-
PDF
- Split View
-
Views
-
Cite
Cite
Qian-Nan Li, Li Li, Guanmei Hou, Zhen-Bo Wang, Yi Hou, Zhong-Hua Liu, Heide Schatten, Qing-Yuan Sun, Glucocorticoid exposure affects female fertility by exerting its effect on the uterus but not on the oocyte: lessons from a hypercortisolism mouse model, Human Reproduction, Volume 33, Issue 12, December 2018, Pages 2285–2294, https://doi.org/10.1093/humrep/dey322
- Share Icon Share
ABSTRACT
What is the impact of glucocorticoid (GC) on female reproduction?
Corticosterone (CORT) exposure causes little damage to oocyte quality or developmental competence but has an adverse effect on the uterus, which causes decreased implantation, embryo death and subsequent infertility.
Chronic treatment with high GC doses is effective in controlling most allergic diseases but may lead to metabolic disorders such as obesity that are closely related with reproductive function.
Hypercortisolism was induced in a female mouse model by supplementing the drinking water with 100 μg/ml of CORT. Controls received vehicle (1% v/v ethanol) only. After 4 weeks treatment mice were either mated or killed in estrus for hormone and organ measurements. In the first experiment, treatment with CORT or control continued during pregnancy but in the second CORT treatment was stopped after mating. To identify the effects of GC exposure on the uterus, blastocysts were generated by IVF of oocytes from CORT and control mice and replaced into recipients receiving the opposite treatment.
The effects of hypercortisolism on female mice were first characterized by living body fat content, body weight, food intake, hormone and biochemical measurements, a glucose tolerance test and an insulin resistance test. Fertility was determined with or without CORT-treatment during pregnancy. Oocyte quality was assessed by oocyte maturation, mitochondrial distribution, reactive oxygen species production, mitochondrial DNA mutations and morphology of blastocysts produced in vivo or in vitro. Blastocyst cross-transfer was done to evaluate the causes of embryonic development failure. Fetus development and uterus morphology evaluation as well as culture of oocytes in vitro with gradient concentrations of CORT were also carried out.
In the hypercortisolism female mouse model, body weight and food intake were much higher than in the control, and corticosterone, estradiol, cholesterol (CHO) and triglycerides (TG) in the plasma of CORT-treated mice was significantly increased. The hypercortisolism female mice were infertile when CORT-treatment was sustained during pregnancy but fertile if CORT-treatment was stopped after mating. The rate of successful implantation in hypercortisolism mice with sustained CORT-treatment during pregnancy was significantly lower than in the control, and the implanted embryos could not develop beyond 13.5 dpc. Blastocyst cross-transfer showed that blastocysts from CORT-treated mice could develop to term in the uterus of control mice, but blastocysts from control mice failed to develop to term when they were transferred into CORT-treated mice, providing evidence that the infertility was mainly caused by an altered uterine environment. CORT administration did not affect oocyte maturation, mitochondrial distribution, ROS production and blastocyst morphology, but increased mitochondrial DNA mutations. Culture of oocytes in vitro with gradient concentrations of CORT showed that only very high concentrations of CORT caused damage to oocyte developmental competence.
NA.
The mouse model has the advantages of a consistent genetic and physiological background and openness to experimental manipulation over clinical studies but may not represent the human situation.
Our findings show that special care should be taken when administering CORT during pregnancy, and provide important information concerning female reproduction when treating patients by subjecting them to chronic GC exposure.
This study was supported by the National Key R&D Program of China (Nos. 2016YFA0100400 and 2017YFC1000600) and the National Natural Science Foundation of China (31472055). The authors have no conflicts of interest.
Introduction
Glucocorticoids (GCs), which are released from the adrenal cortex to maintain homeostasis, are important steroid hormones for life in mammals (McEwen, 1997; Sapolsky et al., 2000). GCs exert their pleiotropic effects on many target organs, and participate in a systemic stress response through activation of the hypothalamic–pituitary–adrenal axis (Herbert, 1998). GCs, including natural GCs (corticosterone in mice; cortisol in humans) and synthetic GCs (such as prednisolone or dexamethasone) which were developed based on the structure of hydrocortisone, are common clinical medications for almost all allergic diseases, however, they cannot be suitable alternatives to the natural GCs in physiological settings. Chronic excessive GC exposure, which arises from either Cushing’s syndrome or long-term GC therapy, can lead to metabolic disorders, obesity, glucose intolerance and others (Arnaldi et al., 2003; Buttgereit et al., 2005).
