-
PDF
- Split View
-
Views
-
Cite
Cite
Rebecca S. Pettit, Natalie Neu, Jeffrey J. Cies, Craig Lapin, Marianne S. Muhlebach, Kimberly J. Novak, Sean T. Nguyen, Lisa Saiman, David P. Nicolau, Joseph L. Kuti, Population pharmacokinetics of meropenem administered as a prolonged infusion in children with cystic fibrosis, Journal of Antimicrobial Chemotherapy, Volume 71, Issue 1, January 2016, Pages 189–195, https://doi.org/10.1093/jac/dkv289
- Share Icon Share
Abstract
Meropenem is frequently used to treat pulmonary exacerbations in children with cystic fibrosis (CF) in the USA. Prolonged-infusion meropenem improves the time that free drug concentrations remain above the MIC (fT>MIC) in adults, but data in CF children are sparse. We describe the population pharmacokinetics, tolerability and treatment burden of prolonged-infusion meropenem in CF children.
Thirty children aged 6–17 years with a pulmonary exacerbation received 40 mg/kg meropenem every 8 h; each dose was administered as a 3 h infusion. Pharmacokinetics were determined using population methods in Pmetrics. Monte Carlo simulation was employed to compare 0.5 with 3 h infusions to estimate the probability of pharmacodynamic target attainment (PTA) at 40% fT>MIC. NCT#01429259.
A two-compartment model fitted the data best with clearance and volume predicted by body weight. Clearance and volume of the central compartment were 0.41 ± 0.23 L/h/kg and 0.30 ± 0.17 L/kg, respectively. Half-life was 1.11 ± 0.38 h. At MICs of 1, 2 and 4 mg/L, PTAs for the 0.5 h infusion were 87.6%, 70.1% and 35.4%, respectively. The prolonged infusion increased PTAs to >99% for these MICs and achieved 82.8% at 8 mg/L. Of the 30 children, 18 (60%) completed treatment with prolonged infusion; 5 did so at home without any reported burden. Nine patients were changed to a 0.5 h infusion when discharged home.
In these CF children, meropenem clearance was greater compared with published values from non-CF children. Prolonged infusion provided an exposure benefit against pathogens with MICs ≥1 mg/L, was well tolerated and was feasible to administer in the hospital and home settings, the latter depending on perception and family schedule.
Introduction
While cystic fibrosis (CF) is a multi-organ disease, it is the decline in pulmonary function as a result of chronic infection and inflammation that results in significant morbidity and mortality.1,2 Acute exacerbations of lung disease, when moderate or severe, typically require intravenous broad-spectrum antibiotics targeted at the most likely identified pathogens, including Pseudomonas aeruginosa, Staphylococcus aureus and other more difficult-to-treat Gram-negative bacteria.3,4 Treatment is typically initiated with anti-pseudomonal β-lactam antibiotics such as ceftazidime, piperacillin/tazobactam or meropenem, often in combination with a second antibiotic targeting P. aeruginosa or an antibiotic that targets MRSA if suspected.
Antibiotic resistance among Gram-negative bacteria in the CF population is very common and appears to be increasing.5–8 As a result, few options are available to treat exacerbations associated with antibiotic-resistant organisms. Previously, it was demonstrated that standard doses for common intravenous β-lactam antibiotics resulted in lower than expected pharmacodynamic exposures in children with and without CF and that administering these drugs as prolonged (i.e. administered over 3 h) or continuous (i.e. administered over 24 h) infusions improved these exposures.9–13 Sub-optimal dosing in a child with CF may lead to poor clinical outcomes and the development of multidrug resistance.14 Like other β-lactam antibiotics, prolonging the infusion of meropenem to 3 h increases the time that free drug concentrations remain above the MIC (fT>MIC), which is the pharmacodynamic index that best correlates with killing bacteria for the carbapenem agents.15 The pharmacokinetics of meropenem have been described in paediatric patients across various ages.16–20 However, despite the common utilization of meropenem to treat CF acute pulmonary exacerbations, none of these studies has included children with CF. Herein, we describe the population pharmacokinetics, tolerability and feasibility of prolonged-infusion meropenem in children with CF.
