-
PDF
- Split View
-
Views
-
Cite
Cite
Yue Qu, Katherine Locock, Jiyoti Verma-Gaur, Iain D. Hay, Laurence Meagher, Ana Traven, Searching for new strategies against polymicrobial biofilm infections: guanylated polymethacrylates kill mixed fungal/bacterial biofilms, Journal of Antimicrobial Chemotherapy, Volume 71, Issue 2, February 2016, Pages 413–421, https://doi.org/10.1093/jac/dkv334
- Share Icon Share
Abstract
Biofilm-related human infections have high mortality rates due to drug resistance. Cohabitation of diverse microbes in polymicrobial biofilms is common and these infections present additional challenges for treatment compared with monomicrobial biofilms. Here, we address this therapeutic gap by assessing the potential of a new class of antimicrobial agents, guanylated polymethacrylates, in the treatment of polymicrobial biofilms built by two prominent human pathogens, the fungus Candida albicans and the bacterium Staphylococcus aureus.
We used imaging and quantitative methods to test the antibiofilm efficacy of guanylated polymethacrylates, a new class of drugs that structurally mimic antimicrobial peptides. We further compared guanylated polymethacrylates with first-line antistaphylococcal and anti-Candida agents used as combinatorial therapy against polymicrobial biofilms.
Guanylated polymethacrylates were highly effective as a sole agent, killing both C. albicans and S. aureus when applied to established polymicrobial biofilms. Furthermore, they outperformed multiple combinations of current antimicrobial drugs, with one of the tested compounds killing 99.98% of S. aureus and 82.2% of C. albicans at a concentration of 128 mg/L. The extracellular biofilm matrix provided protection, increasing the MIC of the polymethacrylates by 2–4-fold when added to planktonic assays. Using the C. albicans bgl2ΔΔ mutant, we implicate matrix polysaccharide β-1,3 glucan in the mechanism of protection. Data for two structurally distinct polymers suggest that this mechanism could be minimized through chemical optimization of the polymer structure. Finally, we demonstrate that a potential application for these polymers is in antimicrobial lock therapy.
Guanylated polymethacrylates are a promising lead for the development of an effective monotherapy against C. albicans/S. aureus polymicrobial biofilms.
Introduction
Microbial biofilms readily form on indwelling medical devices and cause serious disease for which effective therapy is lacking. According to estimates from the CDC and NIH, as many as 65%–80% of infections could be caused by biofilms,1,2 demonstrating the need to develop improved treatment options.
The bacterium Staphylococcus aureus and the fungus Candida albicans are important biofilm-forming pathogens and amongst the most prevalent microorganisms causing healthcare-acquired infections including catheter-related bloodstream infections (CLBSI) with high associated mortality.3–6 In addition to causing single-species disease, these organisms are also implicated in polymicrobial infections. Approximately 20%–40% of cases of candidaemia were reportedly accompanied by bacteraemia, with Staphylococcus species being the predominant accompanying pathogens.7–11
Laboratory studies suggest that polymicrobial biofilm infections present even worse therapeutic problems than their monomicrobial counterparts. For S. aureus and C. albicans, higher microorganism load and increased antimicrobial resistance of polymicrobial biofilms compared with single-species biofilms have been observed.8,10,12–18 For example, when growing in a polymicrobial biofilm with C. albicans, S. aureus becomes more resistant to vancomycin and daptomycin than as a monoculture.13,19 Another bacterial species, Staphylococcus epidermidis, has been shown to protect C. albicans from the action of the antifungal drugs fluconazole and amphotericin B in polymicrobial biofilms.20 Enhanced drug resistance in polymicrobial biofilms has been attributed to components of the extracellular biofilm matrix secreted by fungi and bacteria.13,19–21 For example, C. albicans biofilm matrix polysaccharide β-1,3 glucan can sequester antimicrobial compounds13,19–23 and a fragment of the cell surface mucin Msb2 secreted by C. albicans contributes to daptomycin resistance of S. aureus in polymicrobial biofilms through direct binding to the antibiotic.19 Importantly, enhancement of S. aureus virulence by the copresence of C. albicans has been documented in animal studies15,16,24 (reviewed in Peleg et al.25).
A challenge with the treatment of fungal/bacterial polymicrobial biofilms is the large evolutionary distance between these microorganisms, which means that there are critical biological differences and few clinically relevant compounds kill both fungi and bacteria. We have recently reported a series of new synthetic compounds, the guanylated polymethacrylates, that have high antimicrobial efficiency against planktonic cells of C. albicans and several bacterial species.26,27 Guanylated polymethacrylates are random copolymers of 2-guanidinoethyl methacrylate (2-GEMA), a mimic of the cationic amino acid arginine, and methyl methacrylate (MMA), a mimic of the lipophilic amino acid alanine, and as such are synthetic structural mimics of naturally occurring antimicrobial peptides (AMPs) (Figure 1).26,27 AMPs are promising for the development of antimicrobial therapeutics due to broad-spectrum activity and low toxicity towards human cells.28 Challenges with developing AMPs for clinical use include pharmacokinetic stability of peptides and expensive large-scale production.29 The advantage of guanylated polymethacrylates is that they have been designed to retain the essential amphiphilic structure, cationic-rich nature and low molecular weight of the AMPs, while being cheaper and easier to produce and chemically manipulate.
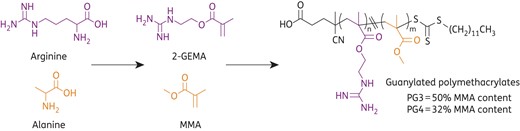
Structures of guanylated polymethacrylates PG3 and PG4. Synthesized from the monomers 2-GEMA, a mimic of the amino acid arginine, and MMA, a mimic of the amino acid alanine.
In this study, we show that guanylated polymethacrylates kill both C. albicans and S. aureus in established polymicrobial biofilms, outperforming multiple combinations of conventional antibacterial and antifungal drugs. Their high activity as monotherapy against fungal/bacterial polymicrobial biofilms is a highly advantageous property. We explore the possibility to further enhance the activity of the polymers by manipulating the extracellular matrix material in biofilms and show their potential in antimicrobial lock therapy.
