-
PDF
- Split View
-
Views
-
Cite
Cite
Mette Pinholt, Sarah Mollerup, Kit Boye, Peder Worning, Barbara Juliane Holzknecht, Sanne Nygaard, Karen Leth Nielsen, Henrik Hasman, Louise Roer, Anette M. Hammerum, Henrik Westh, Kristian Schønning, Investigation of the introduction and dissemination of vanB Enterococcus faecium in the Capital Region of Denmark and development of a rapid and accurate clone-specific vanB E. faecium PCR, Journal of Antimicrobial Chemotherapy, Volume 76, Issue 9, September 2021, Pages 2260–2267, https://doi.org/10.1093/jac/dkab198
- Share Icon Share
Abstract
During 2018–19, an increase of vanB vancomycin-resistant Enterococcus faecium (VREfm) was observed in the Capital Region of Denmark. vanA/vanB PCR performed directly on rectal swabs is accurate in detection of vanA; however, the positive predictive value for vanB-positive samples is low because of the presence of vanB in non-enterococcal gut commensals.
We investigated the epidemiology and clonal relatedness of vanB VREfm from the period 2015–19 and describe the application of a clone-specific vanB VREfm PCR assay for rapid and accurate detection of vanB VREfm in rectal screening samples.
vanB VREfm were investigated using epidemiological data and WGS data. The SeqSphere+ software was used to analyse MLST and cgMLST, and de novo assemblies were annotated to determine insertion sites for the vanB transposon (Tn1549). A clone-specific vanB VREfm PCR assay was designed to detect the sequence bridging Tn1549 and the E. faecium chromosome (araA2) in the dominant cluster.
Two hundred and seventy-five vanB VREfm isolates were identified, of which 76% were identified in 2019. A dominant cluster (Cluster 1, n = 204, 74%), six minor clusters and 15 singletons were identified. All Cluster 1 isolates and six non-Cluster 1 isolates had Tn1549 integrated into araA2. In 2019, the PCR assay would have detected 92% of all rectal screening samples containing vanB VREfm.
vanB VREfm increased due to the introduction and nosocomial transmission of the successful Cluster 1. The clone-specific PCR assay detected vanB VREfm outbreak isolates in rectal screening samples rapidly and accurately.
Introduction
Vancomycin-resistant Enterococcus faecium (VREfm) has emerged as an increasingly important nosocomial pathogen. Acquired resistance to ampicillin and vancomycin has increased worldwide among hospital-associated E. faecium, and VREfm infections are only treatable with last-resort antibiotics.
Vancomycin resistance mechanisms in E. faecium are encoded by several gene clusters (vanA to vanN).1 Worldwide, the vanA and vanB genotypes are most prevalent among clinical E. faecium.2 In Denmark, during 2005–19, the majority of the clinical VREfm cases were vanA VREfm.3 In 2018, an increase of vanB VREfm was observed among surveillance screening samples obtained in the Capital Region and, in 2019, clinical cases also increased.3,4 The vanB gene cluster is carried by a 34 kb conjugative Tn1549 transposon.5 Tn1549 transposons are located on plasmids or integrated into the chromosome.2 The transposon is organized into three functional regions: a region implicated in the excision/integration process; a region containing the vanB gene cluster; and a region implicated in the conjugative transfer.5 It has been suggested that vanB VREfm may arise de novo from hospital-associated vancomycin-susceptible E. faecium (VSEfm) either by transposition of the Tn1549 transposon (e.g. from anaerobic gut commensals) or by homologous recombination including Tn1549 between vanB VREfm and VSEfm.6,7
Infection control measures such as chlorine-based cleaning, VREfm surveillance screening and single-room isolation of patients colonized or infected with VREfm are used to control VREfm outbreaks. Rapid and accurate detection of VREfm is important to initiate infection control measures promptly. vanA/vanB PCR on rectal screening samples is accurate in detection of vanA; however, the positive predictive value for vanB is low because of the presence of vanB in non-enterococcal gut commensals (20%–25% of all rectal screening samples are vanB positive). Therefore, the introduction of vanB VREfm has challenged infection control procedures as definitive identification of vanB VREfm depends on culture results and therefore is delayed by 2–3 days.
