-
PDF
- Split View
-
Views
-
Cite
Cite
Semih Ergintav, Emin Demirbağ, Vedat Ediger, Ruhi Saatçılar, Sedat İnan, Ali Cankurtaranlar, Aynur Dikbaş, Mahmut Baş, Structural framework of onshore and offshore Avcılar, İstanbul under the influence of the North Anatolian fault, Geophysical Journal International, Volume 185, Issue 1, April 2011, Pages 93–105, https://doi.org/10.1111/j.1365-246X.2011.04941.x
- Share Icon Share
Summary
To investigate the onshore/offshore structural framework of the northern shelf of the Sea of Marmara, a multidisciplinary study was carried out including multichannel and single-channel seismic reflection profiling, multibeam bathymetry sounding, side-scan sonar imaging on offshore as well as land studies for S-wave velocity estimation by passive and active array measurements and monitoring of block deformations by Global Positioning System (GPS). Based on the multidisciplinary data, possible interactions between landslides and active faulting have been reviewed and their possible relation to the North Anatolian Fault Zone (NAFZ) has been discussed.
It is observed that structurally the most deformed subsurface zone offshore coincides with the most undulated landform due to landslides in the area. This observation leads to idea that the structural complexities of the area may also contribute to land sliding as much as other contributing mechanisms such as slope instability, shallow groundwater level, lithology and liquefaction. The Çatalca Fault Zone, a branch of the inactive Western Black Sea (WBS) Fault, may constitute weakness surfaces that contribute to land sliding in the deformed Miocene layers in the study area. Based on the multidisciplinary and new data sets, we show that ongoing land sliding in the study area should be related not only to common causes of landslides but also the structural framework of the area.
1 Introduction
A better understanding of the active structural framework of the Sea of Marmara, particularly the influence of the North Anatolian Fault (NAF) and its associated submarine basins and ridges, is very important as the area lies in one of the highly populated regions of Turkey (Barka 1999). After the last devastating İzmit and Düzce earthquakes (Turkey) in 1999, different research groups, by using marine geophysical data, investigated the main question whether the northern branch of the NAF (the Main Marmara Fault) is a single thoroughgoing fault zone (İmren et al. 2001; Le Pichon et al. 2001; Demirbağ 2003; Kuşçu 2009), or a set of faults forming a stepover (Armijo et al. 2002; Carton 2005; Hergert & Heidbach 2010). Some researchers are also interested in the deformation due to the NAFZ on the southern shelf (Parke et al. 1999; Okay et al. 2000; Yaltırak & Alpar 2002; Kurtuluş & Canbay 2007; Kuşçu et al. 2009) while others have focused on the Ganos fault and its submarine extension in the Tekirdağ basin (Okay et al. 1999; McHugh et al. 2006). A fragment of the Western Black Sea fault (WBS), the NW–SE oriented Çatalca Fault Zone, is another important, now inactive, former structural feature but no data is available from its offshore continuation (Figs 1 and 2; Okay et al. 1994; Duman et al. 2004).
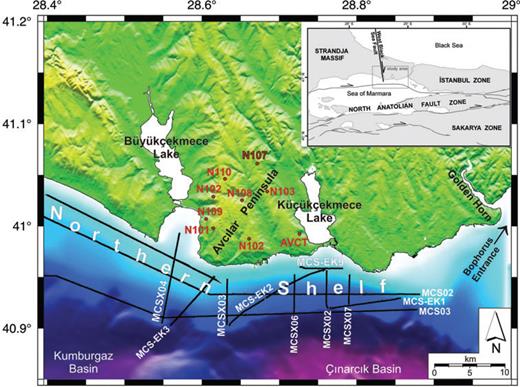
The topographical and bathymetric view of the study area. Topography data are provided by İstanbul Metropolitan Municipality. Bathymetry data merged from this project and Le Pichon et al. (2001). The whole data are processed and mapped by Generic Mapping Tools (GMT; Wessel & Smith 1991). Black lines are the multichannel seismic profiles and labels on land are the GPS sites (AVCT is continuous and the others are campaign GPS sites). Inset map shows the relationship of the West Black Sea Fault (WBSF) to active tectonic and palaeotectonic features in the region (modified from Okay et al. 1994) and study area in the Marmara Sea.
![Simplified geology map of the study area. Note the location of the Çatalca Fault Zone, a fragment of now inactive the West Black Sea (WBS) fault and the distribution of the landslides along the shore lines and Haramidere riverside [simplified from Duman et al. (2004) and Özgül et al. (2005)].](https://oup.silverchair-cdn.com/oup/backfile/Content_public/Journal/gji/185/1/10.1111/j.1365-246X.2011.04941.x/2/m_185-1-93-fig002.jpeg?Expires=1749920237&Signature=omxr6VCxBfRCMNtCt9Dmta5NezBnAtGIillg3~IvjxcmYrmU6vsF9dUusUTxKhoOdTHWbSwJH6S1CnDA2OZHEEAcQVnBfcoer-s58rucrB2lk4IffSgSFl4xDxxzejDFxUZaiOXXtfnjB2DAq6CgcwLZk5Ua66QgIYbySRgXncPC575xyt5e1qPoS9R~yy1s~F4bTxZe-xUiBD-8h9Ys0G5e9ziA9mRxVPJYTBP2IxgN5bMcWQ1dqfq83XEYhVX0SuKwCcIf1sXGNZ65ovebZROroNnOeqE4nIxJago5bAUoyt1djZmL0IlZsRjxyc0LzK0rqPYIzgElEWEHPtlUcQ__&Key-Pair-Id=APKAIE5G5CRDK6RD3PGA)
Simplified geology map of the study area. Note the location of the Çatalca Fault Zone, a fragment of now inactive the West Black Sea (WBS) fault and the distribution of the landslides along the shore lines and Haramidere riverside [simplified from Duman et al. (2004) and Özgül et al. (2005)].