Materials and methods
Strains and growth conditions
The WT C. albicans strain DAY185 (ura3Δ::λimm434/ura3-Δ::λimm434, ARG4:URA3:arg4::hisG/arg4::hisG his1::hisG::pHIS1/his1::hisG) and S. aureus strain ATCC 25923 were used as model microorganisms for monomicrobial and polymicrobial biofilms.21 Both strains are well-known biofilm producers. The C. albicans bgl2ΔΔ mutant strain is described in Taff et al.23 and forms a biofilm with less β-1,3 glucan in the extracellular matrix. Two S. aureus clinical isolates (A8090 and A5937, both MRSA) and two C. albicans clinical isolates (AYP49 and SC5314) were also included in this study. S. aureus isolates A8090 and A5937 and C. albicans isolate AYP49 were from patients with confirmed bloodstream infections in the Alfred Hospital, Melbourne, Australia.30C. albicans SC5314 is a human clinical isolate that is the reference strain for this species (see the Candida Genome Database; http://www.candidagenome.org/). Strains were stored at −80°C in 15% (v/v) glycerol and streaked onto nutrient agar plates (Oxoid, for S. aureus) or YPD plates (2% peptone, 1% yeast extract, 2% glucose and 80 mg/L uridine, for C. albicans) as working stocks. The working stocks were stored at 4°C (S. aureus) or room temperature (C. albicans) and replaced every 2 weeks.
Antimicrobial agents
Four first-line antibiotics and three antifungals were used in this study: antistaphylococcal agents included oxacillin (β-lactam), vancomycin (glycopeptide), ciprofloxacin (fluoroquinolone) and rifampicin (rifamycin); and anti-Candida agents included fluconazole (azole), amphotericin B (polyene) and caspofungin (echinocandin). All agents except caspofungin were purchased from Sigma–Aldrich, Sydney, Australia. Caspofungin was obtained from Merck. According to CLSI guideline M100-S24,31 stock solutions of vancomycin and oxacillin were prepared in distilled H2O at a concentration of 5120 mg/L. Ciprofloxacin was prepared in acidic H2O and rifampicin was prepared in methanol, to concentrations of 5120 and 640 mg/L, respectively. Stock solution of amphotericin B was prepared in DMSO to 1600 mg/L following CLSI guideline M27-S4.32 Stock solution of fluconazole was prepared in DMSO to 5000 mg/L and caspofungin was prepared in sterile distilled H2O to 125 mg/L, following the manufacturers' instructions. Stock solutions of two guanylated polymethacrylates, designated as PG3 (50% MMA and 50% 2-GEMA content) and PG4 (32% MMA and 68% 2-GEMA), were prepared in distilled H2O as described previously.27 For antimicrobial susceptibility tests for monomicrobial planktonic cultures and biofilms, solvent vehicles were added into the growth controls at levels equivalent to drug-treated samples. For the combinatorial drug treatments, medium-only was used in the no-drug controls. After dilution of the stock solutions of the drugs, the solvents were diluted between 500-fold and 1000-fold, and these very low levels of solvents had no effect on the growth of microorganisms used in our study. We therefore chose medium-only for the non-drug controls for a more direct comparison with the polymethacrylates, which were dissolved in distilled H2O.
Antimicrobial susceptibility tests for planktonic cells
MICs of antibacterial and antifungal agents were determined using the broth microdilution method according to CLSI guidelines M07-A9 (for S. aureus) and M27-A3 (for C. albicans),33,34 but replacing Mueller–Hinton broth (MHB) with RPMI-1640 for antibacterial MIC testing. RPMI-1640 was chosen over MHB because of the poor solubility of two guanylated polymethacrylates at higher concentrations in MHB and to be consistent with media used for biofilm assays. Side-by-side comparison of the MICs of four antistaphylococcal antibiotics with RPMI-1640 or MHB as the growth medium showed no differences (Table S1, available as Supplementary data at JAC Online). The inoculated microplates were incubated at 35°C. The incubation times for S. aureus were 18 h for ciprofloxacin and rifampicin and 24 h for oxacillin, vancomycin, PG3 and PG4. For C. albicans, the incubation times were 24 h for caspofungin, PG3 and PG4 and 48 h for amphotericin B and fluconazole. Bacterial or fungal growth was examined with the aid of a microplate reader (Tecan). The antibacterial MIC was the lowest concentration resulting in complete inhibition of bacterial growth. For antifungal activity of amphotericin B or the polymethacrylates, the MIC was determined as the lowest concentration that prevented any growth. For fluconazole or caspofungin, the MIC was determined as the lowest concentration that inhibited ≥50% of growth.
To obtain comparable data on the efficacy of the antimicrobial agents against planktonic cultures and biofilms, the lowest concentration that inhibited ≥80% of growth of planktonic cultures was also determined and is reported as the MIC80. The MIC80 was determined by measuring the OD600 of drug-treated and drug-free cultures and results were further confirmed by the viable count method.
Antimicrobial susceptibility tests for monomicrobial biofilms
Overnight bacterial and fungal cultures were grown in nutrient broth and YPD, respectively, and diluted into RPMI-1640 to a cell density of 1 × 106 cfu/mL. One hundred microlitre aliquots of diluted microbial suspensions were pipetted into wells of a 96-well flat-bottomed tissue culture-treated polystyrene (TCPS) microplate and incubated for 24 h at 37°C with gentle agitation (75 rpm). After incubation, the suspensions were aspirated and the microwells rinsed with PBS to remove non-adherent cells. Two hundred microlitre aliquots of 2-fold serial dilutions of antimicrobial agents were added into each well. The treatment was consistent with that for planktonic cultures and lasted for 18/24 h for S. aureus biofilms, 24/48 h for C. albicans biofilms and 24 h for polymicrobial biofilms. After washing with PBS, the antimicrobial efficacy against monomicrobial biofilms was assessed by the XTT assay.35,36 The biofilm MIC80 (BMIC80) was determined as the lowest concentration that inhibited ≥80% of growth.
