-
PDF
- Split View
-
Views
-
Cite
Cite
Ze-Hua Cui, Wei-Na Ni, Tian Tang, Bing He, Zi-Xing Zhong, Liang-Xing Fang, Liang Chen, Chong Chen, Chao-Yue Cui, Ya-Hong Liu, Xiao-Ping Liao, Jian Sun, Rapid detection of plasmid-mediated high-level tigecycline resistance in Escherichia coli and Acinetobacter spp, Journal of Antimicrobial Chemotherapy, Volume 75, Issue 6, June 2020, Pages 1479–1483, https://doi.org/10.1093/jac/dkaa029
- Share Icon Share
Abstract
The emergence and spread of plasmid-encoded tet(X3/X4) genes that confer high-level tigecycline and eravacycline resistance in Escherichia coli and Acinetobacter spp. pose serious threats to human and animal health. We developed a rapid and robust assay to detect Tet(X3/X4) in Gram-negative bacteria based on eravacycline degradation by the presence of the Tet(X) enzyme in the test strain.
This tetracycline inactivation method (TIM) is based on the degradation of eravacycline by the Tet(X3/X4)-producing strain, which results in reduced eravacycline activity against an acid-producing thermophile Bacillus stearothermophilus indicator strain. For Tet(X)-negative strains, eravacycline retains its antimicrobial activity. Coupled with a pH-sensitive dye (bromocresol purple), the reduced colorimetric inhibition zone can be measured to determine the production of Tet(X3/X4). One hundred and eighteen isolates, including 30 tet(X4)-positive E. coli, 30 tet(X3)-positive Acinetobacter spp. and 58 tet(X)-negative E. coli and Acinetobacter spp., were examined to evaluate the performance of this TIM.
The sensitivity and specificity for E. coli carrying tet(X4) was 96.7% and 100%, respectively, and for Acinetobacter spp. carrying tet(X3) both were 100%. The TIM assay can be completed within 6.5 h.
The TIM is a simple, rapid and cost-effective method for the detection of plasmid-mediated high-level tigecycline resistance in E. coli and Acinetobacter spp.
Introduction
Tigecycline is one of the last-resort antibiotics to treat complicated infections caused by MDR Gram-negative and Gram-positive pathogens.1 Recently, a novel plasmid-mediated enzymatic tigecycline resistance mechanism, Tet(X), has been reported in Enterobacteriaceae and Acinetobacter spp. in China. The tet(X3/X4) genes confer high-level tigecycline resistance and are present on mobilizable plasmids, allowing horizontal transfer of resistance between different strains and species.2 Unlike the general tigecycline resistance mechanism,3,4 the Tet(X3/X4) enzymes can directly inactivate, by hydroxylation at carbon 11a,2,5 all tetracycline antibiotics, including the newly US FDA-approved eravacycline and omadacycline. This threatens the clinical efficacy of the entire family of tetracycline antibiotics.5 Worryingly, plasmid-borne tet(X3/X4) genes have been identified in different ecological niches, including livestock, environment and clinical samples.6
In this study, we developed a simple, rapid and cost-effective tetracycline inactivation method (TIM) to detect Tet(X3/X4)-producing bacteria, based on the ability to degrade tetracycline conferred by the presence of the Tet(X) enzymes. We used Bacillus stearothermophilus as the indicator strain coupled with the pH-sensitive dye bromocresol purple as pH indicator, developing a rapid assay for Tet(X3/X4) in Escherichia coli and Acinetobacter spp.
Materials and methods
Bacterial strains
One hundred and eighteen bacterial strains, consisting of 30 tet(X3)-positive and 15 tet(X)-negative Acinetobacter spp., 30 tet(X4)-positive, 27 tet(X)-negative E. coli but carrying at least one other tetracycline resistance gene, and 16 E. coli that do not possess any tetracycline resistance gene (Table 1). These test strains were isolated from faeces, soil, dust, sewage and vegetable samples. The faecal samples were collected from pigs, chickens, and patients at a tertiary hospital in Guangdong. All strains were identified at species level using MALDI-TOF MS (Axima-Assurance-Shimadzu). Control strains were E. coli JM109 containing the plasmid vectors pBAD24 and pBAD24-tet(X4), which were constructed from E. coli JM109 transformed with the recombinant plasmids pBAD24 and pBAD24-tet(X4), respectively. Plasmid gene expression is controlled by the BAD promoter and all cultures included the inducer, arabinose. B. stearothermophilus 7453 was used as the indicator strain, and was cultured at 60°C.