Another issue is the landslides, which are observed in the northern shelf of the Sea of Marmara, especially along the shorelines and the Haramidere valley in the Avcılar Peninsula (Figs 1 and 2). Land sliding is a major problem in the area and the investigation of the landslides is very important in terms of determination of causes and precautions that can be taken to avoid future damage to urbanized area. A total of 391 landslides are mapped in the area covering 60 km2 (Duman et al. 2004). Approximately half of all landslides are distributed between Büyükçekmece and Gürpınar area of the Avcılar Peninsula, which are important local landforms in the region (Fig. 2). Based on field geological observations, Duman et al. (2004, 2005a,b) have argued that the causes of the landslides are slope instability, shallow groundwater level, lithology and liquefaction.
The first geophysical survey in the northern shelf was carried out to collect single-channel shallow seismic data, gravity cores and surface sediment sampling for studying Quaternary geology in the mid-990s (Oktay et al. 2002). The second stage of data collection was after the disastrous earthquakes of 1999. A remarkable study, such as the multibeam bathymetric survey and shallow seismic reflection profiling, was carried out by Turkish–American–Italian cooperation for exploring possible active faults and evaluating earthquake risk in the area (Cormier et al. 2002). In that study, various types of data were collected including side-scan sonar images, multibeam bathymetric soundings, single-channel seismic sections and gravity cores. Among the data sets, single-channel seismic sections have a few hundreds of milliseconds of recording length corresponding to roughly 100–200 m of penetration depths. In general, this is not a sufficient penetration depth for a thorough investigation of structural features of the northern shelf because there is always a possibility of confusing the shallow deformations (e.g. landslides, irregularities in sediment depositions) with active faults. Therefore, deeper penetration is necessary to distinguish the shallow anomalies from active faults in the seismic sections. Although there were existing multichannel deep seismic lines collected by the Mineral Research and Exploration Directorate of Turkey (MTA) before and after the 1999 earthquakes (Okay et al. 1999, 2000; İmren et al. 2001; Demirbağ 2007), industrial lines by the Turkish Petroleum Corporation (Aksu et al. 2000; Ateş 2003), the Turkish–French cooperation in 2001 (Carton 2005) and the Turkish–American cooperation studies in 2008 (Sorlien et al. 2008) were not particularly designed for investigation of the northern shelf. These multichannel lines were mainly collected for the investigation of the northern branch of the NAF and its associated basins and ridges.
Despite the focused researches in and around the deep basins of the Sea of Marmara, the northern shelf with less than 100 m depth, particularly to the west of the Bosphorus exit, is relatively less investigated by multidisciplinary studies. Widespread damage in the eastern part of Avcılar Peninsula during the 1999 İzmit earthquake attracted attention of some researchers on the possibility of active faulting in the northern shelf. Although apparent low seismicity indicates no active faulting in and around the study area (including northern shelf; Eyidoğan 2006), damage to poorly constructed buildings was high and resulted in several hundred deaths during the 1999 İzmit earthquake (Durukal & Erdik 2008). Parts of this abnormally high devastation and life loss were explained by weak bedrock, which enhanced (amplified) the seismic waves (Ergin et al. 2004). However, taking into consideration that vast areas of Istanbul have similar weak bedrock, we presumed that there may be other reasons leading to this extensive damage in the study area. Considering the structural frame of the study area, the first mechanism that comes to mind is the possible interactions of rejuvenated faults and landslides that cause massive damage to the buildings. Thus we intended to investigate possible interactions between seemingly inactive faults and widely observed landslides.
2 Geology of the Study Area
The study area is located on the boundary between Strandja Massif (Palaeozoic–Mesozoic) and İstanbul Zone (Palaeozoic), and both are overlain by Eocene and younger sediments of Thrace basin (e.g. Okay et al. 2001). The Strandja Massif is represented by phyllite, schist and granitoids, which crop out in the Çatalca Fault Zone, essentially a horst bounded by steeply dipping faults (Fig. 2). The metamorphism age is Late Jurassic–Early Cretaceous (Okay et al. 2001, Sunal et al. 2006). The Istanbul Zone is represented by a thick turbiditic sandstone-shale sequence of Carboniferous age, which crop out northeast of the Küçükçekmece Lake (Fig. 2). Middle–Upper Eocene aged limestone units are transgressive on the Çatalca metamorphics in the west and on the Carboniferous flysch in the east; they cover a major dextral strike-slip fault zone, the West Black Sea Fault (WBSF), with a total slip of more than 100 km (Okay et al. 1994). The Eocene limestones pass up into a clastic series of Upper Eocene–Oligocene in age. A major unconformity separates these Eocene–Oligocene sediments from the overlying Upper Miocene fluviatile to lacustrine deposits, represented by marn, limestone, shale and siltstone (Sakınç 1999; Duman et al. 2004). The Miocene deposits are confined to the coastal region (Fig. 2) and are the sites of all the landslides.
