-
PDF
- Split View
-
Views
-
Cite
Cite
Livia Viganor, Anna Clara M. Galdino, Ana Paula F. Nunes, Kátia R. N. Santos, Marta H. Branquinha, Michael Devereux, Andrew Kellett, Malachy McCann, André L. S. Santos, Anti-Pseudomonas aeruginosa activity of 1,10-phenanthroline-based drugs against both planktonic- and biofilm-growing cells, Journal of Antimicrobial Chemotherapy, Volume 71, Issue 1, January 2016, Pages 128–134, https://doi.org/10.1093/jac/dkv292
- Share Icon Share
Abstract
The beneficial antimicrobial properties of 1,10-phenanthroline (phen)-based drugs, together with the imperative need to develop new chemotherapeutic options for prevention/treatment of infections caused by MDR Gram-negative bacteria, led us to evaluate the effects of phen, 1,10-phenanthroline-5,6-dione (phendione), [Ag(phendione)2]ClO4 and [Cu(phendione)3](ClO4)2·4H2O on planktonic- and biofilm-growing Pseudomonas aeruginosa.
Thirty-two non-duplicated Brazilian clinical isolates of P. aeruginosa with distinct genetic backgrounds were used in all experiments. The effect of test compounds on planktonic bacterial proliferation was determined as recommended by CLSI protocol. The effect on biofilm formation was evaluated by crystal violet incorporation (biomass determination) and XTT (viability assay). Mature biofilm disorganization was evidenced by staining with crystal violet.
Phen-based compounds presented anti-P. aeruginosa activity, but with different potencies concerning the geometric mean MIC: [Cu(phendione)3]2+ (7.76 μM) > [Ag(phendione)2]+ (14.05 μM) > phendione (31.15 μM) > phen (579.28 μM). MICs of each compound were similar irrespective of whether the P. aeruginosa isolates were susceptible or resistant to classical antimicrobials (ceftazidime, meropenem and imipenem). The pretreatment of bacteria with phen, phendione and phendione's metal derivatives at 0.5 × MIC value inhibited biofilm formation, particularly the use of [Cu(phendione)3]2+ and [Ag(phendione)2]+, which significantly reduced both biomass (48% and 44%, respectively) and viability (78% and 77%, respectively). The compounds studied also disrupted mature biofilm in a dose-dependent manner, especially [Ag(phendione)2]+ and [Cu(phendione)3]2+ (IC50, 9.39 and 10.16 μM, respectively).
Coordination of phendione to Ag+ and Cu2+ represents a new promising group of anti-infective agents, which revealed a potent anti-P. aeruginosa action against both planktonic- and biofilm-growing cells.
Introduction
Pseudomonas aeruginosa is a widespread Gram-negative bacterium that inhabits the surfaces of natural and artificial aqueous environments. Moreover, P. aeruginosa is a relevant pathogen able to cause a variety of severe opportunistic infections in immunocompromised individuals, causing significant levels of morbidity and mortality.1 Aggravating this scenario, P. aeruginosa is a pathogen typically isolated from nosocomial infections, and the emergence of MDR P. aeruginosa isolates limits the number of effective antimicrobial agents that are available to treat infected patients, leading to a calamitous situation in the medical arena worldwide.1–3 The biofilm-forming capability of P. aeruginosa isolates is a crucial virulence attribute that decisively contributes to bacterial persistence, being directly responsible for the pathogen's increased resistance to numerous environmental stresses, such as different classes of antibiotics and disinfectants, as well as both cellular and humoral host immune responses.4,5 Given the immediate demand for improved therapeutic drugs and new treatment strategies for combating antibiotic resistance, considerable attention has been paid to the development of novel, potent and less toxic antimicrobial compounds suitable for clinical application. In this context, drugs possessing the ability to inhibit planktonic bacterial growth and/or to eradicate/disarticulate biofilm formed by P. aeruginosa are urgently required.4,6
In recent years, the field of bioinorganic, metal-based chemotherapeutics has undergone a renaissance, and particular attention has been paid to the development of potential drug candidates comprising organic heterocyclic ligands chelated to a central transition metal ion.7 1,10-Phenanthroline (phen) is one of the earliest and most extensively studied N,N′-heterocyclic chelating ligands, and several published papers have shown that phen-based drugs possess potent antimicrobial properties.7–10 For instance, Raman et al.9 synthesized a series of mixed-ligand complexes with the general formula [M(L)(phen)2]Cl2 [where M = Cu(II), Ni(II), Co(II) or Zn(II), L = Schiff base ligand (C15H14O2N2) and phen is a co-ligand], which exhibited a broad spectrum of both antibacterial (e.g. against P. aeruginosa, Escherichia coli, Staphylococcus aureus, Staphylococcus podity and Klebsiella pneumoniae) and antifungal (e.g. against Aspergillus niger, Fusarium solani, Curvularia lunata, Rhizoctonia bataticola and Candida albicans) activities, presenting MIC values ranging from 1.3 to 14.5 mg/L.