Susceptibility of polymicrobial biofilms
Polymicrobial biofilms were established as described for monomicrobial biofilms, except that both S. aureus and C. albicans at 1 × 106 cfu/mL were added into one microwell as initial inocula. Treatment with antimicrobial combinations at serum achievable concentrations (see Table 1 for agents used and concentrations) or guanylated polymethacrylates at 128 mg/L lasted for 24 h at 37°C. The efficacy of the antimicrobial agents was determined based on the reduction in viable counts (cfu). For this, after treatment, biofilms were dissociated from the surface into PBS by scraping with sterile pipette tips and sonicated in a sonication bath at 42 kHz for 10 min. Cells were transferred into an Eppendorf tube and vortexed for 30 s at maximum speed four times. The cell numbers of fungi and bacteria were determined by plating serial dilutions onto trypticase soy agar (TSA) + amphotericin B (2.5 mg/L) plates (to select for S. aureus) or YPD + vancomycin (2 mg/L) plates (to select for C. albicans). The effectiveness of the antimicrobial treatments was determined by calculating the log reduction of viable cells [log10 (untreated culture viable cell density/treated culture viable cell density)]. In parallel, planktonic polymicrobial cultures of S. aureus (1 × 106 cfu/mL) and C. albicans (1 × 106 cfu/mL) were prepared and treated with the same set of antimicrobial agents and the efficacy determined as above.
. | . | . | Antifungals (mg/L) . | ||||
---|---|---|---|---|---|---|---|
Antibiotics (mg/L) . | fluconazole . | . | amphotericin B . | . | caspofungin . | ||
Oxacillin | 16 | + | 8 | or | 2 | or | 4 |
Vancomycin | 40 | 8 | 2 | 4 | |||
Ciprofloxacin | 4.5 | 8 | 2 | 4 | |||
Rifampicin | 10 | 8 | 2 | 4 |
. | . | . | Antifungals (mg/L) . | ||||
---|---|---|---|---|---|---|---|
Antibiotics (mg/L) . | fluconazole . | . | amphotericin B . | . | caspofungin . | ||
Oxacillin | 16 | + | 8 | or | 2 | or | 4 |
Vancomycin | 40 | 8 | 2 | 4 | |||
Ciprofloxacin | 4.5 | 8 | 2 | 4 | |||
Rifampicin | 10 | 8 | 2 | 4 |
The highest achievable serum concentrations were in accordance with: Versalovic et al.,50 for antibiotics; Brammer et al.,51 for fluconazole; and Root et al.52 (p. 389), for amphotericin B. For caspofungin, a concentration of 4 mg/L was used instead of the highest achievable serum concentration of 12 mg/L, in order to avoid its paradoxical effect.53
. | . | . | Antifungals (mg/L) . | ||||
---|---|---|---|---|---|---|---|
Antibiotics (mg/L) . | fluconazole . | . | amphotericin B . | . | caspofungin . | ||
Oxacillin | 16 | + | 8 | or | 2 | or | 4 |
Vancomycin | 40 | 8 | 2 | 4 | |||
Ciprofloxacin | 4.5 | 8 | 2 | 4 | |||
Rifampicin | 10 | 8 | 2 | 4 |
. | . | . | Antifungals (mg/L) . | ||||
---|---|---|---|---|---|---|---|
Antibiotics (mg/L) . | fluconazole . | . | amphotericin B . | . | caspofungin . | ||
Oxacillin | 16 | + | 8 | or | 2 | or | 4 |
Vancomycin | 40 | 8 | 2 | 4 | |||
Ciprofloxacin | 4.5 | 8 | 2 | 4 | |||
Rifampicin | 10 | 8 | 2 | 4 |
The highest achievable serum concentrations were in accordance with: Versalovic et al.,50 for antibiotics; Brammer et al.,51 for fluconazole; and Root et al.52 (p. 389), for amphotericin B. For caspofungin, a concentration of 4 mg/L was used instead of the highest achievable serum concentration of 12 mg/L, in order to avoid its paradoxical effect.53
Biofilm microscopy
Bacterial, fungal and polymicrobial biofilm cultures were set up on silicon discs pre-coated with adult bovine serum overnight in 24-well microplates. The discs were inoculated with 1 mL of an S. aureus and/or C. albicans suspension in RPMI-1640 (∼106 cfu/mL of each organism), followed by overnight incubation at 37°C and washes with 0.9% saline to remove planktonic microorganisms. Scanning electron microscopy was performed as described previously37 using a Hitachi S570 scanning electron microscope. For confocal laser scanning microscopy (CLSM), after exposure to antimicrobial compounds the biofilms were stained with the BacLight Live/Dead Viability Kit (L7007, Invitrogen, which contains 3.35 μM SYTO-9 and 20 μM propidium iodide) at 37°C for 30 min in the dark. The biofilm was washed twice with saline and viewed using a Leica SP5 inverted microscope with a ×60 oil objective. All samples were sequentially scanned, frame by frame, first at 488 nm and then at 561 nm. Three-dimensional biofilm images were reconstructed with Amira 5.4.1 software as described in Harrison et al.38
Sequestration of guanylated polymethacrylates by the biofilm matrix
Extracellular matrix was isolated from Candida or polymicrobial biofilms grown in 6-well TCPS microplates for 48 h at 37°C, according to Taff et al.23 Biofilm supernatants containing extracellular matrix were plated onto TSA plates to ensure they were free of viable cells. The isolated matrix was incubated with drugs and growth medium for 2 h in 96-well plates, prior to addition of C. albicans/S. aureus cells for planktonic antimicrobial susceptibility testing.36 Results were compared with that of susceptibility testing without biofilm matrix.
In addition, C. albicans biofilms formed by the bgl2ΔΔ mutant or the control WT strain DAY185 were exposed to PG3 or PG4 at sub-BMIC80 (32 mg/L) or drug-free growth medium for 24 h. The survival percentage (biofilm cells remaining) was determined by viable counts, dividing live cell numbers after antimicrobial treatment by those from drug-free cultures. Fluconazole was included as a control.
Testing of the efficacy of guanylated polymethacrylates as catheter lock solutions
A regrowth assay following catheter antimicrobial lock was performed as described previously.39 Biofilms were grown for 24 h in 96-well microplates and then exposed to ethanol or the polymethacrylates for 18 h. Ethanol concentrations ranged from 10% to 80% and PG3 and PG4 were at 64–1024 mg/L. Biofilms were washed three times with saline to remove the antimicrobial agents, followed by adding 200 μL of tryptone soya broth (TSB; Oxoid) and incubating at 37°C for 48 h on a microplate shaker (speed 2) (Titreteck, Flow Laboratories, Germany). Following this, 150 μL was transferred to a round-bottomed microplate and examined visually for turbidity. The minimum biofilm eradication concentration (MBEC) was determined as the lowest concentration of antimicrobial agents that resulted in clear wells.