It has been confirmed that GC is also important for reproductive development. Follicular fluid cortisol levels may affect oocyte quality during IVF in human (Simerman et al., 2015), and GC participates in the regulation of mouse endometrial decidualization and embryo implantation during early pregnancy (Whirledge et al., 2015; Thiruchelvam et al., 2016). It can even cross the placental barrier and blood–brain barrier of the fetus at high concentrations (Zarrow et al., 1970; Xu et al., 2011), and it also poses risks to the development of organs (Chen et al., 2012; Ziejewski et al., 2012; Rog-Zielinska et al., 2014). Moreover, there are many target organs or tissues for GC in reproduction, such as the ovary (Hirst et al., 1990; Rae et al., 2004), placenta (Waddell et al., 2000), uterine epithelium (Whirledge et al., 2013), testis and germ cells (Michael et al., 2003). As a result, the effect of GC on reproductive development is complex and multifaceted.
Although GC is crucial for reproduction, its effect on the oocyte is controversial. Adding cortisol to culture medium improved maturation of oocytes and their subsequent fertilization and embryo development in the bovine (da Costa et al., 2016), but impaired oocyte development in the lamb (Gonzalez et al., 2010a; 2010b). Adding dexamethasone decreased oocyte developmental potential in the pig (Yang et al., 1999), however, it did not affect lamb oocytes (Gonzalez et al., 2010a; 2010b). Although cortisol and dexamethasone had little effect on oocytes in the mouse, corticosterone (CORT) exposure significantly decreased oocyte maturation, fertilization and embryo development at high concentrations (Andersen, 2003; Gonzalez et al., 2010a; 2010b).
Although numerous researches about the effect of GCs in vitro have been done, the reproductive repercussions of GC treatment in vivo have not been explored. To further ascertain the influence of GCs in physiological settings, a hypercortisolism female mouse model was established by CORT administration similar to that previously reported in male mice (Karatsoreos et al., 2010).
Materials and Methods
Hypercortisolism female mouse model
Five week-old C57BL/6 J female mice were purchased from SPF (Beijing) Biotechnology Co., Ltd. The feeding conditions obeyed the guidelines stipulated by the Ethics Committee of the Institute of Zoology, Chinese Academy of Sciences. The mice were group-housed (n = 5/cage). After 1-week acclimation, mice were assigned into two treatment groups for 4 weeks. One was CORT-treated mice (CORT), the other was vehicle-treated mice (control). In accordance with a previous report (Karatsoreos et al., 2010), CORT (Aladdin, China) was dissolved in 100% ethanol, and then diluted into the drinking water of CORT-treated mice to a final concentration of 1% v/v ethanol and 100 μg/ml CORT. The drinking water of vehicle-treated mice contained 1% v/v ethanol.
Mice and their surplus food were weighed weekly. After 4 weeks treatment, representative CORT-treated mice and vehicle-treated mice were sacrificed in estrus. Blood was collected by removing eyeballs and stored overnight at 4°C, then centrifuged at 4000 g for 10 min. Plasma samples were stored at −20°C for future measurements. To determine fat distribution, mice were anesthetized and scanned by Quantum FX microCT (PERKINELMER, USA). Then the data were analyzed by Analyze 10.0—Caliper MicroCT Analysis Tools (AnalyzeDirect, USA) asafter (Judex et al., 2010).
Hormone and biochemical measurements
GC concentrations in plasma were measured by IBL-International Corticosterone (Human, Rat, Mouse) ELISA Kit (Tecan Trading AG, Switzerland). Leptin concentration was measured by MOUSE Leptin ELISA Kit (Multi Sciences, China). Estradiol and testosterone were measured by Estradiol Radioimmunoassay Kit and [125I] Testosterone Radioimmunoassay Kit from Beijing North Institute of Biological Technology. Cholesterol (CHO), free fatty acid (FFA) and triglyceride (TG) were measured by corresponding kits from Nanjing Jiancheng Bioengineering Institute. The experimental procedures were as described in the manufacturer’s instructions.
Glucose tolerance test and insulin resistant test
For glucose tolerance test (GTT) and insulin resistance test (ITT), mice underwent fasting for 12 or 2 h, respectively, and 2 g/kg glucose or 1 IU/kg insulin was injected in the abdominal hypodermis. Blood glucose from tail blood was monitored at 0, 15, 30, 45, 60, 90 and 120 min by a glucometer (Roche).
