-
PDF
- Split View
-
Views
-
Cite
Cite
Hye-Won Jung, Kyeongmin Kim, M Maidul Islam, Je Chul Lee, Minsang Shin, Role of ppGpp-regulated efflux genes in Acinetobacter baumannii, Journal of Antimicrobial Chemotherapy, Volume 75, Issue 5, May 2020, Pages 1130–1134, https://doi.org/10.1093/jac/dkaa014
- Share Icon Share
Abstract
Treatment of infections caused by Acinetobacter baumannii nosocomial strains has become increasingly problematic owing to their resistance to antibiotics. ppGpp is a secondary messenger involved in growth control and various stress responses in bacteria. The mechanism for inhibition of antibiotic resistance via ppGpp is still unidentified in various pathogenic bacteria including A. baumannii. Here, we investigated the effects of ppGpp on efflux pump (EP)-related genes in A. baumannii.
ppGpp-deficient and -complementary strains were constructed by conjugation and we confirmed (p)ppGpp measurements by thin-layer chromatography. We observed that the ppGpp-deficient strain (ΔA1S_0579) showed abnormal stretching patterns by transmission electron microscopy analysis. The MICs of antimicrobial agents for the WT A. baumannii (ATCC 17978), ppGpp-deficient and complementary strains were determined by the Etest and broth dilution assay methods. The expression levels of EP-related genes were determined by quantitative RT–PCR.
We observed morphological differences between a ppGpp-deficient strain (ΔA1S_0579) and the WT strain. Dramatic reductions of MICs in the ppGpp-deficient strain compared with the WT were observed for gentamicin (2.6-fold), tetracycline (3.9-fold), erythromycin (4-fold) and trimethoprim (>4-fold). Expression of the EP-related genes abeB (2.8-fold), tet(A) (2.3-fold), adeB (10.0-fold), adeI (9.9-fold), adeJ (11.8-fold) and adeK (14.4-fold) was also decreased in the ppGpp-deficient strain.
This study demonstrates that ppGpp regulates EP-related gene expression in A. baumannii, affecting antibiotic susceptibility. To date, treatment for MDR A. baumannii has had no new antimicrobial agents, so the A1S_0579 gene could be a novel therapeutic target for rational drug design by affecting ppGpp production.
Introduction
Acinetobacter baumannii is a Gram-negative bacterium of the class Gammaproteobacteria1 and exhibits high virulence in people with impaired immune systems, especially those who have been hospitalized for >90 days.2 The incidence of pneumonia and bloodstream infections caused by A. baumannii has increased.3
MDR indicates the ability of bacterial pathogens to withstand otherwise lethal doses of structurally diverse drugs. The efflux pumps (EPs) play important roles in MDR as a means of drug extrusion.4 EPs are proteins that use energy to pump antibiotics and other small molecules to the exterior of the cell membrane, allowing bacteria to tolerate elevated antibiotic levels. A. baumannii has six EP superfamilies: the ATP binding cassette (ABC) family, the resistance-nodulation-cell division (RND) superfamily, the major facilitator superfamily (MFS), the multidrug and toxic compound extrusion (MATE) family, the small multidrug resistance (SMR) family and the proteobacterial antimicrobial compound efflux (PACE) family.5,6 MFS EPs Tet(A), Tet(B) and CmlA induce the efflux of tetracycline, minocycline and chloramphenicol, respectively. AdeABC of the RND family is involved in antimicrobial resistance to various agents, including aminoglycosides, β-lactams, chloramphenicol, erythromycin, tetracycline and fluoroquinolones. AdeIJK of the same RND family is also involved in resistance to trimethoprim. The MATE family EP AbeM is also involved in antimicrobial resistance to norfloxacin, ofloxacin, ciprofloxacin and gentamicin.7
ppGpp and pppGpp are universal signalling molecules in bacteria, where they play roles in various stress responses, including tolerance to antibiotics and expression of virulence genes.8 ppGpp activation poses a serious threat to clinical success and the efficacy of antimicrobial therapy.9 Here we investigate the antibiotic susceptibility of an A. baumannii ppGpp-deficient strain (ΔA1S_0579), EP gene expression and the influence of the mutation on common bacterial phenotypes.
Materials and methods
Bacterial strains, plasmids and culture conditions
The bacterial strains, plasmids and primers used in this study are listed in Tables S1, S2 and S3 (available as Supplementary data at JAC Online). Bacterial strains were cultured in LB broth or on LB plates containing 1.5% (w/v) agar at 37°C.