Preceding studies on the antibacterial properties of metal-free organic compounds, including heteroaromatic derivatives of phenanthrene, such as phen and 1,10-phenanthroline-5,6-dione (phendione), revealed that inclusion of metal-chelating N,N′-atoms in the phenanthrene ring generally increased the antimicrobial activity.7 In this sense, the phendione derivatives revealed a high antimicrobial efficacy against the most-resilient Gram-negative bacterial isolates.7 In an effort to create new phen and phendione metal complex compounds having increased antimicrobial activity and reduced cytotoxicity to host cells, McCann et al.11 synthesized several compounds, among which were [Ag(phendione)2]ClO4 and [Cu(phendione)3](ClO4)2·4H2O (Figure 1). In vivo tests showed that these complexes were well tolerated by the insect larvae of Galleria mellonella (100% survival ≤33.3 mg/kg per larvae) and also by Swiss mice (100% survival ≤150 mg/kg).12 Additionally, blood samples taken from the Swiss mice revealed that levels of the two hepatic enzymes, AST and ALT, were unaffected by treatment with the phendione complexes.12 Interestingly, phendione, in both free- and metal-complexed forms, was able to alter the functioning of a variety of microbial systems.7,8,11,12 For instance, the compounds inhibited the viability of yeasts (e.g. C. albicans, IC50 values <1.4 mg/L), filamentous fungi (e.g. Pseudallescheria boydii, IC50 values <0.2 mg/L) and bacteria such as E. coli (MIC values ≤0.5 mg/L).7,8,12 Due to the promising antimicrobial activity of phendione-based compounds, coupled with the necessity for development of new chemotherapeutic options for prevention and/or treatment of infections caused by MDR Gram-negative bacteria, the aim of the present study was to evaluate the effects of phen, phendione, [Ag(phendione)2]+, [Cu(phendione)3]2+ and the simple metal salts AgNO3 and CuSO4·5H2O on planktonic bacterial proliferation and on biofilm formation and disarticulation observed in clinical isolates of P. aeruginosa.
2·4H2O and [Ag(phendione)2]ClO4 {represented by [Cu(phendione)3]2+ and [Ag(phendione)2]+, respectively}.](https://oup.silverchair-cdn.com/oup/backfile/Content_public/Journal/jac/71/1/10.1093_jac_dkv292/2/m_dkv29201.jpeg?Expires=1747908403&Signature=3KsM2QJegtjTGWcdGpePVuXtwAtYqahhABuXI3S~RP401F2klU3ZccWrPYrnvCfzn-Zvo~5uvW-Wd8gPSPMHV~MrbPvPEgvs8USfz6EF5eq~QHEVnyEDtjFI5JUK2amTYBU4~AFgJ2hk9whevVCvkI0-~YEn5Flq~aDEGJcaZNPOqjUCMR6aTei-ihzRtOVjsHMUkDLcd4ShZarxvT5lW1orjCUbo8taerKzzTAKw7Ee5W11pi46R2Uo8-qqBkX82a-nk3g9vduSZdhIuFilcPPKgOcV9xYXrkN0ps3x40Wa5nC7I2bBX00AVffHcNL0kdywj8UUw1Rb~ELTZ-m2Sg__&Key-Pair-Id=APKAIE5G5CRDK6RD3PGA)
Chemical structures of phen, phendione and phendione's copper(II)- and silver(I)-based complexes, [Cu(phendione)3](ClO4)2·4H2O and [Ag(phendione)2]ClO4 {represented by [Cu(phendione)3]2+ and [Ag(phendione)2]+, respectively}.
