-
PDF
- Split View
-
Views
-
Cite
Cite
Daria Augustyniak, Monika Piekut, Grażyna Majkowska-Skrobek, Jacek Skała, Bactericidal, opsonophagocytic and anti-adhesive effectiveness of cross-reactive antibodies against Moraxella catarrhalis, Pathogens and Disease, Volume 73, Issue 3, April 2015, ftu026, https://doi.org/10.1093/femspd/ftu026
- Share Icon Share
Moraxella catarrhalis is a human-restricted significant respiratory tract pathogen. The bacteria accounts for 15–20% of cases of otitis media in children and is an important causative agent of infectious exacerbations of chronic obstructive pulmonary disease in adults. The acquisition of new M. catarrhalis strains plays a central role in the pathogenesis of both mentioned disorders. The antibody-dependent immune response to this pathogen is critical for its effective elimination. Thus, the knowledge about the protective threshold of cross-reactive antibodies with defined functionality seems to be important. The complex analysis of broad-spectrum effectiveness of cross-reactive antibodies against M. catarrhalis has never been performed. The goal of the present study was to demonstrate and compare the bactericidal, opsonophagocytic and blocking function of cross-reacting antibodies produced in response to this bacterium or purified outer membrane proteins incorporated in Zwittergent-based micelles. The multivalent immunogens were used in order to better mimic the natural response of the host. The demonstrated broad-spectrum effectiveness of cross-reactive antibodies in pathogen eradication or inhibition strongly indicates that this pool of antibodies by recognition of pivotal shared M. catarrhalis surface epitopes seems to be an essential additional source to control host–microbe interaction.
INTRODUCTION
Moraxella catarrhalis is a human-restricted significant respiratory tract pathogen but also a part of the normal bacterial microflora in the nasopharynx (Verhaegh et al., 2011). Clinical and epidemiological studies have revealed high carriage rates in young children and suggested that it may be associated with infection caused by M. catarrhalis. The carriage rate of M. catarrhalis in healthy children varies between 7 and 36% (Verhaegh et al., 2010) and increases to ∼50% in children suffering from upper respiratory tract infections (Konno et al., 2006). The strong relationship between the frequency of M. catarrhalis nasopharyngeal colonization and otitis media was confirmed in infants (Faden et al., 1997). There is some evidence that widespread use of pneumococcal conjugate vaccines has altered nasopharyngeal colonization patterns and causes an increased prevalence of colonization and infection by M. catarrhalis (Murphy and Parameswaran 2009). In adults M. catarrhalis is responsible for exacerbations of chronic obstructive pulmonary disease (COPD), accounting for approximately 2 to 4 million episodes annually (Murphy et al., 2005).
Nowadays, it is confirmed that the antibody-dependent immune response to this pathogen is critical for its effective elimination. The most immunogenic structures of M. catarrhalis which participate in bacterial virulence and confer protective immunity are outer membrane proteins (OMPs) and lipooligosaccharide (LOS), (Hu et al., 2000; De Vries et al., 2009). Some of the OMPs, designated as UspA1, UspA2, OmpCD, CopB, TBP as well as LOS, have been found to be targets for bactericidal antibodies (McMichael et al., 1998; Myers et al., 1998; Chen et al., 1999; Ren et al., 2011a) and are classified as important factors for complement evasion (Norsdström et al., 2005; Attia et al., 2006; Hallström et al., 2011). Some of these proteins including OMPs such as UspA1, UspA2, MID/Hag, OmpCD and McaP contribute to M. catarrhalis adhesion and several of them such as UspA1, UspA2H and Hag also to biofilm formation (LaFontaine et al., 2000; Timpe et al., 2003; Holm et al., 2004; Pearson et al., 2006). OMPs and LOS are also believed to be key players in the process connected with invasion of the host epithelium of the respiratory tract, which tentatively is a reservoir for this bacterium (Heiniger et al., 2007; Slevogt et al., 2007). Because the pathogenesis of infection caused by M. catarrhalis is connected mainly with (1) evasion of the complement cascade, (2) adherence and biofilm formation, and (3) invasion of respiratory epithelial cells accompanied by induction of an inflammatory response (Perez-Vidakovics and Riesbeck 2009); therefore, the antibody-mediated bactericidal activity of complement, antibody-mediated blocking of bacterial adherence as well as effective opsonophagocytosis should represent one of the crucial events triggered during host defense. Although promising vaccine candidates against M. catarrhalis have been shown to induce protective immunity in pulmonary clearance animal models, there is currently no licensed vaccine against M. catarrhalis (Helminen et al., 1993; Liu, McMichael and Baker 2007; Su, Singh and Riesbeck 2012; Smidt et al., 2013).