Thin-layer chromatography (TLC)
(p)ppGpp measurements were performed using the TLC procedure described previously.10 After subsequent steps, cellular debris was removed through centrifugation and then 9 μL of supernatant was spotted onto 20 × 20 cm polyethyleneimine cellulose on polyester TLC plates (Sigma–Aldrich, Germany). Radio-spotted images were visualized using a Fuji phosphorimager. Detailed procedures are described in the Supplementary Materials and methods.
Gram staining
Overnight cultures of each A. baumannii strain [WT: ATCC 17978; ΔA1S_0579; and A1S_0579 complementary (CP) strain] were Gram stained and images were obtained with a Nikon Eclipse E600 microscope (Nikon, Tokyo, Japan).
Transmission electron microscopy (TEM) analysis
Bacterial morphology was assessed by TEM.11 Samples were visualized using a transmission electron microscope (Hitachi H-7500, Hitachi, Japan) operating at 120 keV. Detailed procedures are described in the Supplementary Materials and methods.
Antimicrobial susceptibility testing
The MICs of antimicrobial agents were determined by the broth dilution and Etest methods, according to CLSI guidelines (CLSI, 2015).12Escherichia coli ATCC 25922 and Pseudomonas aeruginosa ATCC 27853 served as quality control strains. The MICs of aztreonam, ceftazidime, gentamicin, imipenem, nalidixic acid, tetracycline, tigecycline and trimethoprim were determined using Etest (bioMérieux, France). MICs of cefoxitin, cefotaxime, chloramphenicol, ciprofloxacin, colistin, erythromycin, imipenem, levofloxacin and meropenem were measured using the broth dilution method. Antimicrobial susceptibility tests were performed in triplicate.
Quantitative RT–PCR (qRT–PCR)
Expression of EP-related genes was quantified by qRT–PCR. Total RNA was isolated at the early stationary phase from each bacterial culture using QIAGEN RNeasy Mini kits (QIAGEN, Hilden, Germany) according to the manufacturer’s instructions. Quantification of gene transcripts was performed using an ABI StepOnePlus Real-Time System (Applied Biosystems). Each experiment was performed in triplicate.
Results
Construction of A. baumannii ppGpp-deficient mutant strains and (p)ppGpp production in response to nutrient deprivation
To construct the ppGpp-deficient A. baumannii strain (ΔA1S_0579) and its complementary strain, we performed the markerless gene deletion method using conjugation.13 We then determined whether the ΔA1S_0579 strain produced (p)ppGpp in response to nutrient deprivation.14 To confirm ppGpp production we performed TLC. As shown in Figure 1(a), WT and CP strains accumulated significant amounts of (p)ppGpp in response to nutrient deprivation. In contrast, negligible levels were observed in extracts from the ΔA1S_0579 strain. These results indicated that the product of the A1S_0579 gene is indispensable for (p)ppGpp production in A. baumannii ATCC 17978 in response to nutrient deprivation.
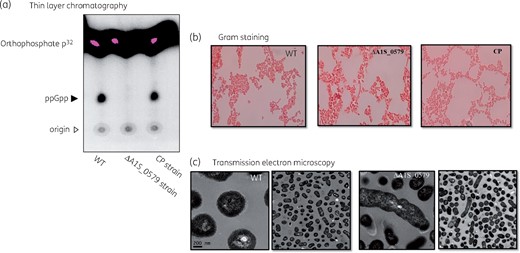
Analysis of ppGpp production by TLC and morphological characteristics of the ΔA1S_0579 strain. (a) Intracellular (p)ppGpp levels detected by TLC analysis. Closed arrowhead indicates ppGpp signal. Open arrowhead indicates origin spot region. (b) Gram staining was applied to cultures of the A. baumannii WT, ΔA1S_0579 and CP strains. The morphology of each strain was observed with an optical microscope. Gram-stained bacteria are red. (c) TEM was used to detect morphological differences between the WT and ΔA1S_0579 strains. Scale bar indicates 200 nm. This figure appears in colour in the online version of JAC and black and white in the print version of JAC.