. | . | . | MIC . | No. positive/total TIM tests . | ||||
---|---|---|---|---|---|---|---|---|
Species . | Strains (n) . | Genes . | TET (1)a . | DOX (2)a . | TGC (3)a . | ERV (4)a . | OMA (4)a . | |
Indicator strain | ||||||||
B. stearothermophilus | 1 | – | 0.25 | 0.125 | 0.06 | 0.004 | 0.125 | – |
Control strains | ||||||||
E. coli-JM109-pBAD24-tet(X4) | 1 | tet(X4) | 64 | 16 | 8 | 2 | 16 | 1/1 |
E. coli JM109-pBAD24 | 1 | – | 2 | 0.5 | 0.03 | 0.008 | 0.125 | 0/1 |
Test strains | ||||||||
TIE producers | 60 | |||||||
Acinetobacter spp. | 30 | tet(X3) | 64–128 | 1–64 | 8–64 | 4–32 | 8–64 | 30/30 |
E. coli | 30 | tet(X4) | 32 to >256 | 32–64 | 4–32 | 4–16 | 16–64 | 29/30 |
Non-TIE producersb | 58 | |||||||
E. coli | 15 | tet(A) | 64–256 | 16–256 | 0.06–1 | 0.06–2 | 0.25–2 | 0/15 |
E. coli | 4 | tet(B) | 256 | 32 to >256 | 0.125–2 | 0.06–1 | 2–8 | 0/4 |
E. coli | 1 | tet(D) | 256 | 32 | 0.25 | 0.25 | 4 | 0/1 |
E. coli | 1 | tet(M) | 128 | 64 | 0.125 | 0.25 | 2 | 0/1 |
E. coli | 4 | tet(D)-tet(M) | 128 to >256 | 32–64 | 0.25–0.5 | 0.25–0.5 | 2–8 | 0/4 |
E. coli | 2 | tet(A)-tet(B) | 256 | 64 | 0.03–0.125 | 0.03–0.06 | 0.25–0.5 | 0/2 |
E. coli 25922 | 1 | – | 2 | 0.5 | 0.03 | 0.06 | 0.25 | 0/1 |
E. coli | 15 | non-tet(X)c | 64 to >256 | 16–64 | 0.03–0.25 | 0.03–0.25 | 0.25–1 | 0/15 |
Acinetobacter spp. | 15 | non-tet(X)c | ≥256 | 32–128 | 0.125–0.5 | 0.125–0.25 | 0.25–2 | 0/15 |
. | . | . | MIC . | No. positive/total TIM tests . | ||||
---|---|---|---|---|---|---|---|---|
Species . | Strains (n) . | Genes . | TET (1)a . | DOX (2)a . | TGC (3)a . | ERV (4)a . | OMA (4)a . | |
Indicator strain | ||||||||
B. stearothermophilus | 1 | – | 0.25 | 0.125 | 0.06 | 0.004 | 0.125 | – |
Control strains | ||||||||
E. coli-JM109-pBAD24-tet(X4) | 1 | tet(X4) | 64 | 16 | 8 | 2 | 16 | 1/1 |
E. coli JM109-pBAD24 | 1 | – | 2 | 0.5 | 0.03 | 0.008 | 0.125 | 0/1 |
Test strains | ||||||||
TIE producers | 60 | |||||||
Acinetobacter spp. | 30 | tet(X3) | 64–128 | 1–64 | 8–64 | 4–32 | 8–64 | 30/30 |
E. coli | 30 | tet(X4) | 32 to >256 | 32–64 | 4–32 | 4–16 | 16–64 | 29/30 |
Non-TIE producersb | 58 | |||||||
E. coli | 15 | tet(A) | 64–256 | 16–256 | 0.06–1 | 0.06–2 | 0.25–2 | 0/15 |
E. coli | 4 | tet(B) | 256 | 32 to >256 | 0.125–2 | 0.06–1 | 2–8 | 0/4 |
E. coli | 1 | tet(D) | 256 | 32 | 0.25 | 0.25 | 4 | 0/1 |
E. coli | 1 | tet(M) | 128 | 64 | 0.125 | 0.25 | 2 | 0/1 |
E. coli | 4 | tet(D)-tet(M) | 128 to >256 | 32–64 | 0.25–0.5 | 0.25–0.5 | 2–8 | 0/4 |
E. coli | 2 | tet(A)-tet(B) | 256 | 64 | 0.03–0.125 | 0.03–0.06 | 0.25–0.5 | 0/2 |