Intraspecies and interspecies cross-reacting antibodies are produced in high frequency against microbial antigens sharing immune-relevant epitopes (Müller-Loennies et al., 2007; Arenas et al., 2008; Keasey et al., 2008). However, the effectiveness of the antibody-mediated antimicrobial immune response strictly depends on whether it is sufficient for protection. The presence of cross-reactive antibodies specific against heterologous M. catarrhalis immunogens as well as other bacteria such as Haemophilus influenzae, Neisseria meningitidis and N. lactamica has been reported (Tronsoco et al., 2004; Gergova et al., 2007; Augustyniak, Mleczko and Gutowicz 2010). The induction of bactericidal cross-reactive antibodies recognizing individual M. catarrhalis OMP has been demonstrated, with the use of both polyclonal and monoclonal antibodies (Yang-Ping et al., 1997; McMichael et al., 1998; Chen et al., 1999). Only limited studies have been performed with a Moraxella multivalent antigenic formulation involving combined LOS conjugates, modified LOS or null LOS mutant (Peng et al., 2005; Ren et al., 2011a,b). In the aforementioned studies, no direct comparison between various multivalent immunogens and multifunctional activity of cross-reacting antibodies was carried out. Therefore, the goal of the present study was to demonstrate and compare the broad-spectrum functionality, namely bactericidal, opsonophagocytic and blocking of cross-reacting antibodies produced in response to whole-cell antigens or purified OMP preparations. The potential involvement of this pool of antibodies seems to be an additional source of defense in the host response against M. catarrhalis where only the interplay of various antibody-mediated mechanisms can overcome the combinatorial activities of virulence factors of this host-adapted bacterium.
MATERIALS AND METHODS
Reagents
Antibodies: affinity pure goat anti-mouse IgG Fcγ subclass 2a- or 2b-specific, horseradish peroxidase (HRP)-conjugated affinity pure goat anti-mouse IgG Fcγ-specific, HRP-conjugated affinity pure rabbit anti-goat IgG (H + L) were from Jackson ImmunoResearch (Baltimore, USA); Avidin-HRP (DAKO, Denmark); bovine serum albumin (BSA) (SERVA, Heidelberg, Germany); 4-chloro-1-naphtol (Sigma, Germany), complement sera from guinea pig (Sigma, USA); EZ-link sulfo-NHS-biotin (Pierce, Rockford, USA); Hank's BSS with Ca++ Mg++ (PAA, Pasching, Austria); antibiotic-antimycotic solution (100×) containing 10 000 units ml−1 of penicillin, 10 000 μg ml−1 of streptomycin and 25 μg ml−1 of Fungizone; glutamax (100×), heat-inactivated fetal bovine serum (FBS), and sodium pyruvate (100×) were from GIBCO, Life Technologies; DMEM, RPMI-1640 media and trypsin-EDTA solution were from Lonza (Verviers, Belgium); o-phenylenediamine dihydrochloride tablets, OPD (Calbiochem, Darmstadt, Germany); sodium thiocyanate NaSCN (Sigma, Germany); TMB substrate reagent (R&D, Minneapolis, USA); trypan blue (Sigma, Steinheim, Germany); Zwittergent 3–14 detergent (Calbiochem, Germany).
Bacterial strain characteristics and growth conditions
The clinical isolates of M. catarrhalis designated as Mc1, Mc5, Mc6, Mc8 were taken from our collection. Previously, the strains were isolated from upper respiratory tract and were obtained from Laboratory of Bacteriology of the Silesian Center of Pediatrics in Wroclaw, Poland. The final identification was made by use of a standardized commercial identification system API-NH (bioMérieux, France). Bacteria were cultured on blood agar (OXOID) containing 5% sterile sheep blood or grown in brain heart infusion (BHI) broth at 37°C with 5% CO2. The strains were stored in BHI containing 16% glycerol at −70°C. The phenotypic characteristics of strains presented in Table 1 involved determination of (i) complement sensitivity with normal human serum (Verduin et al., 1995), (ii) autoagglutination ability with modification (Verhaegh et al., 2008), (iii) adherence potency to A549 cell line (see adhesion section) using biotinylated bacteria (Brouillette et al., 2003) and (iv) biofilm formation (Stepanović et al., 2000). Additionally, the presence of genes such as uspA1, hag/mid and ompCD encoding OMPs or LOS type was confirmed by PCR (Edwards et al., 2005; Verhaegh et al., 2008).
Strains . | Source of origin . | Complement resistance1 . | LOS type2 . | Autoagglutination ability3 . | Adherence potency4 . | Biofilm formation5 . |
---|---|---|---|---|---|---|
Mc1 | Throat | Sensitive | A | 0.38 ± 0.04 | 1.64 ± 0.34 | 0.47 ± 0.12 |
Mc5 | Throat | Sensitive | A | 0.38 ± 0.02 | 1.06 ± 0.24 | 0.32 ± 0.06 |
Mc6 | Oropharynx | Sensitive | A | 0.38 ± 0.07 | 1.0 ± 0.22 | 0.72 ± 0.18 |
Mc8 | Throat | Sensitive | B | 0.87 ± 0.01 | 0.73 ± 0.14 | 0.17 ± 0.04 |
Strains . | Source of origin . | Complement resistance1 . | LOS type2 . | Autoagglutination ability3 . | Adherence potency4 . | Biofilm formation5 . |
---|---|---|---|---|---|---|
Mc1 | Throat | Sensitive | A | 0.38 ± 0.04 | 1.64 ± 0.34 | 0.47 ± 0.12 |
Mc5 | Throat | Sensitive | A | 0.38 ± 0.02 | 1.06 ± 0.24 | 0.32 ± 0.06 |
Mc6 | Oropharynx | Sensitive | A | 0.38 ± 0.07 | 1.0 ± 0.22 | 0.72 ± 0.18 |
Mc8 | Throat | Sensitive | B | 0.87 ± 0.01 | 0.73 ± 0.14 | 0.17 ± 0.04 |
1Determined following incubation with normal human serum.