The aim of the study was to investigate the introduction and dissemination of vanB VREfm to hospitals in the Capital Region of Denmark between January 2015 and August 2019 by analysing their genetic relatedness, epidemiological data and determining the insertion sites of the Tn1549 transposon in the isolates. In addition, vanB VREfm isolates were compared with temporally and geographically related VSEfm isolates to better understand the mobility of the transposon. Finally, we describe the application of a vanB VREfm clone-specific PCR assay that rapidly and accurately detects our vanB outbreak isolates in the routine clinical microbiology laboratory.
Methods
Settings and bacterial isolates
The study was conducted in the Capital Region of Denmark (1.8 million inhabitants) from January 2015 to August 2019. The Capital Region is served by 10 hospitals and three departments of clinical microbiology (DCMs). The first vanB VREfm isolate per patient per year was included in the study. Sample date, submitting ward and hospital, hospitalization history 3 months before sampling date, age, gender and sample site were collected from the laboratory information system. Information regarding admission abroad was obtained from rectal screening samples performed on all patients if they had been hospitalized abroad within the previous 6 months.
To reveal genetic relatedness between vanB VREfm and VSEfm, 296 VSEfm were included in the study: 159 clinical isolates identified in the Capital Region, 2012–15; and 137 isolates from rectal surveillance screenings sampled in the Capital Region, 2015–19. Clinical VSEfm were sequenced for a previous study whereas VSEfm from rectal samples were collected for the present study.8
Isolation and identification of E. faecium
Species identification was performed by MALDI-TOF MS (Bruker, Bremen, Germany). Susceptibility testing was performed by EUCAST disc diffusion technology and by vanA/vanB PCR-based methods [Xpert vanA/vanB assay on the GeneXpert system (Cepheid, Sunnyvale, CA, USA); the BioGX Vancomycin-Resistance OSR kit (BioGX, Amsterdam, Netherlands) on BD-MAX; or a laboratory-developed TaqMan assay on the Roche FLOW (Roche, Basel, Switzerland)].9 VREfm surveillance screening samples testing positive for vanB (∼20%–25%) were inoculated into selective brain heart infusion (BHI) broth containing 4 mg/L vancomycin and 60 mg/L aztreonam. After overnight culture, 10 μL of the enrichment broth was inoculated onto a VRE selective chromogenic plate and incubated for up to 48 h. E. faecium was isolated and the vanB genotype confirmed by PCR.
To isolate VSEfm, rectal swabs were obtained and inoculated into selective tryptic soy broth (TSB) containing 3.5 mg/L cefoxitin and 20 mg/L aztreonam. The overnight culture was inoculated onto an unselective blood agar plate. An ampicillin disc was placed on the agar plate and VSEfm was isolated from the inhibition zone. MALDI-TOF MS was performed for species identification and vanA/vanB PCR was performed to confirm the absence of van genes.
WGS and comparative analysis
WGS of E. faecium isolates was performed locally at the three DCMs in the region and at Statens Serum Institut using Illumina technology (MiSeq or NextSeq). Genomic DNA was extracted and 150 or 250 bp paired-end reads were produced as described previously.8,10,11 Sequencing reads were de novo assembled using SPAdes.12 MLST and core-genome MLST (cgMLST) were performed in SeqSphere+ v.7.2.3 (Ridom GmbH, Münster, Germany) and minimum spanning trees (MSTs) were produced to analyse genetic relatedness among vanB VREfm and among vanB VREfm and VSEfm. According to the previously published E. faecium cgMLST scheme, a complex type (CT) was defined as ≤20 allele distances from the founding isolate.13 Isolates were defined as belonging to a cluster if the distance to a minimum of one isolate was ≤20 alleles (i.e. a cluster can consist of more than one CT).
Determination of Tn1549 insertion sites and location in the genome
Insertion sites of Tn1549 were identified by identifying contigs encoding Tn1549 by BLAST searching using AF_192329.1. Usually the entire Tn1549 sequence was identified in two contigs in the WGS assembly. The outer boundaries of Tn1549 were identified within the WGS assembly and adjoining host bacterial sequences were identified. Adjoining concatenated sequences were used for BLAST searching against the NCBI nr/nt database to verify that the sequences were contiguous in isolates of E. faecium isolates not containing Tn1549.
Contigs were annotated using Rapid Annotation using Subsystem Technology (RAST).14 Nucleotide BLAST analysis of Tn1549 flanking regions (contig sequences upstream and downstream of Tn1549) against the NCBI nr/nt database was performed to reveal whether the sequences were located on the chromosome of a completed genome or on E. faecium plasmids.