E. coli 25922 | 1 | – | 2 | 0.5 | 0.03 | 0.06 | 0.25 | 0/1 |
E. coli | 15 | non-tet(X)c | 64 to >256 | 16–64 | 0.03–0.25 | 0.03–0.25 | 0.25–1 | 0/15 |
Acinetobacter spp. | 15 | non-tet(X)c | ≥256 | 32–128 | 0.125–0.5 | 0.125–0.25 | 0.25–2 | 0/15 |
TET, tetracycline; DOX, doxycycline; TGC, tigecycline; ERV, eravacycline; OMA, omadacycline.
The number in parentheses indicates the generation of tetracycline.
TIE, tetracycline inactivation enzyme.
Non-tet(X) strains lack any tet gene besides tet(X).
. | . | . | MIC . | No. positive/total TIM tests . | ||||
---|---|---|---|---|---|---|---|---|
Species . | Strains (n) . | Genes . | TET (1)a . | DOX (2)a . | TGC (3)a . | ERV (4)a . | OMA (4)a . | |
Indicator strain | ||||||||
B. stearothermophilus | 1 | – | 0.25 | 0.125 | 0.06 | 0.004 | 0.125 | – |
Control strains | ||||||||
E. coli-JM109-pBAD24-tet(X4) | 1 | tet(X4) | 64 | 16 | 8 | 2 | 16 | 1/1 |
E. coli JM109-pBAD24 | 1 | – | 2 | 0.5 | 0.03 | 0.008 | 0.125 | 0/1 |
Test strains | ||||||||
TIE producers | 60 | |||||||
Acinetobacter spp. | 30 | tet(X3) | 64–128 | 1–64 | 8–64 | 4–32 | 8–64 | 30/30 |
E. coli | 30 | tet(X4) | 32 to >256 | 32–64 | 4–32 | 4–16 | 16–64 | 29/30 |
Non-TIE producersb | 58 | |||||||
E. coli | 15 | tet(A) | 64–256 | 16–256 | 0.06–1 | 0.06–2 | 0.25–2 | 0/15 |
E. coli | 4 | tet(B) | 256 | 32 to >256 | 0.125–2 | 0.06–1 | 2–8 | 0/4 |
E. coli | 1 | tet(D) | 256 | 32 | 0.25 | 0.25 | 4 | 0/1 |
E. coli | 1 | tet(M) | 128 | 64 | 0.125 | 0.25 | 2 | 0/1 |
E. coli | 4 | tet(D)-tet(M) | 128 to >256 | 32–64 | 0.25–0.5 | 0.25–0.5 | 2–8 | 0/4 |
E. coli | 2 | tet(A)-tet(B) | 256 | 64 | 0.03–0.125 | 0.03–0.06 | 0.25–0.5 | 0/2 |
E. coli 25922 | 1 | – | 2 | 0.5 | 0.03 | 0.06 | 0.25 | 0/1 |
E. coli | 15 | non-tet(X)c | 64 to >256 | 16–64 | 0.03–0.25 | 0.03–0.25 | 0.25–1 | 0/15 |
Acinetobacter spp. | 15 | non-tet(X)c | ≥256 | 32–128 | 0.125–0.5 | 0.125–0.25 | 0.25–2 | 0/15 |
. | . | . | MIC . | No. positive/total TIM tests . | ||||
---|---|---|---|---|---|---|---|---|
Species . | Strains (n) . | Genes . | TET (1)a . | DOX (2)a . | TGC (3)a . | ERV (4)a . | OMA (4)a . | |
Indicator strain | ||||||||
B. stearothermophilus | 1 | – | 0.25 | 0.125 | 0.06 | 0.004 | 0.125 | – |
Control strains | ||||||||
E. coli-JM109-pBAD24-tet(X4) | 1 | tet(X4) | 64 | 16 | 8 | 2 | 16 | 1/1 |
E. coli JM109-pBAD24 | 1 | – | 2 | 0.5 | 0.03 | 0.008 | 0.125 | 0/1 |
Test strains | ||||||||
TIE producers | 60 | |||||||
Acinetobacter spp. | 30 | tet(X3) | 64–128 | 1–64 | 8–64 | 4–32 | 8–64 | 30/30 |