Materials and methods
Chemicals
Phen, crystal violet, menadione, XTT, DMSO, AgNO3 and CuSO4·5H2O were purchased from Sigma-Aldrich Chemical Co. (St Louis, MO, USA). Tryptone soy agar (TSA) and tryptone soy broth (TSB) were obtained from Merck (Darmstadt, Germany) and Mueller–Hinton broth (MHB) was purchased from Becton, Dickinson and Company (BD, Sparks, MD, USA). Phendione, [Ag(phendione)2]ClO4 and [Cu(phendione)3](ClO4)2·4H2O (Figure 1) were prepared in accordance with the methods described in the literature.11 All other reagents were of analytical grade.
Clinical isolates
A total of 32 non-duplicated clinical isolates of P. aeruginosa from distinct anatomical sites—rectum (n = 6), tracheal aspirate (n = 6), blood (n = 5), mouth (n = 5), venous catheter (n = 4), pleural secretion (n = 2), urine (n = 1), eschar (n = 1), cystic-fibrosis lung (n = 1) and sputum (n = 1)—of patients hospitalized in intensive treatment units between 1999 and 2010 were used in all parts of the present work.13 Recently, these P. aeruginosa clinical isolates were characterized by their antimicrobial susceptibility profiles and genetic diversity, showing both susceptible (n = 18 isolates) and resistant (n = 14 isolates) profiles against ceftazidime, meropenem and imipenem, as well as distinct genotypes as judged by random amplification of polymorphic DNA analysis.13
Effects of test compounds on planktonic bacterial growth
The antimicrobial susceptibility profiles were determined according to the protocol recommended by the CLSI14 using a 96-well microtitre plate with MHB containing 1.56–200 mg/L of each test compound (starting from a 20 mM solution in DMSO). Water dilutions of DMSO corresponding to those used to prepare the drug solutions were assessed in parallel. From the exponential bacterial growth (24 h) in TSA, colonies were suspended in sterile saline (0.85% NaCl) and adjusted to a turbidity equivalent to that of a 0.5 McFarland standard (108 cfu/mL). This bacterial suspension was serially diluted to obtain a final inoculum of ∼106 cfu/mL. Ten microlitres of this final inoculum was added to each plate well containing 190 μL of each test compound. The plate was then incubated for 24 h at 37°C. The lowest concentration of each compound that inhibited bacterial growth, as ascertained by the absence of visible turbidity in each well, was considered the MIC. Systems containing MHB plus bacterial suspension, MHB plus test compound solutions, MHB plus DMSO, MHB plus DMSO plus bacterial suspension and MHB only were used as controls.
Effects of test compounds on biofilm formation
Bacterial suspensions were made in TSB medium at 108 cfu/mL. Afterwards, 100 μL of the culture was distributed in each well of flat-bottomed 96-well polystyrene microtitre plates and incubated for 24 h at 37°C in the absence or in the presence of the test compounds administered at a concentration of 0.5 × MIC. Subsequently, supernatants were collected and the wells were washed three times with 200 μL of saline solution. In order to evaluate biofilm biomass, 100 μL of crystal violet solution (0.3% w/v) was added to all wells. After 15 min, the excess of crystal violet was rinsed off by placing the microtitre plates under running tap water. Finally, after drying the plates, bound crystal violet was released by adding 100 μL of 70% ethanol with 10% isopropyl alcohol. Absorbance was measured spectrophotometrically using a Thermomax Molecular Device microplate reader at 540 nm.13,15 In parallel, the amount of viable cells in the biofilm was assessed by a colorimetric assay that quantitatively measures the metabolic reduction of XTT to a water-soluble, brown formazan product. In this sense, 100 μL of the XTT/menadione solution [4 mg of XTT was dissolved in 10 mL of pre-warmed PBS (0.15 M NaCl/0.01 M phosphate buffer, pH 7.2) and supplemented with 100 μL of menadione stock solution, which contained 55 mg of menadione in 100 mL of acetone] was added to all wells and incubated in the dark at 37°C for 3 h. The contents of the wells were transferred to microcentrifuge tubes and centrifuged at 4000 g for 5 min. A total of 100 μL of supernatant from each well was transferred to a new microtitre plate, and the absorbance value was measured at 490 nm using a microplate reader.13,15
Effects of test compounds on the disarticulation of mature biofilm
Bacterial suspensions were made in TSB medium at 108 cfu/mL. Subsequently, 100 μL of the culture was added to each well of flat-bottomed 96-well polystyrene plates and incubated for 24 h at 37°C. After 24 h of biofilm formation, the test compounds were added over a range of concentrations (1.56–25 mg/L) and incubated for an additional 24 h. The control comprised the bacterial cells in the culture medium and PBS. Biofilm biomass was measured as previously described.13,15
Statistics
All experiments were performed in triplicate, in three independent experimental sets. Data were expressed as mean ± SD. The results were evaluated by analysis of variance (ANOVA) using GraphPad Prism 5 computer software. In all analyses, P values of ≤0.05 were considered statistically significant.