Development of a clone-specific vanB PCR assay based on WGS data
A PCR targeting the sequence bridging Tn1549 and the E. faecium chromosome in isolates belonging to the dominant vanB VREfm cluster was developed. The target sequence was determined using WGS data. Contigs from a vanB VREfm isolate from the dominant cluster (V3183) were imported to CLC Main Workbench (QIAGEN, Aarhus, Denmark) and contigs containing Tn1549 elements were linked into an 83 825 bp sequence using Tn1549 (AF_192329.1) as a reference. Annotation of the sequence revealed that Tn1549 was integrated into an l-arabinose isomerase gene (araA2) (Figure S1, available as Supplementary data at JAC Online.). The target sequence for the araA2/Tn1549 PCR assay was selected with a forward primer in araA2 and a reverse primer in Tn1549. The PCR assay was based on the primer set forward (CCACTTTGTCTCCTTCTGTGACAC) and reverse (GGCTATACCGACATTCAAGAACTTC) and the TaqMan probe (Cyan500-GTTTCTCATGTTGTCACCAAAACGAGCCAC-BBQ), amplifying a 124 bp fragment. The sequences of the forward primer and the probe were only identified in E. faecium whereas the sequence of the reverse primer was identified in both E. faecium and non-enterococcal gut commensals in nucleotide BLAST searches against the NCBI nr/nt database.
The PCR assay was validated on isolates with Tn1549 integrated into araA2 and with an intact araA2, as predicted by genome analysis. In addition, the PCR assay was run on 618 rectal screening samples and compared with culture and WGS results.
Ethics
This project was approved by the Danish Data Protection Agency (2012-58-0004/AHH-2015-047) and the Danish Health and Medicines Authority (3-3013-1118/1).
Accession numbers
Illumina sequencing data for all isolates were deposited in the European Nucleotide Archive: PRJNA702038 and PRJEB40818.
Results
Descriptive data
In total, 275 vanB VREfm cases from 274 patients were identified during the study period, January 2015 to August 2019. During 2015–17, 1–6 cases per year were identified, increasing to 55 cases in 2018 and 210 cases in the first 8 months of 2019 (Figure 1). vanB VREfm isolates were identified in 61 (22%) clinical samples and 214 (78%) rectal screening samples, of which 7 (3%) were from patients screened after hospitalization abroad within the previous 6 months (Table 1). vanB VREfm were present in all hospitals in the region. Eight isolates contained both the vanA and the vanB gene cluster. More metadata are presented in Table 1.
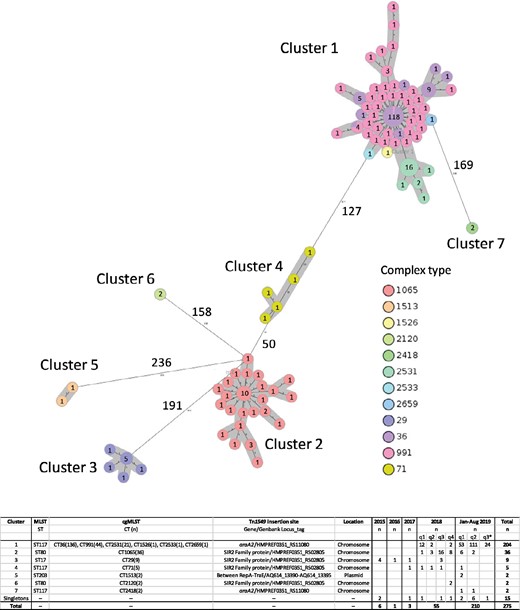
MST based on cgMLST of vanB VREfm (n = 260) identified in the Capital Region of Denmark, January 2015–August 2019. Fifteen singletons are removed from the figure. Circles represent a specific core-genome allelic profile and the numbers correspond to numbers of isolates with this specific profile. The colours indicate complex types (CTs) and clusters are marked in grey. The numbers next to the lines correspond to allele distances between the isolates. The chart indicates the association between clusters, STs, CTs, Tn1549 location in the genome, Tn1549 insertion sites and sampling dates. This figure appears in colour in the online version of JAC and in black and white in the print version of JAC.
