-
PDF
- Split View
-
Views
-
Cite
Cite
Eun Seon Chung, Kwan Soo Ko, Eradication of persister cells of Acinetobacter baumannii through combination of colistin and amikacin antibiotics, Journal of Antimicrobial Chemotherapy, Volume 74, Issue 5, May 2019, Pages 1277–1283, https://doi.org/10.1093/jac/dkz034
- Share Icon Share
Abstract
Persister cells following antibiotic exposure may cause failure of antibiotic treatment. The synergistic effects of antibiotic combinations with respect to eliminating persister cells were investigated based on their characteristics.
For Acinetobacter baumannii clinical isolates, persister assays were performed using colistin, amikacin, imipenem and ciprofloxacin in various ways, including exposure to antibiotics in combination and sequentially. Persister phenotypes were observed through analysis of ATP concentration, membrane potential and transmission electron microscopy.
Each A. baumannii isolate showed a specific survival rate of persister cells against each antibiotic. The persister cells were eradicated effectively by exposure to the combination of colistin and amikacin, especially in the sequential order of colistin then amikacin. While the persister cells were not identified after 6 h when exposed to the antibiotics in the order colistin then amikacin, they remained at 0.016% when antibiotic exposure was done in the order amikacin then colistin. Although membrane potential was low in both colistin and amikacin persisters, depletion of the intracellular ATP concentration was only observed in colistin persisters. In addition, transmission electron microscopy analysis showed that colistin persisters have a unique morphology with a rough and rippled membrane and many outer membrane vesicles. Empty pore-like structures surrounded by cracks were also observed.
In A. baumannii, the combination of colistin and amikacin was most effective for eradication of persister cells, probably due to different mechanisms of persister cell formation between antibiotics. It was also identified that the sequential order of colistin followed by amikacin was important to eradicate the persister cells.
Introduction
Acinetobacter baumannii is a major nosocomial pathogen, causing catheter-related infection, pneumonia, wound infection, empyema or meningitis.1,2,A. baumannii infections are often difficult to treat due to the pathogen’s multidrug resistance. In addition to antibiotic resistance, it has been reported that strains phenotypically susceptible to antibiotics are not completely removed by high doses of antibiotics.3
Persisters are bacterial cells that survive in high concentrations of antibiotics despite their antibiotic susceptibility, and they have been discovered for many bacterial pathogens.4 It is known that they use a permeability barrier to prevent penetration of most chemical compounds from the outside environment, staying in a dormant state.5,6 It has been suggested that persister cells might be one reason for antibiotic treatment failure and might contribute to the evolution of antibiotic resistance.7 Persister cell formation has been investigated in relation to aminoglycosides, cephalosporins and fluoroquinolones. Polymyxins (polymyxin B and colistin) are regarded as last-resort antibiotics to treat MDR Gram-negative pathogens.3,8 High persister formation rates against colistin may underlie treatment failure,8 but the feature of persister formation against colistin has rarely been studied.9
Several strategies to eliminate persisters have been suggested, including killing persisters in the dormant state, activating persisters and treating with traditional antibiotics, and preventing the formation of persisters.10 Treatment using a combination of antibiotics has also been proposed as a way to remove persisters. In particular, the combination of polymyxin B and meropenem was recently reported to eliminate persister cells of A. baumannii, especially at a high dose.11
In this study, we investigated variations in the rate of A. baumannii persister cell formation against antibiotics, including colistin. We found that specific combinations of antibiotics were effective at removing A. baumannii persister cells. It was also shown that their effectiveness may be due to different mechanisms of persister cell formation between antibiotics.
Materials and methods
Bacterial strains
Twenty A. baumannii strains, which were isolated from patients’ blood in Chonnam National University Hospital during 2006–10, were included in this study (Table S1, available as Supplementary data at JAC Online). The species identification was performed using rpoB gene sequencing. Bacteria were routinely grown in LB broth or agar at 37°C.
Antimicrobial susceptibility testing
In vitro antimicrobial susceptibility testing was performed by a broth microdilution method according to the CLSI guidelines.12 Four antibiotic agents were tested in this study: colistin, amikacin, imipenem and ciprofloxacin. Based on their MICs, susceptibility was defined according to CLSI breakpoints.12Escherichia coli ATCC 25922 and Pseudomonas aeruginosa ATCC 27853 were employed as control strains. All tests were repeated with three independent cultures and each was tested in duplicate.