2Determined by PCR.
3Absorbance A600 measured following 60 min sedimentation of bacterial suspension at initial OD = 1. Sedimentation rate for Mc8 was significantly slower (P < 0.05) in comparison for other strains as determined by one-way ANOVA.
4Adherence of biotinylated bacteria to A549 cell line detected by NHS-biotin–avidin ELISA at A490. The data are means ± SEM of the results from at least three independent experiments performed in five replicates. Statistically significant differences between strains for P < 0.005 were found (Kruskal–Wallis ANOVA) except Mc5 and Mc6 comparison.
548 h biofilm determined by colorimetric method with 0.1% crystal violet at A595. The strains differ in biofilm formation (P < 0.00001) as determined by Kruskal–Wallis ANOVA.
Strains . | Source of origin . | Complement resistance1 . | LOS type2 . | Autoagglutination ability3 . | Adherence potency4 . | Biofilm formation5 . |
---|---|---|---|---|---|---|
Mc1 | Throat | Sensitive | A | 0.38 ± 0.04 | 1.64 ± 0.34 | 0.47 ± 0.12 |
Mc5 | Throat | Sensitive | A | 0.38 ± 0.02 | 1.06 ± 0.24 | 0.32 ± 0.06 |
Mc6 | Oropharynx | Sensitive | A | 0.38 ± 0.07 | 1.0 ± 0.22 | 0.72 ± 0.18 |
Mc8 | Throat | Sensitive | B | 0.87 ± 0.01 | 0.73 ± 0.14 | 0.17 ± 0.04 |
Strains . | Source of origin . | Complement resistance1 . | LOS type2 . | Autoagglutination ability3 . | Adherence potency4 . | Biofilm formation5 . |
---|---|---|---|---|---|---|
Mc1 | Throat | Sensitive | A | 0.38 ± 0.04 | 1.64 ± 0.34 | 0.47 ± 0.12 |
Mc5 | Throat | Sensitive | A | 0.38 ± 0.02 | 1.06 ± 0.24 | 0.32 ± 0.06 |
Mc6 | Oropharynx | Sensitive | A | 0.38 ± 0.07 | 1.0 ± 0.22 | 0.72 ± 0.18 |
Mc8 | Throat | Sensitive | B | 0.87 ± 0.01 | 0.73 ± 0.14 | 0.17 ± 0.04 |
1Determined following incubation with normal human serum.
2Determined by PCR.
3Absorbance A600 measured following 60 min sedimentation of bacterial suspension at initial OD = 1. Sedimentation rate for Mc8 was significantly slower (P < 0.05) in comparison for other strains as determined by one-way ANOVA.
4Adherence of biotinylated bacteria to A549 cell line detected by NHS-biotin–avidin ELISA at A490. The data are means ± SEM of the results from at least three independent experiments performed in five replicates. Statistically significant differences between strains for P < 0.005 were found (Kruskal–Wallis ANOVA) except Mc5 and Mc6 comparison.
548 h biofilm determined by colorimetric method with 0.1% crystal violet at A595. The strains differ in biofilm formation (P < 0.00001) as determined by Kruskal–Wallis ANOVA.
Cell line culture conditions
A549 human epithelial cell line (type II alveolar cell carcinoma epithelium) was cultured in DMEM medium supplemented with 10% FBS, 1× glutamax, 1× sodium pyruvate and 1× antibiotic-antimycotic solution at 37°C in the presence of 5% CO2. To obtain a fully confluent monolayer, cells were grown for 2–3 days. Before a new passage, cells were trypsinized with trypsin-EDTA solution (Lonza, Belgium) and washed. A549 was used in adhesion experiments. THP-1 human monocyte/macrophages cell line was maintained in RPMI-1640 supplemented with 10% FBS, 1× glutamax and 1× antibiotic-antimycotic solution. To obtain macrophage phenotype, cells seeded in density 105 per well at final volume 200 μl were activated/stimulated by PMA at final concentration 20 ng ml−1 for 48 h with media refreshment after 24 h. The differentiated macrophages were used for opsonophagocytosis experiments. Both lines were propagated in flasks or microplates from NUNC (ThermoScientific, Denmark).