Fertility determination
After a 4-week treatment, the mice in estrus were selected to mate with healthy males at night. The next day, vaginal plug was checked to confirm their mating. Treatment of the mated female mice with CORT or vehicle continued. The hypercortisolism female mice were divided into two groups. One was treated with CORT during mating and pregnancy, the other was not treated with CORT during mating and pregnancy. Some mice were used for recording births, the others were sacrificed and dissected to obtain uterus, embryos and placentas at 5.5 days post coitus (dpc), 10.5 and 13.5 dpc.
In vitro and in vivo oocyte maturation and fertilization
The processes followed published protocols (Nagy, 2003). Germinal vesicle (GV) oocytes were obtained by mincing ovaries, followed by IVM in M2 medium (Sigma, USA). The number of mature oocytes was recorded after 12–14 h. To collect metaphase 2 (MII) oocytes, mice were i.p. injected with 8 IU pregnant mare serum gonadotrophin (PMSG) (Ningbo, China) and 48 h later, i.p. injection followed with 8 IU hCG (Ningbo, China). Fourteen hours after hCG, MII oocytes were collected from the Fallopian tubes. Cumulus cells were removed by 1 mg/ml hyaluronidase (Sigma, USA) treatment. For IVF, spermatozoa were obtained after the cauda epididymides of C57BL/6 J male mice were punctured by tweezers and incubated in HTF medium at 37°C, 5% CO2 in air for 40 min. Then capacitated spermatozoa were co-incubated with MII oocytes in HTF medium for 6 h, and the embryos were cultured in KSOM (Milipore, Germany) and subsequent embryo development was observed.
The in vivo blastocysts were obtained by natural mating. Mice in estrus were chosen to mate with males at night. The next day, the mice with vaginal plug were used for collecting blastocysts at 3.5 dpc by uterus flushing.
Blastocyst cross-transfer
First, recipients were prepared for blastocyst transfer. Both CORT-treated and vehicle-treated mice in estrus were chosen to mate at night with vasectomized male mice. Mice with vaginal plug were chosen the next morning for embryo transfer in 2 days. MII oocytes were collected from both CORT-treated and vehicle-treated mice after hormone stimulation. Blastocysts were obtained by IVF after culture in KSOM for 4.5 dpc. The blastocysts were transferred into the uterus of recipients. The CORT blastocysts were transferred into vehicle-treated mice, and vice versa, blastocysts from vehicle-treated mice were transferred into CORT-treated mice. Then vehicle-treated recipients continued to be treated with vehicle, and CORT-treated recipients continued to be treated with CORT.
Immunofluorescence
In order to determine reactive oxygen species (ROS) in oocytes, we used Reactive Oxygen Species Assay Kit (ROS Assay Kit) (Beyotime, China). The GV and MII oocytes were incubated with dichlorofluorescein (DCFH) in M2 medium with DCFH diacetate (Beyotime, China) (2 μM) at 37°C for 30 min.
To stain mitochondria, the GV and MII oocytes were incubated with 200 nM MitoTracker Red (Molecular Probes, Eugene, OR, USA) for 30 min at 37°C. All stained samples were processed for imaging by fluorescence microscopy.
MtDNA mutation detection
A total of 12–14 oocytes were used in each of the two groups. DNA amplification was carried out by using whole genome amplification kit (Peking Jabrehoo Med Tech Co., Ltd, China), and library preparation was performed by Easy Prep PGS DNA Lib Prep Kit for Illumina (Peking Jabrehoo Med Tech Co., Ltd). The wild-type mouse mtDNA sequence index was built by bowtie2 software (Langmead et al., 2009), and compared to our sequencing data. Then variation files were obtained through samtools (Li et al., 2009, 2011), which were annotated by VEP (variant Effect Predictor) (McLaren et al., 2016) to obtain the number of mutations.
Culture of embryos in vitro with gradient concentrations of CORT
CORT was dissolved in DMSO and diluted in M2 medium or KSOM. The final concentrations were 10−4, 10−5, 10−6 and 10−7 M. GV and MII oocytes were collected from common C57BL/6 J female without any CORT treatment. The number of GV eggs that showed successful IVM and of embryos that successfully developed to the next stage (e.g. two cell to four cell) were recorded.
Statistical analysis
Fluorescence was measured by fluorescence microscopy (Zeiss LSM510 META), and analyzed by Image J software (Schneider et al., 2012). Data from repeated experiments were analyzed by independent sample T test by SPSS software. Differences at the P < 0.05 level were considered statistically significant.