Statistical analysis
To analyse differences in the biofilm cell survival percentage between WT and bgl2ΔΔ deletion mutant strains, one-way ANOVA tests were performed with Minitab 16 for Windows® using a significance level of 0.05 (P value).
Results
Guanylated polymethacrylates are effective antibiofilm agents for S. aureus and C. albicans
Association of S. aureus with C. albicans into a polymicrobial biofilm has been previously reported.14,19,40 We could confirm that with our laboratory strains and experimental conditions, robust polymicrobial biofilms formed, with S. aureus adhering to C. albicans hyphal cells and some bacterial clumps also evident in the intercellular space of the biofilm scaffold (Supplementary Data).
To test the efficacy of the guanylated polymethacrylates against biofilms, we chose PG3 and PG4 (Figure 1) as these agents showed potent effects against planktonic fungi and bacteria concordant with relatively low in vitro human cell toxicity.26 The BMIC80 of both polymers was 128 mg/L for S. aureus and 64 mg/L for C. albicans (Table 2). Compared with the planktonic MIC80, the BMIC80 was 16-fold higher for S. aureus and 4-fold higher for C. albicans (Table 2). Consistent with numerous published studies, both S. aureus and C. albicans became highly resistant to most first-line antimicrobial agents when grown as monomicrobial biofilms, with only amphotericin B and caspofungin retaining their activities against C. albicans biofilms (Table 2). Therefore, while the antimicrobial activity of the polymers was lower against biofilms than against planktonic cultures, the biofilm-related resistance was much smaller than what occurs in response to several conventional antibiotics. The efficacy of the guanylated polymethacrylates against single-species biofilms was confirmed by CLSM using BacLight Live/Dead staining (Figure 2).
Antimicrobial susceptibility of S. aureus and C. albicans grown as planktonic or biofilm monocultures
. | S. aureus ATCC 25923 . | . | C. albicans DAY185 . | ||||
---|---|---|---|---|---|---|---|
planktonic MIC (mg/L) . | planktonic MIC80 (mg/L) . | BMIC80 (mg/L) . | planktonic MIC (mg/L) . | planktonic MIC80 (mg/L) . | BMIC80 (mg/L) . | ||
PG3 | 8 | 8 | 128 | PG3 | 16 | 16 | 64 |
PG4 | 8 | 8 | 128 | PG4 | 16 | 16 | 64 |
Oxacillin | 0.06 | 0.06 | >1024 | fluconazole | 0.5 | 1 | >1024 |
Vancomycin | 1 | 1 | >1024 | amphotericin B | 1 | 1 | 1 |
Ciprofloxacin | 0.25 | 0.12 | >1024 | caspofungin | 0.12 | 0.12 | 0.12 |
Rifampicin | 0.015 | 0.008 | >128 |
. | S. aureus ATCC 25923 . | . | C. albicans DAY185 . | ||||
---|---|---|---|---|---|---|---|
planktonic MIC (mg/L) . | planktonic MIC80 (mg/L) . | BMIC80 (mg/L) . | planktonic MIC (mg/L) . | planktonic MIC80 (mg/L) . | BMIC80 (mg/L) . | ||
PG3 | 8 | 8 | 128 | PG3 | 16 | 16 | 64 |
PG4 | 8 | 8 | 128 | PG4 | 16 | 16 | 64 |
Oxacillin | 0.06 | 0.06 | >1024 | fluconazole | 0.5 | 1 | >1024 |
Vancomycin | 1 | 1 | >1024 | amphotericin B | 1 | 1 | 1 |
Ciprofloxacin | 0.25 | 0.12 | >1024 | caspofungin | 0.12 | 0.12 | 0.12 |
Rifampicin | 0.015 | 0.008 | >128 |
Antimicrobial susceptibility of S. aureus and C. albicans grown as planktonic or biofilm monocultures
. | S. aureus ATCC 25923 . | . | C. albicans DAY185 . | ||||
---|---|---|---|---|---|---|---|
planktonic MIC (mg/L) . | planktonic MIC80 (mg/L) . | BMIC80 (mg/L) . | planktonic MIC (mg/L) . | planktonic MIC80 (mg/L) . | BMIC80 (mg/L) . | ||
PG3 | 8 | 8 | 128 | PG3 | 16 | 16 | 64 |
PG4 | 8 | 8 | 128 | PG4 | 16 | 16 | 64 |
Oxacillin | 0.06 | 0.06 | >1024 | fluconazole | 0.5 | 1 | >1024 |
Vancomycin | 1 | 1 | >1024 | amphotericin B | 1 | 1 | 1 |
Ciprofloxacin | 0.25 | 0.12 | >1024 | caspofungin | 0.12 | 0.12 | 0.12 |
Rifampicin | 0.015 | 0.008 | >128 |
. | S. aureus ATCC 25923 . | . | C. albicans DAY185 . | ||||
---|---|---|---|---|---|---|---|
planktonic MIC (mg/L) . | planktonic MIC80 (mg/L) . | BMIC80 (mg/L) . | planktonic MIC (mg/L) . | planktonic MIC80 (mg/L) . | BMIC80 (mg/L) . | ||
PG3 | 8 | 8 | 128 | PG3 | 16 | 16 | 64 |
PG4 | 8 | 8 | 128 | PG4 | 16 | 16 | 64 |
Oxacillin | 0.06 | 0.06 | >1024 | fluconazole | 0.5 | 1 | >1024 |
Vancomycin | 1 | 1 | >1024 | amphotericin B | 1 | 1 | 1 |
Ciprofloxacin | 0.25 | 0.12 | >1024 | caspofungin | 0.12 | 0.12 | 0.12 |
Rifampicin | 0.015 | 0.008 | >128 |
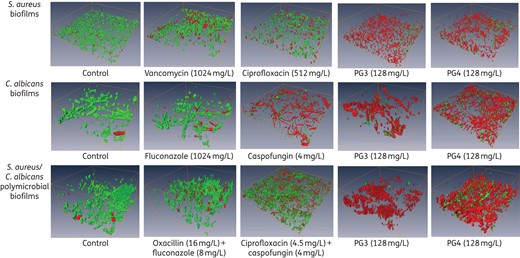
Guanylated polymethacrylates are efficient at killing polymicrobial C. albicans/S. aureus biofilms. Biofilms (24 h) formed by S. aureus and/or C. albicans were exposed to antimicrobial agents for 18 h. Biofilms were stained with the BacLight Live/Dead Viability Kit and imaged by CLSM. CLSM reconstructions show the three-dimensional staining pattern for live cells (SYTO-9, green) and dead cells (propidium iodide, red). The experiments were performed twice and representative images are shown.