The WBSF was probably active from Cretaceous to Mid-Eocene. In Mid-Eocene, the activity of the WBSF was probably ceased during the collision of the Istanbul Zone with the Sakarya continent (Okay et al. 1994). On the other hand, the Çatalca Fault Zone controls the Eocene sedimentation indicating some dip-slip activity during the Middle to Late Eocene. The active NAF passes ca. 10 km of the coast (Okay et al. 1999; Le Pichon et al. 2001) forming an east–west trending segment about 110 km long. It is believed to be active since the Late Miocene–Pliocene (Barka & Hanckok 1985; Aksu et al. 2000).
Marine geophysical data from this study show that the northern shelf area is characterized by a thin blanket of Pliocene/Quaternary deposits underlain by older strata of Miocene and Oligocene age. The older strata are usually parallel layered with gentle slopes and cut by various order of faults. The seismic sections display strong structural anomalies, particularly offshore Avcılar Peninsula, where the older strata are highly deformed. Hence, folding and frequent faulting are observed from seismic reflection sections. To the west, the Miocene and Oligocene strata are gently folded and show no major deformation.
3 Materials and Methods
New offshore data collected in 2007 include: set of multichannel, high resolution, moderate penetration seismic reflection data, multibeam bathymetry sounding, side-scan sonar imaging and single-channel seismic profiling. Onshore studies include: determination of S-wave velocity structure at depth with active/passive array surface wave measurements, monitoring of local block deformations with Global Positioning System (GPS). The multichannel seismic data acquisition is carried out for the first time at west of the Bosphorus in the northern shelf of the Sea of Marmara to investigate offshore structural features such as Çatalca Fault Zone and the shallow deformations as the result of possible submarine landslides. In this paper, we focus on the multichannel reflection data of the project and use the other data sets as supplementary sources to interpret the structural features offshore the Avcılar Peninsula.
3.1 Multichannel and single channel seismic data collection: equipment and parameters
The seismic data were collected on 14 profiles with total of 234 km of length. Data acquisition parameters are selected so that they provide moderate penetration with high resolution for a conventional seismic reflection study (Pritchett 1990). A single generator-injector airgun with total of 180 (75 + 105) cubic inches is used as seismic source. Shots are fired every 12.5 m interval controlled by a differential GPS. The seismic signals are detected by a 96-channel streamer. The receiver interval is selected as 6.25 m for smooth and frequent spatial sampling. The sampling rate in time is 1 ms and the record length is 3 s. Note that 1 ms sampling rate in time results in 500 Hz Nyquist frequency. Most of the seismic lines are in north–south direction and closely spaced offshore Avcılar Peninsula and two east–west lines are located parallel to the shoreline (Fig. 1).
The 6.25 m small receiver interval provides high Nyquist wavenumber for avoiding spatial aliasing. The 96 channels recording with 12.5 m of shot and 6.25 m receiver intervals provides 24 fold common-depth-point (CDP) data. The bandwidth of the recorded signal is observed approximately between 10 and 250 Hz. The dominant frequency of the recorded seismic signal is around 110 Hz. The vertical and lateral resolution at the sea bottom are calculated as 3.5 m based on one-fourth of the dominant wavelength and 22.6 m based on the radius of the Fresnel zone, respectively, by accepting 110 Hz dominant frequency, 1500 m s−1P-wave velocity and a typical water depth of 75 m in the northern shelf (Yılmaz 1987). The average velocity at 1 s two-way traveltime is about 2000 m s−1 according to velocity analysis; therefore, the penetration depth of the multichannel seismic data is about 1000 m at 1 s two-way traveltime.
In addition, single-channel seismic data were collected along the same profiles in Fig. 1 simultaneously with the multichannel seismic data, using engineering equipment (Seabed). The bandwidth of the signal is 3.5 kHz. The sampling rate and shot interval are selected as 0.1 ms and 250 ms, respectively. The penetration depth of the single-channel seismic data is about 30 m.
3.2 Multichannel and single channel seismic data processing: priorities, techniques and parameters
To produce geologically meaningful seismic sections, we used one of industry standard seismic data processing packages installed at TÜBİTAK Marmara Research Center. Our data processing priority is to enhance the signal-to-noise (S/N) ratio by removing all kinds of noise and to increase the available resolution in the raw data. We processed the data by conventional means as follows. Manual editing for noisy and dead seismic traces on shot gathers; bandpass filtering for periodic noise; line geometry definition for relative positioning of the shots, receivers and CDP points; spherical divergence correction; sorting the shot data to CDP order data; pre-stack multiple elimination based on f−k filtering; stacking velocity analysis on the CDP super-gathers; normal-moveout (NMO) correction; muting of stretched signals on NMO corrected CDPs; full offset and near offset partial-stacking; post-stack finite difference migration in the time-domain. The final sections are plotted with and without automated gain correction.
The velocity analysis is carried out on the CDP super-gathers to build a 2-D stacking velocity field for every seismic line. The number of CDPs to form a super gather is selected as three within the limits of calculated lateral resolution based on the radius of the Fresnel zone in the sea bottom (22.6 m) and spatial sampling interval determined by the distance between individual CDP traces (3.125 m). The velocity analysis is applied for every 100 CDP stations and every 50 CDP stations where the subsurface structure is complex. The stacking velocity range is between 1500 and 2900 m s−1 for the first 1 s of the data and it usually varies smoothly both horizontally and vertically. To suppress the sea bottom multiples, we applied the following techniques: f–k filtering, p-Radon, predictive deconvolution and multichannel predictive deconvolution. In some sections, multiples seem very strong and we couldn't remove them (e.g. Fig. 5). Partial stacking with nine near offset traces and full fold stacking provided about three and five times improvement in the S/N ratio, respectively.