E. coli | 30 | tet(X4) | 32 to >256 | 32–64 | 4–32 | 4–16 | 16–64 | 29/30 |
Non-TIE producersb | 58 | |||||||
E. coli | 15 | tet(A) | 64–256 | 16–256 | 0.06–1 | 0.06–2 | 0.25–2 | 0/15 |
E. coli | 4 | tet(B) | 256 | 32 to >256 | 0.125–2 | 0.06–1 | 2–8 | 0/4 |
E. coli | 1 | tet(D) | 256 | 32 | 0.25 | 0.25 | 4 | 0/1 |
E. coli | 1 | tet(M) | 128 | 64 | 0.125 | 0.25 | 2 | 0/1 |
E. coli | 4 | tet(D)-tet(M) | 128 to >256 | 32–64 | 0.25–0.5 | 0.25–0.5 | 2–8 | 0/4 |
E. coli | 2 | tet(A)-tet(B) | 256 | 64 | 0.03–0.125 | 0.03–0.06 | 0.25–0.5 | 0/2 |
E. coli 25922 | 1 | – | 2 | 0.5 | 0.03 | 0.06 | 0.25 | 0/1 |
E. coli | 15 | non-tet(X)c | 64 to >256 | 16–64 | 0.03–0.25 | 0.03–0.25 | 0.25–1 | 0/15 |
Acinetobacter spp. | 15 | non-tet(X)c | ≥256 | 32–128 | 0.125–0.5 | 0.125–0.25 | 0.25–2 | 0/15 |
TET, tetracycline; DOX, doxycycline; TGC, tigecycline; ERV, eravacycline; OMA, omadacycline.
The number in parentheses indicates the generation of tetracycline.
TIE, tetracycline inactivation enzyme.
Non-tet(X) strains lack any tet gene besides tet(X).
Antibiotic susceptibility testing
Antibiotic susceptibility testing was performed and interpreted according to the CLSI guidelines (2015) using the agar dilution method for tetracycline and doxycycline and the microdilution broth method for tigecycline, omadacycline and eravacycline. The tigecycline breakpoints for E. coli and Acinetobacter spp. were interpreted according to the FDA criteria as susceptible (≤2 mg/L), intermediate (4 mg/L) and resistant (≥8 mg/L); eravacycline and omadacycline were uninterpreted as there are no breakpoints. E. coli ATCC 25922 was used as the quality control strain.
Detection of tetracycline resistance genes
The tetracycline resistance genes, including tet(X3), tet(X4), tet(A), tet(B), tet(D) and tet(M), were detected by PCR as previously described.2,7 In addition, a tet(X) universal PCR was designed to examine the potential presence of tet(X) variants other than tet(X3) and tet(X4).
Preparation of colorimetric plates
Colorimetric plates were prepared by dissolving 18 mg bromocresol purple in 10 mL sterile distilled water. Modified Mueller–Hinton agar was prepared by adding 10 mL of bromocresol purple reagent and 10 mL of the log-phase growth indicator strain B. stearothermophilus 7453 (1 × 108 cfu/mL) into 980 mL freshly prepared and cooled (45°–50°C) Mueller–Hinton agar (pH 7.0). Colorimetric plates were prepared by pouring 20 mL modified Mueller–Hinton agar medium into 90 mm bacteriological Petri dishes to obtain a ∼4 mm depth.