Persister assay
Persister assay was performed as described previously,13 with minor modifications. Briefly, cells were grown to exponential phase (OD600=0.5) and 1 mL of the culture was exposed to antibiotics (5×MIC) at 37°C. After incubation with shaking at 185 rpm for 3.5 h, samples were washed twice with PBS and spread on LB agar by serial dilution. The cfu for surviving persister populations were determined [limit of quantification (LOQ)=102.3]. Several incubation times were evaluated, and 3.5 h was chosen as being optimal. To confirm whether the surviving colonies were persister cells, two colonies from each sample were re-inoculated into fresh LB broth and in vitro antimicrobial susceptibility testing was performed to ensure the MIC value had not changed.
The persister assays were carried out in five ways (Figure 1a–e). The first was a simple and repeated persister assay (Figure 1a). Estimates of the persister formation rate within the simple persister assay experiments were repeated three times for each antibiotic to ensure consistency. Next, we performed serial persister assays (Figure 1b and c). The surviving persister cells in the persister assay were regrown and the regrown cells were subjected to the second persister assay with the same antibiotic (Figure 1b) or a different antibiotic (Figure 1c). For regrowth, a single colony in the first persister assay was selected, re-inoculated into fresh LB broth and incubated overnight. Subsequently, the culture was regrown in 1:100 to exponential phase (OD600=0.5), followed by a second antibiotic exposure for the serial persister assay.
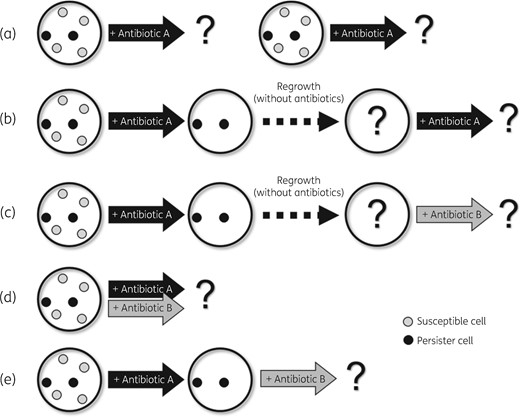
Five persister assay methods to investigate survival rates against antibiotics. (a) A simple and repeated persister assay. Independent experiments to measure the survival rate against four antibiotics were performed repeatedly for each isolate. (b) The surviving persister cells in the persister assay experiment were regrown and the regrown cells were subjected to the second persister assay with the same antibiotic. (c) In this experiment, the regrown cells after the persister assay were treated with a different antibiotic from that used in the first persister assay experiment. (d) Two antibiotics were applied at the same time and the survival rate was evaluated. (e) In this experiment, the surviving persister cells in the persister assay were not regrown. Instead, the second antibiotic was applied 3.5 h after the first antibiotic was applied. Specifically, the second antibiotic was applied directly to the persister cells against the first antibiotic.
To determine the effect of antibiotic combination to eradicate the persister cells, 1 mL of the culture was exposed to two antibiotics (5×MIC each) simultaneously, which was grown to the exponential phase (OD600=0.5) (Figure 1d). The cfu for persister cell survival were determined as follows; after incubation with shaking at 185 rpm for 3.5 h, the cultures were washed twice with PBS and were spread on LB agar by serial dilution. In addition to antibiotic combination, serial exposure to two antibiotics (amikacin and colistin) was also performed to explore whether the order of antibiotic exposure would significantly affect the removal of the persister cells (Figure 1e). After 3.5 h of exposure to one antibiotic, the surviving persister cells were washed twice with PBS and the other antibiotic was applied directly to the persister cells without the process of regrowth. The persister formation rate was evaluated as cfu on the LB plate as in other methods.