Results
Establishment of the hypercortisolism female mouse model
To understand the effect of GCs on female fertility in vivo, we established a hypercortisolism female mouse model by adding 100 μg/ml of CORT to drinking water for 4 weeks. Through the hormone and biochemical measurements, we found that the corticosterone level in the plasma of CORT-treated mice was significantly higher compared to that of vehicle-treated mice. Moreover, plasma concentrations of sexual hormones, leptin and lipid profile (CHO, TG and FFA) were also measured (Table I). Among them, estradiol, CHO and TG were increased significantly.
Effect of administration of corticosterone in drinking water (100 μg/ml; CORT) for 4 weeks on plasma hormone levels and lipid profile of mice.
. | Vehicle . | CORT . |
---|---|---|
Corticosterone (nmol/l) | 33.0 ± 10.2 | 107.1 ± 44.9* |
Testosterone (ng/ml) | 4.41 ± 1.18 | 3.27 ± 1.00 |
Estradiol (pg/ml) | 34.4 ± 10.8 | 48.1 ± 11.5* |
Leptin (pg/ml) | 410.2 ± 86.7 | 514.4 ± 162.3 |
Cholesterol (mmol/l) | 1.76 ± 0.35 | 3.57 ± 0.43* |
Triglycerides (mmol/l) | 1.01 ± 0.13 | 3.24 ± 0.72* |
Free fatty acids (umol/l) | 306.8 ± 133.5 | 480.1 ± 111.2 |
. | Vehicle . | CORT . |
---|---|---|
Corticosterone (nmol/l) | 33.0 ± 10.2 | 107.1 ± 44.9* |
Testosterone (ng/ml) | 4.41 ± 1.18 | 3.27 ± 1.00 |
Estradiol (pg/ml) | 34.4 ± 10.8 | 48.1 ± 11.5* |
Leptin (pg/ml) | 410.2 ± 86.7 | 514.4 ± 162.3 |
Cholesterol (mmol/l) | 1.76 ± 0.35 | 3.57 ± 0.43* |
Triglycerides (mmol/l) | 1.01 ± 0.13 | 3.24 ± 0.72* |
Free fatty acids (umol/l) | 306.8 ± 133.5 | 480.1 ± 111.2 |
Data are expressed as group means ± SEM (n > 3 by group).
*P < 0.05 compared with vehicle.
Effect of administration of corticosterone in drinking water (100 μg/ml; CORT) for 4 weeks on plasma hormone levels and lipid profile of mice.
. | Vehicle . | CORT . |
---|---|---|
Corticosterone (nmol/l) | 33.0 ± 10.2 | 107.1 ± 44.9* |
Testosterone (ng/ml) | 4.41 ± 1.18 | 3.27 ± 1.00 |
Estradiol (pg/ml) | 34.4 ± 10.8 | 48.1 ± 11.5* |
Leptin (pg/ml) | 410.2 ± 86.7 | 514.4 ± 162.3 |
Cholesterol (mmol/l) | 1.76 ± 0.35 | 3.57 ± 0.43* |
Triglycerides (mmol/l) | 1.01 ± 0.13 | 3.24 ± 0.72* |
Free fatty acids (umol/l) | 306.8 ± 133.5 | 480.1 ± 111.2 |
. | Vehicle . | CORT . |
---|---|---|
Corticosterone (nmol/l) | 33.0 ± 10.2 | 107.1 ± 44.9* |
Testosterone (ng/ml) | 4.41 ± 1.18 | 3.27 ± 1.00 |
Estradiol (pg/ml) | 34.4 ± 10.8 | 48.1 ± 11.5* |
Leptin (pg/ml) | 410.2 ± 86.7 | 514.4 ± 162.3 |
Cholesterol (mmol/l) | 1.76 ± 0.35 | 3.57 ± 0.43* |
Triglycerides (mmol/l) | 1.01 ± 0.13 | 3.24 ± 0.72* |
Free fatty acids (umol/l) | 306.8 ± 133.5 | 480.1 ± 111.2 |
Data are expressed as group means ± SEM (n > 3 by group).
*P < 0.05 compared with vehicle.
GTT and ITT were performed by detecting glucose clearance and insulin resistance after CORT treatment. After a 4-week treatment, there was no significant difference between CORT-treated and vehicle-treated mice in the GTT (Fig. 1A), but CORT-treated mice showed insulin resistance and a low insulin sensitivity (Fig. 1B).