Results
Effects of test compounds on bacterial growth
Antimicrobial susceptibility testing for phen, phendione, [Ag(phendione)2]ClO4, [Cu(phendione)3](ClO4)2·4H2O and the simple metal salts AgNO3 and CuSO4·5H2O was performed as described by the CLSI protocol, and the results are summarized in Table 1. From previous investigation, it is known that 18 of the 32 P. aeruginosa clinical isolates are susceptible to ceftazidime, meropenem and imipenem, while the remaining 14 isolates are resistant to the same conventional antibiotics.13 [Cu(phendione)3]2+ was the most effective at inhibiting bacterial viability (geometric mean MIC, 7.76 μM), followed by [Ag(phendione)2]+ (14.05 μM), phendione (31.15 μM) and phen (579.28 μM). Regarding P. aeruginosa isolates that were known to be either susceptible or resistant to classical antibacterial drugs, there was no significant statistical difference observed between these two studied groups in relation to their susceptibility to phen, phendione, [Ag(phendione)2]+ and [Cu(phendione)3]2+ (Table 1). While the simple silver(I) salt AgNO3 showed moderate anti-P. aeruginosa activity (geometric mean MIC, 48.54 μM), the copper(II) salt CuSO4·5H2O was essentially inactive. Aqueous DMSO, which was used as the solvent for all of the test compounds, was inactive against all the bacterial isolates tested (data not shown).
Effects of test compounds on planktonic growth of P. aeruginosa clinical isolates
Compounda . | Range (mg/L) . | MIC (mg/L) . | ||||||||
---|---|---|---|---|---|---|---|---|---|---|
susceptible isolates (n = 18)b . | resistant isolates (n = 14)b . | overall average (n = 32 isolates) . | ||||||||
MIC50c . | MIC90c . | GM-MIC . | MIC50 . | MIC90 . | GM-MIC . | MIC50 . | MIC90 . | GM-MIC . | ||
Phen | 50–200 | 100 | 200 | 108.01 | 100 | 200 | 105.08 | 100 | 200 | 106.71 (579.28 μM) |
Phendione | 3.125–12.50 | 6.25 | 12.50 | 6.25 | 6.25 | 12.50 | 6.90 | 6.25 | 12.50 | 6.53 (31.15 μM) |
[Ag(phendione)2]+ | 3.125–12.50 | 12.50 | 12.50 | 8.84 | 12.50 | 12.50 | 8.84 | 12.50 | 12.50 | 8.84 (14.05 μM) |
[Cu(phendione)3]2+ | 3.125–12.50 | 6.25 | 12.50 | 7.58 | 6.25 | 12.50 | 7.25 | 6.25 | 12.50 | 7.43 (7.76 μM) |
AgNO3 | 6.25–25 | 6.25 | 12.50 | 7.43 | 6.25 | 25 | 8.84 | 6.25 | 12.50 | 8.25 (48.54 μM) |
CuSO4·5H2O | 6.25–200 | >200 | >200 | ND | >200 | >200 | ND | >200 | >200 | ND |
Compounda . | Range (mg/L) . | MIC (mg/L) . | ||||||||
---|---|---|---|---|---|---|---|---|---|---|
susceptible isolates (n = 18)b . | resistant isolates (n = 14)b . | overall average (n = 32 isolates) . | ||||||||
MIC50c . | MIC90c . | GM-MIC . | MIC50 . | MIC90 . | GM-MIC . | MIC50 . | MIC90 . | GM-MIC . | ||
Phen | 50–200 | 100 | 200 | 108.01 | 100 | 200 | 105.08 | 100 | 200 | 106.71 (579.28 μM) |
Phendione | 3.125–12.50 | 6.25 | 12.50 | 6.25 | 6.25 | 12.50 | 6.90 | 6.25 | 12.50 | 6.53 (31.15 μM) |
[Ag(phendione)2]+ | 3.125–12.50 | 12.50 | 12.50 | 8.84 | 12.50 | 12.50 | 8.84 | 12.50 | 12.50 | 8.84 (14.05 μM) |
[Cu(phendione)3]2+ | 3.125–12.50 | 6.25 | 12.50 | 7.58 | 6.25 | 12.50 | 7.25 | 6.25 | 12.50 | 7.43 (7.76 μM) |
AgNO3 | 6.25–25 | 6.25 | 12.50 | 7.43 | 6.25 | 25 | 8.84 | 6.25 | 12.50 | 8.25 (48.54 μM) |
CuSO4·5H2O | 6.25–200 | >200 | >200 | ND | >200 | >200 | ND | >200 | >200 | ND |
GM-MIC, geometric mean MIC; ND, not determined.