Membrane potential analysis
Membrane potential was measured using the BacLight Bacterial Membrane Potential Kit (Molecular Probes, Invitrogen, Carlsbad, CA, USA) and the xMark Microplate Absorbance Spectrophotometer (Bio-Rad, Irvine, CA, USA) according to the manufacturer’s protocol.12 For the assay, overnight cultures were diluted 1:100 in fresh LB medium and grown to the exponential phase (OD600=0.5). As for the persister cells, 1 mL aliquots of cultures grown to the exponential phase were exposed to antibiotic (5×MIC). After 3.5 h of antibiotic exposure, samples were washed twice with PBS. Each bacterial culture was diluted to ∼1 × 106 cfu/mL in filtered PBS. Three aliquots of 1 mL each of the bacterial suspension were prepared for the test sample, depolarized control and unstained control. A 10 μL aliquot of 500 μM CCCP was added to the depolarized control sample. Subsequently, 10 μL of 3 mM DiOC2(3) was added to all tubes, except the unstained control, and incubated for 10 min at 24°C. The ratio of the red (excitation = 530 nm/emission = 590 nm) and green (excitation = 485 nm/emission = 528 nm) fluorescence intensities was used as an indicator of the membrane potential. Data were analysed using the Microplate Manager Software 6.0. The experiments were repeated with three independent cultures and each was tested in triplicate.
ATP concentration analysis
The intracellular ATP concentration was measured with the BacTiter-Glo Assay kit (Promega). Briefly, the overnight culture was diluted 1:100 in fresh LB broth and grown to the exponential phase (OD600=0.5). The persister cells against colistin and amikacin were obtained through the persister assay as previously described. Prior to the experiment, BacTiter-Glo Reagent was formed by transferring the BacTiter-Glo Buffer into the amber bottle containing BacTiter-Glo Substrate to reconstitute the lyophilized enzyme–substrate mixture. Both reagent and cell culture medium were added (100 μL) in each well of a 96-well white plate. After mixing the plate contents on an orbital shaker for 60 s, the luminescence was recorded by a microplate luminometer (Centro LB 960, Berthold Tech., Germany). The experiments were repeated with three independent cultures and each was tested in duplicate.
Transmission electron microscopy
Approximately 109 cfu/mL of surviving persister cells were gathered and used for transmission electron microscopy to investigate phenotypic changes in persister cells. The samples were fixed with 1 mL of 4% paraformaldehyde and 1.5% glutaraldehyde, and incubated overnight at 4°C. Subsequently, the sample was dehydrated through an acetone or ethanol series and passed through a transition solvent. It was also infiltrated and embedded in a liquid resin. After the embedding stage, the sample block was thin sectioned. It was cut into semi-thin sections (1 μm) with a glass knife and stained with Toluidine Blue to select a small area for ultra-thin sectioning. Ultra-thin sections were made at 50–70 nm, using a diamond knife, and placed on a metal grid. The grids were stained with lead uranyl acetate before observation in the HT7800 transmission electron microscope (Hitachi, Tokyo, Japan). Cell membranes of the WT and persister cells, which were antibiotic survivors (amikacin, colistin, imipenem and ciprofloxacin), were detected using transmission electron microscopy.
Statistical analysis
The data are presented as the mean±SD. Pairwise comparison was performed with a one-way analysis of variance (ANOVA) using Prism version 5.01 software for Windows (GraphPad Software, San Diego, CA, USA). P values <0.05 were considered statistically significant.
Results
Persister formation rates against four antibiotics
All 20 A. baumannii clinical isolates tested in this study were susceptible to four antibiotics (colistin, amikacin, imipenem and ciprofloxacin) (Table S1). Simple persister assays showed variable persister cell formation rates upon exposure to all four antibiotics, especially colistin (Figure 2 and Figure S1). In addition, the survival rates, namely the persister formation rates, even in the same isolate, varied among the different types of antibiotic. For example, isolate 07AC-032 showed the lowest persister formation rate against amikacin (2.5 × 10−2), but not against other antibiotics. In addition, the variation of persister formation rates in 20 A. baumannii isolates was higher with colistin exposure (Figure 2). These results may imply that the persister formation mechanism could vary for different types of antibiotic.