Variables . | vanB E. faecium . |
---|---|
Gender, n (%) | |
Male | 148 (54) |
Average age (years), range | 71.5 (28–101) |
Medical specialty, n (%) | |
Cardiology and thoracic surgery | 41 (14.9) |
Infectious diseases | 37 (13.5) |
Geriatric | 31 (11.3) |
Gastrosurgery | 31 (11.3) |
Gastroenterology | 28 (10.2) |
ICU | 23 (8.4) |
Pulmonology | 18 (6.5) |
Orthopaedic surgery | 11 (4.0) |
Oncology | 10 (3.6) |
Other | 45 (16.4) |
Hospitals, n (%) | |
A | 28 (10.2) |
B | 2 (0.7) |
C | 77 (28.0) |
D | 36 (13.1) |
E | 66 (24) |
F | 41 (14.9) |
G | 4 (1.5) |
H | 12 (4.4) |
I | 5 (1.8) |
J | 1 (0.4) |
General practice | 3 (1.1) |
Specimen, n (%) | |
Rectal screening | 207 (75.3) |
Rectal screening, admission abroad | 7 (2.5) |
Blood | 2 (0.7) |
Urinary tract | 43 (15.6) |
Intra-abdominal | 4 (1.5) |
IV catheter | 1 (0.4) |
Other | 11 (4.0) |
Total | 275 |
Variables . | vanB E. faecium . |
---|---|
Gender, n (%) | |
Male | 148 (54) |
Average age (years), range | 71.5 (28–101) |
Medical specialty, n (%) | |
Cardiology and thoracic surgery | 41 (14.9) |
Infectious diseases | 37 (13.5) |
Geriatric | 31 (11.3) |
Gastrosurgery | 31 (11.3) |
Gastroenterology | 28 (10.2) |
ICU | 23 (8.4) |
Pulmonology | 18 (6.5) |
Orthopaedic surgery | 11 (4.0) |
Oncology | 10 (3.6) |
Other | 45 (16.4) |
Hospitals, n (%) | |
A | 28 (10.2) |
B | 2 (0.7) |
C | 77 (28.0) |
D | 36 (13.1) |
E | 66 (24) |
F | 41 (14.9) |
G | 4 (1.5) |
H | 12 (4.4) |
I | 5 (1.8) |
J | 1 (0.4) |
General practice | 3 (1.1) |
Specimen, n (%) | |
Rectal screening | 207 (75.3) |
Rectal screening, admission abroad | 7 (2.5) |
Blood | 2 (0.7) |
Urinary tract | 43 (15.6) |
Intra-abdominal | 4 (1.5) |
IV catheter | 1 (0.4) |
Other | 11 (4.0) |
Total | 275 |
Variables . | vanB E. faecium . |
---|---|
Gender, n (%) | |
Male | 148 (54) |
Average age (years), range | 71.5 (28–101) |
Medical specialty, n (%) | |
Cardiology and thoracic surgery | 41 (14.9) |
Infectious diseases | 37 (13.5) |
Geriatric | 31 (11.3) |
Gastrosurgery | 31 (11.3) |
Gastroenterology | 28 (10.2) |
ICU | 23 (8.4) |
Pulmonology | 18 (6.5) |
Orthopaedic surgery | 11 (4.0) |
Oncology | 10 (3.6) |
Other | 45 (16.4) |
Hospitals, n (%) | |
A | 28 (10.2) |
B | 2 (0.7) |
C | 77 (28.0) |
D | 36 (13.1) |
E | 66 (24) |
F | 41 (14.9) |
G | 4 (1.5) |
H | 12 (4.4) |
I | 5 (1.8) |
J | 1 (0.4) |
General practice | 3 (1.1) |
Specimen, n (%) | |
Rectal screening | 207 (75.3) |
Rectal screening, admission abroad | 7 (2.5) |
Blood | 2 (0.7) |
Urinary tract | 43 (15.6) |
Intra-abdominal | 4 (1.5) |
IV catheter | 1 (0.4) |
Other | 11 (4.0) |
Total | 275 |
Variables . | vanB E. faecium . |
---|---|
Gender, n (%) | |
Male | 148 (54) |
Average age (years), range | 71.5 (28–101) |
Medical specialty, n (%) | |
Cardiology and thoracic surgery | 41 (14.9) |
Infectious diseases | 37 (13.5) |
Geriatric | 31 (11.3) |
Gastrosurgery | 31 (11.3) |
Gastroenterology | 28 (10.2) |
ICU | 23 (8.4) |
Pulmonology | 18 (6.5) |
Orthopaedic surgery | 11 (4.0) |
Oncology | 10 (3.6) |
Other | 45 (16.4) |
Hospitals, n (%) | |
A | 28 (10.2) |
B | 2 (0.7) |
C | 77 (28.0) |
D | 36 (13.1) |
E | 66 (24) |
F | 41 (14.9) |
G | 4 (1.5) |