a[Ag(phendione)2]+ and [Cu(phendione)3]2+ represent [Ag(phendione)2]ClO4 and [Cu(phendione)3](ClO4)2·4H2O, respectively.
bAll the clinical strains of P. aeruginosa used in the present work were previously evaluated by disc-diffusion tests regarding their susceptibility profile to ceftazidime (30 mg/L), meropenem (10 mg/L) and imipenem (10 mg/L).13
cMIC50 and MIC90 correspond to the minimum concentrations of test compounds required to inhibit 50% and 90% of the clinical bacterial isolates, respectively.
Effects of test compounds on planktonic growth of P. aeruginosa clinical isolates
Compounda . | Range (mg/L) . | MIC (mg/L) . | ||||||||
---|---|---|---|---|---|---|---|---|---|---|
susceptible isolates (n = 18)b . | resistant isolates (n = 14)b . | overall average (n = 32 isolates) . | ||||||||
MIC50c . | MIC90c . | GM-MIC . | MIC50 . | MIC90 . | GM-MIC . | MIC50 . | MIC90 . | GM-MIC . | ||
Phen | 50–200 | 100 | 200 | 108.01 | 100 | 200 | 105.08 | 100 | 200 | 106.71 (579.28 μM) |
Phendione | 3.125–12.50 | 6.25 | 12.50 | 6.25 | 6.25 | 12.50 | 6.90 | 6.25 | 12.50 | 6.53 (31.15 μM) |
[Ag(phendione)2]+ | 3.125–12.50 | 12.50 | 12.50 | 8.84 | 12.50 | 12.50 | 8.84 | 12.50 | 12.50 | 8.84 (14.05 μM) |
[Cu(phendione)3]2+ | 3.125–12.50 | 6.25 | 12.50 | 7.58 | 6.25 | 12.50 | 7.25 | 6.25 | 12.50 | 7.43 (7.76 μM) |
AgNO3 | 6.25–25 | 6.25 | 12.50 | 7.43 | 6.25 | 25 | 8.84 | 6.25 | 12.50 | 8.25 (48.54 μM) |
CuSO4·5H2O | 6.25–200 | >200 | >200 | ND | >200 | >200 | ND | >200 | >200 | ND |
Compounda . | Range (mg/L) . | MIC (mg/L) . | ||||||||
---|---|---|---|---|---|---|---|---|---|---|
susceptible isolates (n = 18)b . | resistant isolates (n = 14)b . | overall average (n = 32 isolates) . | ||||||||
MIC50c . | MIC90c . | GM-MIC . | MIC50 . | MIC90 . | GM-MIC . | MIC50 . | MIC90 . | GM-MIC . | ||
Phen | 50–200 | 100 | 200 | 108.01 | 100 | 200 | 105.08 | 100 | 200 | 106.71 (579.28 μM) |
Phendione | 3.125–12.50 | 6.25 | 12.50 | 6.25 | 6.25 | 12.50 | 6.90 | 6.25 | 12.50 | 6.53 (31.15 μM) |
[Ag(phendione)2]+ | 3.125–12.50 | 12.50 | 12.50 | 8.84 | 12.50 | 12.50 | 8.84 | 12.50 | 12.50 | 8.84 (14.05 μM) |
[Cu(phendione)3]2+ | 3.125–12.50 | 6.25 | 12.50 | 7.58 | 6.25 | 12.50 | 7.25 | 6.25 | 12.50 | 7.43 (7.76 μM) |
AgNO3 | 6.25–25 | 6.25 | 12.50 | 7.43 | 6.25 | 25 | 8.84 | 6.25 | 12.50 | 8.25 (48.54 μM) |
CuSO4·5H2O | 6.25–200 | >200 | >200 | ND | >200 | >200 | ND | >200 | >200 | ND |
GM-MIC, geometric mean MIC; ND, not determined.
a[Ag(phendione)2]+ and [Cu(phendione)3]2+ represent [Ag(phendione)2]ClO4 and [Cu(phendione)3](ClO4)2·4H2O, respectively.
bAll the clinical strains of P. aeruginosa used in the present work were previously evaluated by disc-diffusion tests regarding their susceptibility profile to ceftazidime (30 mg/L), meropenem (10 mg/L) and imipenem (10 mg/L).13
cMIC50 and MIC90 correspond to the minimum concentrations of test compounds required to inhibit 50% and 90% of the clinical bacterial isolates, respectively.
