-
PDF
- Split View
-
Views
-
Cite
Cite
Eveline J Bartowsky, Oenococcus oeni and the genomic era, FEMS Microbiology Reviews, Volume 41, Issue Supp_1, August 2017, Pages S84–S94, https://doi.org/10.1093/femsre/fux034
- Share Icon Share
Abstract
Oenococcus oeni is the main lactic acid bacteria species associated with grapes and wine. It is a bacterium that has adapted itself to the harsh conditions of wine, and demonstrated its importance in the production of quality wines. It has a small genome (1.8 Mb); over 200 strains have had their genome sequenced. Genomic analyses have proposed that there are two major branches of O. oeni strains that might be linked to wine style (sparkling wine versus white and red) and metagenomic studies have suggested a possible influence of terroir. This review explores recent developments of O. oeni including genomic studies examining O. oeni diversity and how this might shape future regional-specific commercial O. oeni starter strains.
INTRODUCTION
The production of alcoholic beverages dates back to well over 7000 years. The winemaking process used today is not vastly different from that used in ancient times by the Egyptians and Greeks. Yeasts are the major microorganisms involved in winemaking, catalysing the conversion of grape sugars to ethanol; however, bacteria also play an integral role in wine production (Fig. 1). Early microbiologists, such as Pasteur and Müller-Thurgau, observed the presence of bacteria in wine, and by the early 1900s the importance that they play in winemaking was beginning to be understood. Bacteria were observed in both sound and spoiled wine.
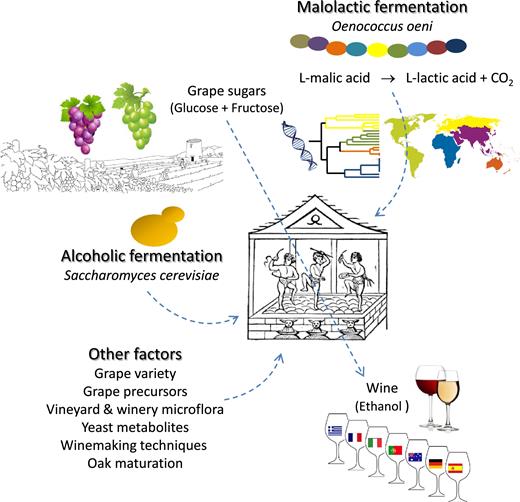
General overview of winemaking and the numerous elements influence the final wine and its regional character. It begins in the vineyard with grape variety, viticultural practices and winegrowing region. The conversion of grape sugars to ethanol/wine is yeast driven; the main species is S. cerevisiae. Malolactic fermentation is a bacteria-mediated reaction, predominantly by O. oeni. Other factors include winemaking techniques and maturation in oak.
As a fermented beverage, the bacteria associated with grapes and wine are members of the lactic acid bacteria (LAB) family and specifically conduct malolactic fermentation (MLF), the decarboxylation of L-malic acid to L-lactic acid (Lonvaud-Funel 1999). This benefits wine 3-fold: it provides microbial stability through the removal of a fermentable carbon source from the wine, reduces acidity by increasing wine pH 0.2–0.5 units and due to bacterial metabolism there are various sensory changes that can occur to the wine (Lonvaud-Funel 1999). Virtually all red wines undergo MLF and a range of white and sparkling-base wines will benefit from this bacterial driven fermentation. The preferred LAB species used in winemaking is Oenococcus oeni (formerly Leuconostoc oenos), specifically because of its ability to grow and perform in the low acidic wine environment and capacity to survive high ethanol, SO2, scarcity of nutrients and low temperature (Lonvaud-Funel 1999). Species of Lactobacillus and Pediococcus have the ability to conduct MLF; however, they are usually more associated with wine spoilage (Bartowsky 2009).
As the initiation of MLF can be unpredictable, when reliant on the indigenous microflora, the development of malolactic starter cultures (Nielsen and Richelieu 1999) has considerably improved MLF efficiency and reliability (Bartowsky 2005). Traditionally, MLF was induced with O. oeni after the yeast-driven alcoholic fermentation; however, more recently the co-inoculation of bacteria with yeast into grape juice has become a more common practice (for examples, see Semon et al.2001; Rosi, Fia and Canuti 2003; Jussier, Dubé Morneau and Mira de Orduña 2006; Zapparoli et al.2009; Pan et al.2011; Abrahamse and Bartowsky 2012).
There have been numerous recent reviews that have specifically focused on the MLF process in winemaking and the sensory impact the bacteria can have on wine, and the reader is referred to recent reviews (Bartowsky 2005; 2009; Lerm, Engelbrecht and Toit du 2010; Sumby, Grbin and Jiranek 2010; Bartowsky and Borneman 2011; Styger, Prior and Bauer 2011; Sumby, Grbin and Jiranek 2014). This review will focus specifically on recent developments around O. oeni, its role in grape vinification and the implications of recent molecular advancements (genome sequencing and metagenomics) in characterising the microbial populations. In addition, there will be a discussion on the role that O. oeni can play in other fruit wines.
HISTORICAL PERSPECTIVE OF WINE BACTERIA
Even though winemaking has been undertaken for over 7000 years and the importance of fermentation was known, it was unclear until relatively recently (about 150 years ago) that yeast and bacteria were the vital drivers. Ancient winemakers knew of the importance of the boiling during fermentation (from the Latin fevere, to boil). With the development of the microscope by Antonie van Leeuwenhoek, the first yeast were observed, albeit in beer wort. The connection between yeast, referred to as Saccharomyces (zuckerpilz, sugar fungus) for the first time in 1838 by Meyen, was finally confirmed by studies by Pasteur in 1866.
It was around this time that Pasteur described the presence of bacteria in wine, Balard recognised that the formation of lactic acid in wine was due to bacteria (Balard 1861), and at the turn of the century, Koch demonstrated that bacteria were responsible for the conversion of malic acid to lactic acid (Bartowsky 2005). Originally, the bacteria were named Bacterium gracile (Müller-Thurgau and Osterwalder 1913), later as Leuconostoc gracile (Bidan, 1956), and in other instances as L. citrovorum (Radler 1958; Kunkee, Pilone and Combs 1965), non-dextran-forming varieties of L. mesenteroides (Fornachon 1964) or combinations of L. mesenteroides, L. dextranicum and L. citrovorum (Garvie 1967). Not until an extensive study of the above strains (excluding the B. gracile strain which was no longer available) would Garvie formally classify the bacteria as L. oenos (Garvie 1967).
The final name change occurred with the advent and establishment of molecular-based techniques; L. oenos was assigned to a new genus and renamed as Oenococcus oeni (Dicks, Dellaglio and Collins 1995). Based on phylogenetic studies using 16S and 23S rRNA sequences revealed that L. oenos represents a distinct subline that is separate from other Leuconostocs spp. Oenococcus, the genus name means berry, little round thing from wine (Gr. N. oinos, wine; Gr. N. kokkus, berry) and the species as the correct Latin genitive form, oeni (Dicks, Dellaglio and Collins 1995). Oenococcus oeni is predominantly associated with grapes and wine; however, some strains have been isolated from cider (Sánchez et al.2010, 2012).
