-
PDF
- Split View
-
Views
-
Cite
Cite
Matthew C. Swearingen, Alex C. DiBartola, Devendra Dusane, Jeffrey Granger, Paul Stoodley, 16S rRNA analysis provides evidence of biofilms on all components of three infected periprosthetic knees including permanent braided suture, Pathogens and Disease, Volume 74, Issue 7, October 2016, ftw083, https://doi.org/10.1093/femspd/ftw083
- Share Icon Share
Bacterial biofilms are the main etiological agent of periprosthetic joint infections (PJI); however, it is unclear if biofilms colonize one or multiple components. Because biofilms can colonize a variety of surfaces, we hypothesized that biofilms would be present on all components. 16S ribosomal RNA (rRNA) gene sequencing analysis was used to identify bacteria recovered from individual components and non-absorbable suture material recovered from three PJI total knee revision cases. Bray–Curtis non-metric multidimensional scaling analysis revealed no significant differences in similarity when factoring component, material type, or suture versus non-suture material, but did reveal significant differences in organism profile between patients (P < 0.001) and negative controls (P < 0.001). Confocal microscopy and a novel agar encasement culturing method also confirmed biofilm growth on a subset of components. While 16S sequencing suggested that the microbiology was more complex than revealed by culture contaminating, bacterial DNA generates a risk of false positives. This report highlights that biofilm bacteria may colonize all infected prosthetic components including braided suture material, and provides further evidence that clinical culture can fail to sufficiently identify the full pathogen profile in PJI cases.
INTRODUCTION
Although the incidence of periprosthetic joint infection (PJI) in total hip and total knee arthroplasties (TKA) is relatively low at 2.0% and 2.4%, respectively (Kurtz et al.2012), PJI is a devastating complication for both the patient and surgeon requiring an arduous course of explantation, antibiotic treatment and re-implantation (Zmistowski et al.2013; Shahi and Parvizi 2015). Lima et al. (2013) and others have shown that Gram-positive bacteria predominate in cases of PJI, involving Staphylococcus aureus and S. epidermidis (Frommelt 2006; Trampuz et al.2007; Del Pozo and Patel 2009; Parvizi 2009; Tornero 2012; Lima et al.2013; Osmon et al.2013). However, a growing body of evidence based on bacterial culture and nucleic acid detection techniques suggests that PJIs commonly involve bacterial biofilms and are often polymicrobial (Tunney et al.1999; Bauer et al.2006; Gomez et al.2012; Evangelopoulos et al.2013; McConoughey et al.2014; Tzeng et al.2015). Bacteria growing in the biofilm phenotype are recalcitrant to antibiotics (Dastgheyb et al.2015), and often fail to grow in culture using routine clinical methods (Costerton et al.2011; Corvec et al.2012; Ehrlich et al.2014; McConoughey et al.2014; Dastgheyb et al.2015; Edmiston et al.2015; Konig et al.2015; Tzeng et al.2015).
In this work, we microbiologically examined individual prosthetic components recovered from three overtly infected prosthetic knee joints to determine (i) the extent of biofilm colonization and (ii) whether specific components or materials might be more prone to colonization or differ in pathogen profile. We also analyzed pieces of permanent polyester braided suture material that were associated with respective cases. Indeed, suture has been shown to harbor biofilms in chronically infected hernia repairs (Kathju et al.2009, 2010a,b; Edmiston et al.2015). We employed PCR coupled with agarose gel and densitometry analysis to determine the presence of bacterial DNA associated with the clinical samples. 16S rRNA gene high-throughput sequencing was performed in order to analyze the pathogen profile and the similarity of said profiles for individual components from three patients undergoing revision for clinically diagnosed periprosthetic infections. Sterile saline used in the operating room (OR) was rinsed in the sterile collection container to control for contaminating bacterial DNA. In addition, we used two methods to confirm biofilm growth on specimens including (i) novel agar encasement culturing method (AECM) to confirm in situ outgrowth from implant material (Stoodley et al.2011), and (ii) confocal laser scanning microscopy (CLSM) (Kathju et al.2009).
MATERIALS AND METHODS
Consent, patient histories and implant retrieval
Patient consent and all specimens were obtained in accordance with Institutional Review Board-approved protocol no. 2013H0353 at The Ohio State University Wexner Medical Center (OSUWMC). The orthopedic materials (OMs) used for research were explanted from patients suffering post-operative infection following primary total knee arthroplasty. All intraoperative clinical cultures taken as part of routine surgical care at OSUWMC are grown on 5% sheep blood agar, chocolate agar, MacConkey agar and thioglycolate broth and are held for 24 h at 35°C. In addition, all intraoperative clinical anaerobic cultures are plated on Schaedler's agar with Vitamin K and 5% sheep blood, CDC (Centers for Disease Control and Prevention) Anaerobe Blood with phenylethyl alcohol (PEA), and CDC Anaerobe Laked Blood Agar with Kanamycin/Vancomycin and are held for 14 days at 35°C. Following surgical explantation in the OR, total knee replacement components were placed in new sterile plastic containers and transported to the research lab in a cooler. The samples were processed immediately on arrival. The various components are shown in Table 1.
Explanted material composition of three different periprosthetic joint infected cases (PJIs). PJI- periprosthetic joint infection, CoCrMo- cobalt chromium molybdenum, TiAlV- titanium aluminium vanadium, UHMWPE- ultra-high molecular weight polyethylene, 316L SS- 316L stainless steel.
PJI-1 . | Material Composition . |
---|---|
Femoral | CoCrMo |
Tibial | TiAlV |
Suture | Polyethylene |
Patellar surface | UHMWPE |
Articulating surface | UHMWPE |
PJI-2 | |
Suture | Polyethylene |
Bone Cement Spacer | PMMA |
PJI-3 | |
Femoral | CoCrMo |
Tibial | TiAlV |
Suture | Polyethylene |
Patellar surface | UHMWPE |
Articulating surface | UHMWPE |
Screws | 316L SS |
PJI-1 . | Material Composition . |
---|---|
Femoral | CoCrMo |
Tibial | TiAlV |
Suture | Polyethylene |
Patellar surface | UHMWPE |
Articulating surface | UHMWPE |
PJI-2 | |
Suture | Polyethylene |
Bone Cement Spacer | PMMA |
PJI-3 | |
Femoral | CoCrMo |
Tibial | TiAlV |
Suture | Polyethylene |
Patellar surface | UHMWPE |
Articulating surface | UHMWPE |
Screws | 316L SS |
Explanted material composition of three different periprosthetic joint infected cases (PJIs). PJI- periprosthetic joint infection, CoCrMo- cobalt chromium molybdenum, TiAlV- titanium aluminium vanadium, UHMWPE- ultra-high molecular weight polyethylene, 316L SS- 316L stainless steel.