Effects of test compounds on biofilm formation
The effects of phen, phendione and phendione's two metal complexes on biofilm formation by P. aeruginosa isolates were analysed in terms of total biomass and cellular metabolic activity. For this test, bacterial cells were incubated with the compounds at 0.5 × MIC value during the whole period of biofilm formation (24 h) on a polystyrene substrate. Phen, phendione, [Ag(phendione)2]+ and [Cu(phendione)3]2+ were able to inhibit biofilm formation, reducing the biomass by ∼23%, 31%, 44% and 48%, respectively (Figure 2a and b), and biofilm viability ∼25%, 65%, 77% and 78%, respectively (Figure 2c and d).
![Effects of test compounds on biofilm formation by P. aeruginosa. Thirty-two clinical isolates were used in this set of experiments. Each bacterial suspension (100 μL) was dispensed into a 96-well polystyrene plate and incubated for 24 h at 37°C in the absence or in the presence of phen, phendione, [Ag(phendione)2]+ and [Cu(phendione)3]2+ at a concentration equivalent to 0.5 × MIC in order to adhere and form a biofilm. Biofilm biomass was measured by crystal violet incorporation and absorbance value at 540 nm (a and b), and cellular viability was quantified by XTT and absorbance value at 490 nm (c and d). The distribution of biofilm biomass (a) and viability (c) in each clinical strain regarding each analysed system was plotted. In parallel, the values representing the mean ± SD concerning biofilm biomass (b) and viability (d) for each analysed system are also shown. The grey lines in (a) and (c) indicate the arithmetic mean of the production of biofilm in each system. Stars denote a significant difference between untreated and treated bacterial cells (P < 0.05; one-way ANOVA, Dunnett's multiple comparison test).](https://oup.silverchair-cdn.com/oup/backfile/Content_public/Journal/jac/71/1/10.1093_jac_dkv292/2/m_dkv29202.jpeg?Expires=1747908403&Signature=yEVtnuuls-FpTxcgiuN3ES9aXzpAcT0tHWvxiIO5tg7qF0x09vF1CvEG4QM4rctOraReThhlRsYzhBPo8b8lu24XxboYuFVuJQ6RbAj9Nt9ZZxMsFW6n21BzTN8Un5WCJdOLKXHObdGt3pG1ySPHZ8Z3-lmaUimHTvBokdqOvCQTH4V5DEDMVwLw9l5epgiWye30ydw93vJRKrKZP170j~~CdmEgFEtQsSnJB2An0CiHeOuYw2wq8c4T3AGpiv2Nm7m4KY5zeC~SVRKT5n7Mh2ishky7d6O0alFawJ9adg9pimQiFOu2gjDovxoau8kKdQ479p2P1oEyQawfnZxn8g__&Key-Pair-Id=APKAIE5G5CRDK6RD3PGA)
Effects of test compounds on biofilm formation by P. aeruginosa. Thirty-two clinical isolates were used in this set of experiments. Each bacterial suspension (100 μL) was dispensed into a 96-well polystyrene plate and incubated for 24 h at 37°C in the absence or in the presence of phen, phendione, [Ag(phendione)2]+ and [Cu(phendione)3]2+ at a concentration equivalent to 0.5 × MIC in order to adhere and form a biofilm. Biofilm biomass was measured by crystal violet incorporation and absorbance value at 540 nm (a and b), and cellular viability was quantified by XTT and absorbance value at 490 nm (c and d). The distribution of biofilm biomass (a) and viability (c) in each clinical strain regarding each analysed system was plotted. In parallel, the values representing the mean ± SD concerning biofilm biomass (b) and viability (d) for each analysed system are also shown. The grey lines in (a) and (c) indicate the arithmetic mean of the production of biofilm in each system. Stars denote a significant difference between untreated and treated bacterial cells (P < 0.05; one-way ANOVA, Dunnett's multiple comparison test).
