AA is an n-6 PUFA essential fatty acid cleaved from phospholipids by PLA2 and phospholipase C, which is then metabolized into thromboxanes and prostaglandins by the COX pathway, lipoxins, LTs, and hydroxyeicosatetraenoic acids by the LOX pathway, and epoxyeicosatrienoic acids by the CYP450 pathway (29). AA was associated with inflammation, angiogenesis, immunoregulation, and platelet aggregation (30). PHO has been reported to be a chronic inflammatory disease associated with typical inflammatory syndromes, including joint and soft-tissue swelling and pain (13). Inflammatory cytokines such as hsCRP, ESR, IL-6, and TNF-α were significantly increased in patients with PHO. We further investigated whether there were proinflammatory oxylipin changes in patients with PHO. As expected, the results showed that the typical proinflammatory oxylipins, including PGE2, 11β-PGE2, PGB2, 5-oxo-ETE, and 15-oxo-ETE, were increased in patients with PHO compared with HCs. Additionally, 9(S)-HODE, 13(S)-HODE, which were synthesized from LA (18:2n-6), 5S-HETrE, and 8S-HETrE, and 15S-HETrE, which was synthesized from γ-LA (18:3n-6), were all increased in patients with PHO. Unexpectedly, the concentration of LTB4 decreased in patients with PHO compared with HCs. It is well known that LTB4 belongs to the LTs, a family of lipid mediators of inflammation and chemotaxis, which are derived from the 5-LOX-catalyzed oxygenation of AA, involved in a large number of inflammatory pathologies, the introduction of pain, and bone destruction (31-34). Patients with PHO with typical clinical features of arthralgia, bone erosion, and joint swelling should have an upregulated level of LTB4. The reduced concentration of LTB4 might result from (1) the proresolution function following the chronic inflammation. The decreased levels of LTB4 might also reflect lower activity of its synthesis enzyme, LTA4H, the final and rate-limiting enzyme regulating the biosynthesis of LTB4. (2) Receptor–ligand interactions. Studies have reported that LTB4 acted on cells via interaction with its receptor BLT1 and/or BLT2 to amplify pathological inflammation, bone resorption, and neuropathic pain (35). The consumption of LTB4 binding with its receptors could explain the decrease in the release of LTB4 into the serum. (3) The level of LTB4 in serum might not reflect the disease condition; pathological tissue should be investigated in further study. Though the study did not find any associations among inflammatory cytokines and the proinflammatory oxylipin metabolites, the above-changed oxylipins indicated potential roles in inflammatory syndromes of patients with PHO.
Evidence has revealed that inflammation of the bone microenvironment induced both stimulation of bone resorption and suppression of bone formation, which contributed to progressive bone loss. The present study also showed increased bone resorption, including high RANKL/OPG ratio levels, and inferior bone microstructure, including low vBMD, Tb.BV/TV, large Tb.Sp, and increased Ct.Po. Studies have postulated that higher intakes of n-6 PUFAs resulted in raised proinflammatory cytokine production that stimulates osteoclastic activity and inhibits osteoblast formation, thereby negatively impacting bone health (36). The present study analyzed the correlations among n-6 PUFAs, bone microstructure parameters, and biochemical bone turnover markers to uncover the effects of the n-6 PUFAs changes on bone morphometry and bone metabolism in patients with PHO. Though there were no existing associations between n-6 PUFAs and biochemical bone turnover markers, the results showed that 5S-HETrE was negatively associated with Tb.Ar, and positively associated with Ct.Po. The low level of LTB4 was also found to be positively correlated with Ct.vBMD. All the above findings suggested that 5S-HETrE and LTB4 might be responsible for the bone microstructure changes of patients with PHO.