PJI-1 . | Material Composition . |
---|---|
Femoral | CoCrMo |
Tibial | TiAlV |
Suture | Polyethylene |
Patellar surface | UHMWPE |
Articulating surface | UHMWPE |
PJI-2 | |
Suture | Polyethylene |
Bone Cement Spacer | PMMA |
PJI-3 | |
Femoral | CoCrMo |
Tibial | TiAlV |
Suture | Polyethylene |
Patellar surface | UHMWPE |
Articulating surface | UHMWPE |
Screws | 316L SS |
PJI-1 . | Material Composition . |
---|---|
Femoral | CoCrMo |
Tibial | TiAlV |
Suture | Polyethylene |
Patellar surface | UHMWPE |
Articulating surface | UHMWPE |
PJI-2 | |
Suture | Polyethylene |
Bone Cement Spacer | PMMA |
PJI-3 | |
Femoral | CoCrMo |
Tibial | TiAlV |
Suture | Polyethylene |
Patellar surface | UHMWPE |
Articulating surface | UHMWPE |
Screws | 316L SS |
Case 1: PJI-1
The patient was a 66-year-old male who originally underwent primary total knee replacement performed at an outside hospital (OSH). Prior to revision, the patient underwent irrigation and debridement (IAD), followed by ultra-high-molecular-weight polyethylene (UHMWPE) articular surface (AS) exchange. Intraoperative cultures taken during the exchange tested positive for Escherichia coli and an Enterococcus species. However, the patient remained febrile with a draining wound, and was subsequently transferred to OSUWMC for operative hardware and non-absorbable suture removal. During the explant operation, a total of two packs of bone cement, each with 4 g of antibiotic evenly divided between tobramycin and vancomycin, were used to create a temporary bone cement spacer that was placed in the patients wound. Intraoperative cultures taken during explantation were positive for Bacteroides fragilis, and a follow-up visit aspirate was positive for Staphylococcus aureus. The patient had an arduous course during the 12 month period following his operation that included wound dehiscence, medial gastrocnemius flap with split-thickness skin graft, operative drainage and debridement of a loculated abscess, bone cement spacer exchange and sepsis. However, at a most recent follow-up, the patient was free of signs and symptoms consistent with infection and continues to ambulate with cane assistance and minimal pain. The patient remains implant free, but the bone cement spacer remains in place.
Case 2: PJI-2
The patient was a 65-year-old male with bilateral knee replacements who was transferred to OSUWMC from an OSH with acute sepsis. The patient had a prolonged course of bilateral knee implant-associated infection for ∼three weeks, and two blood cultures and two knee aspirates tested positive for Streptococcus agalactiae. A bilateral explantation of both knees, with placement of antibiotic-loaded (poly) methyl methacrylate (PMMA) bone cement spacers in the joints, was performed at an OSH. An arthroscopic IAD was performed three days later. However, the patient continued to remain symptomatic with fevers and swelling. At OSUWMC, a computerized tomography scan demonstrated bilateral infections of both wrists and hands. Bilateral wrist and hand IAD was performed at OSUWMC and intraoperative cultures tested positive for S. epidermidis. His PMMA bone cement spacer and suture was explanted at OSUWMC several days after the bilateral wrist and hand IAD due to declining health. Unfortunately, the patient died due to overwhelming infection ∼3 months from the first diagnosis of infection.
Case 3: PJI-3
The patient was a 61-year-old male with a prolonged infection of his total knee replacement that began several weeks after his initial implant surgery. He continued to have infection of this prosthesis with intermittent drainage, which was managed with oral antibiotics for 3 months. However, ∼4 months after implantation, the wound opened and an IAD was performed at an OSH where the draining wound was confirmed to communicate with the knee joint. At OSUWMC, he was taken to the OR for stage one of a two-stage revision procedure. Intraoperative cultures were positive for S. aureus. Explanted hardware was collected at this time, and a mobile antibiotic-loaded PMMA bone cement spacer was placed. He was discharged on continuous nafcillin (narrow spectrum β-lactam of the penicillin antibiotic class) for6 weeks. The second revision stage was uneventful and the patient remains complication free.
Specimen collection
On explantation, the components were immediately placed inside a sterile plastic collection vessel, sealed and then transported to the research lab for processing at ambient temperature. Processing of the specimens had begun within 30 min of explantation.
AECM for in situ growth of biofilm
We used a novel AECM to encase retrieved components in agar to confirm biofilm as outgrowth of colonies from the surface of the components taken from case PJI-1 as described previously (Stoodley et al.2011). The explanted materials were first separated into sterile specimen containers and rinsed twice with gentle agitation in an adequate volume of sterile Dulbecco's phosphate-buffered saline (Gibco, Thermo Fisher Scientific, Waltham, MA, USA) to remove loosely adhered host tissue surgical debris, blood and planktonic bacteria. The first two rinsates were discarded. A third vigorous shaking rinse was saved for DNA extraction. Sonication was not used for total biofilm removal since we wanted to assay in situ biofilm growth on the specimens. The explanted materials were placed in individual sterile glass beakers and gently flooded with 40°C molten brain-heart infusion (BHI) agar (BD Difco, Sparks, MD, USA) via a plastic serological pipette. We filled from the bottom avoiding dispensing the agar directly onto the specimen. The suture specimens were encased agar in sterile 80 mm petri dishes. After solidification of the agar, specimens were incubated in a humidified chamber at 37°C + 5% CO2 for up to 12 days to allow for bacterial outgrowth of slow growing species. The large volumes of agar used in this technique had an advantage in that it was less prone to drying and therefore more conducive for extended incubations. Growth was evidenced as colonies on component surfaces, or as spherical colonies in the body of the agar (presumably from bacteria that dislodged from the surface during the encasement process). Colony growth was documented by digital photography.
Identification of cultures isolated from agar encasement
Bacterial isolates were picked from within the agar using a sterile toothpick and re-streaked on agar plates. The strains were identified for Gram character using Gram-staining method (Coico 2005) and using EnteroPluri test (BD Difco), as per manufacturer's instructions. Briefly, pure isolates from the plate were transferred to BHI broth and incubated at 37°C for 24 h. Ten microliter culture was inoculated onto the sections of the EnteroPluri test strips and the strips were incubated further for 24 h at 37°C. The bacterial identification was done based on change in color of the culture media in the different sectors of EnteroPluri test strips. Sterile BHI medium was used as control.
Identification of bacterial isolates based on Sanger sequencing
Selected bacterial isolates from AECM were sequenced inhouse using 16S rRNA gene analyses. Briefly, eubacterial primers (forward primer 27F 5′-AGAGTTTGATCMTGGCTCAG-3′ (Lane 1991) and 907R reverse primer 5′-CCGTCAATTCMTTTRAGTTT-3′ (Muyzer et al.1995)) were used to amplify the 16S variable region of the isolates using a colony stab as template DNA. An aliquot of each reaction from respective isolates was verified via gel electrophoresis against a no-template control. The remaining portion of the PCR products were purified using a QIAquick PCR purification kit (Qiagen, Valencia, CA, USA) and eluted in 100 μL of water. Aliquots of each reaction were sent to Plant–Microbe Genome Facility at The Ohio State University for Sanger sequencing using both 27F and 907R primers (so as to amplify in both directions). DNA template and primer mixes were combined with 1 μL BigDye Terminator v3.1 cycle sequencing kit (Thermo Fisher, Waltham, MA, USA) and 1.5 μL dilution buffer and thermal cycled using following conditions: 95°C for 1 min followed by 95°C for 10 s, 50°C for 5 s and 60°C for 4 min, 45 cycles were performed and finally a 10°C hold. The sequencing reactions were then purified with HighPrep DTR (MagBio Genomics, MD, USA) beads on a Beckman–Coulter FX liquid handling system (Beckman Coulter Life Sciences, Indianapolis, IN, USA). Purified reactions were sequenced on a Applied Biosystems 3730 (Thermo Fisher) using a 2 kV/30 sec injection. The FASTA sequences generated were queried in the ribosomal database project (Cole et al.2014).