Tetracycline inactivation method
A 10 μL loopful of an overnight culture was vortexed in an Eppendorf tube containing 500 μL of eravacycline 2.5 mg/L and 1 mM NADPH (Sigma, USA). A blank disc was added to the suspension and vortexed for 1 min, followed by incubation at 37°C for 3 h, and then vortexed for 30 s. The disc was placed on a colorimetric plate and incubation was continued at 60°C until inhibition zones were clearly visible (Figure 1a). E. coli JM109-pBAD24 was used as a negative control and E. coli JM109-pBAD24-tet(X4) was used as a positive control. JM109 cultures required supplementation with 0.1% l-arabinose.
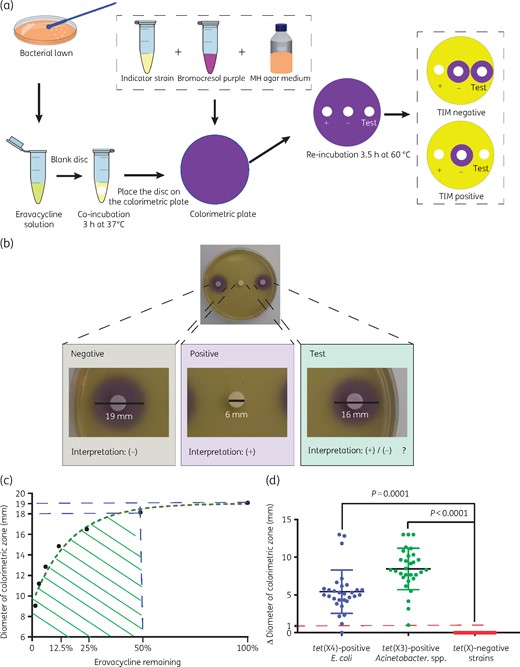
Strategy and results for identification of Tet(X)-producing E. coli and Acinetobacter spp. using the TIM assay. (a) Results are determined by the size of the colorimetric inhibition zone and the total detection time is only 6.5 h. (b) Representative TIM results. The colorimetric inhibition zone diameter of the test strain was 16 mm, 3 mm less than the negative control. The colorimetric inhibition zone of the positive control was completely absent. (c) Determination of positive cut-off values. Eravacycline used at 2.5 mg/L (100%) yielded a colorimetric zone diameter of 19 mm. A colorimetric zone diameter of 18 mm corresponds to a concentration of eravacycline <1.25 mg/L (50%), and this diameter is used as the positive cut-off value. (d) TIM test results for 118 test strains. The changes in diameters of colorimetric inhibition zones of the tet(X)-positive E. coli (30 strains) and Acinetobacter spp. (30) were significant compared with the tet(X)-negative strains (58). This figure appears in colour in the online version of JAC and in black and white in the print version of JAC.
Acid production by the indicator strain resulted in a colour change from purple (∼pH 7) to yellow (pH <5.8). The colorimetric zone diameters were measured with a Vernier calliper. If the test strain carries the plasmid-encoded tet(X3/X4) genes, eravacycline will be degraded, allowing the growth of the susceptible indicator strain, and a yellow colorimetric zone will develop; otherwise the colour will be purple.
Statistical analysis
Descriptive analyses of percentages (together with standard deviation) and prevalence were performed using functions provided in Excel 2010 (Microsoft Software). One-way analysis of variance was performed with GraphPad Prism 7 (GraphPad Software).
Results and discussion
Antibiotic susceptibility testing
The tigecycline MICs of the 118 test strains were determined and ranged from 0.0 to 64 mg/L. Of the 30 tet(X4)-positive E. coli strains 28 (93.3%) were tigecycline resistant, and the remaining two were tigecycline intermediate. Similarly, 30/30 of the tet(X3)-positive Acinetobacter spp. were tigecycline resistant. In addition, the 29 E. coli strains that carried other tetracycline resistance genes were all susceptible to tigecycline (Table 1).