Guanylated polymethacrylates kill polymicrobial C. albicans/S. aureus biofilms and outperform combinations of conventional antimicrobial agents
We next assessed the efficacy of PG3 and PG4 against polymicrobial biofilms and polymicrobial planktonic cultures as a comparison, and compared their activity with combinations of conventional antibacterial and antifungal drugs used at the highest achievable serum concentrations (Table 1).
Planktonic C. albicans DAY185/S. aureus ATCC 25923 polymicrobial cultures were susceptible to all 12 antibiotic/antifungal combinations, with ≥99.9% of S. aureus and 85% of C. albicans being eliminated (Figure 3a). In contrast, the antimicrobial activities of the 12 combinations were drastically reduced when challenging their polymicrobial biofilms. The best-performing combination of ciprofloxacin/caspofungin reduced the S. aureus cell count by 99.6% and C. albicans by 66.6% (Figure 3b). The guanylated polymethacrylates had superior activity to conventional drugs. At a concentration of 128 mg/L, PG3 killed 99.98% of S. aureus and 82.2% of C. albicans cells residing in the biofilm, while PG4 killed 94% of S. aureus and 80.3% of C. albicans (Figure 3b). The efficacy of PG3 and PG4 against polymicrobial biofilms was further confirmed by CLSM and dead/live cell staining (Figure 2).
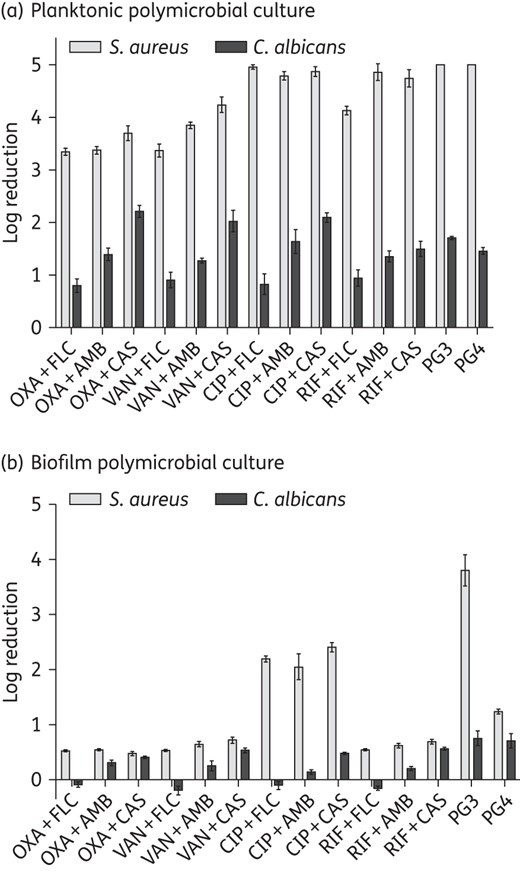
Guanylated polymethacrylates outperform current antimicrobial drug combinations in killing fungal/bacterial polymicrobial biofilms. Shown is the log reduction of viable cells in C. albicans/S. aureus polymicrobial planktonic cultures (a) or biofilms (b) upon antimicrobial exposure. The y-axis (log reduction) shows a 10-fold reduction of the numbers of recoverable cells (1 log reduction = 90% reduction, 2 log reduction = 99% reduction etc.). The experiment was performed with three biological repeats in duplicate; shown are the geometric mean of the data and the standard errors. OXA, oxacillin; VAN, vancomycin; CIP, ciprofloxacin; RIF, rifampicin; FLC, fluconazole; AMB, amphotericin B; CAS, caspofungin.
To generalize our findings, we tested the activity of PG3 and PG4 against planktonic cultures and monomicrobial and polymicrobial biofilms formed by clinical isolates of S. aureus and C. albicans (Table 3 and Figure 4). For comparison, we also determined the susceptibility of these clinical isolates to conventional antimicrobial agents (Table 3). Unlike the laboratory strain that we used, both S. aureus clinical isolates displayed resistance to oxacillin and ciprofloxacin, with MICs of ≥8 and >4 mg/L, respectively (Table 3). The C. albicans clinical isolates were susceptible to conventional antifungal agents (Table 3). PG3 and PG4 were effective in inhibiting planktonic cultures and monomicrobial biofilms of the clinical isolates, with the MIC and BMIC80 equivalent to what we measured for laboratory strains (Table 3; compare with Table 2). Their ability to kill clinical isolates of C. albicans in polymicrobial biofilms was also equivalent to the data for the laboratory strain (Figure 4; compare with Figure 3). The microbicidal activity for S. aureus clinical isolates in polymicrobial biofilms was somewhat reduced compared with the laboratory strain, but nevertheless we observed killing of 80%–95% of cells (Figure 4; compare with Figure 3).