Effects of test compounds on the disarticulation of mature biofilm
The ability to disorganize mature biofilm formed by P. aeruginosa clinical isolates was also assessed. Following 24 h of biofilm formation, phen, phendione, [Ag(phendione)2]+ and [Cu(phendione)3]2+ were then added at different concentrations and the mixtures incubated for an additional 24 h. The disarticulation of mature biofilm was quantified by the incorporation of crystal violet dye (Figure 3). The results showed that phen, phendione, [Ag(phendione)2]+ and [Cu(phendione)3]2+ disrupted mature biofilm in a dose-dependent manner. The compound concentrations required to disarticulate 50% of the pre-formed biofilm were: phen, 134.90 µM; phendione, 42.12 µM; [Cu(phendione)3]2+, 10.16 µM; and [Ag(phendione)2]+, 9.39 µM (Figure 3).
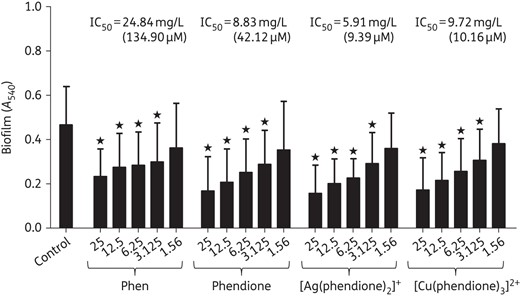
Effects of phen, phendione and phendione's metal complexes on mature biofilm formed by P. aeruginosa. The clinical bacterial isolates (n = 32) were added to a polystyrene substrate (96-well polystyrene plates) for 24 h in order to form biofilm. Subsequently, the test compounds were added (concentrations ranging from 1.56 to 25 mg/L) to each well, and the plates were incubated for an additional 24 h. A control was used in which the bacterial cells were added to just culture medium and PBS. Finally, biofilm biomass was measured by crystal violet incorporation and absorption measurement at 540 nm following test compound administration at IC50 of formed biofilm. The values shown represent the mean ± SD concerning biofilm biomass for each analysed compound. Stars denote a significant difference between untreated and treated bacterial cells (P < 0.05; one-way ANOVA, Dunnett's multiple comparison test).
Discussion
The current emergence of P. aeruginosa infection in hospital settings poses a serious public health problem, because it negatively affects clinical outcomes and contributes to enhanced treatment costs.16,17 Because of the MDR character of P. aeruginosa, the treatment of infections caused by this pathogen with classical antimicrobial drugs often results in successive failures. Moreover, the lack of new antimicrobials has contributed immensely to the extensive emergence, proliferation and dissemination of resistant bacteria.16,17 Consequently, there is an urgent demand for the international scientific community to expand the development of new and/or modified chemotherapeutic drugs having novel mechanisms of action, in order to circumvent the universal problem of bacterial resistance.18
Phen and phendione are powerful chelating ligands with an inherent capacity to form thermodynamically stable complexes with a large variety of transition metal ions.19,20 The established proficiency of metal-free and metal-complexed phenanthrolines as antimicrobials has prompted research efforts directed towards the synthesis of new phen-based compounds possessing enhanced activity, reduced adverse side effects and an ability to counteract bacterial resistance.7,8 The present results have augmented earlier findings of the antimicrobial activity of this class of compound, evidencing the significant antibacterial activity of phendione and its silver(I) and copper(II) complexes against a vast collection of clinical isolates of P. aeruginosa having distinct genetic and phenotypic backgrounds. In this context, it is extremely relevant to highlight that phendione and its metal-containing drugs were able to robustly reduce the viability of clinical isolates of P. aeruginosa previously characterized as resistant to imipenem, meropenem and ceftazidime.13 The compounds tested herein presented the following potency against P. aeruginosa planktonic cells: [Cu(phendione)3]2+ > [Ag(phendione)2]+ > phendione > phen. These findings are in accordance with earlier studies, which reported that phendione and its Ag(I) and Cu(II) complexes are considerably more bioactive than phen and its corresponding metal complexes.7,12,21 Prisecaru et al.21 showed that the use of both metal-free phen and Cu(II) bis-phen complexes was completely ineffective (MIC95 ≥1000 μM) against the reference strain of P. aeruginosa ATCC 9027. So, the use of phendione ligands may be of particular interest towards developing agents that are active against this widespread bacterial species. It was suggested that the more facile electrochemical activity of the phendione ligand, compared with that of phen, possibly augmented its activity by allowing it to participate destructively in cellular redox reactions.7,11,12