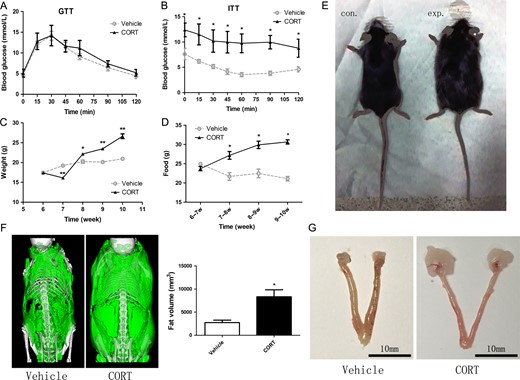
A sketch of the hypercortisolism female mouse model. Mice were treated for 4 weeks with corticosterone (100 μg/ml in drinking water, CORT) or vehicle (1% v/v ethanol; control) (A) Blood glucose levels in glucose tolerance test (GTT). (B) Blood glucose levels in insulin resistance test (ITT). (C) Body weight trend in female mouse model. (D) Weekly food intake. (E) Photograph of a hypercortisolism and a control female mouse. (F) Fat content in CORT and vehicle groups. Pictures show fat (green) from a microCT scan. Histogram was the fat volume in two groups, and the CORT group had significantly more fat than the control group (P < 0.05). (G) The fat pad size close to the ovary was increased, but the ovary size was not changed.
The female mice lost significant weight after the first week of CORT treatment, but their weight began to increase dramatically from the second week on. In subsequent weeks, the weight of CORT-treated mice was significantly higher than that of the vehicle-treated mice (Fig. 1C). Although the weight of CORT-treated female mice decreased during the first week, the weekly food intake was higher than that of the vehicle group during the last 3 weeks (Fig. 1D). After a 4-week CORT treatment, the female mice displayed obvious obese characteristics (Fig. 1E), while their length was almost the same as that of the vehicle-treated mice. The fat was mainly around the abdomen, and the fat content increased significantly in CORT-treated mice (Fig. 1F). The fat pad that was close to the ovary increased, but the ovary size was not changed (Fig. 1G). These results provided an overall picture of the hypercortisolism female mouse model.
Infertility of hypercortisolism female mice with sustained CORT-treatment
To test mouse fertility, CORT-treated (n = 30) and vehicle-treated (n = 10) females were mated with male mice at night during their estrous cycles, and the female mice continued to drink water with CORT. The vaginal plug was checked to verify their mating. All the vehicle-treated mice delivered healthy pups, while CORT-treated mice did not produce offspring.
Fertility of hypercortisolism female mice without CORT-treatment during pregnancy
We also carried out fertility tests on hypercortisolism female mice without CORT-treatment during pregnancy. After 4 weeks CORT treatment was discontinued and the mice were mated with males within 5 days. We found that the CORT-treated females (n = 10) could produce offspring, and there was no significant difference in litter size compared with vehicle-treated (n = 9) females (Fig. 2A).
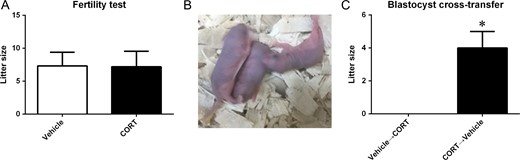
Fertility test and blastocyst cross-transfer. (A) Litter size of the hypercortisolism female mice and controls without corticosterone (CORT)-treatment in pregnancy. (B) Mice vehicle-treated during pregnancy (n = 5) with transferred blastocysts from CORT-treated mice gave birth pups; however, no mice CORT-treated during pregnancy (n = 9) with transferred blastocysts from vehicle-treated mice produced offspring. (C) Litter size of blastocyst cross-transfer.
Blastocyst cross-transfer reveals a CORT effect on the uterus causing infertility
These results showed that CORT-treated mice could produce offspring without CORT-treatment during pregnancy but not if CORT-treatment was continued. To further validate whether the embryo loss of hypercortisolism female mice was caused by the uterus environment or embryo quality, we performed cross-transfers of blastocysts.
MII oocytes from CORT-treated mice were used for blastocyst production by IVF. Then the blastocysts were transferred into the uterus of control mice, and vice versa, blastocysts from control mice were transferred into the uterus of CORT-treated mice which were treated with CORT during pregnancy. We observed that three vehicle-treated mice with transferred blastocysts from CORT-treated mice could give birth to pups (Fig. 2B and C); however, there were no CORT-treated mice (n = 9) with transferred blastocysts from vehicle-treated mice giving birth to pups.