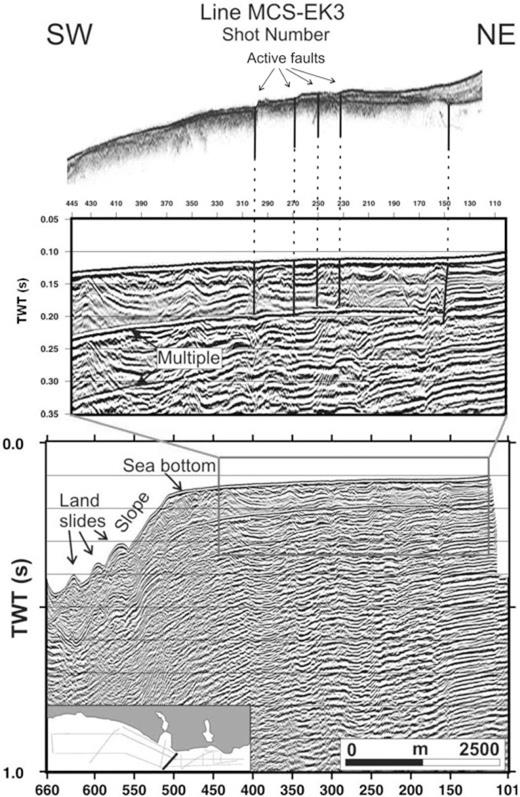
Migrated seismic section MCS-EK3 (bottom panel) and its interpretation of the upper part (middle panel) to minimize the multiple arrival problems. Vertical fault anomalies between shots 150 and 300 in this section are evidences of the Çatalca Fault Zone in this part of the shelf. Southwest part of the section between shots 500 and 660 displays the northern slope and sliding material. Top panel shows the corresponding single-channel shallow section displaying steps in the bathymetry and thin sedimentary coverage, which is an indicator of activity of the Çatalca Fault Zone on offshore.
A high-band filter, which has a 300 Hz cut-off, is applied to single channel data to increase S/N ratio and reduce the swelling effect, after the normalization of the amplitudes in different traces.
3.3 Investigation of S-wave velocity structure
A series of geophysical surveys and geological investigations were carried out to obtain regional information on the macro scale bedrock properties with depth. These surveys include ambient microtremor array (SPACK, f–k), active source surface wave measurements (MASW) and Horizontal-to-Vertical Spectral Ratio (HVSR) from single-station measurements to determine S-wave velocity profile (Park et al. 1999; Chavez-Garcia et al. 2005). Following the data collection and the processing step in the same way as explained by Zor et al. (2010), combined Rayleigh wave dispersion curve produced by processing the array measurements in a broad frequency range (0.5–20 Hz) and the HVSR curve obtained from the single station measurements for each site. Then, with a combined inversion (Dispersion + HVSR) technique, quantitative information on the shear wave velocity structure estimated as a function of depth in the study area.
Average shear velocity down to 30 m is used by the geotechnical engineering society to evaluate the potential site amplifications and the classifications for the different seismic design and building codes (e.g. Turkish Building Code, 2008; NEHRP, 1997). In the case of having inverted velocity model deeper than 30 m, the depth where the layer velocity is greater than a certain velocity can be attributed to the sediment-bedrock depth (Olsen 2000; Zor et al. 2010).
Since we obtained commonly in our velocity models, we choose the certain velocity in this study as 1000 m s−1 described as firm to hard rock in NEHRP classification scheme (Class B in NEHRP; BSSC 1997). For each site, the depth where the layer velocity is greater than 1000 m s−1 is extracted from the velocity model, which we call it as the bedrock depth.
3.4 Geodetic measurements
Eight geodetic measurement sites were constructed in the study area (Fig. 1) to monitor the local deformations. GPS campaigns were conducted at each station, two sessions of 10 h are observed and four campaigns; one every 3 months. After that, two extra campaigns realized for the estimation of the long-term reliability of the estimated GPS velocities within 2 yr. To reduce the antenna phase pattern problems and the errors on the computation of the vertical components, we used the same receivers and antennas at all campaign sites (Trimble 4000 and 5700 with choke-ring and geodetic Zephyr antennas). The GPS data were processed by GAMIT/GLOBK software (King & Bock 1998; Herring 2000), using the all available continuous and campaign sites in the Marmara region (Ergintav et al. 2009). Details about the processing method are described in Ergintav et al. (2009).
All sites are in the Eurasian Plate and the velocities were estimated in the Eurasian reference frame (Ergintav et al. 2009). So, all velocities reflects the local anomalies as a function of ground deformations (e.g. landslides) and the stress loading as the result of the possible active fault branches. The velocities of the sites are given as supplementary Table S1 and Fig. S1 of the paper.
4 Discussion
4.1 Submarine and subbottom features of the northern shelf
In this publication, only the structural interpretations of the seismic sections are presented. The seismic sections can be discussed in two groups based on the trend of the survey line: the first group of sections is across to the shoreline and cross the shelf break. The second group of sections is parallel to both the shoreline and shelf break (Fig. 1). Before making structural evaluations and discussions on their implications, general submarine and subbottom characteristics of the study area need to be summarized from the bathymetry, side-scan sonar and seismic data. Generally, the bathymetry changes smoothly from coastline to 100 m, where the shelf break is located (Fig. 1). Irregular bathymetric features and narrow canyons are observed along the slope. Rocky lineaments are observed in NNW–SSE in limited areas where the older sedimentary strata crops out in strike direction. Deep cut narrow canyons are located on the slope and they connect to the deep basins of the Sea of Marmara. Submarine slides and small bedrock rotations are observed in the slope and canyons. The older folded strata is truncated at top by an angular discontinuity and also cut by a series of NNW–SSE trending faults. A thin blanket of Plio-Quaternary recent deposits cover the older deformed sedimentary strata.