H | 12 (4.4) |
I | 5 (1.8) |
J | 1 (0.4) |
General practice | 3 (1.1) |
Specimen, n (%) | |
Rectal screening | 207 (75.3) |
Rectal screening, admission abroad | 7 (2.5) |
Blood | 2 (0.7) |
Urinary tract | 43 (15.6) |
Intra-abdominal | 4 (1.5) |
IV catheter | 1 (0.4) |
Other | 11 (4.0) |
Total | 275 |
Genetic relatedness based on cgMLST
The MST of vanB VREfm revealed seven clusters and 15 singletons (Figure 1). Cluster 1 (ST117/CT36/CT991/CT2531/CT1526/CT2533/CT2659) was highly dominant, containing 204 isolates (74%). Clusters 2 (ST80/CT1065), 3 (ST17/CT29) and 4 (ST117/CT71) contained 36 (13%), 9 (3%) and 5 (2%) isolates, respectively. The remaining three clusters only contained two isolates. In 2019, more singletons were identified (n = 9) compared with previous years (n = 1–3).
A representative isolate from each vanB VREfm cluster and all singletons were compared with temporally and geographically related VSEfm (n = 296) in an MST (Figure S2). vanB VREfm were spread widely across the MST. Cluster 5 isolates and three singletons clustered with VSEfm. Three vanB VREfm cluster representatives (Clusters 1, 4 and 6) and four singletons had allele distances to the nearest VSEfm between 40 and 100 alleles. The remaining vanB VREfm isolates (n = 11) had allele distances to the nearest VSEfm between 100 and 500 alleles.
Cluster analysis, comparison with epidemiological data and Tn1549 insertion sites
Isolates belonging to the dominant Cluster 1 were identified from January to April 2018 (n = 14) and again from December 2018 to August 2019 (n = 190) (Figure 1). Epidemiological data revealed that the index patient was transferred from an ICU in Germany to an ICU (Hospital A) in the Capital Region of Denmark. The index patient was isolated in a single room throughout the hospitalization. Twelve of 14 isolates were identified in the same hospital (Hospital A) and the remaining 2 isolates were identified in patients hospitalized at Hospital A during the outbreak period, thus indicating nosocomial transmission (Figure 2a).
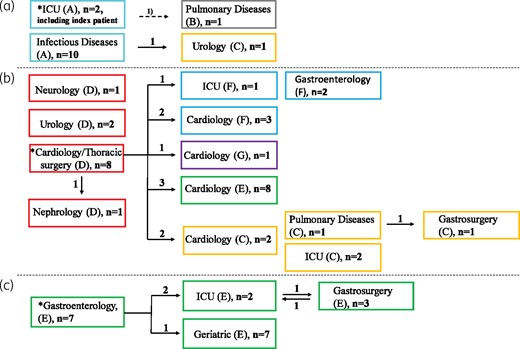
Epidemiological data of vanB VREfm belonging to Cluster 1. (a) Isolates identified from January 2018 to April 2018 (n = 14 patients). (b) and (c) Isolates identified from December 2018 to March 2019 with an epidemiological link to departments of cardiology/thoracic surgery (Hospital D; n = 33 patients) or gastroenterology (Hospital E; n = 19 patients). Squares represent wards. Colours and capital letters in parentheses indicate different hospitals. Numbers (n) of vanB VREfm on different wards are indicated in the squares. Numbers next to the arrows indicate the number of patients who were transferred to another ward and were detected with vanB VREfm in the receiving ward. Arrow labelled ‘1)’ denotes the vanB VREfm-positive patient in the Department of Pulmonology was admitted for 5 days in the same ward (Department of Neurology) as the index patient before transfer to the Department of Pulmonology. * denotes the index ward. This figure appears in colour in the online version of JAC and in black and white in the print version of JAC.