Embryo death of hypercortisolism female mice with continued CORT-treatment during pregnancy
To further confirm the embryo status and embryo death in the uterus, pregnant females were sacrificed at 5.5, 10.5 and 13.5 dpc, respectively. The rate of successful implantation in CORT-treated mice (n = 30) was significantly less than in vehicle-treated mice (n = 10) at 5.5 dpc (Fig. 3A). At 10.5 dpc, the CORT-treated mice showed smaller placentas and embryos also diminished in size but kept the same developmental stage as the vehicle-treated group based on Theiler Stage Definition (Theiler and Westphal, 1989) (Fig. 3B). At 13.5 dpc, the embryos in CORT-treated mice were severely necrotic (Fig. 3C and D).
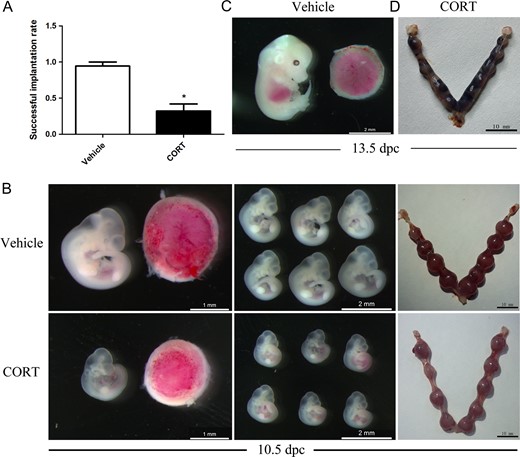
Hypercortisolism female mice treated before and after pregnancy with corticosterone (CORT) were infertile. (A) The rate of successful implantation in the CORT-treated group (n = 30) was less than in the vehicle-treated group (n = 10) at 5.5 days post coitus (dpc). (B) The CORT-treated placentas were smaller and embryos also decreased in size at 10.5 dpc. (C) The vehicle-treated embryos at 13.5 dpc. (D) The uteri of CORT-treated mice contained necrotic fetuses.
The quality and developmental potential of oocytes in CORT-treated mice
The above data already showed that infertility of CORT-treated mice was probably caused by an altered uterus environment but not by decreased oocyte quality and developmental competence. For further proof, GV oocytes from CORT-treated mice (n = 196) and vehicle-treated mice (n = 120) were used for testing maturation ability by IVM (Fig. 4A). There was no significant difference between the two groups. To further understand the oocyte quality of CORT treated mice, we determined the mitochondrial distribution and ROS production in GV and MII oocytes. There was no difference of ROS production in both GV and MII oocytes as revealed by DCFH fluorescence (Fig. 4B and C). Systematic comparative studies of mitochondrial distribution were performed. Different types of mitochondrial distribution were counted separately, and there was no significant difference when comparing the CORT-treated group with the vehicle-treated group (Fig. 5). Next, mtDNA mutations were determined by high-throughput detection techniques. Our analysis found that the number of mtDNA mutations increased significantly in the CORT-treated group compared to the vehicle-treated group (P < 0.05) (Fig. 6A).
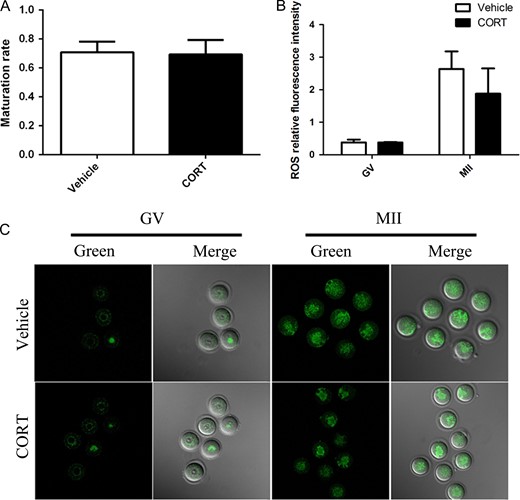
Oocyte maturation and reactive oxygen species (ROS) production in oocytes from corticosterone (CORT)-treated mice. (A) The in IVM maturation rate from CORT-treated mice (n = 196) and vehicle-treated mice (n = 120). (B) Histogram showing quantification of dichlorofluorescein (DCFH) fluorescence; there was no significant difference of ROS production compared to the vehicle-treated group (P > 0.05). (C) ROS production in germinal vesicle (GV) and metaphase 2 (MII) oocytes from CORT-treated mice and vehicle-treated mice.