Antimicrobial susceptibility of S. aureus and C. albicans clinical isolates grown as planktonic and biofilm monocultures
. | S. aureus . | . | C. albicans . | ||||||
---|---|---|---|---|---|---|---|---|---|
A8090 . | A5937 . | SC5314 . | AYP49 . | ||||||
MIC (mg/L) . | BMIC80 (mg/L) . | MIC (mg/L) . | BMIC80 (mg/L) . | MIC (mg/L) . | BMIC80 (mg/L) . | MIC (mg/L) . | BMIC80 (mg/L) . | ||
PG3 | 8 | 128 | 8 | 64 | PG3 | 16 | 64 | 8 | 64 |
PG4 | 8 | 64 | 8 | 64 | PG4 | 16 | 64 | 8 | 64 |
Oxacillin | 8 | >1024 | >16 | >1024 | fluconazole | 1 | >1024 | 1 | >1024 |
Vancomycin | 1 | >1024 | 1 | >1024 | amphotericin B | 1 | 1 | 1 | 1 |
Ciprofloxacin | >4 | >1024 | >4 | >1024 | caspofungin | 0.12 | 0.12 | 0.25 | 0.25 |
Rifampicin | 0.004 | >128 | 0.004 | >128 |
. | S. aureus . | . | C. albicans . | ||||||
---|---|---|---|---|---|---|---|---|---|
A8090 . | A5937 . | SC5314 . | AYP49 . | ||||||
MIC (mg/L) . | BMIC80 (mg/L) . | MIC (mg/L) . | BMIC80 (mg/L) . | MIC (mg/L) . | BMIC80 (mg/L) . | MIC (mg/L) . | BMIC80 (mg/L) . | ||
PG3 | 8 | 128 | 8 | 64 | PG3 | 16 | 64 | 8 | 64 |
PG4 | 8 | 64 | 8 | 64 | PG4 | 16 | 64 | 8 | 64 |
Oxacillin | 8 | >1024 | >16 | >1024 | fluconazole | 1 | >1024 | 1 | >1024 |
Vancomycin | 1 | >1024 | 1 | >1024 | amphotericin B | 1 | 1 | 1 | 1 |
Ciprofloxacin | >4 | >1024 | >4 | >1024 | caspofungin | 0.12 | 0.12 | 0.25 | 0.25 |
Rifampicin | 0.004 | >128 | 0.004 | >128 |
Antimicrobial susceptibility of S. aureus and C. albicans clinical isolates grown as planktonic and biofilm monocultures
. | S. aureus . | . | C. albicans . | ||||||
---|---|---|---|---|---|---|---|---|---|
A8090 . | A5937 . | SC5314 . | AYP49 . | ||||||
MIC (mg/L) . | BMIC80 (mg/L) . | MIC (mg/L) . | BMIC80 (mg/L) . | MIC (mg/L) . | BMIC80 (mg/L) . | MIC (mg/L) . | BMIC80 (mg/L) . | ||
PG3 | 8 | 128 | 8 | 64 | PG3 | 16 | 64 | 8 | 64 |
PG4 | 8 | 64 | 8 | 64 | PG4 | 16 | 64 | 8 | 64 |
Oxacillin | 8 | >1024 | >16 | >1024 | fluconazole | 1 | >1024 | 1 | >1024 |
Vancomycin | 1 | >1024 | 1 | >1024 | amphotericin B | 1 | 1 | 1 | 1 |
Ciprofloxacin | >4 | >1024 | >4 | >1024 | caspofungin | 0.12 | 0.12 | 0.25 | 0.25 |
Rifampicin | 0.004 | >128 | 0.004 | >128 |
. | S. aureus . | . | C. albicans . | ||||||
---|---|---|---|---|---|---|---|---|---|
A8090 . | A5937 . | SC5314 . | AYP49 . | ||||||
MIC (mg/L) . | BMIC80 (mg/L) . | MIC (mg/L) . | BMIC80 (mg/L) . | MIC (mg/L) . | BMIC80 (mg/L) . | MIC (mg/L) . | BMIC80 (mg/L) . | ||
PG3 | 8 | 128 | 8 | 64 | PG3 | 16 | 64 | 8 | 64 |
PG4 | 8 | 64 | 8 | 64 | PG4 | 16 | 64 | 8 | 64 |
Oxacillin | 8 | >1024 | >16 | >1024 | fluconazole | 1 | >1024 | 1 | >1024 |
Vancomycin | 1 | >1024 | 1 | >1024 | amphotericin B | 1 | 1 | 1 | 1 |
Ciprofloxacin | >4 | >1024 | >4 | >1024 | caspofungin | 0.12 | 0.12 | 0.25 | 0.25 |
Rifampicin | 0.004 | >128 | 0.004 | >128 |
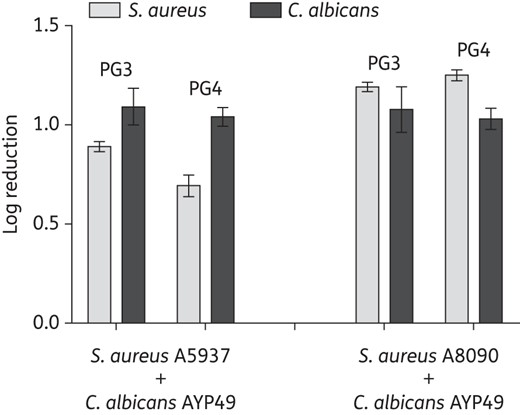
Guanylated polymethacrylates kill polymicrobial biofilms formed by C. albicans and S. aureus clinical isolates. Polymicrobial biofilms formed by C. albicans AYP49 and S. aureus A8090 or S. aureus A5937 were treated overnight with PG3 or PG4 (at 128 mg/L). cfu of the bacteria and fungi were determined in treated samples relative to drug-free growth medium. The y-axis shows log reductions. The experiment was performed with three biological repeats in duplicate; shown are the geometric mean of the data and the standard errors.
Biofilm extracellular matrix reduces the antimicrobial activity of the polymethacrylates
As discussed in the Introduction, a major contributor to the increased antimicrobial resistance of biofilms (bacterial, fungal and polymicrobial) is the extracellular matrix material.22,23,41 To test the impact of biofilm matrix on the polymethacrylates, we tested the antimicrobial susceptibility of planktonic S. aureus and C. albicans cells grown in the presence or absence of C. albicans biofilm matrix or polymicrobial biofilm matrix. Fluconazole, a positive control agent known to be sequestered by the fungal biofilm matrix,36 showed a 16-fold increase in the MIC for C. albicans in the presence of matrix (Table 4). The MICs of PG3 and PG4 for S. aureus and C. albicans increased by 4- and 2-fold, respectively (Table 4). These increases in MICs were less than what is observed with fluconazole, but nevertheless they were highly reproducible, suggesting that the biofilm matrix sequesters the polymethacrylates. An alternative explanation for the reduced susceptibility to the polymers in the presence of matrix material could be that the matrix promotes the adherence of planktonic cells to the substratum and formation of biofilm-like structures.42 To further clarify this question, we used the C. albicans bgl2ΔΔ mutant strain, which displays lower levels of β-1,3 glucan in the biofilm extracellular matrix and is more susceptible to fluconazole.23 To perform this assay, we used a subinhibitory concentration of PG3 and PG4 (32 mg/L) to detect differences between WT and mutant biofilms. Fluconazole was used as positive control. As expected, the bgl2ΔΔ mutant biofilm was more susceptible to fluconazole than the WT biofilm (Figure 5). Of note, under the experimental conditions used here, exposure of Candida biofilms to fluconazole or PG4 at a sub-BMIC80 concentration led to a slight increase in the cfu relative to biofilms grown in medium only (20%–40%), which was, however, statistically insignificant. We also noticed a similar increase in fungal density in polymicrobial biofilms when combinatorial treatments with fluconazole were used (Figure 3b). The reason for this is currently unclear, but we speculate that it could be due to a change in the ratio between hyphal and yeast forms in the treated biofilms, with yeast cells being easier to enumerate in cfu experiments. Regarding the guanylated polymethacrylates, the bgl2ΔΔ mutant was more susceptible than the WT strain to PG4, but not to PG3 (Figure 5), suggesting a role for matrix β-1,3 glucan in the susceptibility to guanylated polymethacrylates depending on their structure.