WHAT OENOCOCCUS OENI OFFERS TO WINE
The main role of O. oeni in winemaking is the deacidification of wine (Lonvaud-Funel 1999). This provides microbial stability to wine by removal of a carbon source for potential wine spoilage yeast and bacteria (Rankine and Bridson 1971; Rankine 1972). Moreover, its main function is to ‘soften’ wine and it impacts on the sensory profile of finished wines (Davis et al.1988).
Through its metabolism during growth in wine (or grape juice if co-inoculated with yeast), O. oeni is able to metabolise a diverse range of grape-, yeast- and oak-(when used) derived compounds to release secondary metabolites, which impact on wine aroma and flavour. One of the most important metabolites formed is diacetyl, which confers a buttery aroma to wine (Rankine et al.1970; Bartowsky and Henschke 2004). With a suite of enzymatic activities, O. oeni metabolism can impact of wine sensory; for example, fruity impacting esters (Davis et al.1988; Matthews, Grbin and Jiranek 2006; Costello, Francis and Bartowsky 2012; Costello et al.2013; Sumby, Jiranek and Grbin 2013), glycosidase activity to release fruity and floral aglycons (Ugliano, Genovese and Moio 2003; D’Incecco et al.2004) and oak lactones (Gagné et al.2011), production of vanillin (Bloem et al.2007), production of sulfur-containing compounds (Pripis-Nicolau et al.2004; Knoll et al.2011) and biogenic amines (Coton et al.1998; Marcobal et al.2006).
More information on the sensory influence of O. oeni on wines can be found in numerous recent reviews (Bartowsky 2005; 2009; Lerm, Engelbrecht and Toit du 2010; Sumby, Grbin and Jiranek 2010; Bartowsky and Borneman 2011; Styger, Prior and Bauer 2011; Sumby, Grbin and Jiranek 2014; Cappello et al.2017).
IMPACT OF THE GENOMIC ERA
Oenococcus oeni has a compact genome ∼1.8 Mb which undoubtedly has resulted from specialisation to the relatively narrow ecological nice of wine (Gibbons and Rinker 2015; Campbell-Sills et al.2015). The first genomic analysis using DNA restriction profiles suggested the Leuconostoc oenos genome to be 1.8–2.1 Mb in size (Lamoureux et al.1993) with the average size proposed to be 1.84 Mb (Tenreiro et al.1994) which has been substantiated from genome sequence studies (Mills et al.2005; Borneman et al.2010, 2012; Campbell-Sills et al.2015).
Understanding the relationship between O. oeni strains has been an interesting journey. Early 1990s studies, based on total soluble cell protein patterns, suggested two major groupings of L. oenos (van Vuuren and Dicks 1993). Likewise, using low-frequency restriction fragment analysis of 28 L. oenos isolates, two major groupings were found (Tenreiro et al.1994) which was in agreement with another study encompassing similar strains (Lamoureux et al.1993; Ze-Ze, Tenreiro and Paveia 2000; Ze-Ze, Chelo and Tenreiro 2008). With all the recent genomic analyses of large numbers of O. oeni strains, there still appears to be a separation of the strains into two major groups (Borneman et al.2010, 2012; Campbell-Sills et al.2015; Sternes and Borneman 2016). There are currently over 200 O. oeni strains with their genome sequenced; a summary of the origins of these strains is in Table 1.
O. oeni straina . | Origin . | Wine* . | Year of isolation . | Genome size (bp) . | G+C% . | No of predicted genes . | No. of predicted proteins . | Reference . | |
---|---|---|---|---|---|---|---|---|---|
PSU-1 | Pennsylvania, USA | Red blend | 1972 | 1780 517 | 38 | 1691 | Mills et al. (2005) | ||
ATCC BAA-1163 | Aquitaine, France | Red | 1984 | 1753 879 | ND | 1395 | ND | Unpublished | |
AWRIB429 | Abruzzi, Italy | 37.9 | 2161 | ND | Borneman et al. (2010) | ||||
AWRIB129 | France | Red | Details not providedb | Borneman et al. (2012) | |||||
AWRIB418 | Sonoma, USA | ||||||||
AWRIB419 | Cote de Rhone, France | ||||||||
AWRIB553 | Tuchan Languedoc, France | ||||||||
AWRIB548 | France | ||||||||
AWRIB422 | NP | ||||||||
AWRIB202 | Coonawarra, Australia | Cab Sauv | 1994 | ||||||
AWRIB304 | Adelaide Hills, Australia | Zinfandel | 1999 | ||||||
AWRIB318 | Merbein, Australia | ||||||||
AWRIB568 | Yarra Valley, Australia | ||||||||
AWRIB576 | Yarra Valley, Australia | ||||||||
36 strains sequenced in this study | Dimopoulou et al. (2014) | ||||||||
11 | France | Red | Details not provided | ||||||
4 | France | Sparkling | |||||||
12 | France | ||||||||
4 | Lebanon | Red | |||||||
1 | England | White | |||||||
4 | France | Cider | |||||||
OM22 | Apulia, Italy | Nero di Troia | 2007 | 1862 817 | 38 | 1895 | 1772 | Capozzi et al. (2014) | |
OT25 | 1834 661 | 37.9 | 1845 | 1731 | |||||
OT4 | 1779 962 | 37.9 | 1776 | 1654 | |||||
OT5 | 1767 097 | 37.8 | 1775 | 1655 | |||||
OT3 | 1769 724 | 37.8 | 1780 | 1658 | |||||
OM27 | Apulia, Italy | Nero di Troia | 2007 | 1786 146 | 37.9 | 1810 | 1682 | Lamontanara et al. (2014) | |