Methods
Study design and patients
This was a prospective, multicentre, open-label pharmacokinetic study in paediatric CF patients receiving at least three doses of prolonged-infusion (3 h) meropenem (NCT#01429259) during treatment of a pulmonary exacerbation. This study was reviewed and approved by the institutional review board of all participating centres [Hartford Hospital #003498HE, UNC #4248-12-0198, Drexel (St. Christopher's Hospital for Children) #12050534, Columbia University Medical Center #IRB-AAAI6001, Nationwide Hospital for Children #IRB13-00064, Indiana University (Riley Hospital for Children) IRB#1212010146, Connecticut Children's Medical Center #13-004, Southwestern Texas University (Children's Hospital) #012013-018] and informed assent and consent were required of all participants and their parents/legal guardians. Eligible patients included children with CF aged 6–17 years who were hospitalized for an acute pulmonary exacerbation and required treatment with intravenous antibiotics as per their treating CF physician. An acute pulmonary exacerbation was defined using a modification of the Fuchs criteria;21 exacerbations included at least four signs and symptoms excluding sinus pain or tenderness as recommended by the advisory panel of the FDA. In addition, children deemed to clinically require intravenous antibiotic therapy by their treating CF physician were eligible for enrolment. Patients were excluded if they had known or suspected hypersensitivity to meropenem, concomitant intravenous antibiotic therapy with another β-lactam antibiotic, known fungal or viral infection, pregnancy, moderate to severe renal dysfunction, solid organ transplant within 6 months or participated in another antibiotic clinical trial in the previous 30 days.
Antibiotic therapy
Patients received 40 mg/kg meropenem (maximum 2000 mg per dose) every 8 h with each dose infused as a 3 h infusion. Meropenem was supplied from the participating hospital's pharmacy department and prepared in accordance with US prescribing preparation instructions.22 All doses were prepared either in syringes or infusion bags as per local pharmacy guidelines and were stored in the refrigerator until administered (within 24 h). Patients received a minimum of three doses of meropenem as prolonged infusion, but providers were encouraged to complete the full treatment course (14–21 days) using prolonged infusion. Combination therapy with an intravenous aminoglycoside, fluoroquinolone or polymyxin antibiotic was permitted along with vancomycin or linezolid for MRSA.
Meropenem concentration determination
Blood samples were collected no earlier than after the third dose of prolonged-infusion meropenem. Four samples per patient were collected at the following timepoints: 3–3.5, 4–5, 5–6 and 7–8 h after the initiation of the prolonged infusion, to allow some flexibility with patient care. Actual sampling times were recorded and used for the pharmacokinetic analyses. All blood samples were placed on ice for no longer than 5 min and then immediately centrifuged at 2000 g for 10 min to collect the separated serum. The serum was divided equally into two cryovials for storage at −80°C until meropenem concentrations were determined at the Center for Anti-Infective Research and Development (Hartford, CT, USA) using a previously published validated HPLC assay.23 The inter-day coefficient of variation for high (30 mg/L) and low (0.5 mg/L) check samples were 5.5% and 4.4%, respectively; intra-day coefficients of variation were 1.6% and 2.1%, respectively.
Pharmacokinetic analyses
Concentration data were modelled using the non-parametric adaptive grid program (NPAG) with adaptive gamma in the Pmetrics package for R (version 1.4.1).24 One- and two-compartment models were differentiated based on log-likelihood and the Akaike information criterion (AIC).25 Additionally, predictive performance of the final model was based on the mean weighted predicted–observed error (bias), the bias-adjusted, mean weighted squared predicted–observed error (imprecision) and weighted residual plots. A multiplicative assay variance model was determined by fitting a polynomial to the plot of the inter-day assay standard deviation versus the measured meropenem concentrations, generating the following formula: SD = γ × (0.00761 + 0.0392 × C), where C is meropenem concentration and γ represents all environmental variability excluding the assay. Once the base model was established, linear regression (Sigma Plot Version 12.0, Systat, Chicago, IL, USA) was used to characterize the relationship between clearance and volume of distribution parameters and patient covariates [age (years), height (cm), weight (kg), serum creatinine (mg/dL), glomerular filtration rate (GFR) (mL/min) as estimated by the Schwartz et al.26 equation, BMI (kg/m2), body surface area (m2) and lean body weight (kg) estimated as described by Peters et al.27]. Covariates that were statistically significant were then incorporated back into the population model and tested for model superiority by further reductions in AIC. Body size covariates were tested proportionally to clearance and volume of distribution as well as by allometric scaling.28
Monte Carlo simulation
A 5000-patient Monte Carlo simulation was conducted using the simulator in Pmetrics for meropenem dosage regimens of 40 mg/kg every 8 h as 0.5 and 3 h infusions. Concentrations were simulated at 15 min intervals for six doses. The mean, standard deviation and range for the weight of the participants were used as the simulated population. During simulation, Pmetrics will rebuild a covariance matrix that includes the pharmacokinetic parameters and included covariates, so that these are sampled consistent with their relationships. Probability of pharmacodynamic target attainment (PTA) was assessed over a range of MICs between 0.03 and 128 mg/L in doubling dilutions. The pharmacodynamic index targeted was an fT>MIC ≥40% of the dosing interval.15 The fraction unbound for meropenem was fixed at 0.98.22 An a priori PTA of ≥95% was considered optimal.29 For comparison, the concentration–time profiles for the 30 participants were re-simulated based on the identified individual posterior pharmacokinetic parameter estimates and the actual dosing regimen and timings received for the first 24 h. Concentrations were corrected for the fraction unbound as above and the mean, SD and PTA were calculated at MICs of 2, 4 and 8 mg/L.