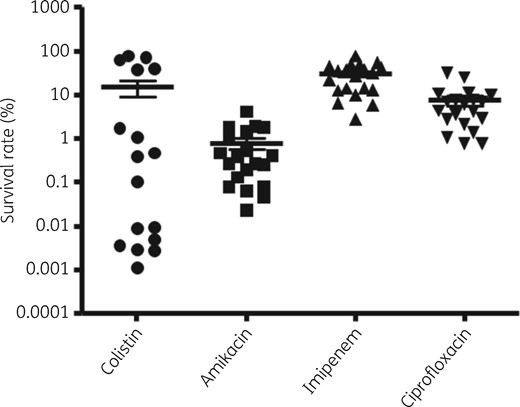
Comparison of persister cell formation rates of four antibiotics against 20 A. baumannii clinical isolates.
For the repeated persister assays, we randomly selected two isolates for each antibiotic (Figure 1a and b). There were no significant differences among the isolates in persister formation rates in three independent persister assays (Figure 1a and Figure S2a). When the persister assays were performed with the same antibiotic, the persister formation rates were also maintained after regrowth (Figure 1b and Figure S2b). Thus, the persister formation rate is probably a consistent characteristic in the isolate for each antibiotic.
The consistency of the persister formation rate was also shown in serial persister assays with different antibiotics (Figure 1c). When the persister assays were performed with a different antibiotic from the first antibiotic after regrowth of four A. baumannii isolates, the persister formation rate was similar to that of the second antibiotic (Figure S3). When colistin or amikacin was applied in the first assay and different antibiotics were applied to the regrown cells after the first assay, the persister formation rates were different from those of the antibiotic in the first assay (Figure S3a and b, respectively). Instead, they were more similar to those of the second antibiotic in the independent persister assays (Figure S3c). Regardless of the antibiotic used in the initial exposure, the persister formation rate after regrowth was dependent on the antibiotic used in the subsequent exposure.
Effect of antibiotic combination
The effect of exposure to antibiotics in combination on persister formation was investigated for four A. baumannii isolates (Figure 1d). Among the antibiotic combinations using colistin, amikacin, imipenem and ciprofloxacin, exposure to colistin and amikacin in combination was the most effective at eradicating the persister cells (Figure 3a). In four A. baumannii isolates, no persister cells were observed when colistin and amikacin were applied simultaneously. In addition, the antibiotic combinations against all four isolates based on colistin (colistin+amikacin, colistin+imipenem and colistin+ciprofloxacin) were more effective than the other combinations (Figure 3a). Therefore, the antibiotic combinations based on colistin might be more effective at removing the A. baumannii persister cells.
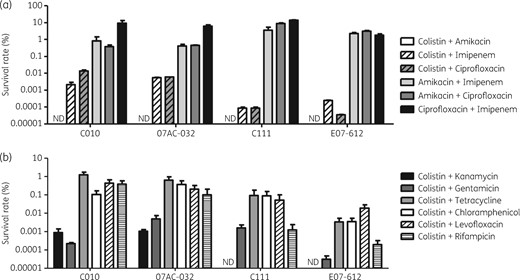
Survival rates in persister assays with antibiotic combinations as in Figure 1(d and e). (a) Persister cell formation rates in antibiotic combinations using colistin, amikacin, imipenem and ciprofloxacin. (b) Persister cell formation rates in antibiotic combinations using colistin and other antibiotics. ND, not detected.
Thus, we performed the persister assays using other antibiotic combinations based on colistin (Figure 3b). As a result, the combinations of colistin and an aminoglycoside (kanamycin and gentamicin) showed a greater synergistic effect with respect to lowering the persister formation rates than other combinations. Thus, it could be concluded that the combination of colistin and aminoglycosides was the most synergistic with respect to removing A. baumannii persister cells.
We treated the A. baumannii isolate C010 with colistin and amikacin subsequently, without the process of regrowth (Figure 1e). As a result, the order of the antibiotic exposure affected the persister formation rate. When colistin was applied first, followed by amikacin, to the colistin-persister cells, persister cells were not identified after 6 h (Figure 4). However, the persister cells remained at 0.016% when antibiotic exposure was performed in the order of amikacin and then colistin.