Isolation of M. catarrhalis OMPs
The OMPs of Mc1, Mc5, Mc6 and Mc8 were obtained using zwitterionic detergent Zwittergent Z 3–14 according to previously described method (Augustyniak, Mleczko and Gutowicz 2010). Briefly, the harvested bacteria from 200 ml of culture were suspended in 5 ml of buffer (1 M CH3COOH, 1 mM β-mercaptoethanol; pH 4.0). To this suspension, a 45 ml volume of a solution of 0.5 M CaCl2 containing 5% Zwittergent was added and stirred for 1 h at room temperature. Nucleic acids were precipitated by the addition of 12.5 ml cold absolute ethanol and subsequent centrifugation (17 000 × g, 10 min, 4°C). The pellet was discarded and the remaining in supernatant proteins were precipitated by the addition of 187 ml of cold ethanol and collected by centrifugation (17 000 × g, 20 min, 4°C). The pellet was air-dried and then resuspended in 10 ml of Z buffer (0.05% Zwittergent, 50 mM Tris, 10 mM EDTA; pH 8.0). Such mixture was stirred for 1 h at room temperature, centrifuged at 12 000 × g for 10 min at 4°C and the soluble fraction containing OMPs retained. OMPs were divided into aliquots and stored at −70°C. The OMPs concentration was determined by using BCA protein assay kit (Pierce). The appropriate protein profile was confirmed after SDS-PAGE and gel staining with Coomassie (GelCode blue stain reagent; Pierce). SDS-PAGE profiles of obtained proteins were presented previously (Augustyniak, Mleczko and Gutowicz 2010).
Immunization and preparation of mouse antisera
BALB/c mice, at 8–10 weeks of age, were used for immunization under a protocol approved by the Institutional Animal Care and Use Committee (Wroclaw, no 58/04). Groups of 5–7 mice of approximately equal weight were immunized intraperitoneally with relevant antigen in a 0.1 ml doses per animal, three times, in 2-week intervals (on days 0,14 and 28). The immunizing antigens were OMPs (10 μg dose−1) or heat-inactivated bacteria in saline (5 × 107 cfu dose−1) of strains: Mc1, Mc5, Mc6 and Mc8. In 10 days, after the last immunization mice were terminally bled through the retro-orbital sinus. Sera for each group of mice were pooled, divided and stored in aliquots at −70°C until use. This study complied with the animal experimentation guidelines of the author's institution.
Preparation of bacteria for whole-cell ELISA
16 h BHI cultures of M. catarrhalis were centrifuged (8000 × g, 15 min), washed twice with saline and suspended in saline to OD550 = 1. The bacterial suspensions were supplemented with 20% glycerol, mixed, divided and stored in 2 ml aliquots at −20°C until use.
Whole-cell ELISA
The bacterial suspension (OD550 = 1), washed and diluted 1:5 in carbonate–bicarbonate buffer (100 μM) was coated onto flat-bottom 96-well microtiter plates MaxiSorp (NUNC) by incubation at room temperature for 18 h. Wells were blocked with 300 μl of 1% BSA in PBS (pH 7.4) for 1 h at 37°C and then incubated for 2 h at 37°C with 100 μl per well of 2-fold serial dilutions of the mouse antisera (anti-Mc and anti-OMPMc) at TPBS-BSA buffer (PBS with 0.05% Tween 20 supplemented with 1% BSA). After four washes with 400 μl of TPBS, IgG-specific mouse antibodies were detected by using HRP-conjugated Fcγ-specific goat antibodies diluted 1:10 000. At that step, the plate was incubated for 2 h at 37°C. After four-time washing, 100 μl of substrate solution containing 5 mg OPD and 20 μl H2O2 in 10 ml citric acid buffer (pH 4.9) was added to each well and the plate was incubated for 20 min at room temperature in the dark. The enzymatic reaction was stopped with 50 μl 1M H2SO4. Optical density was read at 490 nm at Dynatech MR 5000 reader. The specific mouse subclass IgG2a and IgG2b in anti-Mc and anti-OMPMc sera were detected by 2 h incubation with subclass-specific goat antibodies diluted 1: 10 000 (2 h at 37°C/100 μl per well) followed by analogous incubation with HRP-conjugated affinity pure rabbit anti-goat IgG antibodies diluted 1:40 000. Enzymatic reaction was developed with 100 μl per well of TBM substrate for 10 min and stopped with 50 μl 1M H2SO4. The plate was read at 450 nm. The antibody endpoint titers were defined as the reciprocal value of the antiserum dilution giving an absorbance extrapolated from a linear portion of the serum titration curve of control mouse serum enlarged by 2 standard deviation (SD). Results are presented as geometric mean titers.
Avidity ELISA
To assess the relative avidity index (AI) of antibodies to surface antigens, ELISA method described by Ostberg, Russell and Murphy (2009) with minor modifications was performed. Sodium thiocyanate (NaSCN) was used to dissociate low-avidity antigen-antibody binding. In preliminary study, various concentrations of NaSCN (ranging from 0 to 3M) were tested to determine the optimal assay conditions for measuring AI. Briefly, after coating the plate with bacterial suspension (see the section ‘whole-cell ELISA’), blocking with 1% BSA and 2 h incubation at 37°C with a series of 2-fold serially diluted mice antisera to be tested, the wells were washed with TPBS-BSA. The serum dilutions were chosen from the linear portion of the serum titration curve. After washing, half of the wells on each plate were incubated with 100 μl of 1.5 M NaSCN, whereas the corresponding wells on the other half of the plate were incubated with the same volume of PBS (as a control) for 15 min at room temperature. After four-time washing, antibodies were detected with anti-mouse IgG conjugate diluted 1:10 000 and OPD as a substrate. Results are expressed as an AI which refers to the percentages of antibodies that remained bound to the antigen after treatment with NaSCN, and was calculated as follows: AI = (OD490 with NaSCN)/(OD490 without NaSCN) × 100. To calculate the total AI, multiple data points obtained from dilutions of the test sera that yielded optical density (OD) values in the linear portion of the curve were averaged.