Feasibility, safety and treatment burden
The safety and tolerability of prolonged-infusion meropenem were assessed throughout the treatment course until the end of therapy assessment, performed within 7 days of the last meropenem prolonged-infusion dose. Safety was determined by assessment of adverse events reported by the patient and any clinically significant changes in physical examination or laboratory values (chemistry, haematology, liver function tests) between baseline and completion of therapy. Feasibility was assessed by quantifying the percentage of patients completing antibiotic therapy with the prolonged-infusion regimen in and outside of the hospital. Treatment burden was quantified by administering the Cystic Fibrosis Questionnaire-Revised (CFQ-R) before and after the prolonged-infusion meropenem therapy.30 Age-appropriate versions of the CFQ-R were used for children (6–11 years of age, administered by study coordinator to caregiver; 12–13 years of age, self-reported) and adolescents (≥14 years of age, self-reported) participating in the study. These data were analysed qualitatively for conclusions about the burden of prolonged infusion. A minimally clinically significant difference was defined as a change of 4 points in the treatment burden domain.31
Results
Participants
Thirty children were enrolled and completed the pharmacokinetic study. Demographic and clinical characteristics, including body weight parameters used for the Monte Carlo simulation, are provided in Table 1. The bacteria isolated from participants included P. aeruginosa (n = 18/30 patients, 60%), S. aureus [n = 18/30, 60%; 8/18 (44%) were MRSA], Stenotrophomonas maltophilia (n = 5/30, 17%), Achromobacter xylosoxidans (n = 1/30, 3%), Enterobacter cloacae (n = 1/30, 3%), Citrobacter freundii complex (n = 1/30, 3%) and Inquilinus limosus (n = 1/30, 3%). Four (13%) participants had no bacteria isolated at baseline.
Baseline demographic and clinical characteristics of 30 children with CF receiving prolonged-infusion meropenem
Characteristic . | . |
---|---|
Age (years), mean ± SD (range) | 12.7 ± 2.9 (8–17) |
Weight (kg), mean ± SD (range) | 40.9 ± 12.2 (20.4–68.2) |
Lean body weighta (kg), mean ± SD (range) | 33.8 ± 9.1 (19.0–54.4) |
Height (cm), mean ± SD (range) | 149 ± 17 (123–183) |
BMI (kg/m2), mean ± SD (range) | 18.0 ± 2.6 (11.9–24.8) |
Male, n (%) | 14 (47) |
GFRb (mL/min/1.73 m2), mean ± SD (range) | 162 ± 34 (97–229) |
Baseline FEV1 (% predicted), mean ± SD (range) | 58 ± 24 (18–126) |
Historical best FEV1 (% predicted), mean ± SD (range) | 71 ± 25 (23–123) |
Fuchs criteria fulfilled, n (%) | 23 (77) |
Characteristic . | . |
---|---|
Age (years), mean ± SD (range) | 12.7 ± 2.9 (8–17) |
Weight (kg), mean ± SD (range) | 40.9 ± 12.2 (20.4–68.2) |
Lean body weighta (kg), mean ± SD (range) | 33.8 ± 9.1 (19.0–54.4) |
Height (cm), mean ± SD (range) | 149 ± 17 (123–183) |