The present finding that the antimicrobial action of the silver(I) and copper(II) phendione complexes was significantly (P < 0.05; one-way ANOVA, Tukey's multiple comparison test) greater than that of metal-free phendione and the simple metal salts AgNO3 and CuSO4·5H2O suggests a potentiating activity of the whole metal–phendione coordination complex as a unit against the planktonic bacterial cells, taking into consideration the micromolar concentration of each test compound able to inhibit the bacterial viability. It has been speculated that, in comparison with the simple metal salts, the formation of the general ligated metal complex increases its lipophilicity because of the reduction of the total electron density on the free ligand, as well as the sharing of the positive charge of the metal with the ligand's donor atoms, which induces an electron delocalization over the whole chelate ring.22 All of these physicochemical features may aid in improving the permeability of the complex through the bacterial cell envelope, culminating in a more favourable way to bind to the intracellular target site(s) in comparison with the parental ligand or the metal ion alone.22 Previous studies have also revealed that some metal-based drugs are able to: (i) disturb the respiration process; (ii) block the synthesis of proteins and thus restrict a new cycle of growth; (iii) interact with DNA by a variety of means including intercalation, groove binding or external electrostatic binding, causing severe perturbation of the genetic flow; and (iv) cleave nucleic acids and proteins.7,23–25
Bacterial infection is the result of an imbalance between the microbial virulence armamentarium and host defences.26 Virulence factors help the bacterial cells to colonize, invade and evade the host responses, which decisively contribute to the establishment, development, aggravation and persistence of an infectious process.2,4,26 Following this line of thinking, the ability of P. aeruginosa cells to bind to both biotic and inert surfaces and subsequent biofilm formation are crucial steps in the establishment of a chronic disease, whose treatment is an extremely arduous task.2,4,27 By taking into consideration factors such as total biomass and bacterial viability, the present study evidenced that phendione and its silver(I) and copper(II) complexes significantly (P < 0.05; one-way ANOVA, Dunnett's multiple comparison test) inhibited the capacity of clinical isolates of P. aeruginosa to build a biofilm structure on the inert substrate, polystyrene. Moreover, the test compounds were able to disrupt mature biofilm in a dose-dependent manner. However, no significant difference was observed between the treatment of bacterial cells with phendione and [Ag(phendione)2]+ or between phendione and [Cu(phendione)3]2+ concerning all biofilm parameters analysed (e.g. biomass, viability and biofilm disruption). Beeton et al.28 described that copper(II) complexes with general chemical structure [Cu(IL)(AL)]2+ (IL represents functionalized phen and AL represents 1S,2S- or 1R,2R-diaminocyclohexane) presented anti-biofilm-forming activity against a clinical isolate of MRSA, reducing the biomass by ∼35%–68% at an administered concentration of 25 mg/L. Tay et al.29 determined the MIC and MBC values of phendione for Enterococcus faecalis as 2 mg/L and 16 mg/L, respectively. However, in order to kill E. faecalis cells embedded in a biofilm structure, a concentration of 64 mg/L of this metal-free chelator was required.
In conclusion, phendione chelated to silver(I) or copper(II) ions represents a new promising group of anti-infective agents, which present a potent anti-P. aeruginosa effect against both planktonic- and biofilm-growing cells. Encouragingly, previous in vivo studies conducted by our research groups revealed that [Ag(phendione)2]+ and especially [Cu(phendione)3]2+ had no significant toxicity towards a murine model, which opens up the realistic potential of future clinical acceptance of these complexes.7,12 Another prospective application would be the incorporation into or the surface coating of medical devices, such as catheters, with these metal-based drugs in order to deter biofilm formation and, consequently, the onset of biofilm-related infections.30 Numerous experiments are currently in progress in our laboratories in an attempt to decipher the mechanisms of action behind the anti-P. aeruginosa effects of metal complexes formulated with phendione.
Funding
This study was supported by grants from both Brazilian and Irish agencies: Fundação de Amparo à Pesquisa no Estado do Rio de Janeiro (FAPERJ), Fundação de Amparo à Pesquisa e Inovação do Espírito Santo (FAPES), Conselho Nacional de Desenvolvimento Científico e Tecnológico (CNPq), Coordenação de Aperfeiçoamento de Pessoal de Nível Superior (CAPES), Research Brazil Ireland (RBI) and Science Foundation Ireland (SFI).
Transparency declarations
None to declare.
Acknowledgements
We would like to thank Denise Rocha de Souza, who is supported by an FAPERJ scholarship, for technical assistance.
References
Author notes
These authors contributed equally to this work.