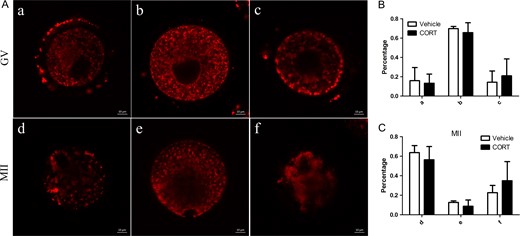
Mitochondrial redistribution in oocytes from corticosterone (CORT)-treated mice. (A) Systematic comparative studies of mitochondrial redistribution in germinal vesicle (GV) and metaphase 2 (MII) oocytes. Mitochondria in GV oocytes showed: a, perinuclear distribution; b, homogenous distribution or c, clustering distribution. In MII oocytes mitochondria showed: d, polarized distribution; e, homogeneous distribution or f, clustering distribution. (B, C) Histograms showing the results of statistical analysis of different types. There was no significant difference compared to vehicle-treated mice (P > 0.05).
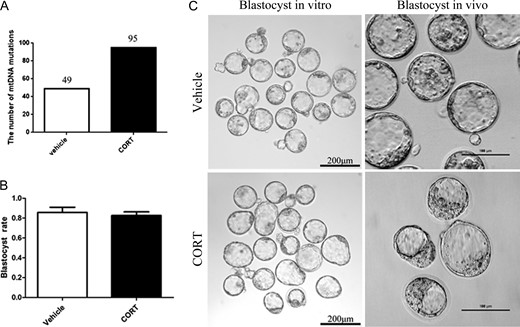
MtDNA mutations in oocytes and blastocyst production in vitro and in vivo in corticosterone (CORT)-treated mice. (A)The number of mtDNA mutations changed significantly compared to the vehicle-treated group (P < 0.05). (B) Histogram showing blastocyst rate by IVF (P < 0.05). (C) The morphology of blastocysts produced in vitro and in vivo.
When MII oocytes were used for IVF, they could develop normally to blastocysts (Fig. 6B). Blastocysts that developed in vivo by natural mating of CORT-treated mice had normal morphology (Fig. 6C). These results showed that there was no obvious difference in oocyte maturation and blastocyst development between the CORT-treated and vehicle-treated mice. CORT-treatment had little effect on oocyte developmental competence.
Only high concentrations of CORT have adverse effects on oocyte competence in vitro
To better understand the relationship between oocyte quality and CORT, we performed IVM and IVF of oocytes with increasing concentrations of CORT (10−7, 10−6, 10−5 and 10−4 M) in the culture medium. Both oocyte maturation and embryo development suddenly decreased at the concentrations between 10−5 and 10−4 M (Fig. 7). Based on these data, we conclude that only high concentrations of CORT had adverse effects on oocytes. This explained why there was no effect on fertility before embryos entered the uterus of CORT-treated mice.
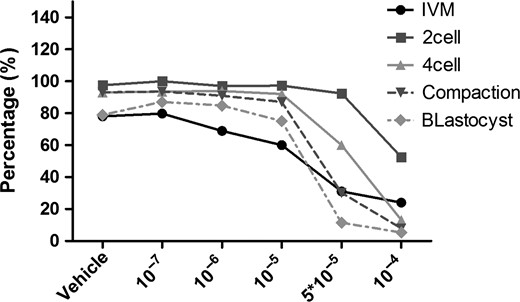
Culture and development in vitro of oocytes with gradient concentrations of corticosterone (CORT). In vitro maturation and in vitro fertilization with gradient concentrations of CORT. Both oocyte maturation and embryo development decreased only at concentrations between 10−5 and 10−4 M. The Y axis represents the percentage of eggs or embryos in the previous stage that successfully develop to the stage shown, e.g. germinal vesicle eggs that show successful in vitro maturation (IVM) by extruding the polar body, embryos which successfully cleave to the two cell stage, two cell embryos that develop to four cell and so on. The X axis shows the molar concentration of CORT added to the culture medium.