BMI (kg/m2), mean ± SD (range) | 18.0 ± 2.6 (11.9–24.8) |
Male, n (%) | 14 (47) |
GFRb (mL/min/1.73 m2), mean ± SD (range) | 162 ± 34 (97–229) |
Baseline FEV1 (% predicted), mean ± SD (range) | 58 ± 24 (18–126) |
Historical best FEV1 (% predicted), mean ± SD (range) | 71 ± 25 (23–123) |
Fuchs criteria fulfilled, n (%) | 23 (77) |
Baseline demographic and clinical characteristics of 30 children with CF receiving prolonged-infusion meropenem
Characteristic . | . |
---|---|
Age (years), mean ± SD (range) | 12.7 ± 2.9 (8–17) |
Weight (kg), mean ± SD (range) | 40.9 ± 12.2 (20.4–68.2) |
Lean body weighta (kg), mean ± SD (range) | 33.8 ± 9.1 (19.0–54.4) |
Height (cm), mean ± SD (range) | 149 ± 17 (123–183) |
BMI (kg/m2), mean ± SD (range) | 18.0 ± 2.6 (11.9–24.8) |
Male, n (%) | 14 (47) |
GFRb (mL/min/1.73 m2), mean ± SD (range) | 162 ± 34 (97–229) |
Baseline FEV1 (% predicted), mean ± SD (range) | 58 ± 24 (18–126) |
Historical best FEV1 (% predicted), mean ± SD (range) | 71 ± 25 (23–123) |
Fuchs criteria fulfilled, n (%) | 23 (77) |
Characteristic . | . |
---|---|
Age (years), mean ± SD (range) | 12.7 ± 2.9 (8–17) |
Weight (kg), mean ± SD (range) | 40.9 ± 12.2 (20.4–68.2) |
Lean body weighta (kg), mean ± SD (range) | 33.8 ± 9.1 (19.0–54.4) |
Height (cm), mean ± SD (range) | 149 ± 17 (123–183) |
BMI (kg/m2), mean ± SD (range) | 18.0 ± 2.6 (11.9–24.8) |
Male, n (%) | 14 (47) |
GFRb (mL/min/1.73 m2), mean ± SD (range) | 162 ± 34 (97–229) |
Baseline FEV1 (% predicted), mean ± SD (range) | 58 ± 24 (18–126) |
Historical best FEV1 (% predicted), mean ± SD (range) | 71 ± 25 (23–123) |
Fuchs criteria fulfilled, n (%) | 23 (77) |
Meropenem pharmacokinetics
The two-compartment model fitted the data best, as determined by a smaller AIC score of 122.74 versus 405.73 for the one-compartment model. Among tested covariates, only body weight (kg), lean body weight (kg), height (cm) and body surface area (m2) were significantly associated with meropenem clearance and/or volume of the central compartment (Vc). A detailed description of the covariate model selection and the final weighted residual plot can be found in the Supplementary data available at JAC Online (http://jac.oxfordjournals.org/). The final model produced an AIC of 100.37 and estimated clearance as a ratio of body weight (CL = CL0 × weight) and Vc by linear allometric scaling (Vc = Vc0 × weight/40.9). The final parameter estimates from the population model are presented in Table 2. The final mean ± SD values for clearance and Vc among these 30 participants were 16.14 ± 9.25 L/h and 11.57 ± 5.60 L, respectively. Corrected for body weight, these values were 0.41 ± 0.23 L/h/kg and 0.30 ± 0.17 L/kg, respectively. The final estimate for γ was 0.755, indicating little environmental variability. The observed versus population-predicted meropenem concentration and the observed versus individual-predicted meropenem concentration are provided in Figures 1 and 2, respectively.