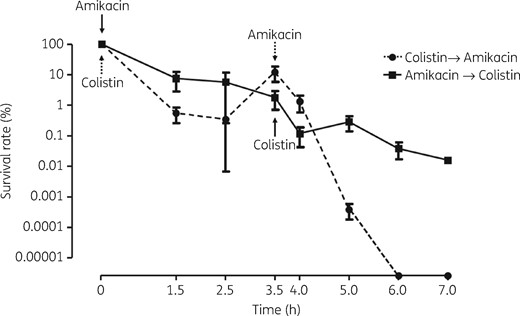
Survival rates when two antibiotics (colistin and amikacin) were applied in alternate order without regrowth. The survival rates were evaluated over time for four isolates of A. baumannii C010. The surviving cells were susceptible to two antibiotics, indicating that they are persister cells.
Membrane potential and ATP concentration
We performed membrane potential and ATP concentration analyses to determine whether the change in persister cells was different according to different antibiotic exposures. Membrane potential was compared between exponential-phase cells before antibiotic treatment, i.e. original WT cells, and persister cells against colistin and amikacin. Lower membrane potential was observed in both colistin and amikacin persisters, compared with the WT (Figure 5a). The level of membrane potential in persister cells was similar to that when CCCP, an inhibitor of the proton motive force, was applied to WT and persister cells.
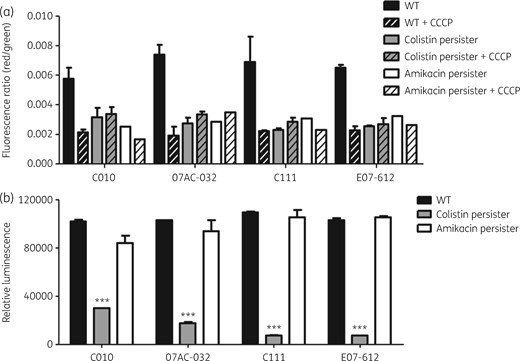
Membrane potential and ATP concentration. (a) Membrane potential in four A. baumannii isolates (C010, 07AC-032, C111 and E07-612) and their colistin or amikacin persisters. Independent experiments were repeated three times and each experiment was performed in duplicate. CCCP was used as the negative control. (b) ATP concentration inside the cells in four A. baumannii isolates (C010, 07AC-032, C111 and E07-612) and their colistin or amikacin persisters. Three independent assays were repeated, and each was performed in triplicate. ***P < 0.0001.
However, the ATP concentration was significantly lower inside the colistin-persister cells than inside WT cells for all four A. baumannii isolates (Figure 5b). In contrast, the ATP concentration inside persister cells against amikacin was not different from that inside WT cells.
Morphological changes in persister cells
Morphological changes in persister cells were investigated using A. baumannii isolate C010 and transmission electron microscopy analysis. The persister cells against amikacin showed no significant morphological change compared with the WT (Figure 6a and c). However, the persister cells against colistin showed rough membrane surfaces with outer membrane vesicles (OMVs) (Figure 6d). The cell membrane was ripped out and leaked cytoplasm could be observed. In addition, empty pore-like structures were detected, with variable sizes, which were surrounded by cracks. However, regrown cells of colistin persisters restored the WT phenotype, as in regrown cells of amikacin persisters (Figure 6c and d). The WT cells treated with CCCP also showed no phenotypic difference compared with untreated WT cells (Figure 6b).
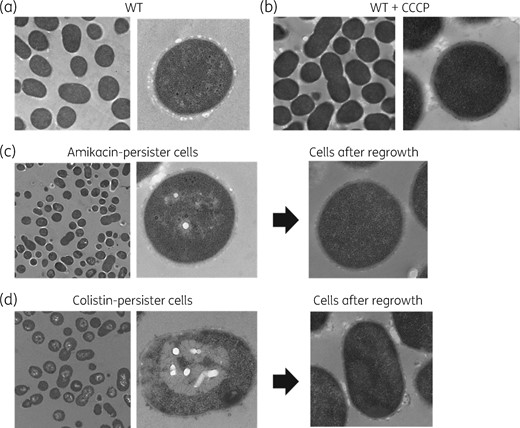
Cell morphology of A. baumannii isolate C010 using transmission electron microscopy. (a) Cells of a susceptible WT isolate. (b) Cells of a susceptible WT isolate when grown with CCCP. (c) Persister cells against amikacin and their regrown cells. (d) Persister cells against colistin and their regrown cells.