In the following paragraphs, we focus on the large and deep structural anomalies rather than small scale and shallow features. A significant anomaly of the Çatalca Fault Zone is observed in seismic section MCSX04 (Figs 1 and 3). A narrow subbottom trough between shots 800 and 850 is seen in the section. This trough is formed between two subvertical faults joining at deeper parts of the section. An important fault is located at shot 700 that is possible responsible for a push up ridge between shots 550 and 800 as an indicator of a fault bending in a dextral strike-slip fault zone. Section MCS01 displays another important anomaly of the Çatalca Fault Zone between shots 500 and 750 (Figs 1 and 4). This seismic section is located parallel to the shoreline and mouth of Büyükçekmece gulf. Gently folded, SE-dipping strata are severely cut by succession of vertical faults between shots 500 and 750 forming a wide trough. The single-channel seismic reflection section shows the same deformation field in shallow scale (Fig. 4, top section). Plio-Quaternary recent deposits with maximum 33 m of thickness covering the older deformed sedimentary strata are observed. The multichannel section MCS-EK3 is shown with its counterpart single-channel section in Fig. 5. During the data collection, both the single-channel and multichannel sections were recorded simultaneously. To minimize the multiple arrival problems, the upper part of MCS-EK3 and its counterpart single-channel section were reinterpreted to note an activity of the Çatalca fault zone in this part of the shelf. Fault anomalies at shots 230, 250, 270 and 300 affect the sea bottom and can be defined as possible active fault branches. Note that southwest part of the section between shots 500 and 660 displays the northern slope and sliding material.
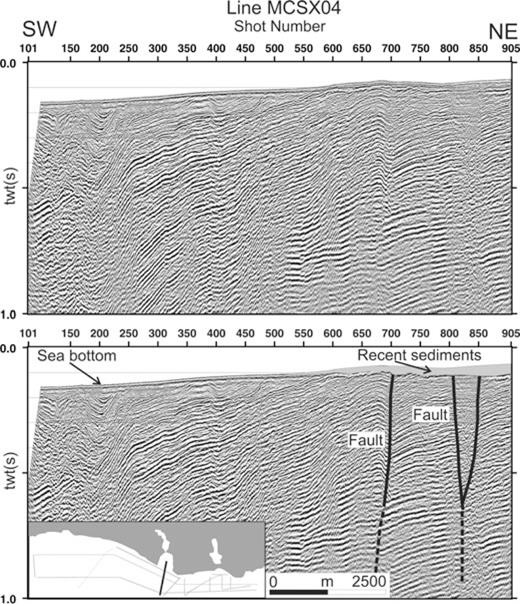
Migrated seismic section MCSX04 and its interpretation. Anomaly of the Çatalca Fault Zone, a fragment of the WBS fault, is observed in this seismic section. A small trough between shots 800 and 850 is seen in the section. This trough is formed between two vertical faults connecting to each other at greater depths. Another fault anomaly is located at shot 700, which is possible, responsible for a push up ridge between shots 550 and 800.
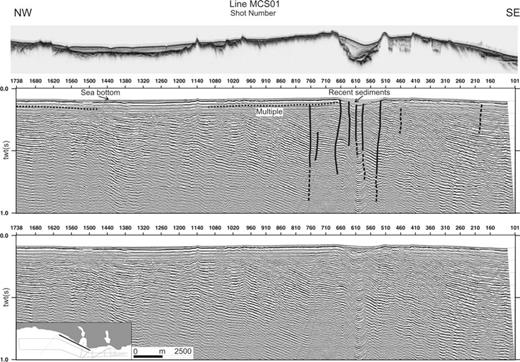
(a) Top figure shows the corresponding single-channel shallow section. (b) Migrated seismic section MCS01 and its interpretation. This section displays an anomaly of the Çatalca Fault Zone between shots 500 and 750. Gently folded, SE-dipping strata are cut by successive subvertical faults between shots 500 and 750.
In each test site, depths at which the reference shear velocity, obtained from the investigation of S-velocity structure, were considered as reference depth (Fig. 6a). The variation of the reference depth in the study area shows that the eastern side of the Çatalca Fault Zone is nearly 250 m deeper than the western side of the fault (Fig. 6b) and possibly controlled by the Çatalca Fault Zone. This velocity anomaly from land studies as well as seismic survey in the shelf define that Çatalca Fault Zone is an important southerly segment of the now inactive WBS fault and its morphological and subsurface characteristics are stand still in the study area. The units showing reflections down to about 1000 m depths in shore-parallel seismic sections are the oldest units in Oligocene to Upper Eocene age based on comparison to natural gas borehole drilled by Turkish Petroleum Corporation in the area (the location cannot be given due to proprietary reasons). Fault activity displacing Oligocene aged strata indicates that the activity on the Çatalca Fault Zone did not cease in Middle Eocene as implied by earlier workers (Okay et al. 1994) or it was rejuvenated after Middle Eocene.