Confocal microscopy of PJI-1 suture material
Some fragments of suture were preserved for CLSM as previously described (Kathju et al.2009). Briefly, after rinsing in phosphate-buffered saline (PBS), the sutures were preserved in 4% paraformaldehyde in PBS overnight at 4°C, and subsequently preserved in 50% ethanol in PBS at –20°C the following day. We aseptically cut 1–2 mm sections of suture with a razor blade and fixed them to the bottom of a petri dish with 1% agar. The specimens were pipette-spot stained with 1 μL of either propidium iodide (PI, red) or SYTO 9 (green) nucleic acid stain from Molecular Probes® BacLight kit (Life Technologies, Grand Island, NY, USA). For some specimens, we stained with a combination of PI and 1 μL of wheat germ agglutinin-Alexa Fluor® 488 (WGA488, green). WGA is a lectin specific for poly-N-acetyl glucosamine (pNAG), a component of extracellular polymeric slime (EPS) produced by ica+ staphylococci (Cramton et al.1999). After 15 min of incubation, excess stain was rinsed away with sterile PBS, and the sutures were flooded with sterile PBS so they could be imaged in a fully hydrated state. For imaging, we used an upright Olympus FV1000 MPE Laser Scanning Confocal microscope (Center Valley, PA, USA) using either a 60× (N.A. 0.90) or 100× (N.A. 1.0) water immersion objective lens. Metamorph® 3D (Metamorph Premier v 7.7.6.0, Molecular Devices, Sunnyvale, CA, USA) and Imaris 8.0.1 (Bitplane, Zurich, Switzerland) were used for image processing and analysis. The underlying suture material was visualized using transmitted light.
Bacterial DNA extractions from orthopedic components
Each component was rinsed twice in an appropriate volume of PBS depending on the size of the component (between 100 and 500 mL) to remove host tissue debris and any loosely adhered or planktonic bacteria. The third rinsates were concentrated via centrifugation at 5000× g for 20 min to 2 mL total volume, then pelletized at 13 000× g for 30 s. The pellet was resuspended with 1% Triton-X 100 in PBS and incubated at RT for 10 min to promote lysis of host cells and reduce human DNA. The rinsates were re-concentrated into a pellet and the supernatant was discarded. The pellet was washed with 1 mL of PBS, centrifuged and resuspended in 500 μL of bacterial genomic DNA lysis buffer (bioWORLD, Dublin, OH, USA). Proteinase K (25 μL; Sigma-Aldrich, St. Louis, MO, USA) at 10 mg/mL concentration was added to each DNA extract and incubated overnight at 37°C (Mariani et al.1995). The following day, samples were subjected to bead beating with 0.1 mm sterile nuclease-free zirconia/silica beads (BioSpec Products, Bartlesville, OK, USA). The lysates were spun at 2000× g for 30 s to remove cell debris, and specimen extracts were applied to a GenElute Genomic DNA preparation column (Sigma) and purified as per manufacturer's instructions. The genomic DNA was eluted in 100 μL of UltraPure DNase/RNase-Free distilled water (Invitrogen, Carlsbad, CA, USA), and DNA concentrations were quantified using a Nanodrop spectrophotometer (Thermo Fisher Scientific). Samples were shipped on ice to Molecular Research DNA (Shallowater, TX, USA; www.mrdnalab.com) for sequencing.
PCR negative control
Negative controls (negative control 1–4) for possible bacterial DNA present in collection vessels, saline and extraction materials was performed. These included 1 L of sterile unused OR water (Baxter, Deerfield, IL, USA), sterile unused suture material (Ethicon, Somerville, NJ, USA), sterile unused OR gloves (Biogel, Norcross, GA, USA) and a sterile unused but capped OR marking pen, all combined in a large sterile OR container similar to the one used for specimen collection. The contents were swirled for 1 min at RT prior to DNA extraction. Samples were shipped on ice to Molecular Research DNA (Shallowater, TX, USA; www.mrdnalab.com) for Illumina MiSeq sequencing.
Relative quantification of bacterial DNA by agarose gel densitometry analysis
In order to determine the relative abundance (RA) of bacterial DNA in the patients and control samples, PCR was performed using eubacterial universal primers. The forward primer 27F 5′-AGAGTTTGATCMTGGCTCAG-3′ (Lane 1991) and 907R reverse primer 5′-CCGTCAATTCMTTTRAGTTT-3′ were used (Muyzer et al.1995). PCR was performed in 25 μL reactions containing 50 nmol each of two primers and 23 μL of PCR supermix (Invitrogen). The reactions received 1 μL of DNA preparation as template. PCR was performed with 30 cycles of 94°C for 30 s, 55°C for 30 s and 72°C for 1 min followed by a hold sequence at 4°C. Aliquots taken from reactions at the final cycle were electrophoresed on 1.2% agarose gel at 20 volts/cm for 30 min and stained with 1 μg/mL ethidium bromide, added to the agarose. Gel electrophoresis images were captured with a BioRad gel documentation system, and the densitometry feature of Thermo Scientific (myImage Analysis software version 2) was used to measure band intensities. The relative intensities of specific bands were calculated by reference to the GeneRuler (1 kb) Plus DNA ladder from the molecular weight marker.
16S rRNA gene microbial sequencing using Illumina MiSeq
Bacterial DNA extracted from the clinical samples and negative controls were sequenced using an Illumina MiSeq sequencing platform (Illumina, San Diego, CA, USA) and conducted at Molecular Research DNA (Shallowater, TX, USA; www.mrdnalab.com). DNA library preparation was performed by PCR amplification of the V1–V3 region of 16S rRNA gene, using modified universal primers 27F (5′-AGRGTTTGATCMTGGCTCAG) and 519R (5′-GTNTTACNGCGGCKGCTG) (Myer et al.2016). A 30 cycle PCR was run using the HotStarTaq Plus Master Mix Kit (Qiagen) in the following reaction: 94°C for 3 min, followed by 28 cycles of 94°C for 30 s, 53°C for 40 s, 72°C for 1 min and a final 5 min elongation at 72°C. Amplification was confirmed by gel electrophoresis. After amplification, PCR products were checked in 2% agarose gel to determine the success of amplification and relative intensity of bands. Multiple samples were pooled together in equal proportions based on their molecular weight and DNA concentrations. Pooled samples were purified using calibrated Ampure XP beads and the pooled, purified PCR product was used to prepare Illumina DNA library. Sequencing was performed at MR DNA (www.mrdnalab.com; Shallowater, TX, USA) on an Illumina MiSeq following the manufacturer's guidelines. Sequence data were processed using MR DNA analysis pipeline (MR DNA, Shallowater, TX, USA). The sequences were depleted of barcodes, sequences <150 bp or with ambiguous base calls were removed, operational taxonomic units (OTUs) were generated with de-noised sequences, and sequence chimeras were removed (Dowd et al.2008; Kumar et al.2011; Swanson et al.2011). OTUs were defined by clustering at 3% divergence (97% similarity), and the final OTUs were taxonomically classified using BLAST nucleotide against a curated GreenGenes database (DeSantis et al.2006).
Statistical analysis
Primer-E Ltd. v6 (Ivybridge, UK) was used for statistical analysis of the 16S rRNA gene data for genus and species identification to assess similarity of the microbiology profiles between the various patient components. A rank-abundance analysis was used to determine the total OTU per specimen, and then the RA of species. Bray–Curtis non-metric multidimensional scaling analysis (MDS), with accompanying stress test (50 iterations each), was then used to determine the similarity of bacterial profiles between three factors: (i) patients and control, (ii) general component material (metal versus plastic) and (3) suture versus non-suture material. The level of similarity was determined from the genera and species present and their abundance measured as OTUs. Analysis of similarity (ANOSIM) multivariate data analysis was used to determine if the bacterial profile of any one component was statistically different from the others as a group. A P-value of <0.05 was considered to represent a statistically significant difference in all statistical tests. Primer 6 was also used for non-metric MDS analysis and the data presented as 2D and 3D scatter plots to show clustering of the data with similarity contours.