Seven months later, Cluster 1 isolates were identified in two other hospitals (Hospitals D and E). In both hospitals, epidemiological data revealed an increase of Cluster 1 isolates in their index wards [cardiology/thoracic surgery, Hospital D (n = 8); and gastroenterology, Hospital E (n = 7)] and subsequent dissemination via patient transfer to new wards and hospitals (Figures 2b and c). Within 4 months (December 2018–March 2019), 33 and 19 Cluster 1 isolates with an epidemiological link to their index wards were identified in Hospitals D and E, respectively. The outbreak identified at Hospital E disseminated to other wards within the hospital, whereas the outbreak at Hospital D (a tertiary referral hospital) disseminated within the hospital and to four other hospitals within wards of the same specialty (cardiology). In 16 cases, patients were transferred from a vanB VREfm ward and were detected with vanB VREfm in the receiving ward, supporting nosocomial transmission and dissemination based on patient transfer (Figure 2). Identical insertion site in all Cluster 1 isolates also supports nosocomial transmission. Three Cluster 1 isolates had no epidemiological link.
At the end of the study period (April–August 2019), Cluster 1 continued to increase (n = 135) and was identified in all but one hospital (n = 9) in the region, mainly in the same specialties as previously described [gastroenterology/surgery (20%), cardiology/thoracic surgery (13%), geriatric (13%), infectious diseases (13%), respiratory diseases (9%), ICU (8%) and orthopaedic surgery (7%)].
Cluster 2 isolates (n = 36) were identified in a 13 month period (June 2018–June 2019). The index patient was transferred from a Greek hospital to Hospital C. Most isolates (n = 30) were identified at Hospital C and four isolates were identified in patients with previous admission to this hospital. The Tn1549 insertion site was the same in all isolates, thus genetic and epidemiological data suggested nosocomial transmission.
Cluster 3 isolates (n = 9) were identified in the same hospital (Hospital A). A cluster of four screening isolates was incidentally found within 1 week in 2015 in relation to a vanA VREfm outbreak. In 2016 and 2017, two isolates were found from one patient and, on the same ward, three isolates were identified within 1.5 months in 2018. cgMLST revealed genetically closely related isolates (≤5 allele distances) (Figure S3) and all isolates had the same Tn1549 insertion site. Thus, readmission of patients involved in the outbreak is likely to explain this cluster, but reintroduction of the clone via patient transfer from foreign countries cannot be excluded.
Tn1549 insertion sites and location in the genome
Isolates belonging to Cluster 1 and Cluster 7 and four singletons had the same Tn1549 insertion site with the transposon integrated into araA2 (GenBank locus_tag number: HMPREF0351_RS11080) (Table S1). Flanking regions of Tn1549 were located on the chromosome of >15 E. faecium completed genomes at NCBI (Figure 1), thus indicating that Tn1549 was integrated into the chromosome. In addition, isolates belonging to Clusters 2, 3, 4 and 6 and four singletons had Tn1549 integrated into a gene encoding a silent information regulator 2 (SIR2) family protein (GenBank locus_tag: HMPREF0351_RS02805) and also here, flanking regions of Tn1549 were located on the chromosome of >15 E. faecium genomes. In Cluster 5, Tn1549 was integrated between two genes encoding replication initiator protein A (GenBank locus_tag: _13390) and conjugal transfer protein TraE (GenBank locus_tag: _13395). Tn1549 flanking regions were located on >5 E. faecium plasmids (e.g. E. faecium strain UW7606x64/3 TC1 plasmid pWCF-TC1, CP013010.1) indicating that Cluster 5 isolates had Tn1549 located on a plasmid. In Table S1, annotations of Tn1549-carrying contigs are shown for a representative isolate of each cluster.
Validation and application of the clone-specific araA2/Tn1549 PCR assay
The araA2/Tn1549 PCR assay was tested on 16 E. faecium isolates with Tn1549 integrated into araA2 and 7 isolates with an intact araA2. Results from PCR were fully congruent with WGS. In the final 38 days of the study period, the araA2/Tn1549 PCR was run on all routine vanA/vanB VREfm rectal screening samples submitted to one DCM (n = 618 samples). One hundred and sixty-one samples were vanB positive, of which eight samples were araA2/Tn1549 positive. Subsequent culturing and WGS of araA2/Tn1549-positive samples confirmed that Tn1549 was integrated into araA2 in all isolates and the PCR results were true positives. The remaining 153 vanB-positive and araA2/Tn1549-negative samples were culture negative, confirming that the araA2/Tn1549 PCR results were true negatives and that no other vanB VREfm clones were present during the 38 days.