The tetracycline inactivation method
The initial tests of the TIM assay using the positive and negative E. coli control strains indicated that after 3 h of incubation at 37°C with the antibiotic disc on colorimetric plates, colorimetric zones started to form and became clearly visible by 3.5 h (Figure 1b). The colorimetric zone began to blur at 8 h and the colorimetric zones in the positive control disappeared at ∼18 h. Thus, the entire TIM assay can be completed within 6.5 h (3 h incubation at 37°C with test strain in a tube and 3.5 h induction on a colorimetric plate at 60°C).
The colorimetric zone diameters for eravacycline diffusion ranged from 23 to 9 mm with the addition of discs from 0.039 to 10 mg/L. Within this eravacycline range, the diameters of colorimetric zones (X) were linearly related to the natural log of eravacycline concentration (Y) (R2 = 0.9943) (Figure 1c). When the eravacycline concentration was 2.5 mg/L, the diameter of the colorimetric zone was 19 mm and at 1.25 mg/L it was ≤18 mm. Therefore, the positive cut-off value was determined as 18 mm. If the zone diameter was ≤18 mm, the test strain was considered tet(X3/X4) positive; otherwise the test strain was considered tet(X3/X4) negative.
The positive cut-off value in this study was determined to be 18 mm but this is dependent on B. stearothermophilus inoculum size and eravacycline concentration. In a total of 60 tet(X3/X4)-positive test strains, the colorimetric zones of 2 strains (1 E. coli and 1 Acinetobacter) were reduced by 1 mm compared with the negative control while 57/60 (95%) of the tet(X3/X4)-positive strains showed decreases >3 mm and were obvious to the naked eye.
Susceptibility and specificity tests
In E. coli, 29 (96.7%) out of the 30 tet(X4)-positive strains were TIM positive and the remaining strains, including strains harbouring other tetracycline resistance genes and strains without tet genes, were TIM negative. However, one tet(X4)-positive strain was determined to be TIM negative. The sensitivity and specificity of TIM for detection of E. coli carrying the tet(X4) genes were 96.7% and 100%, respectively. In Acinetobacter spp., all tet(X3)-positive strains (n = 30) were TIM positive while the tet(X)-negative strains were determined to be TIM negative. The susceptibility and specificity of TIM for detection of Acinetobacter spp. carrying the tet(X3) genes was 100% and 100%, respectively (Figure 1d).
B. stearothermophilus is widely used in medical and biotechnology fields as a sterilization standard,10,11 but as far as we know this study is the first use of B. stearothermophilus as an indicator strain to detect high-level bacterial tigecycline resistance. Using B. stearothermophilus as the indicator strain dramatically shortened the incubation time. Compared with the other phenotypic detection methods,12,13 the TIM assay is cost-effective and easy to perform, with excellent sensitivity and specificity.
As an eravacycline degradation assay, TIM can potentially be used to detect additional tetracycline-inactivating enzymes, including other Tet(X) variants; however, the detection performance should be further determined. In addition, clinical samples of blood or urine were not tested, even though this type of assay has been developed for carbapenem hydrolysis.14 Evaluation of the TIM assay to detect clinical samples is worth further study.
Conclusions
Overall, the high sensitivity and specificity of TIM combined with its rapid readout make it a useful tool in clinical microbiology laboratories for the detection of plasmid-mediated high-level tigecycline resistance in E. coli and Acinetobacter spp. The TIM is a rapid, consistent and cost-effective assay that can be adopted on a routine basis in resource-limited regions, and may be a useful tool to detect and monitor the spread of plasmid-mediated high-level tigecycline resistance.
Acknowledgements
We thank Ling Jia and Min-Ge Wang (South China Agricultural University) for collecting samples.
Funding
This work was jointly supported by the National Key Research and Development Program of China (2016YFD0501300), the Program for Innovative Research Team in the University of Ministry of Education of China (IRT_17R39) and the Foundation for Innovation and Strengthening School Project of Guangdong, China (2016KCXTD010).
Transparency declarations
None to declare.