RESULTS
AECM for mapping biofilm from PJI-1
Colony forming unit (CFU) outgrowth on the femoral component surface was observed after 48 h incubation (Fig. 1). The CFUs were flat white colonies of variable size, with no obvious variation. The CFU outgrowth on the femoral component was heterogeneously distributed on the surface (Fig. 1A). We also observed patchy ivory-colored bacterial outgrowth from the sutures 12 days post-incubation occurring from both knotted and non-knotted locations (Fig. 1B). There was no obvious outgrowth from the surface of the femoral component or the titanium tibial tray. However, we enumerated 9.7 × 103 (±1.5 × 103) and 1.4 × 104 (± 2.1 × 103) CFU in the bulk agar for the femoral component and tibial tray, respectively. We were unable to distinguish outgrowth from the white-colored UHMWPE components because of the lack of contrast with the agar.
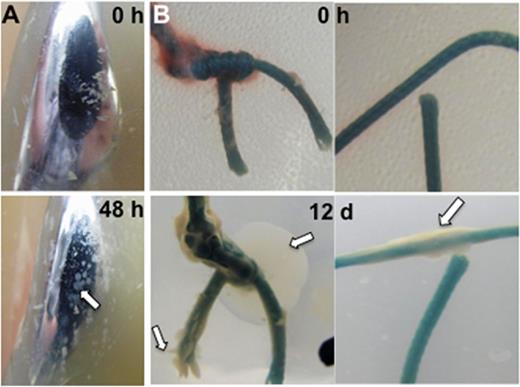
Biofilm outgrowth on infected explanted materials. (A) Anterior face of femoral component, (B) braided suture; 0 h–0 h of growth, 48 h–48 h of growth, white arrow indicates bacterial outgrowth.
We selected four bacterial isolates from AECM of the femoral component and a fifth isolate from the suture material for 16S rRNA gene analyses (Wang et al.2007). All four bacterial isolates from the femoral component were most similar (>99% identity) to Staphylococcus aureus (K16-13). The fifth isolate from a suture had an unknown sequence identity (<77% similarity), but a 24 h metabolic Enteropluri test identified it as Stenotrophomonas maltophilia. Failure to identify Steno maltophilia via 16S analysis may be due to polymicrobial growth patterns in these AECM colonies.
Confocal microscopy of braided permanent suture material from PJI-1
Dual staining with PI and WGA488 revealed adherent biofilms of cocci aggregates contoured between the individual suture filaments making up the braids. The cocci (red) were surrounded by green WGA488-stained halos consistent with the staining of a staphylococcal biofilm with pNAG in the EPS (Fig. 2A and B). In a separate specimen, we identified a small cluster of bacterial rods of ∼1 μM in length (Fig. 2C).
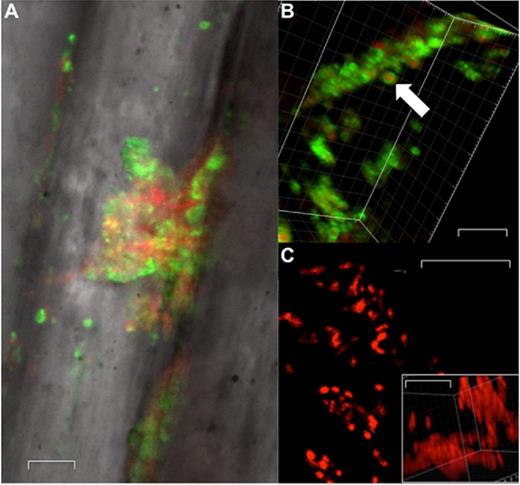
Confocal microscopy of biofilm on representative suture material. (A) Confocal image at ×63 magnification, (B) WGA488 stain of biofilm component of S. aureus at ×100 magnification, (C) 2D lateral image projection of suture (×100 magnification, ×6 optical zoom) with DNA stain, white arrow indicates bacterial cell. Inset image represents a 3D image stack of the same filed. Scale bars represent 5 μm.
Bacterial 16S rRNA gene microbiology of orthopedic components
We wanted to determine the organism profile associated with each specimen recovered from the three cases. To do this, we performed 16S sequence analysis of the total DNA extracts prepared from individual components for each case. A commonly used genetic marker for culture-independent characterization of microbial consortia is the 16S rRNA hypervariable V1–V3 region. This hypervariable region (V1–V3) allows species identification and comparison of complex clinical samples (Zheng et al.2015; Myer et al.2016). We consistently observed organisms that were grown in clinical culture which had OTUs ≥ 0.3% RA out of the entire patient's 16S data for all pooled explanted material. Thus, we used 0.3% or above for the cut-off of species OTU data to present. RA percent was calculated by dividing the OTU counts of individual bacterial species on individual components or controls by the total OTUs for the entire sequencing results of that specific component or control.
Bacterial species identification
Table 2 demonstrates the percent OTUs for each species over 0.3% RA for each component. Among the most common species present for PJI-1, Bacteroides fragilis was the most abundant comprising 85% of the two Gram-negative organisms, Pseudomonas aeruginosa and Stenotrophomonas Maltophilia represented the second most abundant OTUs at 8% and 2.5%, respectively. Oxalobacter spp. represented 2.1% of the pooled OTUs among components in this case (PJI-1). Staphylococcus aureus, which was also identified via direct clinical culture (GenBank accession KX364052.1 (www.ncbi.nlm.nih.gov/nuccore/KX364052.1)), represented 0.35% of OTUs. Therefore, the genus and species results for pooled total OTUs among all components in PJI-1 correlated.
RA of bacteria identified at species level. RA of species per orthopedic material specimen per case and of four negative controls. PJI—periprosthetic joint infection. Total richness represents the sum of OTUs for the patients sequencing analysis. ‘*’ indicates species that were cultured clinically.