In silico PCR revealed the presence of the 124 bp target sequence in WGS data from all Cluster 1 isolates, Cluster 7 isolates (n = 2) and four singletons. In 2019, 92% (n = 147) of the rectal screening isolates and 92% (n = 47) of the clinical isolates would have been detected by the PCR assay. In 2018, only 29% (n = 16) of the isolates would have been detected, and during 2015–17, no isolates would have been detected as they belonged to other clusters.
Discussion
The present study conducted in the Capital Region of Denmark, between January 2015 and August 2019, revealed a marked increase in the number of vanB VREfm clinical cases and intestinal carriers during 2018–19. WGS revealed an outbreak with a highly dominant cluster and epidemiological data demonstrated that many patients were hospitalized in the same ward or hospital at the same time (within days to a few months), providing strong evidence for nosocomial transmission of vanB VREfm. This was supported by a recent study reporting closely related VREfm from patients and their direct hospital environment.15 In addition, the study demonstrated multiple patients who were transferred from a vanB VREfm ward and were detected with vanB VREfm in the receiving ward, thus suggesting that intra- and interhospital transfer of unknown vanB VREfm carriers was an important driver for dissemination of vanB VREfm in the hospital network and for initiation of local outbreaks. In 2019, the outbreak clone was identified in all but one hospital (n = 9) in the region, which shows how fast a new VREfm clone can be established and disseminated. This underlines the importance of rapid PCR screening diagnostics and ongoing surveillance to detect VREfm and intervention early in the process. cgMLST with a standardized nomenclature is useful for regional (or national or international) surveillance as comparison of data is possible even though sequencing is performed at several facilities, as in our region. In the Capital Region, VREfm sequencing data were shared at Computerome, the National Life Science Supercomputing Center (https://www.computerome.dk/).
Another driver of vanB VREfm was importation via patients transferred from foreign hospitals. In the present study, the outbreak clone was introduced to the Capital Region in 2018 via the index patient, who was transferred from a German hospital. This was supported by sequencing data revealing that prior to 2018, the outbreak cluster was not identified in Denmark, neither among VREfm nor VSEfm (n = 296);4 however, the outbreak clone was reported among German blood and screening isolates during 2017–18.16,17 In addition to the index patient of Cluster 1, six more vanB VREfm patients were transferred from foreign hospitals, including the index patient of Cluster 2, who was transferred from a Greek hospital. Finally, Cluster 2 (ST80/CT1065) and Cluster 4 (ST117/CT71) were highly frequent among German vanB VREfm during 2017–18, revealing the occurrence of identical VREfm clones across national borders.16–18 Therefore, VREfm surveillance screening of patients hospitalized abroad is important to identify VREfm carriers and prevent unknown carriers starting outbreaks.
Analyses of the insertion site of Tn1549 revealed that most transposons were chromosomally integrated. Tn1549 only had two insertion sites among 97% (n = 266) of the vanB VREfm isolates, which is partly explained by the clonal pattern among the vanB VREfm. The two insertion sites were identified in 6 of 7 clusters and 8 of 15 singletons, which may represent introductions of several clones containing the same insertion site. Others have found similar results with a high frequency of the same SIR2 family protein insertion site among German ST192 vanB VREfm.6
Repeated insertion of Tn1549 from gut commensals into ‘hotspot’ positions in E. faecium could also explain the frequent occurrence of the two insertion sites in vanB VREfm; however, genome-based analyses of vanB VREfm around the world demonstrated various potential insertion sites across the genome.6,7,19 Another explanation for the frequent occurrence of the two insertion sites could be generation of vanB VREfm from the circulating VSEfm population via homologous recombination of large fragments among vanB VREfm and VSEfm. Four representative vanB VREfm clustered with VSEfm and seven representatives had 40–100 allele distances to VSEfm (Figure S2). Filter-mating experiments have demonstrated that Tn1549 exhibits the capability to transfer as part of a large chromosomal fragment (100 000–600 000 bp).6 Recombination events involving >100 000 bp probably result in multiple allele differences, thus indicating that vanB VREfm and VSEfm with <100 allele distances could be related. The six non-Cluster 1 isolates with Tn1549 integrated into araA2 were epidemiologically related to Cluster 1 as they were identified while the outbreak peaked and on Cluster 1 outbreak wards. In addition, three of the non-Cluster 1 isolates had 15, 40 and 85 allele distances to VSEfm, thus indicating that homologous recombination between Cluster 1 vanB VREfm and VSEfm may have generated new VREfm clones. However, the representative isolates of the two most prominent vanB VREfm Clusters 1 and 2 and several other representative isolates were not intermingled with VSEfm ST/CT types, but stood alone at far branches of the MST, thus suggesting that generation of vanB VREfm from VSEfm progenitors was not the main driver for vanB VREfm.