139 | Maipo Valley, Chile | Cab Sauv | 2013 | 1949 000 | 38.06 | ND | ND | Jara and Romero (2015) | |
399 | 1750 000 | 38.09 | |||||||
565 | 1755 000 | 37.71 | |||||||
X2L (CRL1947) | Northwest Argentina | Malbec | 1986 | 1812 711 | 37.90 | ND | ND | Mendoza, Saavedra and Raya (2015) | |
142 strains sequenced in this study | Sternes and Borneman (2016) | ||||||||
1 | Australia | 1970s | Details not provided | ||||||
4 | Australia | 1980s | |||||||
12 | 1980s | ||||||||
2 | Australia | 1990s | |||||||
4 | 1990s | ||||||||
16 | |||||||||
6 | Australia | ||||||||
2 | Switzerland | ||||||||
2 | USA | ||||||||
7 | Australia | Red | 1980s | ||||||
5 | Australia | Red | 1990s | ||||||
3 | Australia | Red | 2000s | ||||||
1 | Australia | White | 2002 | ||||||
1 | Australia | White | |||||||
1 | Cab Sauv | 1981 | |||||||
4 | Australia | Cab Sauv | 1980s | ||||||
14 | Australia | Cab Sauv | 2000s | ||||||
2 | Australia | Cab Sauv | 2010s | ||||||
3 | Australia | Chardonnay | 1980s | ||||||
9 | Australia | Chardonnay | 2000s | ||||||
6 | Australia | Chardonnay | 2010s | ||||||
3 | Australia | Grenache | 2004 | ||||||
2 | Australia | Merlot | 2002, 2009 | ||||||
3 | Australia | Pinot Gris | 2009 | ||||||
4 | Australia | Pinot Noir | 1980s | ||||||
2 | Australia | Pinot Noir | 2002, 2003 | ||||||
1 | Australia | Pinot Noir | 2012 | ||||||
1 | Australia | Sauv blanc | 1996 | ||||||
9 | Australia | Shiraz | 1980s | ||||||
6 | Australia | Shiraz | 2000s | ||||||
5 | Australia | Sparkling | 2009 | ||||||
1 | Australia | Viognier | 2009 |
O. oeni straina . | Origin . | Wine* . | Year of isolation . | Genome size (bp) . | G+C% . | No of predicted genes . | No. of predicted proteins . | Reference . | |
---|---|---|---|---|---|---|---|---|---|
PSU-1 | Pennsylvania, USA | Red blend | 1972 | 1780 517 | 38 | 1691 | Mills et al. (2005) | ||
ATCC BAA-1163 | Aquitaine, France | Red | 1984 | 1753 879 | ND | 1395 | ND | Unpublished | |
AWRIB429 | Abruzzi, Italy | 37.9 | 2161 | ND | Borneman et al. (2010) | ||||
AWRIB129 | France | Red | Details not providedb | Borneman et al. (2012) | |||||
AWRIB418 | Sonoma, USA | ||||||||
AWRIB419 | Cote de Rhone, France | ||||||||
AWRIB553 | Tuchan Languedoc, France | ||||||||
AWRIB548 | France | ||||||||
AWRIB422 | NP | ||||||||
AWRIB202 | Coonawarra, Australia | Cab Sauv | 1994 | ||||||
AWRIB304 | Adelaide Hills, Australia | Zinfandel | 1999 | ||||||
AWRIB318 | Merbein, Australia | ||||||||
AWRIB568 | Yarra Valley, Australia | ||||||||
AWRIB576 | Yarra Valley, Australia | ||||||||
36 strains sequenced in this study | Dimopoulou et al. (2014) | ||||||||
11 | France | Red | Details not provided | ||||||
4 | France | Sparkling | |||||||
12 | France | ||||||||
4 | Lebanon | Red | |||||||
1 | England | White | |||||||
4 | France | Cider | |||||||
OM22 | Apulia, Italy | Nero di Troia | 2007 | 1862 817 | 38 | 1895 | 1772 | Capozzi et al. (2014) | |
OT25 | 1834 661 | 37.9 | 1845 | 1731 | |||||
OT4 | 1779 962 | 37.9 | 1776 | 1654 | |||||
OT5 | 1767 097 | 37.8 | 1775 | 1655 | |||||
OT3 | 1769 724 | 37.8 | 1780 | 1658 | |||||
OM27 | Apulia, Italy | Nero di Troia | 2007 | 1786 146 | 37.9 | 1810 | 1682 | Lamontanara et al. (2014) | |
139 | Maipo Valley, Chile | Cab Sauv | 2013 | 1949 000 | 38.06 | ND | ND | Jara and Romero (2015) | |
399 | 1750 000 | 38.09 | |||||||
565 | 1755 000 | 37.71 | |||||||
X2L (CRL1947) | Northwest Argentina | Malbec | 1986 | 1812 711 | 37.90 | ND | ND | Mendoza, Saavedra and Raya (2015) | |
142 strains sequenced in this study | Sternes and Borneman (2016) | ||||||||
1 | Australia | 1970s | Details not provided | ||||||
4 | Australia | 1980s | |||||||
12 | 1980s | ||||||||
2 | Australia | 1990s | |||||||
4 | 1990s | ||||||||
16 | |||||||||
6 | Australia | ||||||||
2 | Switzerland | ||||||||
2 | USA | ||||||||
7 | Australia | Red | 1980s | ||||||
5 | Australia | Red | 1990s | ||||||
3 | Australia | Red | 2000s | ||||||
1 | Australia | White | 2002 | ||||||
1 | Australia | White | |||||||
1 | Cab Sauv | 1981 | |||||||
4 | Australia | Cab Sauv | 1980s | ||||||
14 | Australia | Cab Sauv | 2000s | ||||||
2 | Australia | Cab Sauv | 2010s | ||||||
3 | Australia | Chardonnay | 1980s | ||||||
9 | Australia | Chardonnay | 2000s | ||||||
6 | Australia | Chardonnay | 2010s | ||||||
3 | Australia | Grenache | 2004 | ||||||
2 | Australia | Merlot | 2002, 2009 | ||||||
3 | Australia | Pinot Gris | 2009 | ||||||
4 | Australia | Pinot Noir | 1980s | ||||||
2 | Australia | Pinot Noir | 2002, 2003 | ||||||
1 | Australia | Pinot Noir | 2012 | ||||||
1 | Australia | Sauv blanc | 1996 | ||||||
9 | Australia | Shiraz | 1980s | ||||||
6 | Australia | Shiraz | 2000s | ||||||
5 | Australia | Sparkling | 2009 | ||||||
1 | Australia | Viognier | 2009 |
Strain name or the number of strains sequenced in the study.
Individual strain details were not published.
*Cab Sauv—Cabernet Sauvignon; Sauv blanc—Sauvignon blanc; ND—not determined.