The effect of ΔA1S_0579 on A. baumannii morphological characteristics
Because (p)ppGpp production is affected in ΔA1S_0579 strains and (p)ppGpp plays a vital role in the stringent response of bacteria under nutritional stress, we investigated the impact of the ΔA1S_0579 mutation on cell morphology. Gram staining (Figure 1b) was performed on the A. baumannii WT, ΔA1S_0579 and CP strains. CP cells were similar to the WT cells but ΔA1S_0579 cells were significantly larger than WT cells. Furthermore, the form changed from coccobacillus to bacillus. We also observed abnormal stretching patterns. These morphological differences are seen more clearly by TEM (Figure 1c). These results imply that the ΔA1S_0579 mutation affects the morphology of A. baumannii.
Antibiotic susceptibility of ΔA1S_0579
To investigate antibiotic susceptibility of the ΔA1S_0579 strain, MICs of different antibiotics were determined in the WT, ΔA1S_0579 and CP strains (Table 1). After we confirmed that control strains were within quality control ranges of the antimicrobial agents according to the CLSI guidelines, the results of susceptibility to antimicrobials were analysed. Most antibiotics generally showed a decrease in MIC for the ΔA1S_0579 strain compared with the WT (the ΔA1S_0579 mutant strain was highly impaired for tolerance compared with WT). Notable differences in MICs between the WT and ΔA1S_0579 strains were observed for gentamicin (2.6-fold), tetracycline (3.9-fold), erythromycin (4-fold) and trimethoprim (>4-fold). These observations suggest that the ppGpp deficiency of the ΔA1S_0579 strain may be involved in various antimicrobial susceptibilities.
Antimicrobial agent . | MIC (mg/L)a . | Fold change (WT/mutant) . | ||
---|---|---|---|---|
WT . | ΔA1S_0579 . | CP . | ||
Aztreonam (ⅰ) | 16 | 12 | 32 | 1.3 |
Ceftazidime (ⅰ) | 3 | 1.5 | 4 | 2 |
Gentamicin (ⅰ) | 0.5 | 0.19 | 1.0 | 2.6 |
Imipenem (ⅰ) | 0.19 | 0.094 | 0.19 | 2 |
Nalidixic acid (ⅰ) | 3 | 1.5 | 3 | 2 |
Tetracycline (ⅰ) | 1.5 | 0.38 | 1.5 | 3.9 |
Tigecycline (ⅰ) | 0.125 | 0.094 | 0.125 | 1.3 |
Trimethoprim (ⅰ) | >32 | 8 | >32 | >4 |
Cefoxitin (ⅱ) | 128 | 64 | 128 | 2 |
Cefotaxime (ⅱ) | 16 | 8 | 16 | 2 |
Chloramphenicol (ⅱ) | 128 | 64 | 128 | 2 |
Ciprofloxacin (ⅱ) | 0.12 | 0.06 | 0.12 | 2 |
Colistin (ⅱ) | 2 | 1 | 4 | 2 |
Erythromycin (ⅱ) | 16 | 4 | 16 | 4 |
Levofloxacin (ⅱ) | 0.125 | 0.062 | 0.125 | 2 |
Meropenem (ⅱ) | 0.5 | 0.25 | 0.5 | 2 |
Antimicrobial agent . | MIC (mg/L)a . | Fold change (WT/mutant) . | ||
---|---|---|---|---|
WT . | ΔA1S_0579 . | CP . | ||
Aztreonam (ⅰ) | 16 | 12 | 32 | 1.3 |
Ceftazidime (ⅰ) | 3 | 1.5 | 4 | 2 |
Gentamicin (ⅰ) | 0.5 | 0.19 | 1.0 | 2.6 |
Imipenem (ⅰ) | 0.19 | 0.094 | 0.19 | 2 |
Nalidixic acid (ⅰ) | 3 | 1.5 | 3 | 2 |
Tetracycline (ⅰ) | 1.5 | 0.38 | 1.5 | 3.9 |
Tigecycline (ⅰ) | 0.125 | 0.094 | 0.125 | 1.3 |
Trimethoprim (ⅰ) | >32 | 8 | >32 | >4 |
Cefoxitin (ⅱ) | 128 | 64 | 128 | 2 |
Cefotaxime (ⅱ) | 16 | 8 | 16 | 2 |
Chloramphenicol (ⅱ) | 128 | 64 | 128 | 2 |
Ciprofloxacin (ⅱ) | 0.12 | 0.06 | 0.12 | 2 |
Colistin (ⅱ) | 2 | 1 | 4 | 2 |
Erythromycin (ⅱ) | 16 | 4 | 16 | 4 |
Levofloxacin (ⅱ) | 0.125 | 0.062 | 0.125 | 2 |
Meropenem (ⅱ) | 0.5 | 0.25 | 0.5 | 2 |
Antimicrobial susceptibility tests were performed by Etest (i) and broth dilution (ii) methods.
aMICs are mean values of triplicate assays.