The few Tn1549 insertion sites identified in the study highly suggested that it was possible to develop PCR assays detecting vanB VREfm rapidly and accurately on a rectal screening sample. Development of the araA2/Tn1549 PCR assay was preferred as 92% of all vanB VREfm screening isolates contained the araA2 insertion site in 2019. The PCR was introduced in parallel with the vanA/vanB PCR assay in the routine laboratory in one DCM in July 2019. The main advantage was the rapid detection of the majority of vanB VREfm in Copenhagen and initiation of infection control measures, as the PCR result is available within 8–24 h compared with approximately 3 days for the culture result. Culture of vanB-positive samples is still necessary to identify vanB VREfm with other Tn1549 insertion sites and to gain WGS data for surveillance. Zhou et al.20 evaluated the Xpert vanA/vanB PCR assay (GeneXpert) on rectal swabs and on enriched inoculated broths and demonstrated that a CT value of ≤25 for enriched inoculated broths is compatible with the presence of vanB VREfm in the samples determined by culturing. This method identified all subtypes of vanB VREfm and was faster than culture, albeit delayed by 24 h compared with the araA2/Tn1549 PCR assay.
In addition to fast detection of vanB VREfm, the PCR assay was almost clone specific in the Capital Region setting, where 97% (204) of the isolates with Tn1549 integrated into araA2 belonged to Cluster 1. Therefore, the PCR could be used as a typing tool with a resolution like cgMLST and a turnaround time of only 8–24 h compared with 10 working days in our routine WGS laboratory. Of course, this typing approach requires ongoing WGS surveillance of vanB VREfm to identify new clones and Tn1549 insertion sites.
Development of the second PCR assay (SIR2/Tn1549) was postponed due to the COVID-19 pandemic. The SIR2/Tn1549 bridging PCR assay would not be cluster specific in the Capital Region setting, however; the PCR assay would be sufficient to determine whether vanB VREfm is present or not in a rectal screening sample, which is of great importance for infection control of VREfm. We know that Tn1549 exhibits the capacity to transfer as part of a large fragment. In a future study, the exact excision and insertion site of the element could be resolved using long-read sequencing and the information could be used to better understand dissemination of Tn1549 and to develop new cluster-specific PCR assays. In addition to long-read sequencing, it would be interesting to determine all Tn1549 insertion sites in vanB VREfm uploaded to NCBI in order to design PCR assays that can detect the most frequent insertion sites.
In conclusion, vanB VREfm increased in the Capital Region of Denmark during 2018–19, mainly due to a successful clone that has disseminated in the hospital network within less than a year. We succeeded in developing a PCR assay that addresses the challenge of detecting vanB VREfm directly in the rectal screening sample by PCR and, as an additional benefit, the PCR assay acted as a high-resolution typing tool, detecting the outbreak clone in our region. Ongoing WGS surveillance is necessary to identify new vanB VREfm clones introduced via patient transfer from foreign hospitals or generated by homologous recombination, to develop new clone-specific vanB VREfm PCR assays and to initiate infection control measures promptly.
Acknowledgements
Part of the data was presented at the 12th International Meeting on Microbial Epidemiological Markers, Dubrovnik, Croatia, 2019 (Poster 289). We thank WGS staff at DCM, Hvidovre; DCM, Herlev; DCM, Rigshospitalet; and Statens Serum Institut for excellent technical assistance.
Funding
This work was supported by Copenhagen University Hospital—Amager and Hvidovre.
Transparency declarations
None to declare.
Supplementary data
Table S1 and Figures S1 to S3 are available as Supplementary data at JAC Online.
References
DANMAP. Use of antimicrobial agents and occurrence of antimicrobial resistance in bacteria from food animals, food and humans in Denmark. DANMAP. http://www.danmap.org// (all reports).
EUCAST. Clinical breakpoints. http://www.eucast.org/clinical_breakpoints/.
Epidemiologisches Bulletin, 35/2019. https://www.rki.de/DE/Content/Infekt/EpidBull/Archiv/2019/Ausgaben/35_19.htm.