O. oeni straina . | Origin . | Wine* . | Year of isolation . | Genome size (bp) . | G+C% . | No of predicted genes . | No. of predicted proteins . | Reference . | |
---|---|---|---|---|---|---|---|---|---|
PSU-1 | Pennsylvania, USA | Red blend | 1972 | 1780 517 | 38 | 1691 | Mills et al. (2005) | ||
ATCC BAA-1163 | Aquitaine, France | Red | 1984 | 1753 879 | ND | 1395 | ND | Unpublished | |
AWRIB429 | Abruzzi, Italy | 37.9 | 2161 | ND | Borneman et al. (2010) | ||||
AWRIB129 | France | Red | Details not providedb | Borneman et al. (2012) | |||||
AWRIB418 | Sonoma, USA | ||||||||
AWRIB419 | Cote de Rhone, France | ||||||||
AWRIB553 | Tuchan Languedoc, France | ||||||||
AWRIB548 | France | ||||||||
AWRIB422 | NP | ||||||||
AWRIB202 | Coonawarra, Australia | Cab Sauv | 1994 | ||||||
AWRIB304 | Adelaide Hills, Australia | Zinfandel | 1999 | ||||||
AWRIB318 | Merbein, Australia | ||||||||
AWRIB568 | Yarra Valley, Australia | ||||||||
AWRIB576 | Yarra Valley, Australia | ||||||||
36 strains sequenced in this study | Dimopoulou et al. (2014) | ||||||||
11 | France | Red | Details not provided | ||||||
4 | France | Sparkling | |||||||
12 | France | ||||||||
4 | Lebanon | Red | |||||||
1 | England | White | |||||||
4 | France | Cider | |||||||
OM22 | Apulia, Italy | Nero di Troia | 2007 | 1862 817 | 38 | 1895 | 1772 | Capozzi et al. (2014) | |
OT25 | 1834 661 | 37.9 | 1845 | 1731 | |||||
OT4 | 1779 962 | 37.9 | 1776 | 1654 | |||||
OT5 | 1767 097 | 37.8 | 1775 | 1655 | |||||
OT3 | 1769 724 | 37.8 | 1780 | 1658 | |||||
OM27 | Apulia, Italy | Nero di Troia | 2007 | 1786 146 | 37.9 | 1810 | 1682 | Lamontanara et al. (2014) | |
139 | Maipo Valley, Chile | Cab Sauv | 2013 | 1949 000 | 38.06 | ND | ND | Jara and Romero (2015) | |
399 | 1750 000 | 38.09 | |||||||
565 | 1755 000 | 37.71 | |||||||
X2L (CRL1947) | Northwest Argentina | Malbec | 1986 | 1812 711 | 37.90 | ND | ND | Mendoza, Saavedra and Raya (2015) | |
142 strains sequenced in this study | Sternes and Borneman (2016) | ||||||||
1 | Australia | 1970s | Details not provided | ||||||
4 | Australia | 1980s | |||||||
12 | 1980s | ||||||||
2 | Australia | 1990s | |||||||
4 | 1990s | ||||||||
16 | |||||||||
6 | Australia | ||||||||
2 | Switzerland | ||||||||
2 | USA | ||||||||
7 | Australia | Red | 1980s | ||||||
5 | Australia | Red | 1990s | ||||||
3 | Australia | Red | 2000s | ||||||
1 | Australia | White | 2002 | ||||||
1 | Australia | White | |||||||
1 | Cab Sauv | 1981 | |||||||
4 | Australia | Cab Sauv | 1980s | ||||||
14 | Australia | Cab Sauv | 2000s | ||||||
2 | Australia | Cab Sauv | 2010s | ||||||
3 | Australia | Chardonnay | 1980s | ||||||
9 | Australia | Chardonnay | 2000s | ||||||
6 | Australia | Chardonnay | 2010s | ||||||
3 | Australia | Grenache | 2004 | ||||||
2 | Australia | Merlot | 2002, 2009 | ||||||
3 | Australia | Pinot Gris | 2009 | ||||||
4 | Australia | Pinot Noir | 1980s | ||||||
2 | Australia | Pinot Noir | 2002, 2003 | ||||||
1 | Australia | Pinot Noir | 2012 | ||||||
1 | Australia | Sauv blanc | 1996 | ||||||
9 | Australia | Shiraz | 1980s | ||||||
6 | Australia | Shiraz | 2000s | ||||||
5 | Australia | Sparkling | 2009 | ||||||
1 | Australia | Viognier | 2009 |
O. oeni straina . | Origin . | Wine* . | Year of isolation . | Genome size (bp) . | G+C% . | No of predicted genes . | No. of predicted proteins . | Reference . | |
---|---|---|---|---|---|---|---|---|---|
PSU-1 | Pennsylvania, USA | Red blend | 1972 | 1780 517 | 38 | 1691 | Mills et al. (2005) | ||
ATCC BAA-1163 | Aquitaine, France | Red | 1984 | 1753 879 | ND | 1395 | ND | Unpublished | |
AWRIB429 | Abruzzi, Italy | 37.9 | 2161 | ND | Borneman et al. (2010) | ||||
AWRIB129 | France | Red | Details not providedb | Borneman et al. (2012) | |||||
AWRIB418 | Sonoma, USA | ||||||||
AWRIB419 | Cote de Rhone, France | ||||||||
AWRIB553 | Tuchan Languedoc, France | ||||||||
AWRIB548 | France | ||||||||
AWRIB422 | NP | ||||||||
AWRIB202 | Coonawarra, Australia | Cab Sauv | 1994 | ||||||
AWRIB304 | Adelaide Hills, Australia | Zinfandel | 1999 | ||||||
AWRIB318 | Merbein, Australia | ||||||||
AWRIB568 | Yarra Valley, Australia | ||||||||
AWRIB576 | Yarra Valley, Australia | ||||||||
36 strains sequenced in this study | Dimopoulou et al. (2014) | ||||||||
11 | France | Red | Details not provided | ||||||
4 | France | Sparkling | |||||||
12 | France | ||||||||
4 | Lebanon | Red | |||||||
1 | England | White | |||||||
4 | France | Cider | |||||||
OM22 | Apulia, Italy | Nero di Troia | 2007 | 1862 817 | 38 | 1895 | 1772 | Capozzi et al. (2014) | |
OT25 | 1834 661 | 37.9 | 1845 | 1731 | |||||
OT4 | 1779 962 | 37.9 | 1776 | 1654 | |||||
OT5 | 1767 097 | 37.8 | 1775 | 1655 | |||||
OT3 | 1769 724 | 37.8 | 1780 | 1658 | |||||
OM27 | Apulia, Italy | Nero di Troia | 2007 | 1786 146 | 37.9 | 1810 | 1682 | Lamontanara et al. (2014) | |
139 | Maipo Valley, Chile | Cab Sauv | 2013 | 1949 000 | 38.06 | ND | ND | Jara and Romero (2015) | |
399 | 1750 000 | 38.09 | |||||||
565 | 1755 000 | 37.71 | |||||||
X2L (CRL1947) | Northwest Argentina | Malbec | 1986 | 1812 711 | 37.90 | ND | ND | Mendoza, Saavedra and Raya (2015) | |
142 strains sequenced in this study | Sternes and Borneman (2016) | ||||||||
1 | Australia | 1970s | Details not provided | ||||||
4 | Australia | 1980s | |||||||
12 | 1980s | ||||||||
2 | Australia | 1990s | |||||||
4 | 1990s | ||||||||
16 | |||||||||
6 | Australia | ||||||||
2 | Switzerland | ||||||||
2 | USA | ||||||||
7 | Australia | Red | 1980s | ||||||
5 | Australia | Red | 1990s | ||||||
3 | Australia | Red | 2000s | ||||||
1 | Australia | White | 2002 | ||||||
1 | Australia | White | |||||||
1 | Cab Sauv | 1981 | |||||||
4 | Australia | Cab Sauv | 1980s | ||||||
14 | Australia | Cab Sauv | 2000s | ||||||
2 | Australia | Cab Sauv | 2010s | ||||||
3 | Australia | Chardonnay | 1980s | ||||||
9 | Australia | Chardonnay | 2000s | ||||||
6 | Australia | Chardonnay | 2010s | ||||||
3 | Australia | Grenache | 2004 | ||||||
2 | Australia | Merlot | 2002, 2009 | ||||||
3 | Australia | Pinot Gris | 2009 | ||||||
4 | Australia | Pinot Noir | 1980s | ||||||
2 | Australia | Pinot Noir | 2002, 2003 | ||||||
1 | Australia | Pinot Noir | 2012 | ||||||
1 | Australia | Sauv blanc | 1996 | ||||||
9 | Australia | Shiraz | 1980s | ||||||
6 | Australia | Shiraz | 2000s | ||||||
5 | Australia | Sparkling | 2009 | ||||||
1 | Australia | Viognier | 2009 |
Strain name or the number of strains sequenced in the study.
Individual strain details were not published.
*Cab Sauv—Cabernet Sauvignon; Sauv blanc—Sauvignon blanc; ND—not determined.