Antimicrobial agent . | MIC (mg/L)a . | Fold change (WT/mutant) . | ||
---|---|---|---|---|
WT . | ΔA1S_0579 . | CP . | ||
Aztreonam (ⅰ) | 16 | 12 | 32 | 1.3 |
Ceftazidime (ⅰ) | 3 | 1.5 | 4 | 2 |
Gentamicin (ⅰ) | 0.5 | 0.19 | 1.0 | 2.6 |
Imipenem (ⅰ) | 0.19 | 0.094 | 0.19 | 2 |
Nalidixic acid (ⅰ) | 3 | 1.5 | 3 | 2 |
Tetracycline (ⅰ) | 1.5 | 0.38 | 1.5 | 3.9 |
Tigecycline (ⅰ) | 0.125 | 0.094 | 0.125 | 1.3 |
Trimethoprim (ⅰ) | >32 | 8 | >32 | >4 |
Cefoxitin (ⅱ) | 128 | 64 | 128 | 2 |
Cefotaxime (ⅱ) | 16 | 8 | 16 | 2 |
Chloramphenicol (ⅱ) | 128 | 64 | 128 | 2 |
Ciprofloxacin (ⅱ) | 0.12 | 0.06 | 0.12 | 2 |
Colistin (ⅱ) | 2 | 1 | 4 | 2 |
Erythromycin (ⅱ) | 16 | 4 | 16 | 4 |
Levofloxacin (ⅱ) | 0.125 | 0.062 | 0.125 | 2 |
Meropenem (ⅱ) | 0.5 | 0.25 | 0.5 | 2 |
Antimicrobial agent . | MIC (mg/L)a . | Fold change (WT/mutant) . | ||
---|---|---|---|---|
WT . | ΔA1S_0579 . | CP . | ||
Aztreonam (ⅰ) | 16 | 12 | 32 | 1.3 |
Ceftazidime (ⅰ) | 3 | 1.5 | 4 | 2 |
Gentamicin (ⅰ) | 0.5 | 0.19 | 1.0 | 2.6 |
Imipenem (ⅰ) | 0.19 | 0.094 | 0.19 | 2 |
Nalidixic acid (ⅰ) | 3 | 1.5 | 3 | 2 |
Tetracycline (ⅰ) | 1.5 | 0.38 | 1.5 | 3.9 |
Tigecycline (ⅰ) | 0.125 | 0.094 | 0.125 | 1.3 |
Trimethoprim (ⅰ) | >32 | 8 | >32 | >4 |
Cefoxitin (ⅱ) | 128 | 64 | 128 | 2 |
Cefotaxime (ⅱ) | 16 | 8 | 16 | 2 |
Chloramphenicol (ⅱ) | 128 | 64 | 128 | 2 |
Ciprofloxacin (ⅱ) | 0.12 | 0.06 | 0.12 | 2 |
Colistin (ⅱ) | 2 | 1 | 4 | 2 |
Erythromycin (ⅱ) | 16 | 4 | 16 | 4 |
Levofloxacin (ⅱ) | 0.125 | 0.062 | 0.125 | 2 |
Meropenem (ⅱ) | 0.5 | 0.25 | 0.5 | 2 |
Antimicrobial susceptibility tests were performed by Etest (i) and broth dilution (ii) methods.
aMICs are mean values of triplicate assays.
EP gene expression in the ΔA1S_0579 strain using qRT–PCR
To confirm that the differences in MICs between the WT and ΔA1S_0579 strains were due to EP activity, expression levels of EP genes were verified using qRT–PCR (Figure 2). Expression of the trimethoprim resistance-related EP gene adeB was reduced (10.0-fold) in the ΔA1S_0579 strain compared with the WT, as was the expression of the AdeIJK multidrug EP genes adeI (9.9-fold reduction), adeJ (11.8-fold reduction) and adeK (14.4-fold reduction). Expression of the gentamicin and tetracycline resistance-related EP genes abeB and tet(A) was also decreased in the ΔA1S_0579 strain (2.8-fold and 2.3-fold reduction, respectively). These results indicate a relationship such that the ΔA1S_0579 mutation affects antibiotic susceptibility by controlling the expression of EPs, likely through ppGpp deficiency.