Western blotting
In order to detect cross-reactive murine antibodies, nitrocellulose strips with bounded OMPs, which were previously separated by standard 12% SDS-PAGE electrophoresis, were blocked overnight in PBS (pH 7.4) containing 1% BSA. The strips were then incubated with 4 ml of various murine anti-OMPs sera diluted 1:1 000 with TPBS-BSA, for 1 h at 37°C and for 0.5 h at room temperature with gentle mixing. The strips were rinsed four times in TPBS and incubated again at 37°C with HRP-conjugated affinity pure goat anti-mouse IgG Fcγ antibodies diluted 1:4000 as indicated above. After four final washes in TPBS, the strips were immersed in freshly prepared substrate solution (20 mg 4-chloro-1-naphtol, 4 ml methanol, 16 ml PBS, 20 μl 30% H2O2) for 30 min with gentle mixing in the dark. The blots were rinsed twice with TPBS.
Antibody-mediated bactericidal activity of complement
The bactericidal capacity of the antibodies present in serum was assessed as previously described (Rodriguez et al., 2002; Becker et al., 2007) with own modification. In brief, bacteria were grown in BHI broth at 37°C with shaking until the OD550 was 0.2–0.25. Then, the culture was diluted 1: 4000 in sterile Hank's BSS with Ca++ Mg++ containing 0.1% BSA. As a source of bactericidal antibodies, the heat-inactivated (by incubation at 56°C for 30 min) pooled mouse antisera diluted 3, 10, 30, 100, 300, 1000 and 3000 × were used. As an exogenous source of complement 10% (v/v), a guinea pig complement serum was used. The effective guinea pig complement fixation by murine antibodies was confirmed (Dangl et al., 1988). The bactericidal assay was performed on 96-well, round-bottom microplate (NUNC) by mixing 20 μl of bacteria in HBSS-BSA with 20 μl of heat-inactivated, serially diluted test mouse antiserum and incubation at 37°C for 30 min with gentle rotation (80 rpm) to obtain opsonization of bacteria. The complement serum was then added to final concentration of 10%, and the mixture in final volume of 50 μl was next incubated for additional 30 min at 37°C. At the end of the test, the 10 μl of assay mixture from each well was quickly spotted in triplicates on BHI agar plate. All plates were next incubated for 24–36 h at 35°C with 5% CO2 and resulting colonies were counted. The bactericidal titer of mouse antiserum was defined as the reciprocal of the highest dilution of antiserum at which 50% killing of the target strain was observed, and is the mean determination of bactericidal assays carried out in triplicate. The interpolated titer value refers to the reciprocal antiserum dilution at the point where the antibody curve intersects the 50% cut-off line. To avoid the possible presence of false results, the following appropriate controls were included: (i) bacteria with HBSS alone as a control of bacteria viability, (ii) bacteria with antiserum without complement serum as a control of internal bactericidal activity of antiserum, (iii) bacteria with complement in the absence of antiserum as a control of the complement potency (less than 10% of bacteria were killed in its presence).
Biotinylation of bacteria
Biotinylated bacteria were prepared as described previously (Brouillette et al., 2003) with modifications. Briefly, 16 h BHI culture of M. catarrhalis (5 ml) was centrifuged, washed twice with carbonate–bicarbonate buffer (pH 8.0) and adjusted to ∼109 CFU ml−1 (OD550 = 1) with cold carbonate–bicarbonate buffer. The bacterial suspension (2 ml) centrifuged and the resulted pellet was suspended in 200 μl of the same buffer and 2 μl of EZ-linked sulfo-NHS-biotin (10 mg ml−1) was added. For biotinylation, the bacteria were incubated for 30 min at room temperature with gentle horizontal mixing (190 rpm). The biotinylated bacteria were washed three times, with 2 ml of carbonate–bicarbonate buffer, then adjusted to OD550 = 1 in DMEM, 2-fold serially diluted (from 1:4 to 1: 128) and used in adhesion assay. The biotinylation did not cause the loss of viability as determined by standard colony count method.
Bacterial adhesion and adhesion blocking assay
The NHS-biotin–avidin ELISA was performed for detection of bacterial adhesion (Ofek et al., 1986) with own modifications. For the adhesion experiment, A549 cells were seeded at an initial density of 2 × 104 cells per well at a final volume of 100 μl in 96-well flat-bottom microplates (NUNC) and incubated under 5% CO2 at 37°C for 24 h (∼90–95% confluence). The cells were washed drop by drop with warm PBS, pH 7.4 to rinse out antibiotics and the plate was blocked with 200 μl of 2% BSA in PBS for 45 min. After blocking, the 2-fold serially diluted (range from 1:4 to 1:128) of biotinylated bacteria in 100 μl of DMEM without antibiotics were added to each well in and the plate was incubated under 5% CO2 at 37°C for 1.5 h. The wells without bacteria and non-biotinylated bacteria were used as controls. The differences in adhesion properties between M. catarrhalis strains diluted 1:8 were calculated based on the curve linearity.