Final population pharmacokinetic parameter estimates for 30 children with CF receiving prolonged-infusion meropenem
. | Mean . | SD . | Median . |
---|---|---|---|
Model-derived pharmacokinetic parameters | |||
CL0 (L/h/kg) | 0.41 | 0.23 | 0.37 |
Vc0 (L) | 12.29 | 6.79 | 12.31 |
Kcp (h−1) | 0.738 | 1.165 | 0.195 |
Kpc (h−1) | 1.71 | 1.598 | 0.950 |
Calculated pharmacokinetic parameters | |||
CL (L/h), CL = CL0 × body weight in kg | 16.14 | 9.25 | 14.31 |
Vc (L), Vc = Vc0 × body weight in kg/40.9 | 11.57 | 5.60 | 9.88 |
Vc (L/kg) | 0.30 | 0.17 | 0.30 |
Vss (L/kg) | 0.43 | 0.34 | 0.38 |
half-life (h) | 1.11 | 0.38 | 1.00 |
. | Mean . | SD . | Median . |
---|---|---|---|
Model-derived pharmacokinetic parameters | |||
CL0 (L/h/kg) | 0.41 | 0.23 | 0.37 |
Vc0 (L) | 12.29 | 6.79 | 12.31 |
Kcp (h−1) | 0.738 | 1.165 | 0.195 |
Kpc (h−1) | 1.71 | 1.598 | 0.950 |
Calculated pharmacokinetic parameters | |||
CL (L/h), CL = CL0 × body weight in kg | 16.14 | 9.25 | 14.31 |
Vc (L), Vc = Vc0 × body weight in kg/40.9 | 11.57 | 5.60 | 9.88 |
Vc (L/kg) | 0.30 | 0.17 | 0.30 |
Vss (L/kg) | 0.43 | 0.34 | 0.38 |
half-life (h) | 1.11 | 0.38 | 1.00 |
CL0, clearance; Vc0, allometric volume of distribution of the central compartment; Kcp, microtransfer rate constant from the central compartment to the peripheral compartment; Kpc, microtransfer rate constant from the peripheral compartment to the central compartment; CL, clearance; Vc, volume of distribution of the central compartment, Vss, total volume of distribution at steady state.
Final population pharmacokinetic parameter estimates for 30 children with CF receiving prolonged-infusion meropenem
. | Mean . | SD . | Median . |
---|---|---|---|
Model-derived pharmacokinetic parameters | |||
CL0 (L/h/kg) | 0.41 | 0.23 | 0.37 |
Vc0 (L) | 12.29 | 6.79 | 12.31 |
Kcp (h−1) | 0.738 | 1.165 | 0.195 |
Kpc (h−1) | 1.71 | 1.598 | 0.950 |
Calculated pharmacokinetic parameters | |||
CL (L/h), CL = CL0 × body weight in kg | 16.14 | 9.25 | 14.31 |
Vc (L), Vc = Vc0 × body weight in kg/40.9 | 11.57 | 5.60 | 9.88 |
Vc (L/kg) | 0.30 | 0.17 | 0.30 |
Vss (L/kg) | 0.43 | 0.34 | 0.38 |
half-life (h) | 1.11 | 0.38 | 1.00 |
. | Mean . | SD . | Median . |
---|---|---|---|
Model-derived pharmacokinetic parameters | |||
CL0 (L/h/kg) | 0.41 | 0.23 | 0.37 |
Vc0 (L) | 12.29 | 6.79 | 12.31 |
Kcp (h−1) | 0.738 | 1.165 | 0.195 |
Kpc (h−1) | 1.71 | 1.598 | 0.950 |
Calculated pharmacokinetic parameters | |||
CL (L/h), CL = CL0 × body weight in kg | 16.14 | 9.25 | 14.31 |
Vc (L), Vc = Vc0 × body weight in kg/40.9 | 11.57 | 5.60 | 9.88 |
Vc (L/kg) | 0.30 | 0.17 | 0.30 |
Vss (L/kg) | 0.43 | 0.34 | 0.38 |
half-life (h) | 1.11 | 0.38 | 1.00 |
CL0, clearance; Vc0, allometric volume of distribution of the central compartment; Kcp, microtransfer rate constant from the central compartment to the peripheral compartment; Kpc, microtransfer rate constant from the peripheral compartment to the central compartment; CL, clearance; Vc, volume of distribution of the central compartment, Vss, total volume of distribution at steady state.
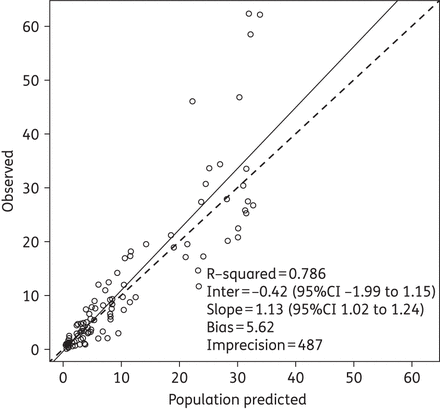
Observed versus population-predicted (using median parameter estimates) meropenem concentrations (mg/L) for the final model. The continuous line is the regression line and the broken line is the line of unity.