PJI-1 . | Suture . | Femoral . | Tibial . | Patellar . | Articulating surface . | Bone cement spacer . | Tibial screws . |
---|---|---|---|---|---|---|---|
. | (Su) . | (FC) . | (TC) . | (P) . | (AS) . | (BCS) . | (TS) . |
B. fragilis* | 66.82% | 98.75% | 92.24% | 88.42% | 87.54% | ||
P. aeruginosa | 23.41% | 0.38% | 2.97% | 4.38% | 11.36% | ||
Steno. maltophilia* | 1.17% | 0.87% | 4.30% | 6.83% | 0.60% | ||
S. aureus* | 8.12% | 0.00% | 0.01% | 0.01% | 0.02% | ||
Oxalobacter spp. | 0.48% | 0.00% | 0.48% | 0.36% | 0.47% | ||
PJI-2 | Suture | Femoral | Tibial | Patellar | Articulating surface | Bone cement spacer | Tibial screws |
(Su) | (FC) | (TC) | (P) | (AS) | (BCS) | (TS) | |
P. aeruginosa | 99.15% | 0.21% | |||||
Strep. agalactiae* | 0.04% | 95.11% | |||||
S. aureus | 0.78% | 1.50% | |||||
Prop. acnes | 0.02% | 2.03% | |||||
S. epidermidis* | 0.00% | 1.14% | |||||
PJI-3 | Suture | Femoral | Tibial | Patellar | Articulating surface | Bone cement spacer | Tibial screws |
(Su) | (FC) | (TC) | (P) | (AS) | (BCS) | (TS) | |
S. aureus* | 96.77% | 26.72% | 63.59% | 85.70% | 99.03% | 97.96% | |
P. aeruginosa | 2.84% | 73.05% | 36.15% | 13.97% | 0.66% | 1.73% | |
B. fragilis | 0.39% | 0.23% | 0.27% | 0.33% | 0.31% | 0.31% | |
Negative control | |||||||
P. tolaasii | 37.00% | ||||||
Prop. acnes | 22.61% | ||||||
V. paradoxus | 8.22% | ||||||
R. pickettii | 7.15% | ||||||
Strep. thermophilus | 6.92% | ||||||
R. insidiosa | 3.64% | ||||||
Staphylococcus spp. | 3.15% | ||||||
P. putida | 2.72% | ||||||
Stenotrophomonas spp. | 2.39% | ||||||
Achromobacter xylosoxidans | 1.71% | ||||||
Propionibacterium spp. | 1.15% | ||||||
Acinetobacter johnsonii | 1.14% | ||||||
Delftia spp. | 1.11% | ||||||
Hydrogenophilus spp. | 0.7% | ||||||
Rubrobacter spp. | 0.4% |
PJI-1 . | Suture . | Femoral . | Tibial . | Patellar . | Articulating surface . | Bone cement spacer . | Tibial screws . |
---|---|---|---|---|---|---|---|
. | (Su) . | (FC) . | (TC) . | (P) . | (AS) . | (BCS) . | (TS) . |
B. fragilis* | 66.82% | 98.75% | 92.24% | 88.42% | 87.54% | ||
P. aeruginosa | 23.41% | 0.38% | 2.97% | 4.38% | 11.36% | ||
Steno. maltophilia* | 1.17% | 0.87% | 4.30% | 6.83% | 0.60% | ||
S. aureus* | 8.12% | 0.00% | 0.01% | 0.01% | 0.02% | ||
Oxalobacter spp. | 0.48% | 0.00% | 0.48% | 0.36% | 0.47% | ||
PJI-2 | Suture | Femoral | Tibial | Patellar | Articulating surface | Bone cement spacer | Tibial screws |
(Su) | (FC) | (TC) | (P) | (AS) | (BCS) | (TS) | |
P. aeruginosa | 99.15% | 0.21% | |||||
Strep. agalactiae* | 0.04% | 95.11% | |||||
S. aureus | 0.78% | 1.50% | |||||
Prop. acnes | 0.02% | 2.03% | |||||
S. epidermidis* | 0.00% | 1.14% | |||||
PJI-3 | Suture | Femoral | Tibial | Patellar | Articulating surface | Bone cement spacer | Tibial screws |
(Su) | (FC) | (TC) | (P) | (AS) | (BCS) | (TS) | |
S. aureus* | 96.77% | 26.72% | 63.59% | 85.70% | 99.03% | 97.96% | |
P. aeruginosa | 2.84% | 73.05% | 36.15% | 13.97% | 0.66% | 1.73% | |
B. fragilis | 0.39% | 0.23% | 0.27% | 0.33% | 0.31% | 0.31% | |
Negative control | |||||||
P. tolaasii | 37.00% | ||||||
Prop. acnes | 22.61% | ||||||
V. paradoxus | 8.22% | ||||||
R. pickettii | 7.15% | ||||||
Strep. thermophilus | 6.92% | ||||||
R. insidiosa | 3.64% | ||||||
Staphylococcus spp. | 3.15% | ||||||
P. putida | 2.72% | ||||||
Stenotrophomonas spp. | 2.39% | ||||||
Achromobacter xylosoxidans | 1.71% | ||||||
Propionibacterium spp. | 1.15% | ||||||
Acinetobacter johnsonii | 1.14% | ||||||
Delftia spp. | 1.11% | ||||||
Hydrogenophilus spp. | 0.7% | ||||||
Rubrobacter spp. | 0.4% |
RA of bacteria identified at species level. RA of species per orthopedic material specimen per case and of four negative controls. PJI—periprosthetic joint infection. Total richness represents the sum of OTUs for the patients sequencing analysis. ‘*’ indicates species that were cultured clinically.
PJI-1 . | Suture . | Femoral . | Tibial . | Patellar . | Articulating surface . | Bone cement spacer . | Tibial screws . |
---|---|---|---|---|---|---|---|
. | (Su) . | (FC) . | (TC) . | (P) . | (AS) . | (BCS) . | (TS) . |
B. fragilis* | 66.82% | 98.75% | 92.24% | 88.42% | 87.54% | ||
P. aeruginosa | 23.41% | 0.38% | 2.97% | 4.38% | 11.36% | ||
Steno. maltophilia* | 1.17% | 0.87% | 4.30% | 6.83% | 0.60% | ||
S. aureus* | 8.12% | 0.00% | 0.01% | 0.01% | 0.02% | ||
Oxalobacter spp. | 0.48% | 0.00% | 0.48% | 0.36% | 0.47% | ||
PJI-2 | Suture | Femoral | Tibial | Patellar | Articulating surface | Bone cement spacer | Tibial screws |
(Su) | (FC) | (TC) | (P) | (AS) | (BCS) | (TS) | |
P. aeruginosa | 99.15% | 0.21% | |||||
Strep. agalactiae* | 0.04% | 95.11% | |||||
S. aureus | 0.78% | 1.50% | |||||
Prop. acnes | 0.02% | 2.03% | |||||
S. epidermidis* | 0.00% | 1.14% | |||||
PJI-3 | Suture | Femoral | Tibial | Patellar | Articulating surface | Bone cement spacer | Tibial screws |
(Su) | (FC) | (TC) | (P) | (AS) | (BCS) | (TS) | |
S. aureus* | 96.77% | 26.72% | 63.59% | 85.70% | 99.03% | 97.96% | |
P. aeruginosa | 2.84% | 73.05% | 36.15% | 13.97% | 0.66% | 1.73% | |
B. fragilis | 0.39% | 0.23% | 0.27% | 0.33% | 0.31% | 0.31% | |
Negative control | |||||||
P. tolaasii | 37.00% | ||||||
Prop. acnes | 22.61% | ||||||
V. paradoxus | 8.22% | ||||||
R. pickettii | 7.15% | ||||||
Strep. thermophilus | 6.92% | ||||||
R. insidiosa | 3.64% | ||||||
Staphylococcus spp. | 3.15% | ||||||
P. putida | 2.72% | ||||||
Stenotrophomonas spp. | 2.39% | ||||||
Achromobacter xylosoxidans | 1.71% | ||||||
Propionibacterium spp. | 1.15% | ||||||