In adhesion blocking experiments, heat-inactivated (56°C for 30 min) post-immune murine sera diluted for test 1:50 and 1:100 (final dilution) were incubated for 30 min at 37°C with 100 μl of homologous or heterologous bacteria diluted 1:4 (30 min at 37°C) and subsequently added to the cell monolayer. To exclude non-specific blocking of bacteria adherence by tested sera, simultaneously control experiments with non-specific murine, bovine and human sera were included. After 1.5 h, adherence microplate was gentle washed three times with PBS-1% BSA (pH 8.0) to remove non-adherent bacteria and 100 μl of 1:2000 avidin-HRP in PBS-1% BSA was applicated for 1 h to detect adherent bacteria. After final gentle three washes, 100 μl of OPD in citrate buffer (pH 4.9) was added. The reaction was stopped after 20 min with 50 μl of 1M H2SO4 and absorbance (λ = 490) was measured on DYNATECH 5000 reader. Results of relative adhesion are expressed as the percentage of M. catarrhalis adhesion in the presence of antisera with respect to 100% adhesion of bacteria in the control wells without antisera. The following controls were included: (i) A549 incubated without bacteria but with avidin-HRP as a control of avidin–cell line interaction, (ii) A549 incubated with non-biotinylated bacteria followed by avidin-HRP as a control of internal biotin-dependent reactivity. During validation of the method, to assess a detection threshold for the biotin–avidin adhesion assay, dose-dependent curves representing adherent bacteria for various infective concentrations of M. catarrhalis were performed.
Opsonophagocytic assay
THP1 differentiation: THP-1 cell line was differentiated into macrophages in 96-well-flat-bottom microplates (NUNC) in the presence of 20 ng ml−1 of PMA for 24 h in total volume of 200 μl of complete growth medium at starting density 5 × 105 cells ml−1 (105 cells per well). Cells were cultured in RPMI-1640 medium supplemented with 1× glutamax, 1× antibiotic-antimycotic solution and 10% heat-inactivated FBS at 37°C in a 5% CO2 atmosphere.
Opsonization: freshly recultivated bacteria at density of 0.3 (OD600 = 0.3) were harvested, washed with PBS and resuspended into Hank's BSS Ca++ Mg++ / PBS buffer (1:1) in order to obtain ∼2 × 104 cells ml−1. 60 μl of bacterial suspension was mixed with 30 μl of serially diluted, heat-inactivated, murine antisera and incubated at 37°C for 30 min with agitation on rotary shaker (250 rpm). Next 60 μl of active guinea pig complement diluted in Hank's BSS Ca++Mg++/PBS buffer was added (final concentration 1.5%, v/v).
Bacterial uptake and killing performed according to Winter and Barenkamp (2003) with own modifications: THP-1 monolayer of macrophages in each well was washed twice with Hank's BSS Ca++Mg++/PBS buffer and 50 μl of opsonized bacteria was added to each well in duplicates following by incubation at 37°C in a 5% CO2 for 2 h. After opsonophagocytosis, non-adherent bacteria were removed by five-time washing with 200 μl of PBS each time. Cells were lysed with 200 μl of 0.05% trypsin/0.08% saponin solution (v/v) for 10 min with shaking. The lysates were then transferred to 1.5 ml Eppendorf tubes and vortexed vigorously for 30 s. Aliquots were serially diluted and 10 μl of each dilution was plated on BHI plates in triplicates. All assays were performed at three independent experiments. The antibody-mediated phagocytic killing was determined for each dilution of antiserum and was defined as the reciprocal of the highest dilution of antiserum at which ≥ 50% killing of the target strain was observed.
Statistical analysis
Normality and homogeneity of variance assumptions were checked using Shapiro–Wilk's and Levene's test, respectively. Differences between antibody avidities in anti-OMPMc sera within homologous or heterologous strains in comparison to relevant anti-Mc sera were analyzed using Student's t test for unpaired samples. For multiple comparisons of phenotypic characteristics, the non-parametric Kruskal–Wallis analyses of variance (ANOVA) was used. For multiple comparisons of adhesion blocking experiments in the presence of cross-reactive antisera, data were analyzed using one-factor ANOVA and were followed by post-hoc Tukey's test. For these comparisons, the data were normalized to a control bacterial adhesion (without antisera) of 100%.
Spearman's rank coefficient was calculated to assess the correlation between complement-mediated bactericidal activity of antisera and quantity of IgG subclasses responsible for complement activation. A P-value < 0.05 was used to assess the significance of all statistical analyses. All the analyses were performed with STATISTICA 9 software.
RESULTS
Phenotypic characterization of M. catarrhalis isolates
The four M. catarrhalis nasopharynx isolates Mc1, Mc5, Mc6 and Mc8 were from our collection. The strain characteristics are presented in Table 1. They were all complement sensitive and differ in LOS type, autoagglutination ability, cell line adherence potency and biofilm formation.