Analyses of 50 O. oeni strains based on various phylogentic and phylogenomic reconstructions using MLST (multilocus sequence typing), Tetra (genomic signature of tetranucleotides by Tetra algorithm), SNP (single nucleotide polymorphism) and ANIm (average nucleotide identity [ANI] algorithm by MUMmer alignment) demonstrated two major groupings (Campbell-Sills et al.2015). From this study, a third putative group was proposed comprising one strain, intriguingly isolated from cider (Bridier et al.2010) An earlier study of 258 diversely sourced O. oeni strains, using MLST, distinct region-specific populations of O. oeni were observed (Bridier et al.2010). In addition to being able to group these O. oeni strains according to wine style (red and white wines versus sparkling wines), when a neighbor-joining phylogenic tree was constructed from the concatenated sequence of the seven housekeeping genes, two major phylogenetic groups formed in congruence with the studies described above. However, a single O. oeni strain (DIV 5.7), isolated from cider, composed a third group (Bridier et al.2010). This O. oeni strain is the same O. oeni cider isolate as genome sequenced in the Campbell-Sills study (IOEB_C52) (Campbell-Sills et al.2015).
Sternes and Borneman's study of 191 O. oeni strains (including the 50 strains from the Campbell-Sills study) was based on pan genome (invariant [core] and variable [flexible] regions) analysis, proposes only two broad genetic groups, with the putative third branch being the smaller second branch of their group B; this branch now comprises 12 strains (including the 1 strain from the Campbell-Sills study) (Sternes and Borneman 2016).
The separation of O. oeni strains into two distinct groups has interesting associations for MLF. All the O. oeni strains are clearly capable of conducting the malic acid to lactic acid conversion; however, it is the efficiency of the reaction that is most important to winemakers. A study which used MLST analysis in conjunction with MLF performance of 21 O. oeni strains showed that the best MLF performing strains were present in both groups (Bordas et al.2013), suggesting that O. oeni strain grouping based on housekeeping genes is not directly related to adaptation and ethanol tolerance. Other genetic sequences have been found to be more indicative of MLF performance; significant association was observed between high-performing strains and the presence of eight stress-responsive genes (Bon et al.2009). In addition, alleles (L and H) of the rpoB gene separated O. oeni strains that were prevalent in grape must and early in fermentation (L allele) from those strains present later and were more resistant to post-fermentation stress (Renouf et al.2009). Furthermore, there was a higher diversity amongst the L group strains which were prevalent early in fermentation, whereas there was higher homogeneity within the H group of strains which predominated only during ageing. Additionally to support this, grape must O. oeni isolates have been shown to be more metabolically diverse (higher carbohydrate fermentation diversity) than wine isolates (Cruz-Pio et al.2017). The separation of O. oeni strains into two distinct groups based on MLF efficiency is further supported by separation of strains on performance in red or white wine which present different challenges to O. oeni strains (Khoury El et al.2017).
One of O. oeni’s qualities is its ability to impact on wine aroma due to a suite of enzymatic activities. However, the presence of an enzyme or metabolite pathway is not enough to guarantee the production of an influential aroma compound. The wine matrix (grape variety) and winemaking conditions exert a myriad of influences on the metabolism of O.oeni during MLF. For example, studies on citrate metabolism, which leads to diacetyl formation responsible for buttery aroma, emphasised that different conditions (ethanol and pH) affect the transcriptional response of citrate pathway genes; ethanol had a greater influence than pH (Olguín, Bordons and Reguant 2009). This highlights the interplay of the O. oeni cell adapting to wine stress (ethanol and pH) and gene transcription levels within the citrate pathway leading to diacetyl production and potentially buttery wines. In addition, there is variation in the ability of different O. oeni strains to produce diacetyl (Martineau and Henick-Kling 1995; Bartowsky and Henschke 2004); therefore, this type of gene transcriptional analyses needs to be undertaken with multiple O. oeni strains.
Ultimately from a wine perspective it is the MLF performance and metabolite synthesis which will determine whether a strain is used in winemaking. The consistent production of a berry-fruity wine following MLF has been shown to be due to an O. oeni strain which has additional glycosidase genes (Borneman et al.2010; Bartowsky and Borneman 2011) and higher production ability of ethyl esters (ethyl hexanoate and ethyl octanoate) (Costello et al.2013).
Genome annotation and comparisons between O. oeni strains have thus far focused on carbohydrate metabolism (Cibrario et al.2016), amino acid biosynthesis and sugar transport and utilisation (Sternes and Borneman 2016), and exopolysaccharide synthesis (Dimopoulou et al.2014) which provide an insight into growth and survival in wine, however not the sensory impact of O. oeni on wine. The next step will be to use the genome information to investigate other pathways that lead to aroma important metabolites, an in-depth analysis of differences and potential of metabolite synthesis by O. oeni strains. Such studies will require the ability to find connections between genome, metabolite production and concentration, sensory analyses and wine composition. With over 200 O. oeni genome sequences now available, this opens the door to studies which can utilise this information and use techniques including microarrays and RNA-seq to assess gene transcriptional patterns especially in red and white wine matrices.
Geographical origin
Wine regional identity has always been important for ‘old world’ wine regions. Characteristic wine style will be determined by grape cultivar, geology, climate and viticultural and winemaking practices which all will contribute to the wine regional identity (Bartowsky, Costello and Chambers 2014). There is also mounting evidence that the local, indigenous, bacterial microflora contribute to a wine's terroir (see e.g. Gilbert, van der Lelie and Zarraonaindia 2014; Zarraonaindia et al.2015), and thus there is a growing interest in the application of regional malolactic bacteria (Capozzi and Spano 2011).
Analysis of the genetic variation in indigenous LAB communities of different grape varieties in various geographical locations has generated much interest over the last 10 years. For example, one study of Tempranillo wines from 10 different La Rioja wineries over three vintages suggested that there is an endemic microbiota for the region (González-Arenzana et al.2013) and similarly for Tempranillo wines produced in Castilla-la-Mancha wineries over three vintages (González-Arenzana et al.2015). In the same way, genomic diversity of 121 O. oeni strains isolated from three winemaking regions in Portugal was examined using M13 PCR fingerprint analysis; a high level of intraspecific diversity was observed and the diversity could be partitioned according to the geographical origin of the O. oeni isolates (Marques et al.2011).
Numerous studies have investigated the genetic polymorphism of indigenous O. oeni strains within a specific region or grape variety. Examples from different countries (Australia, China, France, Germany, Greece, Italy, Portugal, Spain) are shown in Table 2. All these studies demonstrate the diversity of O. oeni strains and that strains isolated from different regions within a country cluster together according to wine region. In addition, the majority of the studies grouped their strains into two major branches, a theme which is confirmed with whole genome studies.
Studies that have examined the genetic polymorphism of indigenous O. oeni strains within a specific region or grape variety.