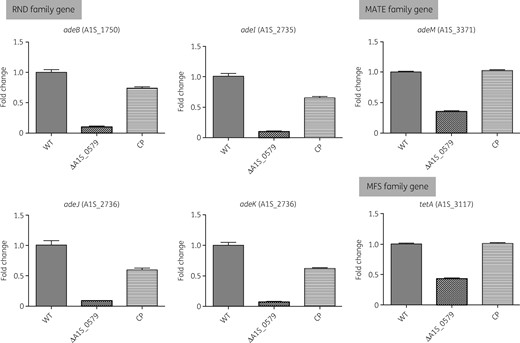
Effect of ΔA1S_0579 mutation on expression of EP genes using qRT–PCR. The A. baumannii WT, ΔA1S_0579 and CP strains were cultured to early stationary phase. Gene expression levels of EPs (RND, MATE and MFS families) were quantified by qRT–PCR.
Discussion
In this study, we focused on the antibiotic susceptibility of A. baumannii and whether ppGpp regulates this feature. We constructed a ppGpp-deficient strain to investigate the role of ppGpp production in susceptibility to different antimicrobials. To confirm mutant strains, PCR experiments were performed (Figure S1). Differences in growth rate between the WT, ΔA1S_0579 and CP strains were insignificant by OD600 and there was no significant difference between the WT and ΔA1S_0579 strains by cfu counting (Figure S2). Intracellular levels of (p)ppGpp are regulated by two RelA/SpoT homologues (RSHs) in many Gram-negative bacteria.15 RelA can only synthesize ppGpp in response to amino acid deficiency by sensing uncharged transfer RNAs at the A site of a ribosome.16 According to the Biocyc database collection (https://biocyc.org), the A1S_0579 product is predicted to have such bifunctional features (Figure S3). This implication of ppGpp biosynthesis was confirmed through TLC (Figure 1). The signalling molecule ppGpp acts through various pathways and enables diverse phenotypes in bacteria. The results of Gram staining and TEM analysis (Figure 1b and c) showed that ΔA1S_0579 was morphologically larger and longer than WT cells. By studying previous research that used other pathogenic strains, we identified that the morphology of the ΔA1S_0579 strain had changed in a similar way. In general, the size of most bacterial strains increases in conditions where ppGpp is depleted and decreases where it is elevated.17,18 The reason for this phenomenon is owing to ppGpp being a pleiotropic repressor of the biosynthetic pathway and a major determinant of cellular shape and size, resulting in an inverse relationship between bacterial morphology and accumulation of ppGpp.19 Our results are consistent with this phenomenon. In most antibiotic-resistant strains, ppGpp production increases antibiotic resistance and ppGpp-depleted strains are more susceptible.8,20 Our MIC results for 14 antibiotics also showed an increase in susceptibility of at least 2-fold in the ΔA1S_0579 strain compared with the susceptibility of the WT strain (Table 1). Furthermore, we focused on EPs as a means of affecting antibiotic susceptibility through ppGpp levels. We examined the expression of RND, MATE and MFS family genes, which are important EPs in A. baumannii. Expression levels of these EP genes were decreased due to the lack of the A1S_0579 gene (Figure 2). To the best of our knowledge, this study is the first to demonstrate a connection between ppGpp and antibiotic susceptibility in A. baumannii, a prevalent bacterium that is resistant to almost all antibiotics. This MDR limits the deployment of effective antibiotics for infection treatment, indicating an urgent need for new drug development. Our research will contribute to the development of drugs that target ppGpp production. Further research will be needed to examine the virulence of ppGpp-deficient strains in vivo and to develop antimicrobial peptides targeting ppGpp production.
Funding
This study was supported by a grant from the National Research Foundation of Korea (grant 2016R1D1A1B01008960 to M.S.).
Transparency declarations
None to declare.
References
Author notes
Hye-Won Jung and Kyeongmin Kim contributed equally to this study.
- gentamicin sulfate (usp)
- erythromycin
- gene expression
- chromatography, thin layer
- genes
- gentamicins
- guanosine tetraphosphate
- reverse transcriptase polymerase chain reaction
- trimethoprim
- bacteria
- tetracycline
- antimicrobials
- acinetobacter baumannii
- antimicrobial susceptibility
- microscopes, transmission electron
- malnutrition-inflammation-cachexia syndrome