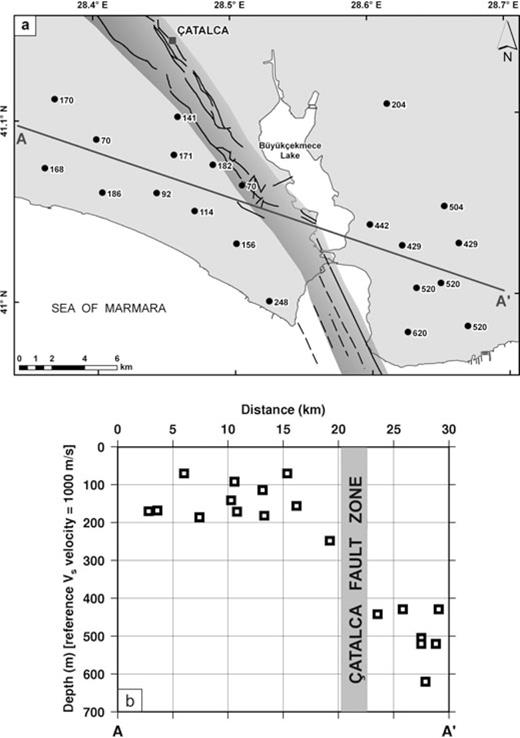
Correlation of shear velocity variation around the Çatalca Fault Zone: (a) Distribution of S-wave velocity measurement sites. Numbers indicate the depths of reference shear wave velocity (See the text). (b) Depth variation of reference shear wave velocity along the AA′ profile. Note that Çatalca Fault Zone causes a vertical offset in the S-wave velocity variation.
Seismic section MCS02 (Figs 1 and 7) clearly displays strongly deformed older substrata covered by thin recent deposits between shots 3750 and 4550. Strong folding and successive faulting is observed particularly across Avcılar peninsula, which is geographically delimited by Büyükçekmece and Küçükçekmece lakes. In this seismic section, we calculated maximum apparent slope of 25° in the layers by converting the time units into depth units in the vertical axis using an average velocity of 2000 m s−1. Rocky outcrops of possible Oligocene age seem as the surface markers of these layers and the evidence of the relatively strong materials in the depths and marked on the single-channel seismic section, clearly (see Fig. 4 top section). The folded layers are cut by vertical faults trending in NNW–SSE direction as indicated by small to moderate vertical offsets in the layers. NW continuations of the faults that cause block tilting coincide with the Haramidere valley, which suggest that the formation of this valley might be structurally controlled (Fig. 7, bottom panel).
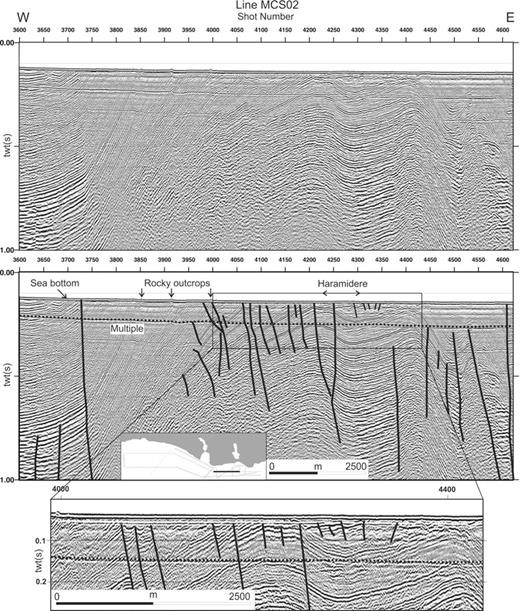
Migrated seismic section MCS02 and its interpretation. This section is the most striking section in this study. It clearly displays strongly deformed older substrata covered by thin recent deposits between shots 3750 and 4550. Strong folding and successive faulting is observed particularly offshore Avcılar district. The folded layers are cut by faults extending in NNW–SSE direction causing small to moderate vertical separations in the layers. Enlarged section (bottom panel) shows tilted blocks among faults indicating a structural background in formation of the Haramidere valley.
Seismic section MCS-EK9 is located close to the Küçükçekmece and displays a few shallow small normal faults terminating at about 100 ms depths shore (Figs 1 and 8). The extension of these faults coincides with western bank of Lake Küçükçekmece, where the land sliding is observed (Fig. 2). Furthermore, some faults cutting the horizontal strata at greater depths at shot locations 150, 250, 350 and 450 are observed in the section. The layers between shots 150 and 250 are depressed and this is more pronounced at greater depths indicating the depression is delimited by faults.
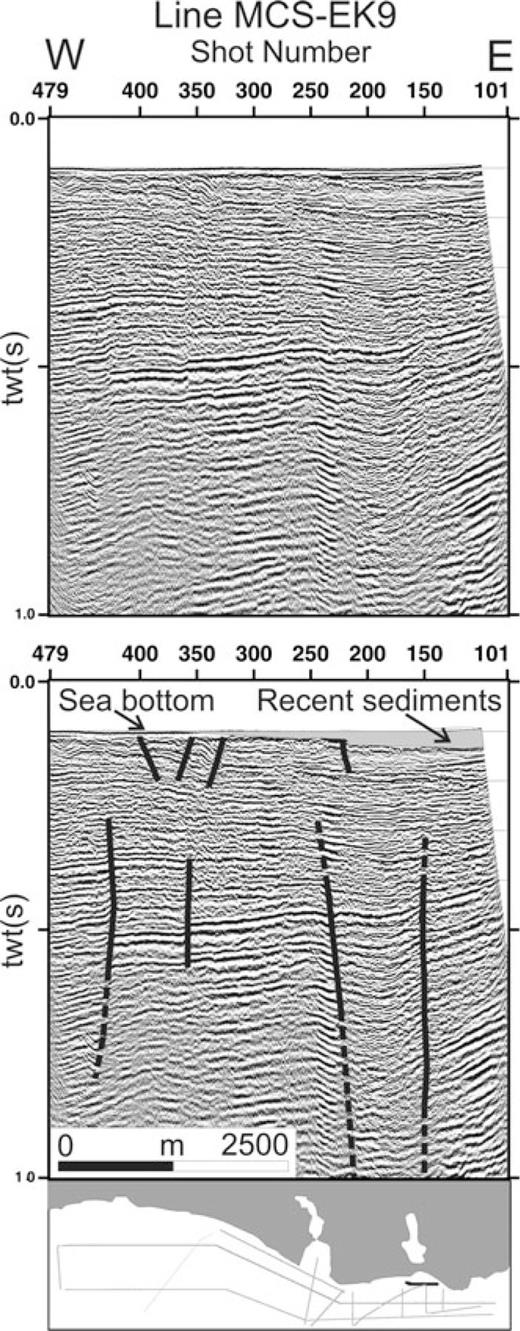
Migrated seismic section MCS-EK9 and its interpretation. This section displays shallow normal faults terminating at about 100 ms depths. The extension of these faults coincides with western bank of Küçükçekmece Lake where land sliding is observed (see Fig. 2). Some other faults cutting the strata at shot locations 150, 250 and 350 are observed in the section. The layers between shots 150 and 250 are depressed and this depression is more pronounced at greater depths indicating fault controlled mechanism.