Wine region . | Grape variety . | Reference . |
---|---|---|
Australia | ||
Adelaide Hills, Barossa Valley, Clare Valley, Eden Valley, Margaret River, Yarra Valley | Chardonnay, Cabernet Sauvignon, Sauvignon blanc, Shiraz, Zinfandel | Bartowsky, McCarthy and Henschke (2003) |
China | ||
Six regions, not specified | Various red varieties (not specified) | Wang et al. (2015) |
Yueqiannian | Red | Liu (2014) |
France | ||
Bordeaux | Various red varieties (not specified) | Delaherche et al. (2006); Renouf et al. (2009) |
Aquitaine, Burgundy, Languedoc-Roussillon, Val de Loire | Red and white (variety not specified | Khoury El et al. (2017) |
Germany | ||
Ahr, Mosel-Saar-Ruwer, Rheingau, Rheinhessen, Saale/Unstrut, Württemberg, | Various (not specified) | Larisika, Claus and König (2008) |
Greece | ||
Peloponnese, Naoussa, Goumenissa, Crete, Attica, Khalkidhiki, Larisa, Kavala, | Cabernet Sauvignon, Merlot, Syrah, Xinomavro, Kotsifali, Agiorgitiko, Refosco d.p. Rosso, Hamburg Muscatel | Pramateftaki et al. (2012) |
Italy | ||
Apulia | Primitivo | Cappello et al. (2008) |
Apulia | Nero di Troia | Capozzi et al. (2010) |
Apulia | Negroamaro | Cappello et al. (2014) |
Apulia | Uva di Troia | Garofalo et al. (2015) |
Basilicata | Aglianico | Lechiancole et al. (2006); Cafaro et al. (2016) |
Salento region, Apulia | Malvasia Nero | Cappello et al. (2010) |
Valtellina | Red | Vigentini et al. (2009) |
Four regions (not specified) | Variety not specified | Solieri et al. (2009) |
Portugal | ||
Alentejo, Dão, Ribatejo | Various (not specified) | Marques et al. (2011) |
Spain | ||
Castilla-La Mancha | Cencibel | Ruiz et al. (2008) |
Castilla-La-Mancha | Tempranillo | Ruiz et al. (2010); González-Arenzana et al. (2015) |
Catalonia (Priorat, Tarragona) | Grenache, Cabernet Sauvignon, Carignan, Syrah, Merlot | Bordas et al. (2013) |
La Rioja | Tempranillo | González-Arenzana et al. (2013, 2015) |
Méntrida | Cabernet Sauvignon, Garnacha Merlot, Syrah, Tempranillo | Pérez-Martín, Seseña and Palop (2014) |
Wine region . | Grape variety . | Reference . |
---|---|---|
Australia | ||
Adelaide Hills, Barossa Valley, Clare Valley, Eden Valley, Margaret River, Yarra Valley | Chardonnay, Cabernet Sauvignon, Sauvignon blanc, Shiraz, Zinfandel | Bartowsky, McCarthy and Henschke (2003) |
China | ||
Six regions, not specified | Various red varieties (not specified) | Wang et al. (2015) |
Yueqiannian | Red | Liu (2014) |
France | ||
Bordeaux | Various red varieties (not specified) | Delaherche et al. (2006); Renouf et al. (2009) |
Aquitaine, Burgundy, Languedoc-Roussillon, Val de Loire | Red and white (variety not specified | Khoury El et al. (2017) |
Germany | ||
Ahr, Mosel-Saar-Ruwer, Rheingau, Rheinhessen, Saale/Unstrut, Württemberg, | Various (not specified) | Larisika, Claus and König (2008) |
Greece | ||
Peloponnese, Naoussa, Goumenissa, Crete, Attica, Khalkidhiki, Larisa, Kavala, | Cabernet Sauvignon, Merlot, Syrah, Xinomavro, Kotsifali, Agiorgitiko, Refosco d.p. Rosso, Hamburg Muscatel | Pramateftaki et al. (2012) |
Italy | ||
Apulia | Primitivo | Cappello et al. (2008) |
Apulia | Nero di Troia | Capozzi et al. (2010) |
Apulia | Negroamaro | Cappello et al. (2014) |
Apulia | Uva di Troia | Garofalo et al. (2015) |
Basilicata | Aglianico | Lechiancole et al. (2006); Cafaro et al. (2016) |
Salento region, Apulia | Malvasia Nero | Cappello et al. (2010) |
Valtellina | Red | Vigentini et al. (2009) |
Four regions (not specified) | Variety not specified | Solieri et al. (2009) |
Portugal | ||
Alentejo, Dão, Ribatejo | Various (not specified) | Marques et al. (2011) |
Spain | ||
Castilla-La Mancha | Cencibel | Ruiz et al. (2008) |
Castilla-La-Mancha | Tempranillo | Ruiz et al. (2010); González-Arenzana et al. (2015) |
Catalonia (Priorat, Tarragona) | Grenache, Cabernet Sauvignon, Carignan, Syrah, Merlot | Bordas et al. (2013) |
La Rioja | Tempranillo | González-Arenzana et al. (2013, 2015) |
Méntrida | Cabernet Sauvignon, Garnacha Merlot, Syrah, Tempranillo | Pérez-Martín, Seseña and Palop (2014) |
Studies that have examined the genetic polymorphism of indigenous O. oeni strains within a specific region or grape variety.
Wine region . | Grape variety . | Reference . |
---|---|---|
Australia | ||
Adelaide Hills, Barossa Valley, Clare Valley, Eden Valley, Margaret River, Yarra Valley | Chardonnay, Cabernet Sauvignon, Sauvignon blanc, Shiraz, Zinfandel | Bartowsky, McCarthy and Henschke (2003) |
China | ||
Six regions, not specified | Various red varieties (not specified) | Wang et al. (2015) |
Yueqiannian | Red | Liu (2014) |
France | ||
Bordeaux | Various red varieties (not specified) | Delaherche et al. (2006); Renouf et al. (2009) |
Aquitaine, Burgundy, Languedoc-Roussillon, Val de Loire | Red and white (variety not specified | Khoury El et al. (2017) |
Germany | ||
Ahr, Mosel-Saar-Ruwer, Rheingau, Rheinhessen, Saale/Unstrut, Württemberg, | Various (not specified) | Larisika, Claus and König (2008) |
Greece | ||
Peloponnese, Naoussa, Goumenissa, Crete, Attica, Khalkidhiki, Larisa, Kavala, | Cabernet Sauvignon, Merlot, Syrah, Xinomavro, Kotsifali, Agiorgitiko, Refosco d.p. Rosso, Hamburg Muscatel | Pramateftaki et al. (2012) |
Italy | ||
Apulia | Primitivo | Cappello et al. (2008) |
Apulia | Nero di Troia | Capozzi et al. (2010) |
Apulia | Negroamaro | Cappello et al. (2014) |
Apulia | Uva di Troia | Garofalo et al. (2015) |
Basilicata | Aglianico | Lechiancole et al. (2006); Cafaro et al. (2016) |
Salento region, Apulia | Malvasia Nero | Cappello et al. (2010) |
Valtellina | Red | Vigentini et al. (2009) |
Four regions (not specified) | Variety not specified | Solieri et al. (2009) |
Portugal | ||
Alentejo, Dão, Ribatejo | Various (not specified) | Marques et al. (2011) |
Spain | ||
Castilla-La Mancha | Cencibel | Ruiz et al. (2008) |
Castilla-La-Mancha | Tempranillo | Ruiz et al. (2010); González-Arenzana et al. (2015) |
Catalonia (Priorat, Tarragona) | Grenache, Cabernet Sauvignon, Carignan, Syrah, Merlot | Bordas et al. (2013) |
La Rioja | Tempranillo | González-Arenzana et al. (2013, 2015) |
Méntrida | Cabernet Sauvignon, Garnacha Merlot, Syrah, Tempranillo | Pérez-Martín, Seseña and Palop (2014) |
Wine region . | Grape variety . | Reference . |
---|---|---|
Australia | ||
Adelaide Hills, Barossa Valley, Clare Valley, Eden Valley, Margaret River, Yarra Valley | Chardonnay, Cabernet Sauvignon, Sauvignon blanc, Shiraz, Zinfandel | Bartowsky, McCarthy and Henschke (2003) |
China | ||
Six regions, not specified | Various red varieties (not specified) | Wang et al. (2015) |