Discussion
High persister formation rates against aminoglycosides and heterogeneous persister cell formation against colistin have been reported in A. baumannii.3,8,14 Persister cells may be one cause of treatment failure with the potential to evolve into a resistant population;5,8,15 several strategies to prevent or overcome persister cells have been proposed, including novel antibiotic compounds.16,17 Although many in vitro and in vivo studies based on antibiotic combinations have been reported to find effective treatment options against A. baumannii, only a few have been performed on antibiotic combinations against persister cells.9 The effectiveness of antibiotic combinations may be different for removing persister cells and susceptible cells. For example, it was reported that the combination of polymyxin B and meropenem effectively removed the A. baumannii persister cells.11 In this study, we show the effectiveness of the colistin and amikacin antibiotic combination in eradicating persister cells. In addition, the order of antibiotic exposure may be relevant, which is due to different persister cell formation mechanisms between antibiotics.
The persister formation rate was the consistent feature of each A. baumannii isolate against each antibiotic. The persister formation rates were similar in independently repeated persister assays and serial persister assays using the same and different antibiotics after regrowth. When a different antibiotic was applied to the regrown cells from persister cells, the persister cell formation rate was determined by which antibiotic was applied last. This may imply that persister formation is an intrinsic feature with respect to isolates and antibiotics, and the persister formation mechanisms may be different according to the antibiotic.
Due to different mechanisms of persister cell formation between antibiotics, it is inferred that antibiotic combinations should be synergistic with respect to removing persister cells. We found that the antibiotic combinations based on colistin showed a synergistic effect; in particular, the combination of colistin and an aminoglycoside (amikacin, kanamycin and gentamicin) would be the most effective at eradicating persister cells. In addition, the order of antibiotic exposure affected the degree of eradication of persister cells. It has been suggested that targeting bacterial membranes would be an effective approach to eradicate persister cells.9 Colistin is known to interact with the bacterial outer membrane and solubilize the membrane.18 Therefore, its action on the cell membrane may induce increased uptake of other antibiotics.
Although the molecular mechanism to form persister cells against colistin is unknown, the partial disruption of the cell membrane by colistin could be associated with persister cell formation. Lack of ATP inside the persister cells against colistin may also be due to leakiness of the cell membrane, which is shown in the cell morphology (transmission electron microscopy analysis). The phenotype of several holes surrounded by a crack would be the result of the imbalance of ion distribution inside and outside of the cellular membrane.19 Differences underlying the mechanisms and physiological changes of persister cell formation with different antibiotics may be the reason why combination treatment with amikacin and colistin showed an excellent effect on eradication of persister cells.
Additional treatment of impaired colistin-persister cells with other antibiotics may maximize the effectiveness of eradicating the persister cells. Since aminoglycosides, such as amikacin, inhibit protein synthesis through irreversible binding to the ribosome, there would be no resources for regrowth after awakening from the dormant state.18 Thus, the combination of colistin and amikacin may show the greatest synergistic effect. However, treatment with amikacin prior to colistin was not effective at removing the persister cells. Additional application of colistin to amikacin persisters may allow colistin to act as an independent antibiotic, and different types of persister cells might survive instead of killing amikacin persisters. As such, other combinations not based on colistin could show synergistic effects with respect to killing the persister cells, probably due to the similar mechanism of persister cell formation.
In this study, we showed that combination of colistin and amikacin antibiotics would be effective at eradicating persister cells. In addition, the killing effect on persister cells was different with respect to the order of antibiotic treatment. It may be because the mechanism of persister cell formation by colistin is different from that by other antibiotics. It is expected that the combination regimen of colistin and amikacin would help in treating serious infectious diseases without any relapse of the disease caused by the surviving persister cells against antibiotics. These in vitro microbiological results from our studies may have important clinical implications.
Acknowledgements
We are grateful to Professor Sook-In Jung (Chonnam National University Hospital, Korea) for kindly providing A. baumannii isolates used in this study.
Funding
This research was supported by the Basic Science Research Program through the National Research Foundation of Korea (NRF), funded by the Ministry of Science and ICT (grant number NRF-2016R1A2A2A05005075). E. S. C. was partly supported by the NRF Grant funded by the Korean Government (NRF-2015-Fostering Core Leaders of the Future Basic Science Program/Global Ph.D. Fellowship Program).
Transparency declarations
None to declare.
References
Clinical and Laboratory Standards Institute.