Magnitude and cross-reactivity of IgG antibody response
In order to elucidate the magnitude of immune responses, BALB/c mice were immunized intraperitoneally either with heat-inactivated bacteria or purified total OMPs from four clinical M. catarrhalis isolates. To determine the extent to which post-immune sera containing antibodies cross-reacted with surface-exposed epitopes, whole-cell ELISA was performed. We found that there were high titers of cross-reactive systemic IgG antibodies both in anti-Mc and anti-OMPMc sera (Table 2). As expected, the response was much more pronounced when purified OMPs were used as immunogens. Analysis of antibody binding between homo- versus heterologous isolates for particular anti-Mc sera demonstrated that IgG yielded a lower level of binding to the heterologous isolates, giving mean titers up to 7-fold lower (median: 2.51) compared to homologous ones. In contrast, the degree of IgG binding to homo- and heterologous isolates in anti-OMPMc sera was very similar and usually did not reach a 2-fold (median: 1.28) decrease in reactivity of the latter. In general, the titers of IgG from anti-OMPMc sera were higher than that from anti-Mc sera, giving in homologous systems a 2-fold to nearly 7-fold (median: 4.36) titer increase. In the case of heterologous isolate binding, this increase in reactivity between anti-OMPMc and anti-Mc sera was from 3- to 17-fold (median: 7.69). These results indicate that IgG antibodies produced exclusively to OMPs of M. catarrhalis more efficiently recognize both homologous and cross-reactive surface-exposed epitopes in multiple Moraxella strains in whole-cell ELISA. We conclude, therefore, that cross-reacting antibodies to OMPs and to a lesser degree to whole cells of random strains of M. catarrhalis have a potential to be protective.
Comparison of ELISA reactivity of anti-Mc and anti-OMPMc IgG antibody response to homologous and heterologous M. catarrhalis isolates.
Serum* . | Reciprocal IgG ELISA titers to isolates . | |||
---|---|---|---|---|
. | Mc1 . | Mc5 . | Mc6 . | Mc8 . |
Anti-Mc1 | 8406 | 9423 | 7796 | 6820 |
Anti-Mc5 | 7466 | 22 641 | 8342 | 3434 |
Anti-Mc6 | 7325 | 25 461 | 28 819 | 9033 |
Anti-Mc8 | 7975 | 8286 | 4184 | 18 636 |
Anti-OMPMc1 | 56 588 | 35 711 | 22 125 | 52 714 |
Anti-OMPMc5 | 43 399 | 54 389 | 31 589 | 30 451 |
Anti-OMPMc6 | 112 068 | 155 913 | 118 385 | 69 067 |
Anti-OMPMc8 | 65 289 | 101 380 | 72 529 | 85 778 |
Control serum | <100 | <100 | <100 | <100 |
Serum* . | Reciprocal IgG ELISA titers to isolates . | |||
---|---|---|---|---|
. | Mc1 . | Mc5 . | Mc6 . | Mc8 . |
Anti-Mc1 | 8406 | 9423 | 7796 | 6820 |
Anti-Mc5 | 7466 | 22 641 | 8342 | 3434 |
Anti-Mc6 | 7325 | 25 461 | 28 819 | 9033 |
Anti-Mc8 | 7975 | 8286 | 4184 | 18 636 |
Anti-OMPMc1 | 56 588 | 35 711 | 22 125 | 52 714 |
Anti-OMPMc5 | 43 399 | 54 389 | 31 589 | 30 451 |
Anti-OMPMc6 | 112 068 | 155 913 | 118 385 | 69 067 |
Anti-OMPMc8 | 65 289 | 101 380 | 72 529 | 85 778 |
Control serum | <100 | <100 | <100 | <100 |
*Groups of 5–7 BALB/c mice were immunized intraperitoneally three times with 0.1 ml dose, each containing the whole killed bacteria at 5 × 108 cfu ml−1 (anti-Mc sera) or OMPs at 100 μg ml−1 (anti-OMPMc sera). The end point IgG titer determined for pooled control and post-immunized serum by whole-cell ELISA was defined as the reciprocal value of the serum dilution giving an absorbance of control non-immunized mouse serum enlarged by 2 SD. The data are presented as the geometric mean titers from at least two independent experiments. Bold numbers indicate homologous systems.
Comparison of ELISA reactivity of anti-Mc and anti-OMPMc IgG antibody response to homologous and heterologous M. catarrhalis isolates.
Serum* . | Reciprocal IgG ELISA titers to isolates . | |||
---|---|---|---|---|
. | Mc1 . | Mc5 . | Mc6 . | Mc8 . |
Anti-Mc1 | 8406 | 9423 | 7796 | 6820 |
Anti-Mc5 | 7466 | 22 641 | 8342 | 3434 |
Anti-Mc6 | 7325 | 25 461 | 28 819 | 9033 |
Anti-Mc8 | 7975 | 8286 | 4184 | 18 636 |
Anti-OMPMc1 | 56 588 | 35 711 | 22 125 | 52 714 |
Anti-OMPMc5 | 43 399 | 54 389 | 31 589 | 30 451 |
Anti-OMPMc6 | 112 068 | 155 913 | 118 385 | 69 067 |
Anti-OMPMc8 | 65 289 | 101 380 | 72 529 | 85 778 |
Control serum | <100 | <100 | <100 | <100 |
Serum* . | Reciprocal IgG ELISA titers to isolates . | |||
---|---|---|---|---|
. | Mc1 . | Mc5 . | Mc6 . | Mc8 . |
Anti-Mc1 | 8406 | 9423 | 7796 | 6820 |
Anti-Mc5 | 7466 | 22 641 | 8342 | 3434 |
Anti-Mc6 | 7325 | 25 461 | 28 819 | 9033 |
Anti-Mc8 | 7975 | 8286 | 4184 | 18 636 |
Anti-OMPMc1 | 56 588 | 35 711 | 22 125 | 52 714 |
Anti-OMPMc5 | 43 399 | 54 389 | 31 589 | 30 451 |
Anti-OMPMc6 | 112 068 | 155 913 | 118 385 | 69 067 |
Anti-OMPMc8 | 65 289 | 101 380 | 72 529 | 85 778 |
Control serum | <100 | <100 | <100 | <100 |
*Groups of 5–7 BALB/c mice were immunized intraperitoneally three times with 0.1 ml dose, each containing the whole killed bacteria at 5 × 108 cfu ml−1 (anti-Mc sera) or OMPs at 100 μg ml−1 (anti-OMPMc sera). The end point IgG titer determined for pooled control and post-immunized serum by whole-cell ELISA was defined as the reciprocal value of the serum dilution giving an absorbance of control non-immunized mouse serum enlarged by 2 SD. The data are presented as the geometric mean titers from at least two independent experiments. Bold numbers indicate homologous systems.