Yueqiannian | Red | Liu (2014) |
France | ||
Bordeaux | Various red varieties (not specified) | Delaherche et al. (2006); Renouf et al. (2009) |
Aquitaine, Burgundy, Languedoc-Roussillon, Val de Loire | Red and white (variety not specified | Khoury El et al. (2017) |
Germany | ||
Ahr, Mosel-Saar-Ruwer, Rheingau, Rheinhessen, Saale/Unstrut, Württemberg, | Various (not specified) | Larisika, Claus and König (2008) |
Greece | ||
Peloponnese, Naoussa, Goumenissa, Crete, Attica, Khalkidhiki, Larisa, Kavala, | Cabernet Sauvignon, Merlot, Syrah, Xinomavro, Kotsifali, Agiorgitiko, Refosco d.p. Rosso, Hamburg Muscatel | Pramateftaki et al. (2012) |
Italy | ||
Apulia | Primitivo | Cappello et al. (2008) |
Apulia | Nero di Troia | Capozzi et al. (2010) |
Apulia | Negroamaro | Cappello et al. (2014) |
Apulia | Uva di Troia | Garofalo et al. (2015) |
Basilicata | Aglianico | Lechiancole et al. (2006); Cafaro et al. (2016) |
Salento region, Apulia | Malvasia Nero | Cappello et al. (2010) |
Valtellina | Red | Vigentini et al. (2009) |
Four regions (not specified) | Variety not specified | Solieri et al. (2009) |
Portugal | ||
Alentejo, Dão, Ribatejo | Various (not specified) | Marques et al. (2011) |
Spain | ||
Castilla-La Mancha | Cencibel | Ruiz et al. (2008) |
Castilla-La-Mancha | Tempranillo | Ruiz et al. (2010); González-Arenzana et al. (2015) |
Catalonia (Priorat, Tarragona) | Grenache, Cabernet Sauvignon, Carignan, Syrah, Merlot | Bordas et al. (2013) |
La Rioja | Tempranillo | González-Arenzana et al. (2013, 2015) |
Méntrida | Cabernet Sauvignon, Garnacha Merlot, Syrah, Tempranillo | Pérez-Martín, Seseña and Palop (2014) |
Wine regional identity is continually being explored. For example, the genome of five autochthonous O. oeni strains isolated from the same terroir (spontaneous MLF in Nero di Trioa wine) have been sequenced (Capozzi et al.2014). These five strains fall within Group A of the study by Sternes and Borneman (2016) but in two different sections of the group: two identical strains within the large cluster of very closely related strains and the other three identical strains in a different branch of Group A. Similarly, three Chilean isolates from the Maipo Valley have had their genomes sequenced (Jara and Romero 2015): two strains (identical) fall within Group A and the third strain in Group B (Sternes and Borneman 2016). This highlights the diversity of O. oeni strains within a region, which in turn contribute to regional character.
From a different stance, a study of 95 O. oeni strains isolated from Apulian Negroamaro wine throughout MLF showed that the strains divided into two main clusters and these were associated with stage of MLF when the strains were isolated; most of the O. oeni grouped in cluster A were isolated at the beginning of MLF, whereas those in cluster B were isolated at the middle and at the end of MLF (Cappello et al.2014). Similar speculation was proposed with Australian O. oeni isolates, where a large proportion of the Australian isolates were found to cluster closely together (Sternes and Borneman 2016). Even though little was known about the stage of MLF the strains were isolated from, one hypothesis was that they represent a robust variety that is capable of outcompeting other O. oeni strains during fermentation. This hypothesis is consistent with the rpoB allele (L and H) segregating dependent on its prevalence during grape vinification (early or late fermentation) (Renouf et al.2009). Similarly, O. oeni fitness (i.e. MLF performance) was shown to be linked to a set of gene sequences; a significant positive association was observed between high-performing strains and the presence of eight gene sequences (Bon et al.2009).
MLST of large collections of O. oeni strains isolated from various geographical locations have not only demonstrated the presence of two groupings, as discussed above, but also possibly the subdivision into subgroups linked to specific regions, such as Chile and South Africa (Bilhère et al.2009; Bridier et al.2010). Another study which examined over 500 O. oeni strains isolated from six different regions and wines (red and white, and cider) highlighted the complexity of regionality; each region had a high diversity of strains that constituted a unique population (Khoury El et al.2017). Strains present in each region belonged to diverse phylogenetic groups and these groups of strains were also detected in different regions. Yet, within the Burgundy region there was greater O. oeni strain identity, with strains grouping according to product (cider, white or red wine) suggesting genetic adaptation to these beverage styles.
Studies examining large sets of O. oeni strains isolated from one wine region have demonstrated similarity between their specific group of strains. Bokulich et al. (2016a), using the wine grape microbiome with the metabolome and fermentation behavior, have proposed that there is a microbial contribution to regional wine characteristics. Over 200 commercial fermentations (Chardonnay and Cabernet Sauvignon) were surveyed within Napa and Sonoma Counties, California, and the authors demonstrated that grape and wine microbiota exhibit regional patterns that correlate with chemical composition, suggesting that the grape microbiome may influence terroir. This is mirrored by the observations from a French study of over 500 O. oeni strains that each region ‘holds a patchwork of strains that belong to different genetic groups’ (Khoury El et al.2017). Consequently, terroir requires a unique combination of vineyard microorganisms (Bokulich et al.2014), wine yeasts (Knight et al.2015) and O. oeni diversity. It is this microbial diversity that contributes to wine regional character.
Whole-genome sequence analysis of O. oeni strains has begun to provide an insight into the evolution and adaptation of the species to its ecological niche, wine, with indications of ancestral O. oeni strains adapting to low ethanol environments (e.g. overripe fruits) and domestication to wine and even to specific wines such as champagne (Campbell-Sills et al.2015). As the diversity of regional O. oeni strains is examined in greater depth, such as mining the pan genome for differences in metabolite metabolism, we will be able to further define regional terroir. Such future analyses will be very interesting and have great potential in the development of regional-specific strains or suites of strains to augment regional wine character.