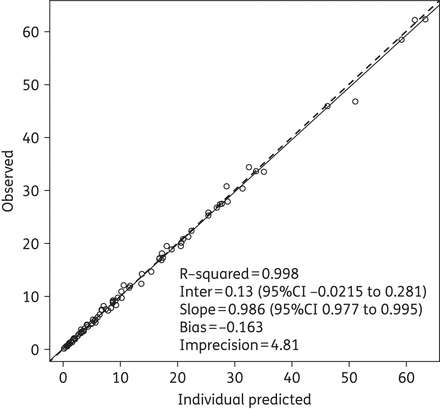
Observed versus MAP Bayesian individual-predicted (using median population parameter estimates) meropenem concentrations. The continuous line is the regression line and the broken line is the line of unity. Lines overlap in the figure.
Monte Carlo simulation
The PTA results for 40 mg/kg meropenem every 8 h simulated as 0.5 and 3 h infusions are displayed in Figure 3. The simulated prolonged-infusion regimen achieved greater PTA compared with the 0.5 h standard infusion at MICs of 0.5, 1, 2, 4, 8 and 16 mg/L. Optimal PTA was achieved for the standard infusion for MICs ≤0.25 mg/L while the prolonged-infusion regimen achieved optimal PTA for MICs ≤4 mg/L.
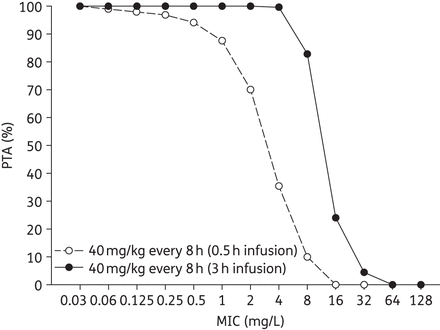
PTA at each MIC for 40 mg/kg meropenem every 8 h regimens administered as 0.5 and 3 h infusion times in children with CF.
For the 30 individual participants, the mean ± SD fT>MIC for their prolonged-infusion regimen of 40 mg/kg every 8 h was 72.8 ± 10.6%, 61.4 ± 8.8% and 49.4 ± 9.4% at MICs of 2, 4 and 8 mg/L, respectively. This corresponded with actual PTAs of 100%, 100% and 90%, respectively.
Feasibility, safety and treatment burden
Prolonged-infusion meropenem was well tolerated in this study, with only one patient discontinuing therapy because of an adverse event. Adverse effects were reported in 8 participants and included leucocytosis (n = 1), thrombocythaemia (n = 1), rash (n = 2), fever (n = 2), elevated C-reactive protein (n = 3), Clostridium difficile infection (n = 2), pancytopenia (n = 1), elevated liver function tests (n = 2) and hyperbilirubinaemia (n = 1).
Participants received a mean ± SD of 32 ± 16 meropenem doses as 3 h infusions during the study, of which 28 ± 16 were received in the hospital. The median meropenem treatment duration was 14 (IQR 11–20) days. Eighteen of the 30 participants (60%) completed their entire course of antibiotic treatment with prolonged-infusion meropenem. Among these 18 participants, 13 (72%) completed their course within the hospital and 5 (28%) were discharged to complete prolonged infusion at home. An additional nine participants (30%) completed antibiotic treatment with meropenem, but had therapy modified to a 0.5 h infusion when discharged home. Three additional participants (10%) had modification of their antimicrobial therapy while in the hospital due to adverse events (n = 1) or lack of response (n = 2).
No participants had prolonged infusion discontinued in the hospital setting due to feasibility concerns. Among the five participants completing prolonged-infusion meropenem at home, none of their families reported a burden. In contrast, the families of the nine participants who chose to receive 0.5 h infusions at home reported doing so because of a perceived burden from using 3 h infusions that would interfere with school attendance, care of other children or both. The CFQ-R was completed (pre- and post-meropenem treatment) by 11 children (6–13 years old), 12 adolescents and 11 caregivers. Among the children/caregivers, data for nine participants were completed by both. Treatment burden scores for the 11 children were 63.6 ± 19.3 and 62.6 ± 27.8 at pre- and post-meropenem evaluations, respectively. Treatment burden scores for the 12 adolescents were 48.6 ± 21.9 and 46.3 ± 21.6 at pre- and post-meropenem evaluations, respectively. Finally, treatment burden scores for the 11 caregivers were 45.0 ± 17.7 and 34.3 ± 16.8 at pre- and post-meropenem evaluations, respectively. The caregivers were the only group reporting an increase in treatment burden after the prolonged-infusion meropenem, as noted by a reduction in score by ≥4 points.