One of the important observations in the seismic sections is a dome shaped subsurface feature offshore Avcılar district as seen in section MCSX03 (Figs 1 and 9). The dome is located to the south of the section and the older strata shows sharp upslope bending at shot 375 and the layers rest on the dome further south. The internal reflection configuration of the dome is not layered. Note that there is also no surface indication of the dome in the bathymetry in the northern shelf (Fig. 1).
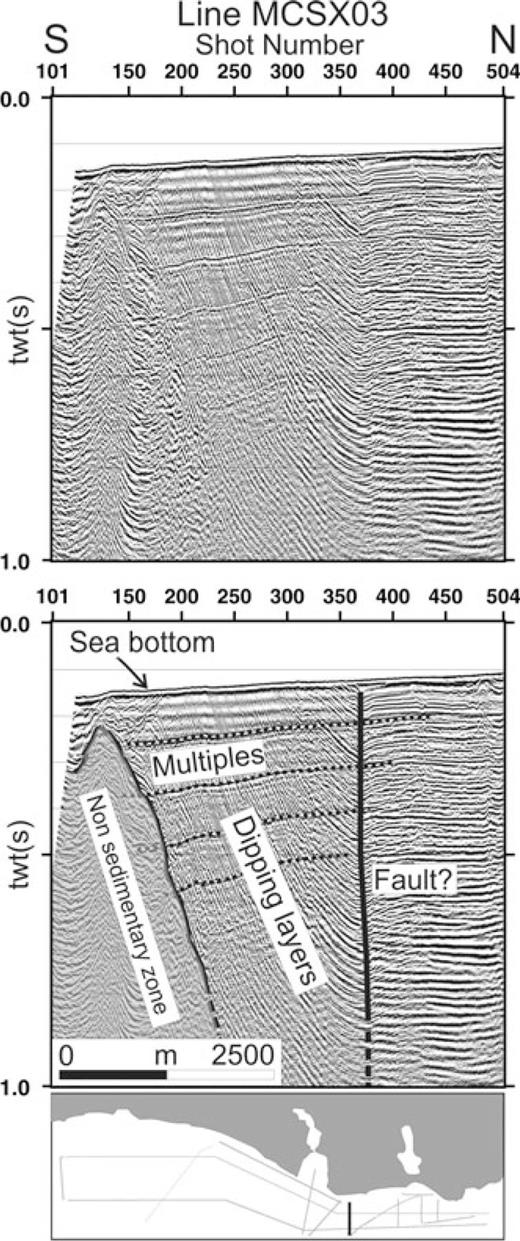
Migrated seismic section MCSX03 and its interpretation. A dome shaped subsurface feature offshore Avcılar district is observed in this section. Older strata show sharp uprise bending at shot 375 and the layers rest on the dome further south. Internal reflection configuration of the dome indicates non-reflective zone.
4.2 Multidisciplinary data and their interpretation
The northern shelf area is characterized by a thin blanket of Pliocene/Quaternary deposits underlain by Miocene and Oligocene age older strata. The older strata is usually parallel layered with gentle slopes and cut by various importance of faults. Near the shelf break, the older strata are carved by long steep canyons connecting to deep basins of the Sea of Marmara. Slumping and sliding are in progress towards to canyon axes and to deep basins.
The dome shaped subbottom feature offshore Avcılar district adds more complexity by causing the older strata bend upward and the layers rest on at east, west and northern walls of the dome. It is where the older strata have the most observed dipping layers. The internal reflection configuration of the dome is not layered; no reflections are seen in the sections. This may lead to interpretation that the dome is a non-reflective zone. Furthermore, the dome is located to the north of the NAFZ and it may be the northern smaller part of a larger portion located south the NAF now belonging to the Central Ridge of the Sea of Marmara separating the Çınarcık and Central Basins. The dome can also be the reason of the aseismic zone in this region (İmren et al. 2001; Eyidoğan & Güreli 2006).