Discussion
GCs are important hormones for development, growth, metabolism and immunity, and widely used in clinics. Both natural and synthetic GCs have various effects on different organs in many species. To further understand the impact of GCs on reproduction in female mice, we established a hypercortisolism female mouse model through drinking water supplemented with CORT. After a 4-week CORT-treatment, the female mice were obviously obese and the fat was mainly around the abdomen, but lighter than male mice with the same CORT-treatment (Karatsoreos et al., 2010). Moreover, CORT-treated mice were infertile when CORT-treatment continued during pregnancy but fertile if CORT-treatment ceased after mating. Cross-transfer of blastocysts proved that the infertility was mainly caused by a defective uterine environment. Infertility was characterized by decreasing implantation rates, and finally fetus death before 13.5 dpc. We also examined the quality of oocytes and embryos before entering the uterus. CORT-treatment did not significantly change the quality and developmental potential of oocytes, but only caused an increase in mtDNA mutations.
A CORT-treated male mouse model was reported in 2010 (Karatsoreos et al., 2010). It was shown that after the first week of treatment, male mice treated with 100 μg/ml CORT showed no change in body weight, but the 25 μg/ml CORT-treatment group showed a slight weight loss, and then the weight of both groups significantly increased. In our experiment, the weight trend in female mice treated with 100 μg/ml CORT was not the same as in male mice with the same treatment, but much closer to the trend of the males treated with 25 μg/ml CORT. We believe that the reason accounting for this phenomenon is a sex difference. Although factors regulating physiological feedback by pulses of GCs are sparsely defined in relation to gender (Roelfsema et al., 2016), GCs have interactions with estrogen which is different between the female and male (Rhen et al., 2003). GCs also caused an estradiol increase as evaluated by hormone measurements in this paper, which supported this view.
Recent studies have shown that the GC receptor is necessary for maintaining uterus integrity and fertility (Whirledge et al., 2015), while it can also induce fetal development toxicity by affecting the placental GC barrier (Xu et al., 2011). Here we showed that chronic CORT exposure both before and after pregnancy decreased the implantation rate, and caused the embryos and placentas to diminish in size at 10.5 dpc, finally leading to death before 13.5 dpc. These results provide evidence for the view that GCs not only exert effects on implantation, but also affect embryo development so they gradually die in the uterus with the passing time. The effect of GCs on the fetus’ intrauterine growth may depend on alterations of multiple factors, which need further exploration and clarification.
To understand whether decreased oocyte quality causes infertility with CORT-treatment, we checked the quality and developmental potential of oocytes in a variety of ways; however, and we did not find abnormalities except for increased mitochondrial DNA mutations. In addition, treatment with CORT before pregnancy did not affect female fertility. We also could obtain healthy blastocysts by natural mating from continued CORT-treated mice. The blastocyst cross-transfer experiment confirms that factors before entering the uterus can be ruled out as the reason for infertility. The cross-blastocyst transfer procedures used ART, i.e. MII oocytes were fertilized in vitro and developed to blastocysts and then transferred to the recipient’s uterus. The cross-transfer between CORT-treated mice and vehicle-treated mice showed that an effect of GCs on oocytes is not involved in causing infertility. The oocytes from CORT-treated mice can grow normally in the uterus of vehicle-treated mice.
Both oocyte maturation and embryo development were affected only after in vitro treatment of oocytes with CORT at concentrations much higher than encountered in physiological or pharmacological settings, indicating that clinical use of CORT before pregnancy is reproductively safe, and this is also consistent with the results that female mice treated with CORT before mating show normal fertility.
In conclusion, CORT-treated female mice were obviously overweight, and CORT treatment before pregnancy did not evidently affect oocyte quality and fertility, while CORT treatment during pregnancy caused infertility. The infertility was caused by GCs effect on implantation and subsequent uterus and fetal development. Cross transplantation of blastocysts showed that the adverse CORT effect on the uterus might be the main reason for infertility. These results provide important information concerning female reproduction when treating patients by subjecting them to chronic GC exposure.
Acknowledgements
We are grateful to Xiwen Lin and Changsheng Wu for their technical assistance and all members of Sun lab for their helpful advice.
Authors’ roles
Q.Y.S. proposed and designed the experiment, revised the article, and provided a source of funds for the experiment. Q.N.L. and L.L. jointly completed the main experiment, and wrote the article. G.M.H. analyzed the data about mtDNA mutation detection. Z.B.W., Y.H. and Z.H.L. participated in the experiments and contributed to the discussion. H.S. reviewed the article. All authors approved the final article.
Funding
National Key R&D Program of China (Nos. 2016YFA0100400 and 2017YFC1000600) and the National Natural Science Foundation of China (31472055).
Conflict of interest
There is no conflict of interest in this study.
References
Author notes
Qian-Nan Li and Li Li The authors consider that the first two authors should be regarded as joint First Authors.