Discussion
In the USA, meropenem is one of a few anti-pseudomonal β-lactam antibiotics routinely prescribed for the treatment of acute pulmonary exacerbations in CF children.32 Despite this common practice, meropenem pharmacokinetics have never been determined in children with CF, a population that is known to eliminate some antibiotics more rapidly than non-CF patients.33–35 Additionally, pharmacokinetic data from adult patients with CF suggest that administering meropenem as prolonged or continuous infusions effectively increases the fT>MIC and enables treatment of exacerbations associated with organisms with higher MICs.36–38 In the current study, meropenem was administered as a 3 h prolonged infusion to 30 children with CF who were admitted for a pulmonary exacerbation to determine pharmacokinetics, tolerability, feasibility and treatment burden.
Increased total body clearance of renally eliminated drugs in CF patients is thought to be due to increased GFR secondary to a primary tubular transport defect or higher resting energy expenditure.39,40 We observed that the clearance of meropenem in these children, when scaled to body weight, was slightly greater compared with values reported from non-CF children. Meropenem clearance in this study was 0.41 ± 0.23 L/h/kg (median 0.37 L/h/kg) and ranged from 0.17 to 1.36 L/h/kg. As identified in many paediatric meropenem pharmacokinetic studies,16–20 weight was a significant covariate of total body clearance. During our modelling, the addition of body weight as proportional to clearance (CL = CL0 × body weight in kg) significantly improved the fit of the model over that of allometric scaling or assessment of other tested body size descriptors (i.e. lean body weight, height) (see Supplementary data available at JAC Online). Our estimates of clearance were greater than that of Du et al.,17 who determined the population pharmacokinetics in non-CF children aged 0.08–17 years (mean weight 16.8 kg) and observed a mean clearance of 0.33 ± 0.09 L/h/kg. Our observations are also greater than the clearance observed in 40 non-CF Japanese children aged 0.2–14 years (mean weight 23 kg), reported at ∼0.34 L/h/kg.20 In contrast, half-life as estimated by the beta terminal elimination phase was largely similar (1.1 h) to that of non-CF children (1.3 h).17 Notably, GFRs, as calculated by the Schwartz et al.26 method, were not significantly associated with clearance in this study. This is different from other paediatric meropenem studies, where creatinine clearance was an important covariate of clearance;17 however, all of the CF participants in the current study had relatively high GFR at baseline, which may have prevented any observation of a significant relationship. In the absence of standard therapeutic drug monitoring for meropenem, further research is needed to identify patient factors that may be predictive of increased clearance and low concentrations.
One in every four CF patients experiencing a pulmonary exacerbation do not recover to their baseline forced expiratory volume in 1 s (FEV1) after treatment.41 As lung function decline has significant morbidity and mortality for CF patients, it is prudent to optimize the treatment of these exacerbations, including proper dosing of antibiotics. This is increasingly important in patients with CF, given the higher rates of antibiotic-resistant bacteria.8 It is well established that β-lactam antibiotics kill bacteria best when their concentrations are maintained above their MIC for a given percentage of the dosing interval.15 Murine infection models have confirmed these observations for meropenem, identifying 40% fT>MIC as the maximum bactericidal exposure during 24 h experiments.15 Unfortunately, these studies have not been conducted in the CF population, so we must assume that the pharmacodynamic index is similar between CF and non-CF patients. Using this exposure as the threshold, the Monte Carlo simulation showed that a standard dosing regimen of 40 mg/kg every 8 h infused over 0.5 h would not achieve optimal PTA once the MIC was above 0.5 mg/L (Figure 3). Of note, P. aeruginosa susceptibility to meropenem is defined by CLSI as an MIC ≤2 mg/L, while 4 mg/L defines intermediate susceptibility and ≥8 mg/L denotes resistance.42 Breakpoints defined by EUCAST are ≤2 mg/L for susceptible and >8 mg/L for resistant.43 We found that prolonging the infusion to 3 h during the simulation resulted in nearly 100% attainment of the 40% exposure threshold for MICs ≤4 mg/L. Furthermore, the prolonged infusion was also able to achieve 40% fT>MIC in 82.8% of simulated patients at an MIC of 8 mg/L, suggesting that this regimen could provide empirical exposure for some non-susceptible P. aeruginosa provided the MIC is not greater than 8 mg/L. These values were in agreement with the actual PTAs achieved by the 30 participants over the first 24 h of exposure (i.e. 100%, 100% and 90% at MICs of 2, 4 and 8 mg/L, respectively) and are also in agreement with studies in non-CF children and adults.10,15,44 It is also worth mentioning that although meropenem could be administered as a continuous infusion,36 its short room-temperature stability of ∼4–6 h makes the 3 h prolonged-infusion regimen particularly attractive.15