Antimicrobial susceptibility of S. aureus and C. albicans in the presence or absence of biofilm matrix
. | MIC (mg/L) for S. aureus ATCC 25923 . | . | MIC (mg/L) for C. albicans DAY185 . | ||||
---|---|---|---|---|---|---|---|
medium only . | C. albicans biofilm matrix . | polymicrobial biofilm matrix . | medium only . | C. albicans biofilm matrix . | polymicrobial biofilm matrix . | ||
Vancomycin | 2 | 1 | 2 | fluconazole | 0.5 | 8 | 8 |
PG3 | 8 | 32 | 32 | PG3 | 16 | 32 | 32 |
PG4 | 8 | 32 | 32 | PG4 | 16 | 32 | 32 |
. | MIC (mg/L) for S. aureus ATCC 25923 . | . | MIC (mg/L) for C. albicans DAY185 . | ||||
---|---|---|---|---|---|---|---|
medium only . | C. albicans biofilm matrix . | polymicrobial biofilm matrix . | medium only . | C. albicans biofilm matrix . | polymicrobial biofilm matrix . | ||
Vancomycin | 2 | 1 | 2 | fluconazole | 0.5 | 8 | 8 |
PG3 | 8 | 32 | 32 | PG3 | 16 | 32 | 32 |
PG4 | 8 | 32 | 32 | PG4 | 16 | 32 | 32 |
Antimicrobial susceptibility of S. aureus and C. albicans in the presence or absence of biofilm matrix
. | MIC (mg/L) for S. aureus ATCC 25923 . | . | MIC (mg/L) for C. albicans DAY185 . | ||||
---|---|---|---|---|---|---|---|
medium only . | C. albicans biofilm matrix . | polymicrobial biofilm matrix . | medium only . | C. albicans biofilm matrix . | polymicrobial biofilm matrix . | ||
Vancomycin | 2 | 1 | 2 | fluconazole | 0.5 | 8 | 8 |
PG3 | 8 | 32 | 32 | PG3 | 16 | 32 | 32 |
PG4 | 8 | 32 | 32 | PG4 | 16 | 32 | 32 |
. | MIC (mg/L) for S. aureus ATCC 25923 . | . | MIC (mg/L) for C. albicans DAY185 . | ||||
---|---|---|---|---|---|---|---|
medium only . | C. albicans biofilm matrix . | polymicrobial biofilm matrix . | medium only . | C. albicans biofilm matrix . | polymicrobial biofilm matrix . | ||
Vancomycin | 2 | 1 | 2 | fluconazole | 0.5 | 8 | 8 |
PG3 | 8 | 32 | 32 | PG3 | 16 | 32 | 32 |
PG4 | 8 | 32 | 32 | PG4 | 16 | 32 | 32 |
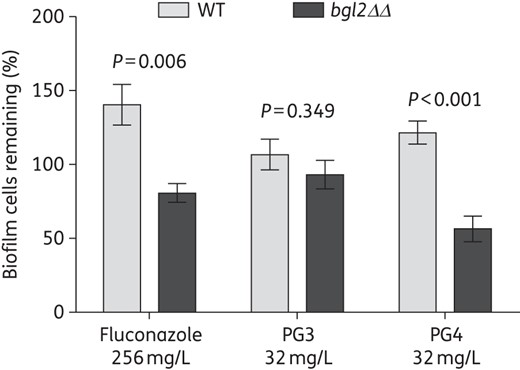
Role of the extracellular matrix in the susceptibility of C. albicans biofilms to the guanylated polymethacrylates. The efficacy of the guanylated polymethacrylates against biofilms of C. albicans DAY185 and bgl2ΔΔ mutant strains was tested by treatment of pre-formed biofilms overnight in the presence or absence of PG3, PG4 or fluconazole. cfu were determined in treated samples versus non-treated controls and are expressed as the percentage of biofilm cells remaining. The experiment was performed with three biological repeats in triplicate; shown are averages and the standard errors.
Potential of guanylated polymethacrylates as antimicrobial lock solutions
Salvage of infected catheters is important in clinical situations when removal is not the preferred option. This can be achieved by the so-called ‘antimicrobial lock therapy’, which entails subjecting the catheter to high concentrations of antimicrobial agents to kill the infecting organisms. Ethanol has been reported as an effective lock solution against C. albicans/S. aureus polymicrobial biofilms,18 but its application in antimicrobial lock therapy has been hindered by side effects.43 To test the potential of guanylated polymethacrylates as catheter lock agents, we assayed regrowth of biofilms after exposure of the pre-formed biofilm to PG3 or PG4 for 18 h. PG3 at 128 mg/L or PG4 at 256 mg/L were effective in salvaging infected biomaterials, effectively eradicating C. albicans/S. aureus polymicrobial biofilms. This effect was comparable to exposure to 20% ethanol overnight (Table 5).