Persistent inflammation at the joint could contribute to joint degeneration (37). Consistent with our previous study (13), which revealed joint degeneration in patients with PHO, the present study found increased serum MMP1 and MMP13 levels in patients with PHO. MMP1 and MMP13 were both involved in the process of collagen degradation. Meanwhile, MMP13 was the key enzyme responsible for cartilage destruction and collagen degradation, which was thought to be a major contributor to the degenerative process occurring during osteoarthritis (OA) pathogenesis (38). OA joints have been shown to contain specialized proresolving lipid mediators, which included lipoxins derived from AA, EPA (20:5n-3), and DHA (22:6n-3) and their precursors. Moreover, there was ample evidence that lipoxins derived from α-LA (18:3n-3), EPA, and DHA were able to reduce the expression of COX2, iNOS, inflammatory cytokines, and cartilage-degrading proteinases, leading to reduced inflammation, chondrocyte apoptosis, and extracellular matrix degradation (27). Notably, the present study revealed that the concentrations of n-3 PUFAs were elevated in patients with PHO compared with HCs, including 13(S)-HOTrE(γ), which was synthesized from α-LA, and ±19,20-EpDPE, ±13-HDoHE, ±8-HDoHE, which were synthesized from DHA, and 5S-HEPE and 15S-HEPE, which were synthesized from EPA. Correlation analysis between the above-changed n-3 PUFAs and levels of MMP1 and MMP13 revealed significant positive correlations between MMP13 and n-3 PUFAs, including ±19,20-EpDPE, ±13-HDoHE, ±8-HDoHE, 5S-HEPE, and 15S-HEPE. Meanwhile, MMP13 was found positively correlated with 9(S)-HODE, 13(S)-HODE, 11β-PGE2, and 8S,15S-DiHETE, the n-6 PUFAs. It was well known that n-3 and n-6 PUFAs had opposing effects on metabolic functions in the body, playing a significant role in regulating body homeostasis of inflammation and anti-inflammation, vasodilatation and vasoconstriction, and platelet aggregation and anti-aggregation (39). In patients with PHO, arthritic diseases such as arthralgia and arthritis were not the common syndrome, which only presented in 20%∼40% of patients with PHO (1). The increased concentration of the above n-3 PUFAs might result from feedback regulation, decreasing in an effort to resolve the inflammatory, platelet aggregation pathological conditions, and cartilage destruction and collagen degradation in patients with PHO. Overall, n-3 PUFAs including ±19,20-EpDPE, ±13-HDoHE, ±8-HDoHE, 5S-HEPE, and 15S-HEPE, and n-6 PUFAs including 9(S)-HODE, 13(S)-HODE, 11β-PGE2, and 8S,15S-DiHETE tended to affect joint development of patients with PHO.
PHOAR1 and PHOAR2 are the 2 subtypes of PHO, caused by either mutations in HPGD or in SLCO2A1, defecting in cytoplasmic oxidation of PGE2 or PGE2 uptake across the plasma membrane, respectively, resulting in the impairment of PGE2 degradation (4, 5). Defecting of SLCO2A1 resulted in the accumulation of PGE2. However, other forms of prostaglandin transporter, such as SLCO3A1 and SLCO4A1, could still transport PGE2 into the cell, which caused the increment of PGEM (7). Our previous study revealed that the urinary PGE2/PGEM level was significantly higher in patients with PHOAR1 than in patients with PHOAR2, suggesting that patients with PHOAR1 had more active PGE2 accumulation intracellularly. Patients with PHOAR1 had inferior bone microstructure and bone strength than patients with PHOAR2 (40). Associations between urinary PGE2/PGEM and trabecular bone microstructure suggested the time- and concentration-dependent PGE2 skeletal anabolism in trabecular bone microstructure between patients with PHOAR1 and patients with PHOAR2 (40). Therefore, to detect if the pathogenic genes influenced oxylipin metabolism, the study analyzed the statistical significance among patients with PHAOR1, patients with PHOAR2, and HCs in the differentially expressed metabolites. However, probably due to small samples of patients with PHOAR1, no significant differences in the metabolism of oxidized lipids between the 2 subtypes of patients with PHO were found. Moreover, the results of differentially expressed metabolites in patients with PHOAR1 and patients with PHOAR2 were similar to those in patients with PHO. The altered metabolite profile was due to increased PGE2 levels or disrupted SLCO2A1 function, yet to be determined in future studies. Association analysis revealed significant correlations among 5S-HETrE, 8S-HETrE, 15S-HETrE, 5S-HEPE, 15S-HEPE, 13(S)-HOTrE(γ), and 8S,15S-DiHETE and trabecular compartment including Tb.vBMD, Tb.BV/TV, Tb.N, and Tb.Sp only in patients with PHOAR2. 5S-HETrE, 8S-HETrE, 15S-HETrE, and 13(S)-HOTrE(γ) were derived from LA, and 5S-HEPE, and 15S-HEPE were synthesized from EPA. The above findings suggested that, besides the COX2 pathway, the LOX and CYP45 pathways had potential roles in the alterations of trabecular bone microstructure in patients with PHOAR2.
LTE4 was found to decrease in patients with PHO compared with HCs. Unlike LTB4, LTE4 was mainly responsible for bronchoconstriction, vasoconstriction, increased postcapillary permeability with edema, and smooth muscle constriction (31). The pathogenesis of finger clubbing was the deposition of collagen fibers in soft tissue, interstitial edema, dilated small blood vessels, vascular wall hyperplasia, and perivascular lymphocyte infiltration (8). Skin biopsy from the thickened forehead showed dermal edema, marked infiltration of mast cells in the dermis, mucin deposition, elastic fibrosis, sebaceous hypertrophy, and hyperplasia (41). The lower level of LTE4 in patients with PHO might have decreased the vasoconstriction and, in reverse, increased vascular wall permeability, contributing to the development of finger clubbing. Interestingly, LTE4 was positively correlated with total, cortical, and trabecular bone cross-sectional areas, suggesting that a lower level of LTE4 in patients with PHO might have an adverse effect on bone health by reducing bone formation.