Metagenomics studies
The use of genome sequencing has provided an amazing insight into the similarities and differences of O. oeni strains; however, this has been dependent on the ability to isolate and culture the strains. As a bacterium, O. oeni is fastidious and slow growing, thus often making isolation and cultivation difficult (Garvie 1967). Improved molecular profiling methods have enabled a much better understanding of the microbial ecology of wine fermentation, which in the past has been dependent on culture-based techniques, slow throughput of a high number of samples and low resolution of early molecular ecology techniques (Bokulich et al.2014). With advancements in sequencing technologies (for example, next-generation sequencing and 454 pyrosequencing), much more extensive studies of fermentation communities have been possible, a metagenomic approach to investigating the diversity of yeast and bacteria (Bokulich et al.2012; Bokulich and Mills 2012). These types of studies have highlighted the complexity of the microbiology of grape fermentation. For example, the bacterial biodiversity in botrytised wine (Bokulich et al.2012), the complex relationship between microbial communities in grape musts and wine fermentations and linking to wine composition ((Bokulich et al.2016b), and that regional, site-specific and grape variety factors can shape the yeast and bacterial consortia inhabiting wine-grape surfaces (Bokulich et al.2014). Interestingly, these metagenomic studies following the evolution of yeast and bacteria through fermentation, not unexpectedly, show the same type of growth and decline profile as observed with traditional culturing methodologies (Fleet, Lafon-Lafourcade and Ribéreau-Gayon 1984).
This metagenomic approach to scrutinise the microbiota of food fermentations has led to unexpected species being identified by their genome sequence. For example, a recent phylogenetic analysis of spontaneous cocoa bean fermentation has indicated the presence of O. oeni in this type of fermentation (Illeghems et al.2012). The percentage of metagenomic DNA identified as O. oeni was 0.0%–1.8%, which is somewhat equivalent or higher than other low ranking species (for example, L. reuteri [0.0–2.6] or L. rhamnosus [0.0–0.2] compared with L. fermentum [9.3–96.8]). Though the presence of O. oeni has not been previously observed using culture-dependent methods (personal communication, De Vuyst), perhaps in the future, culturable O. oeni strains will be isolated. Alternatively, detecting the presence of O. oeni by genome comparison in this type of fermentation is an anomaly of this type of methodology.
Oenococcus oeni is usually more associated with high alcohol and acidic environments than what is experienced in cocoa fermentations (Lonvaud-Funel 1999). It will be interesting in the future, with more metagenomic studies on fermented foods, to see whether O. oeni is detected in other low alcohol fermentation environments.
OTHER FRUIT WINES AND OENOCOCCUS OENI
Oenococcus oeni has almost exclusively been isolated from Vitis vinifera grapes and wine (Garvie 1967; Dicks, Dellaglio and Collins 1995; Lonvaud-Funel 1999). To a much lesser extent, O. oeni strains have been isolated from apple juice and cider (Sánchez et al.2010, 2012) with one reference to isolation from stone fruit mash and perry (pear cider) (Bridier et al.2010).
Other Vitis grape varieties that have a high malic acid content benefit from MLF conducted by O. oeni. Wines made from the French-American hybrid Chambourcin grapes (V. vinifera and American vine species) were shown to benefit from O. oeni induced MLF, both for acid reduction and overall sensory profile (Homich et al.2016). Similarly, O. oeni inoculation with Saccharomyces cerevisiae of Cynthiana grape juice (V. aestivalis Michx.) improved the wine quality (Main and Morris 2004). However, there is no indication in the literature that O. oeni strains have been isolated from these varieties.
The use of O. oeni in cider making is varied. As mentioned above, O. oeni strains have been isolated from apple cider and their genetic relationship with grape-wine isolated strains has been studied (Bridier et al.2010); even though only a limited number of strains have been examined, there appears to be a tendency of cider-isolated O. oeni strains to group close to each other. Inoculation with O. oeni strains during cider production has been used as a means to mainly deacidify the cider (e.g. Herrero et al.2001, 2005; Reuss et al.2010). However, O. oeni has also been used in a limited number of other fruit wines to initiate and conduct MLF; predominantly these fruit wines have low acidity, due to high malic acid content. High malic acid containing sea buckthorn wines (7–61 g/L) were considered more drinkable through MLF conducted by O. oeni strains (Tiitinen et al.2005, 2006). Oenococcus oeni inoculation of black and white currant juices and bilberry juice successfully reduced the malic acid content and decreased the acidity to more palatable levels (Viljakainen and Laakso 2002). However, in the same study, O. oeni did not grow or conduct MLF successfully in lingonberry juice, due to high concentrations of benzoic acid, which is especially inhibitory at low pH, as is the case with lingonberry juice. The sensory quality of cherry wines was shown to be improved by the co-inoculation (bacteria are inoculated for MLF at the same time as yeast for alcoholic fermentation) of O. oeni strains (Sun et al.2013).
Another example of the use of O. oeni in fruit winemaking is in the improvement of the sensory quality of durian wine, where O. oeni-driven MLF was utilised more for sensory impact than its reduction of acidity; an increase of higher alcohols, acetate and ethyl esters conferred a positive sensory impact and was found to be a novel way of modulating the volatile and aroma profile of durian wine (Taniasuri, Lee and Liu 2016; Lu et al.2017). Similarly, a recent study in lychee wine has shown the use of O. oeni as a means of retaining lychee character, which is often lost with yeast fermentation (Chen and Liu 2016). By utilising O. oeni glycosidase activity (Grimaldi, Bartowsky and Jiranek 2005; Ugliano et al.2006; Gagné et al.2011) during lychee winemaking, the release of glycosidically bound aroma compounds resulted in wines with elevated concentrations of key lychee aroma impacting terpenes and monoterpenes (linalool, geraniol and cis-rose oxide). Additionally, the quality of mango wine has been shown to improve through the use MLF with O. oeni (Varakumar et al.2013). MLF was efficient in both co-inoculation and sequentially inoculation, completely metabolising malic acid within 8 and 10 days, respectively. These mango wines exhibited better sensorial attributes in flavor, fruity aroma and overall acceptability, especially when using a co-inoculation strategy.
These limited number of studies in exotic berry and novel fruit wines have revealed the beneficial use of O. oeni metabolism in improving and enhancing sensory qualities of fruit wines, yet it is does not appear that conducting MLF in non-grape winemaking processes is a common practice (Rupasinghe and Clegg 2007). However, there have been limited attempts to isolate O. oeni strains from these types of fruit wines. Metagenomic studies of these fruit fermentations would provide an insight into the microbial diversity and may provide a pathway to identifying O. oeni strains associated with these other fruits and ascertaining their relationship with grape and cider originating strains.
CONCLUSIONS
Oenococcus oeni is a fastidious bacterium with a small genome that has adapted itself to the harsh conditions of wine, and demonstrated its importance in the production of quality wines. It is interesting to see the use of O. oeni and MLF in non-grape wine production and what this bacterium can offer to these fruit wines. Classical and genome analysis has suggested two major branches of O. oeni strains that appear to be linked to wine style (sparkling wine versus white and red). Characterisation of large numbers of regional O. oeni strains has shown the importance of strain diversity within a region or winery and contribution to terroir. Future ongoing investigations into diversity of O. oeni will be very interesting in the next few years. Such studies will undoubtedly be of interest in the development of regional-specific commercial O. oeni starter strains.
Conflict of interest. None declared.