Prolonged-infusion meropenem was overall well tolerated in the study. The adverse effects observed were those already reported in the meropenem package insert with the standard infusion time.22 No adverse events were specifically reported due to the prolonged infusion. One critical consideration regarding the prolonged-infusion regimen is the impact on the quality of life of CF patients. CF care creates a substantial treatment burden; however, the children and adolescents completing the CFQ-R in this study did not report any change in treatment burden after receiving the prolonged-infusion regimen. Notably, there was a change in treatment burden scores for the caregivers. Our observations indicate that it is feasible to incorporate prolonged-infusion meropenem during inpatient hospitalization. However, prolonged infusion during home intravenous therapy may be more difficult to incorporate into daily life, especially among school-age children. Despite this perception, two crossover design studies have compared ceftazidime continuous infusion versus intermittent infusion three times a day in paediatric patients in the home setting. These studies found no difference in quality of life scores; moreover, 82% of patients preferred the continuous-infusion regimen in one study.45,46 In our study, patients were given the option of receiving the prolonged infusion at home and five patients successfully completed prolonged infusion in this setting with no reported treatment burden. The nine patients that elected to complete therapy at home with the 0.5 h infusion reported doing so because of the perceived burden that prolonged infusions would interfere with school attendance, care of other children or both. Additional studies are needed to clarify the role and potential value of prolonged infusion in the home setting.
This study has some limitations. The study was not designed to assess clinical outcome; therefore, no control was included. Only 30 participants were included, which, although reasonable for determining pharmacokinetics in a population, may have prevented us from identifying any characteristics that could predict patient outliers and low meropenem concentrations. The small sample size limited assessment of treatment burden, our secondary outcome measure, especially since not all participants completed the CFQ-R and only 5 of the 14 patients who completed intravenous therapy at home received the prolonged infusion in that setting. However, nearly all patients (n = 13/16) completing antibiotics in the hospital remained on the prolonged-infusion regimen. These patients may have been sicker or have had organisms that were more difficult to treat, and are an important target population. Nonetheless, these promising observations, coupled with the pharmacokinetic and Monte Carlo simulation results, justify future studies comparing standard and prolonged-infusion regimens.
Conclusions
To our knowledge, this is the first pharmacokinetic study of prolonged-infusion meropenem in children with CF. The data suggest some differences in meropenem pharmacokinetics compared with non-CF children, notably more rapid total body clearance, which could result in lower meropenem concentrations. Considering this, prolonged-infusion meropenem provided a greater likelihood of obtaining 40% fT>MIC against pathogens with meropenem MICs of 1–8 mg/L. Prolonged-infusion meropenem was well tolerated and feasible to administer in the hospital and home settings, the latter depending on perception and family schedule.
Funding
This study was funded by Thrasher Research Fund, Salt Lake City, UT, USA (grant number 9178 to J. L. K.).
Transparency declarations
None to declare.
Acknowledgements
This study was presented in part at the Fifty-fourth Interscience Conference on Antimicrobial Agents and Chemotherapy, Washington, DC, 2014 (Abstract A-732).
We would like to thank the following members of the Meropenem Extended infusion Response and Outcomes (MERO) Study Group: Alison Champagne, Lisa Bendy, Julia Zhou, Marcella Aramburo, Laurie Varlotta, Stephanie Allen and Carolyn Cannon. We acknowledge Christina Sutherland for conducting HPLC analyses. We also thank Michael Neely, MD, for his guidance during the population pharmacokinetic analysis and Monte Carlo simulation.
References
Author notes
Present address: The Medical Affairs Company, LLC, Kennesaw, GA, USA.