There were some limitations concerning our study which should be recognized. First, the sample size was insufficient since PHO is a rare disease. We recruited 16 patients with PHO, which may have introduced selection bias because of interindividual differences. Thus, external replication and more extensive sample-sized studies are required to validate the observed differences in metabolomics. Second, we only used a metabolomics approach, and the possibility that single-omic data restricted the interpretation of our results cannot be excluded. Proteomics and genomics analyses are needed to confirm our findings. Third, the current study collected serum samples from the patients with PHO before treatment, considering that treatment with the COX2 selective inhibitor etoricoxib in patients with PHO has been revealed to alleviate the symptoms of PHO, including pachydermia, hyperhidrosis, joint swelling, and joint pain (6, 14). Establishing correlations between metabolic alterations and clinical outcomes would be of great interest. Future studies in large cohorts are needed to clarify associations between metabolites and clinical outcomes. Last but not least, these key metabolic signatures were measured in serum joint synovium fluid, which is more sensitive and accurate in defining the fate of articular cartilage. Future research would be focused on obtaining the synovium fluid to detect the effect of metabolites on joint lesions.
In conclusion, our study in this work provided the first targeted metabolomics analysis in PHO. UPLS-MS–based metabolomics of serum obtained from patients with PHO revealed distinct compositional differences compared with HCs. In addition to the COX/PGE2 pathway, the study for the first time revealed that the LOX pathway could also be activated in PHO. Furthermore, our study revealed that LTB4 and 5s-HETrE had adverse effects on bone development by impairing bone microstructure and reducing bone mineral density. In patients with PHOAR2, the LOX and CYP45 pathways had potential roles in the alterations of trabecular bone microstructure. Moreover, the study revealed that the n-3 PUFAs, including ±19,20-EpDPE, ±13-HDoHE, ±8-HDoHE, 5S-HEPE, and 15S-HEPE had positive roles in joint development, probably by decreasing to resolve the inflammatory, platelet aggregation pathological conditions and cartilage destruction and collagen degradation in patients with PHO. The study expanded our insight into uncovering the pathogenesis of skeletal and joint development in patients with PHO.
Acknowledgments
The authors acknowledge all the participants.
Funding
This study was supported by the National Key Research and Development Program of China (2021YFC2501700), Beijing Municipal Natural Science Foundation (7242106), National Natural Science Foundation of China (No. 82200993, No. 82100942, No. 82270938), Chinese Academy of Medical Sciences Innovation Fund for Medical Sciences (CIFMS) 2021-I2M-1-002, National High Level Hospital Clinical Research Funding (2022-PUMCH-D-006, 2022-PUMCH-B-014, 2022-PUMCH-A-202), and the Non-profit Central Research Institute Fund of Chinese Academy of Medical Sciences (2023-PT320-10).
Author Contributions
All authors were involved in drafting the article or revising it critically for important intellectual content, and all authors approved the final version to be published.
Disclosures
All authors have declared no conflicts of interest. Weibo Xia and Mei Li are Editorial Board Members for the Journal of Clinical Endocrinology & Metabolism and played no roles in the Journal's evaluation of the manuscript.
Data Availability
The data presented in this study are available on request from the corresponding author. The data are not publicly available due to privacy restrictions.
Ethics Approval and Consent to Participates
The study was rigorously approved by the Ethics Committee of Peking Union Medical College Hospital (Reference number I-23PJ2019).
References
Abbreviations
- AA
arachidonic acid
- aBMD
areal bone mineral density
- API
atmospheric pressure ionization
- AUC
area under the ROC curve
- COX
cyclooxygenase
- β-CTX
C-terminal telopeptide of type I collagen
- CYP
cytochrome P
- diHETE
dihydroxyeicosatetraenoic acid
- ELISA
enzyme-linked immunosorbent assay
- ESR
erythrocyte sedimentation rate
- FDR
false discovery rate
- HR-pQCT
high-resolution peripheral quantitative computed tomography
- hsCRP
high sensitivity C-reactive protein
- IL-6
interleukin 6
- LA
linolenic acid
- LOX
lipoxygenase
- LT
leukotriene
- MMP
matrix metalloproteinase
- MRM
multiple reaction monitoring
- NSAID
nonsteroidal anti-inflammatory drug
- OA
osteoarthritis
- OPG
osteoprotegerin
- OPLS-DA
orthogonal projections to latent structures discriminant analysis
- PLA2
phospholipase A2
- PGE2
prostaglandin E2
- PHO
primary hypertrophic osteoarthropathy
- PHOAR
primary hypertrophic osteoarthropathy autosomal recessive
- PUFA
polyunsaturated fatty acid
- PUMCH
Peking Union Medical College Hospital
- ROC
receiver operating characteristic
- TNF-α
tumor necrosis factor alpha
- UHPLC-MS/MS
ultra-high–performance liquid chromatography-tandem mass spectrometry
- VIP
variable importance in the projection