Acinetobacter johnsonii | 1.14% | ||||||
Delftia spp. | 1.11% | ||||||
Hydrogenophilus spp. | 0.7% | ||||||
Rubrobacter spp. | 0.4% |
PJI-1 . | Suture . | Femoral . | Tibial . | Patellar . | Articulating surface . | Bone cement spacer . | Tibial screws . |
---|---|---|---|---|---|---|---|
. | (Su) . | (FC) . | (TC) . | (P) . | (AS) . | (BCS) . | (TS) . |
B. fragilis* | 66.82% | 98.75% | 92.24% | 88.42% | 87.54% | ||
P. aeruginosa | 23.41% | 0.38% | 2.97% | 4.38% | 11.36% | ||
Steno. maltophilia* | 1.17% | 0.87% | 4.30% | 6.83% | 0.60% | ||
S. aureus* | 8.12% | 0.00% | 0.01% | 0.01% | 0.02% | ||
Oxalobacter spp. | 0.48% | 0.00% | 0.48% | 0.36% | 0.47% | ||
PJI-2 | Suture | Femoral | Tibial | Patellar | Articulating surface | Bone cement spacer | Tibial screws |
(Su) | (FC) | (TC) | (P) | (AS) | (BCS) | (TS) | |
P. aeruginosa | 99.15% | 0.21% | |||||
Strep. agalactiae* | 0.04% | 95.11% | |||||
S. aureus | 0.78% | 1.50% | |||||
Prop. acnes | 0.02% | 2.03% | |||||
S. epidermidis* | 0.00% | 1.14% | |||||
PJI-3 | Suture | Femoral | Tibial | Patellar | Articulating surface | Bone cement spacer | Tibial screws |
(Su) | (FC) | (TC) | (P) | (AS) | (BCS) | (TS) | |
S. aureus* | 96.77% | 26.72% | 63.59% | 85.70% | 99.03% | 97.96% | |
P. aeruginosa | 2.84% | 73.05% | 36.15% | 13.97% | 0.66% | 1.73% | |
B. fragilis | 0.39% | 0.23% | 0.27% | 0.33% | 0.31% | 0.31% | |
Negative control | |||||||
P. tolaasii | 37.00% | ||||||
Prop. acnes | 22.61% | ||||||
V. paradoxus | 8.22% | ||||||
R. pickettii | 7.15% | ||||||
Strep. thermophilus | 6.92% | ||||||
R. insidiosa | 3.64% | ||||||
Staphylococcus spp. | 3.15% | ||||||
P. putida | 2.72% | ||||||
Stenotrophomonas spp. | 2.39% | ||||||
Achromobacter xylosoxidans | 1.71% | ||||||
Propionibacterium spp. | 1.15% | ||||||
Acinetobacter johnsonii | 1.14% | ||||||
Delftia spp. | 1.11% | ||||||
Hydrogenophilus spp. | 0.7% | ||||||
Rubrobacter spp. | 0.4% |
For PJI-2, 16S sequencing indicated that the most abundant species present in the infection was P. aeruginosa, with ∼72% RA, followed by Streptococcus agalactiae (25%), S. aureus (1.0%), Propionibacterium at (0.6%), and S. epidermidis (0.3%). As noted in the PJI-2 case history, clinical cultures identified were Strep. agalactiae and S. epidermidis. Therefore, the clinical culture data partially confirm the 16S sequence identification of species. Furthermore, we observed that S. epidermidis had a RA of ∼0.3% of OTUs, a result similar to PJI-1 where S. aureus was identified via direct culture, which had 0.35% RA. These data suggest that a threshold of ≥0.3% RA provided a reasonable agreement with clinical culture.
For PJI-3, S. aureus was the most abundant species comprising ∼79% of the OTUs, followed by P. aeruginosa (20%) and B. fragilis (0.31%).
Negative control DNA extractions, PCR and sequencing
The top five species identified in the negative control were P. tolaasii (37%), Propionibacterium acnes (23%), Variovorax paradoxus (8%), Ralstonia pickettii (7%) and Strep. thermophilus (7%) RA, respectively. The full list of species identified in our negative controls that had a RA of ≥0.3% is shown in Table 2.
PCR and densitometry analysis
The PCR product was analyzed using 1.2% agarose gel. Densitometry analysis using myImage Analysis software showed band detection sensitivity of 100% using the GeneRuler 1 kb DNA ladder. The standard DNA ladder has 12 bands and the PCR products showed bands corresponding with 1.5 bp size (Fig. 3). PCR products contained amplified DNA that was present in orthopedic samples (ranging from 69 to 77 ng) as well as the OR negative control samples (saline and specimen collection vessel; 19–28 ng), but in significantly lower amounts than from the retrieved material. No contaminating DNA was observed in the DNA isolation or PCR reagents or water that was used during the process (Fig. S1, Supporting Information).
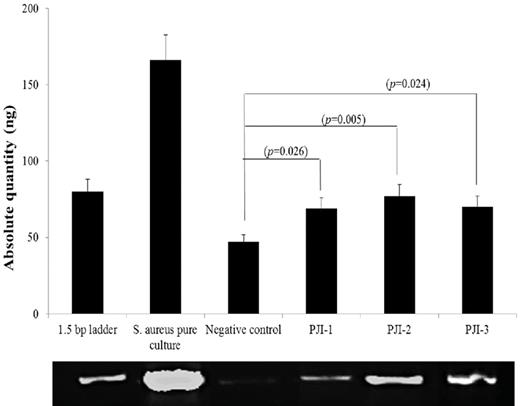
Gel electrophoresis of amplification of PCR products. (i) 1.5 bp ladder, (ii) pure culture of S. aureus, (iii) negative control 1, (iv) suture material 1 (PJI-1), (v) suture material 2 (PJI-2) and (vi) suture material (PJI-3), ng—nanogram.
Analysis of similarity
We conducted a Bray–Curtis non-metric MDS analysis of the rank abundances of species per OM specimen, and performed ANOSIM of the organism profiles with respect to location within the knee, OM type and whether or not suture acts as a source for infection. Material type and suture versus non-suture material factors were not statistically significant (P = 0.76, and 0.769, respectively) (Table 3). In addition, we conducted ANOSIM of the organism species profiles with respect to each case. Upon ANOSIM between cases based on species data, a statistically significant difference (P < 0.001) was observed, indicating that each case was a unique infection (Fig. 4; Table 3). Finally, all PJIs were statistically significantly different from our four negative controls on ANOSIM (P = 0.002) (Fig. 5).
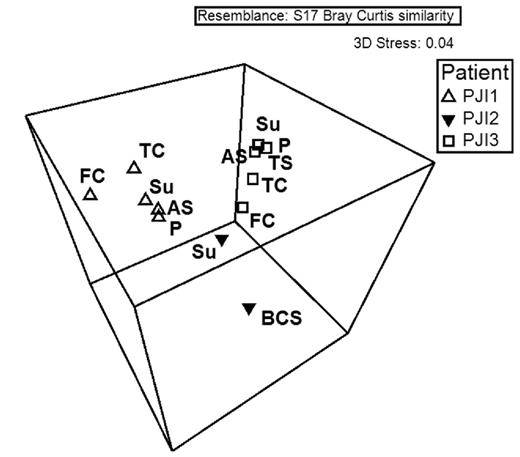
ANOSIM between cases based on species data. Note: on boxes for PJI-3, components P and TS overlap. Su—suture, FC—femoral component, TC—tibial component, P—patellar component, AS—articulating surface, BCS—bone cement spacer, TS—tibial screws.
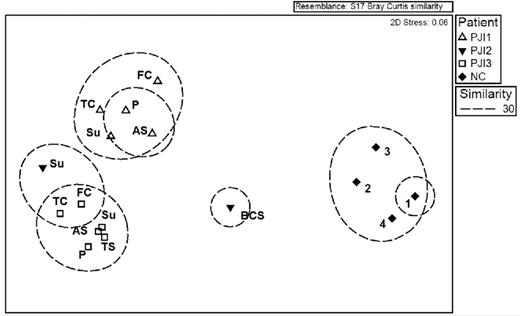
ANOSIM between cases and controls based on species data. Su—suture, FC—femoral component, TC—tibial component, P—patellar component, AS—articulating surface, BCS—bone cement spacer, TS—tibial screws, 1–4—negative control 1–4.