MBECs of the polymers as catheter lock solutions against 24 h monomicrobial or polymicrobial biofilms
. | Ethanol (v/v) . | PG3 (mg/L) . | PG4 (mg/L) . |
---|---|---|---|
S. aureus ATCC 25923 | 20% | 128 | 256 |
C. albicans DAY185 | 20% | 128 | 256 |
Polymicrobial biofilm | 20% | 128 | 256 |
. | Ethanol (v/v) . | PG3 (mg/L) . | PG4 (mg/L) . |
---|---|---|---|
S. aureus ATCC 25923 | 20% | 128 | 256 |
C. albicans DAY185 | 20% | 128 | 256 |
Polymicrobial biofilm | 20% | 128 | 256 |
MBECs of the polymers as catheter lock solutions against 24 h monomicrobial or polymicrobial biofilms
. | Ethanol (v/v) . | PG3 (mg/L) . | PG4 (mg/L) . |
---|---|---|---|
S. aureus ATCC 25923 | 20% | 128 | 256 |
C. albicans DAY185 | 20% | 128 | 256 |
Polymicrobial biofilm | 20% | 128 | 256 |
. | Ethanol (v/v) . | PG3 (mg/L) . | PG4 (mg/L) . |
---|---|---|---|
S. aureus ATCC 25923 | 20% | 128 | 256 |
C. albicans DAY185 | 20% | 128 | 256 |
Polymicrobial biofilm | 20% | 128 | 256 |
Discussion
To our knowledge, guanylated polymethacrylates are the first class of drug to have potent efficacy against polymicrobial biofilms formed by S. aureus and C. albicans as a sole agent. As fungal and bacterial pathogens display distinct cell biology and commonly have different cellular targets for antimicrobial therapy, standard treatment for polymicrobial infections involves a combination of antifungal and antibacterial agents. This approach, however, is not very effective, e.g. a study reported ∼70% treatment failure for Candida/bacteria polymicrobial bloodstream infections.8 This is in agreement with our results that 12 different antimicrobial combinations composed of first-line antibacterial and antifungal agents showed poor efficacy against mature C. albicans/S. aureus polymicrobial biofilms. Interestingly, while we observed that a high concentration of ciprofloxacin (1024 mg/L) failed to eliminate a mature S. aureus ATCC 25923 biofilm, in accordance with previously published studies,35,44,45 a much lower concentration of ciprofloxacin (4 mg/L) used in combination with antifungal agents was effective against the same bacterium grown in a polymicrobial biofilm with C. albicans, causing a 99.6% reduction in viable cell counts. The difference in ciprofloxacin effectiveness against monomicrobial and polymicrobial biofilms might be due to differences in the biofilm structure, cell physiology or extracellular matrix composition between single bacterial and mixed fungal/bacterial biofilms.
In contrast to conventional antimicrobial drugs, the guanylated polymethacrylates had excellent efficacy against C. albicans/S. aureus polymicrobial biofilms as a sole agent, killing ≥94% of S. aureus and ≥80% of C. albicans in the model polymicrobial biofilm formed by laboratory strains. The guanylated polymethacrylates were also active against clinical isolates of S. aureus and C. albicans grown as planktonic cultures, or in monomicrobial and polymicrobial biofilms, with efficacies comparable to the laboratory strains. One exception was S. aureus growing in a polymicrobial biofilm with C. albicans, for which the activity of the polymers was lower for the clinical isolates than for the laboratory strain, but still 80%–95% of the clinical isolates were killed, showing that strong activity was maintained.
The antimicrobial activity of the guanylated polymethacrylates was reduced by 2–4-fold against biofilms compared with planktonic cultures. This reduction is significantly less pronounced than the loss of potency against biofilms observed with many first-line antimicrobial agents, particularly fluconazole and all of the tested antibacterial agents (Table 2 and Figure 3). Our data suggest that disruption of the extracellular matrix material could further improve the efficacy of the polymers and the guanylated polymethacrylates themselves could be further optimized for reduced interactions with the biofilm matrix. We base this conclusion on the fact that addition of isolated biofilm matrix to planktonic cultures led to reduced susceptibility of C. albicans and S. aureus to the polymers. Furthermore, the C. albicans biofilm matrix mutant bgl2ΔΔ was more susceptible than the WT to PG4, but interestingly not to PG3. PG3 differs structurally from PG4 in that it has a higher mole percentage of MMA side chains (50% for PG3 versus 32% for PG4).26 Thus, PG4 is more polar than PG3 as it has a higher ratio of cationic 2-GEMA groups present in the polymer chain. The polar nature of PG4 might be responsible for driving a stronger interaction with the polar groups of the glucan polysaccharide and could explain the increased potency of PG4 against a biofilm mutant with reduced β-1,3 glucan in the matrix. The ease of chemical manipulation of these compounds will allow further optimization of their biofilm eradication activities, e.g. through reduction of matrix interactions, as well as achieving low-toxicity profiles.
We addressed one potential application of guanylated polymethacrylates as antibiofilm agents, by showing that they are effective as catheter lock solutions. The IDSA recommends removal of central venous catheters (CVCs) for both S. aureus and C. albicans biofilm-related infections.46 However, on many occasions removal of a CVC is not practical, due to limited venous access and high mortality related to CVC replacement. Ethanol lock has recently been recommended for CLBSI caused by microorganisms with relatively low virulence (e.g. coagulase-negative staphylococci)47 and has been studied in vitro against monomicrobial and polymicrobial biofilms formed by C. albicans or S. aureus.18,48,49 Although ethanol has advantages, there are concerns about side effects when used as an antimicrobial lock solution, such as systemic toxicity, damage to the catheter materials and the related intraluminal obstruction and thrombosis.43,47 Given this, it is of interest to develop new approaches, such as what we demonstrate here with guanylated polymethacrylates. Further potential uses for guanylated polymethacrylates are chemotherapy for CLBSI, in burn wounds and as coating materials to prevent biofilm formation.
Funding
This work was supported by the National Health & Medical Research Council of Australia (Project grant APP1066647 awarded to A. T.) and the Monash University Interdisciplinary Research grant (awarded to Y. Q., K. L., I. D. H., L. M. and A. T.). Y. Q. is an Australian Research Council (ARC) SuperScience Fellow. J. V.-G. is an ARC DECRA Fellow. We also wish to acknowledge the postdoctoral fellowship awarded to K. L. by the CSIRO's Office-of-the-Chief Executive.
Transparency declarations
None to declare.
Acknowledgements
We thank Joan Clark (Monash MicroImaging Facility), for her assistance with scanning electron microscopy, and Anton Peleg, for providing the clinical isolates of S. aureus and C. albicans.
References
Author notes
These authors contributed equally to this work.