The continuation of inferred faults from the sea area toward the shelf and land closely matches with location of the aligned landslides. One of the main questions is to find any activity along this high deformation zone to mitigate earthquake hazard in the region. In the past, based on available seismological catalogues, researchers assumed seismic activity in the study area (Oktay et al. 2002), but earthquake locations in the catalogues can have errors as high as 4–5 km and contain many quarry blasts as well (Eyidoğan & Güreli 2006). New catalogues from the local organizations (İnan et al. 2007) include no earthquakes for this area. However, these catalogues are based on weak station distributions and geometries. Hence, a local seismological network is needed to fill the gaps of available seismogical catalogues to catch any microseismological activity.
Strong structural anomalies in the seismic sections, particularly offshore Gürpınar part of Avcılar Peninsula, are observed. The older strata are tilted and faulted. It is also noted that the most affected zone from landslides comes across the most deformed subsurface zone offshore Avcılar district. This leads to idea that the landslides in this area may also be related to the structural complexities of the area as well as common causes of landslides. As one of the important structural anomalies, marked by seismic sections and shear wave velocity anomalies, the Çatalca Fault Zone with its zone of secondary features now constituting weakness surfaces may cause instability thus contributing to landsliding in the deformed older Miocene layers in Gürpınar region.
Another contributing mechanism of the land sliding may be the interaction of Çatalca Fault Zone and the NAF in the area. Note that the NAF is oriented in east–west direction in the area and the Çatalca Fault Zone comes towards the NAF, which may constitute a junction offshore Avcılar Peninsula. The GPS velocity field indicates a biggest deformation zone in the northern part of The Sea of Marmara (Fig. 10) as the summation of the long-term regional deformation (stress loading as the result of the possible active branches of NAF) and short-term local deformation field (e.g. landslides, substance). As an important feature, the deformations has different order of magnitude between the western and eastern part of Haramidere (Fig. 2). So, this can be marker of the extension on land and linked to the block tilting around the structural controlling valley, observed on the seismic section MCS02 (Fig. 7).
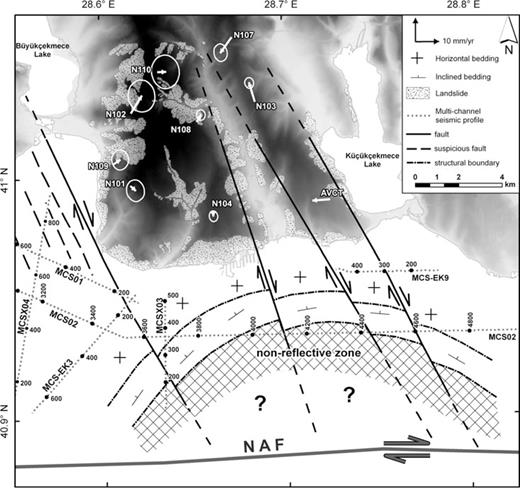
The horizontal GPS velocity field w.r.t. Eurasian Plate and the location of non-reflective zone, cut by the fault zone. Seismic lines shown on the map provide a link between the seismic sections and the structural features in this figure. Note the correlation between the locations of the heavy landslides and the fault zone.
Interpretation of seismic sections suggest that the dome structure has been cut by a series faults in the east of Çatalca Fault Zone and this pattern can be explained by the right lateral motion of NAF. Under the continuous right lateral motion along the NAF, this land deformation zone may propagate toward east through time. Fig. 10 summarizes these features. What is apparent is that this relatively extended area is further sliced down by the north–south oriented faults (determined from offshore seismic) and this interaction has led to north–south aligned heavy landslides that are observed along the east and west sides of the Avcılar peninsula and Haramidere riverside, as a localized response of the wider extended area.
5 Conclusion
We have shown that there is evidence from offshore seismic reflection data as well as geological, geodetical, geophysical land data that ongoing landsliding in the Avcılar Peninsula is interrelated to the structural framework of the area and this relation maybe more dominant as the cause of landslides than commonly believed and reported causes (e.g. slope instability, shallow groundwater level and liquefaction).
We believe that the study area, located to the north of the Main Marmara Fault of NAFZ, which seems to show aseismic character deserves further studies. Thus, future plans include establishment of dense seismological network, conducting additional GPS surveys and obtaining and processing more PSInSAR images to better reveal the activity of suspected faults and shallow deformation in the form of landslides.
Acknowledgments
The authors thank Istanbul Metropolitan Municipality and TUBITAK Marmara Research Center for the financial support. We also thank the research team in Seislab of Institute of Marine Sciences in Dokuz Eylül University (DEU) under the leaderships of the Prof. Dr. Günay Ciftçi and Assoc. Prof. Dr. Derman Dondurur for their unlimited support in data collection stage of surveys, the captains and crews of R/V Koca Piri Reis for their professional and effective assistance, which ensured the completion of the cruises under difficult conditions in very heavy marine traffic. We thank Prof. Dr. Aral Okay (İTU) for his valuable contribution and criticism. Finally, we thank the Department of Ground and Earthquake Survey of the Istanbul Metropolitan Municipality (namely, Hikmet Karaoğlu, Kemal Duran, Ahmet Emre Basmacı and Salim Gümüş) for continuous interest and support in this study.
Supporting Information
Additional Supporting Information may be found in the online version of this article:
Table S1. Table of east and north GPS velocity components (E Vel, N Vel) and 1-sigma uncertainties (Sig E, Sig N), correlation between E and N velocity components (RHO) in a Eurasia-fixed reference frame.
Figure S1. GPS velocities, in Eurasia-fixed reference frame. Dominant deformations are in our study area of the northern part of Sea of Marmara. Note that, all sites are in the Eurasian Plate.
Please note: Wiley-Blackwell are not responsible for the content or functionality of any supporting materials supplied by the authors. Any queries (other than missing material) should be directed to the corresponding author for the article.
References