ANOSIM for the rank abundance of species for various factors pertaining to OMs and for individual cases. PJI—periprosthetic joint infection, UHMWPE—ultra-high-molecular-weight polyethylene.
Material type . | P-value . |
---|---|
Metal versus plastic | 0.76 |
Suture versus non-suture | 0.769 |
Case profile | |
PJI-1 versus PJI-2 | 0.048 |
PJI-1 versus PJI-3 | 0.002 |
PJI-2 versus PJI-3 | 0.036 |
All PJIs versus negative control | 0.002 |
Overall | 0.001 |
Material type . | P-value . |
---|---|
Metal versus plastic | 0.76 |
Suture versus non-suture | 0.769 |
Case profile | |
PJI-1 versus PJI-2 | 0.048 |
PJI-1 versus PJI-3 | 0.002 |
PJI-2 versus PJI-3 | 0.036 |
All PJIs versus negative control | 0.002 |
Overall | 0.001 |
ANOSIM for the rank abundance of species for various factors pertaining to OMs and for individual cases. PJI—periprosthetic joint infection, UHMWPE—ultra-high-molecular-weight polyethylene.
Material type . | P-value . |
---|---|
Metal versus plastic | 0.76 |
Suture versus non-suture | 0.769 |
Case profile | |
PJI-1 versus PJI-2 | 0.048 |
PJI-1 versus PJI-3 | 0.002 |
PJI-2 versus PJI-3 | 0.036 |
All PJIs versus negative control | 0.002 |
Overall | 0.001 |
Material type . | P-value . |
---|---|
Metal versus plastic | 0.76 |
Suture versus non-suture | 0.769 |
Case profile | |
PJI-1 versus PJI-2 | 0.048 |
PJI-1 versus PJI-3 | 0.002 |
PJI-2 versus PJI-3 | 0.036 |
All PJIs versus negative control | 0.002 |
Overall | 0.001 |
This analysis was performed using genera data as well and revealed there was no significant difference among material type and suture versus non-suture material factors. However, ANOSIM on the genus data did reveal that organism profiles with respect to each case were statistically significantly different (P < 0.001) and that case profiles were statistically significantly different from the negative controls (P = 0.002).
DISCUSSION
Polymicrobial infections in orthopedics represent a major health and economic burden, and research in this area is needed. Some literature suggest that certain cohorts or ‘pathogroups’ of organisms can exacerbate and/or complicate an infection by tipping the balance between acute and chronic disease (Short, Murdoch and Ryan 2014). Our goal was to determine if tendencies exist concerning organism profiles with respect to the component type or material within the failed reconstructed knee and whether non-absorbable suture might also act as a nidus for infection.
The agar encasement method was effective at visualizing bacterial outgrowth associated with CoCrMo (cobalt chromium molybdenum) and suture materials. We observed bacterial outgrowth occurring in highly localized areas, but did not note any obvious correlation with surface topographical features. There were ∼103 and 104 total CFU per femoral and tibial component, respectively, which was consistent with those reported in other PJI cases and animal models (Stoodley et al.2011; Holinka et al.2012; Niska et al.2012; Portillo et al.2013; Lass et al.2014). The method had limitations for assessing outgrowth from the surface of UHMWPE and titanium components due to limited contrast between potential colonies and the surface color and topography; however, it is possible that optically clear agar might be better suited to the assay. Another limitation with the larger components was that colonies growing in the bulk agar presumably detached during the flooding stage made it difficult to identify outgrowth directly from the surface and indeed might have reattached at different locations.
While agar encasement was useful for promoting biofilm outgrowth and allowing us to localize biofilm on the suture without disrupting the biofilm by the necessity for removal, we performed a 16S rRNA phylogenetic analysis of bacterial DNA extracted directly from explanted hardware to determine the full pathogen profile and similarity between the different components and different patients. We chose to include this identification technique to overcome possible false negatives with routine clinical culturing.
Bacteroides fragilis was the most common OTU recovered in all components for PJI-1, a finding consistent with intraoperative cultures. Bacteroides fragilis is a slow-growing anaerobic gut organism occasionally associated with PJI, especially when there is surgical site drainage (Brook 2008). There was also reasonable consistency between clinical culture, species identification from the agar encasement culturing, confocal microscopy and the 16S rRNA phylogenetic data with respect to Staphylococcus aureus in PJI-1; however, it is interesting to note that S. aureus was not detected by clinical culture at the time of explantation, but rather 2 months later at a follow-up appointment to treat a loculated abscess in the knee. Pseudomonas aeruginosa is a common nosocomial pathogen (Balasubramanian et al.2013) and was also identified in our rRNA analysis for PJI-1, but P. aeruginosa was neither isolated nor identified by culture, particularly since Pseudomonas spp. were identified in the negative control and thus we suspect it could be a contaminant from the laboratory environment. Pseudomonas aeruginosa biofilms are known to produce slow-growing small-colony phenotypes (Kirisits et al.2005) and, more recently, ‘culture-impaired’ mutants (Penterman et al.2014), which could explain inability to culture P. aeruginosa from this chronic PJI case. Taken together, these data reiterate that the time (pre-operation, in-OR, post-op, in follow-up) and method (culture, PCR) of culturing do not accurately reflect the pathogen profile (Huang et al.2012; Jacovides et al.2012; Parvizi, Erkocak and Della Valle 2014; Peel et al.2015). While our global analysis of PJIs indicated a polymicrobial infection involving biofilms on multiple components and different OMs, it appears that neither clinical nor research culture by themselves reflected the true complexity of the infection at the time of explantation. However, although P. aeruginosa was not identified specifically in the species analysis from DNA from our negative controls, we need to be cautious in our interpretation regarding the presence of this species in the clinical samples since Pseudomonas spp. identified at the species level were abundant in the negative controls.
An important limitation related to 16S sequencing for diagnostics is its potential to overrepresent the organisms present in clinical samples due to potential contaminating stable DNA molecules (Bauer et al.2006; Marin et al.2012). For this reason, we included a negative control that included common OR material and sterile water. None of the sterile negative control materials (capped unused marking pen, OR gloves, water, suture and specimen tub) came into patient contact, all material remained unused during the case, and all of the material was collected aseptically. Thus, the negative control sequencing data provide an interesting picture into potentially contaminating DNA with respect to genera and species. The top five species identified in our control were P. tolaasii (37%), Propionibacterium acnes (23%), Variovorax paradoxus (8%), Ralstonia pickettii (7%) and Streptococcus thermophilus (7%). Most of these organisms are non-pathogenic and are environmental organisms or inhabiting normal humans (Kaewkla and Franco 2013; Lynch et al.2014; de Lima Brossi et al.2015; Hou et al.2015; Razaif-Mazinah et al.2016; Rothig et al.2016). While it was reassuring that many of the species identified in our negative control were not pathogens, Prop. acne, a common skin bacteria and an opportunistic pathogen, did represent 23% RA in the sample. Thus, it is not possible to rule out entirely that the 16S species data for this PJI-2 represent, at least in part, material from the OR environment. In addition, Pseudomonas spp. represented major proportion of our negative controls. Although most Pseudomonas species were identified in larger numbers in our negative control, all were non-pathogenic (tolaasii, 37%; putida, 2.72%). Ruling out DNA contamination from the patient, the OR personnel and sterile OR materials is of concern in these types of studies as observed in case of Prop. acnes present in negative controls, our data illustrate issues with contaminating DNA present in sterile collection and washout materials. In the future, cleaning collection material with DNase might reduce the contamination load; however, it must be recognized that the OR environment is not DNA free and contamination will remain an issue.
16S bacterial identification based on the variable region is a prime choice for community analysis; however, the choice of the variable region can affect the taxonomic classification, OTU richness and diversity (Claesson et al.2009). It is common for current studies to rely on smaller regions, specifically V1–V3 or V3–V5, produced on the Illumina MiSeq or other platforms, for reasons of economy; however, full-length 16S rRNA gene (flanking from variable regions V1–V9) is used as gold standard for accurate taxonomic identification (Myer et al.2016). While direct observation of biofilms is recommended as the ‘gold standard’ for the direct detection of biofilms, limited sample and samples which are not readily sub-sectioned (such as OMs) constrain the analyses that may be performed (Hall-Stoodley et al.2012; Hoiby et al.2015).
Molecular diagnostics have played a crucial role in determining the role that complex communities might have in human disease in microbiologically diverse anatomical locations (i.e. the gut, vagina, oral cavity, etc.) (Murray et al.2014). However, molecular diagnostics may also be useful for identifying patterns between the microbial communities and the course of infection in deep tissue surgical site infections. The ANOSIM of the Bray–Curtis MDS did not indicate any single component material as being statistically significantly different from the others in our limited number of PJIs. In addition, a study of 32 patients with clinically infected joint replacement components found that bacterial attachment was unrelated to component type or material type (Gomez et al.2012).
In addition, an abundance of green braided suture was discovered during the removal of the primary implant materials deep within the tissue. It is well documented that permanent sutures and other foreign bodies can serve as a chronic infection nidus (Kathju et al.2009, 2010a,b). Indeed, the suture materials exhibited outgrowth using the AECM, and the presence of biofilm on the sutures was confirmed using confocal microscopy. It is not clear whether the infection spreads from-or-to the sutures and the ANOSIM did not indicate a statistical difference from other components (Fig. 5; Table 3); however, it is possible that the surface area and topography of braided suture can present an ideal surface for colonization along with the orthopedic prosthetic hardware.
The data from our study and those of others based on enhanced (sonication) culture and PCR detection suspect that a greater number of these infections are indeed polymicrobial and may be going unidentified by conventional clinical culture methods. A wealth of infectious diseases among humans may entail polymicrobial etiologies (Kesavalu et al.2007; Dowd et al.2008; Rasouli et al.2012; Evangelopoulos et al.2013; Tetreault et al.2013; Aggarwal et al.2014; Misiakos et al.2014; Payne and Bayatibojakhi 2014; Tang et al.2014; Charles et al.2015; Earl, An and Ryan 2015; Ganesh et al.2015; Wimmer et al.2015; Yousif, Jamal and Raad 2015). One large cohort study identified polymicrobial infections in as many as 46.6% of PJI cases (Wimmer et al.2015). Other groups have also reported incidences of polymicrobial infection in PJI (Rasouli et al.2012; Evangelopoulos et al.2013; Tetreault et al.2013; Aggarwal et al.2014; Wimmer et al.2015).
Currently, a gap in the knowledge exists related to whether there are principal infecting organism(s) driving chronic polymicrobial PJI or if the global polymicrobial community plays a major role. For instance, Wimmer et al. (2015) also reported that polymicrobial infections reduce the PJI cure rate. In the future, a larger cohort of 16S sequence data from PJI cases, including monobacterial PJIs, is needed to truly determine if polymicrobial infection in PJI is indeed a polybacterial disease, in which two or more infecting organisms act symbiotically to exacerbate the infection (Ramsey, Rumbaugh and Whiteley 2011). While, more research is needed to accurately estimate the rate of polymicrobial infections in PJIs, we believe future work will reveal polymicrobial infectious etiology as more widespread in PJIs than currently considered, given the complex nature of biofilm communities coupled with the implanted components' susceptibility to biofilm growth. A better understanding of the biology of polymicrobial infections in PJI may translate to more effective treatment strategies.
Furthermore, timing of explantation and then revision surgery in the infected PJI has been and continues to be debated. There is available clinical evidence to support the idea that two-stage revisions (whereby components are explanted, then new components implanted after an interim period of antibiotic treatment) in TKA can yield infection-free results (upto 97%–100%) in knee PJI cases (Insall, Thompson and Brause 1983; Windsor et al.1990). In addition, infection-free results of only 48%–57% are often obtained in one-stage revisions (where infected materials are removed, the wound is subjected to IAD, and then new components are implanted during one procedure) (Gardner, Gioe and Tatman 2011; Buller et al.2012). Nonetheless, considerable debate on this clinical decision point currently exists (Radoicic et al.2012; Massin et al.2015).
Overall, we were unable to find any correlation between biofilm outgrowth with AECM and material type or surface type in our biofilm mapping distribution attempts. Multispecies bacterial biofilms may colonize all implanted components of PJI cases, including suture material. Thus, no foreign material should be considered free of biofilm infection in PJI cases and should be considered for explantation. In addition, we confirmed that culture can fail to detect the multispecies nature of complex biofilm infections.
This study has several limitations; first, while 16S sequencing of PJI yields robust results, we acknowledge that the use of 16S sequencing is somewhat controversial because there exists a high risk of generating false positive results (Bauer et al.2006; Marin et al.2012). Because DNA is a relatively stable molecule, allowing it to remain sufficiently intact long after the organism has perished (Bauer et al.2006). DNA could remain on surfaces (DNA contamination) of sterilized sampling containers or surgical instruments, thus, contaminating molecular grade reagents (i.e. nuclease free water), or be endemic to the specific laboratories handling the DNA (Salter et al.2014). It has been demonstrated that different DNA extraction kits yield different 16S sequencing results from the same sample, suggesting that the DNA extraction kits themselves have their own nucleic acid profile, or have insufficiencies (i.e. complete bacteria lysis) with regards to purifying the DNA (Kennedy et al.2014). Therefore, we were careful to scrutinize and validate the 16S sequencing results obtained here. To do this, we relied on clinical and research culture data to help confirm the 16S sequencing results, which, for the most part, satisfied our skepticisms.
It is also important to note, however, that the use of direct culture to analyze the organism profile of PJI is also not fully reliable because there exists a high rate of false negatives (failure to culture biofilm bacteria). For instance, for all case organism profiles, 16S sequencing identified Pseudomonas in varying, yet above threshold RAs; however, Pseudomonas was never cultured. It is possible that this genus represents contamination from OR materials and water; however, the negative control indicated primarily the presence of non-pathogenic Pseudomonas species. In addition, the difficulty in clinical culture of bacteria from PJI has been reported throughout the literature, and is likely attributed to the biofilm growth phenotype assumed by the infecting organism(s) (Costerton et al.2011; Corvec et al.2012; McConoughey et al.2014; Dastgheyb et al.2015).
SUPPLEMENTARY DATA
The authors would like to thank Maurice Manring, Ph.D.; Kelly Lindsey, B.S.; Kelly Wrighton, Ph.D. and Joan-Miquel Balada-Llasat, PharmD., Ph.D. D. (ABMM), for their guidance in the preparation of this manuscript.
FUNDING
This work was supported by and partially funded by the (PHPID) program (phpid.osu.edu) to PS and an NIH/NIAID award # , a NRSA training grant administered by the Center for Microbial Interface Biology (CMIB), at The Ohio State University to MS.
Conflict of interest. None declared.
REFERENCES