The quality of murine IgG antibody response against OMPs was assessed next using the Western blotting method. When OMPs from each Moraxella strain were probed with a panel of pooled cross-reactive sera, a high level of qualitatively different bands was detected. The more frequent shared cross-reactive OMPs are shown in Fig. 1.
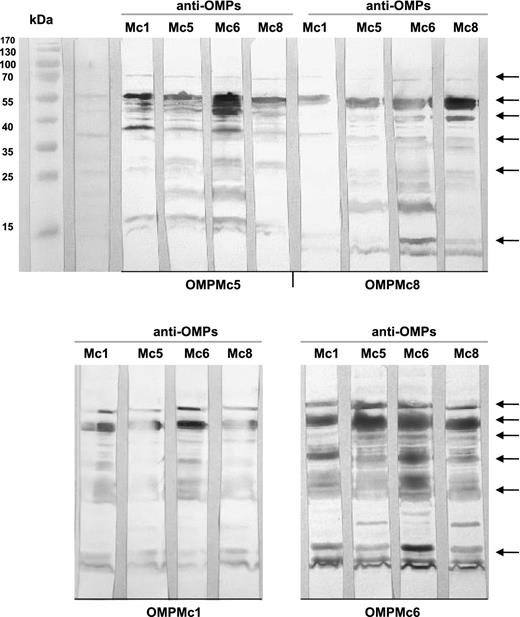
Antigenic cross-reactivity of murine antisera against four strains of M. catarrhalis. OMPs after separation in SDS-PAGE and blotting onto immobilon P membrane reacted with various anti-OMPs sera diluted 1: 1000. The more frequent shared cross-reactive OMPs are indicated by arrows.
Avidity of anti-Mc and anti-OMPMc cross-reactive antibodies
In order to further evaluate the differences in antibody cross-reactivity in reference to both formulations of used immunogens, relative binding avidity studies in the presence of sodium thiocyanate were performed. Using whole-cell ELISA for homologous strains, the AIs of anti-OMPMc sera were significantly higher than those of anti-Mc sera (Fig. 2a). The differences were even more pronounced when cross-reacting antibodies were analyzed, yielding a 17% to nearly a 30% increase in avidity of anti-OMPMc sera as compared with anti-Mc sera (Fig. 2b). The AIs of cross-reactive anti-Mc antibodies were in the range from 46.98 to 78.18%, usually exhibiting a 10–20% decrease of total avidity as compared with homologous counterparts. In contrast, the anti-OMPMc cross-reactive antibodies exhibited AIs which were either higher or comparable to homologous counterparts, and their values were often more than 90%. The results indicate that cross-reactive antibodies recognizing exclusively OMPs possess significantly stronger avidity and as a consequence lower dissociation potency than those which recognize whole-cell antigens.

Avidity was tested by whole-cell ELISA elution assay, using the chaotropic agent 1.5 M sodium thiocyanate (NaSCN) to disrupt weak antigen-antibody binding. In the assay, the parallel samples were tested in the presence or absence of NaSCN. Results are expressed as the mean AI ± SEM, where AI = (OD490 with NaSCN/OD490 without NaSCN) × 100. Significance of antibody binding in anti-OMPMc sera within homologous (a) or heterologous (b) strains in comparison to relevant anti-Mc sera was determined. Asterisks indicate statistically significant differences with *P < 0.00001, **P < 0.005 and ***P < 0.05 as determined by Student ‘s t- test for unpaired samples.
Complement-dependent bactericidal activity of cross-reactive antibodies
Antisera raised against the purified OMPs or whole bacteria were tested in vitro for their ability to promote complement-mediated killing of the homologous and heterologous M. catarrhalis isolates. A guinea pig serum was used as a source of complement. To avoid any false results, key controls were always included: no more than 10% killing when bacteria were incubated with guinea pig complement only, the complete abolition of complement-dependent killing in the presence of heat-inactivated complement serum and the lack of internal bactericidal activity among studied antisera. The results of complement-dependent bactericidal activity of studied antisera are presented in Table 3. As expected, all four OMP-specific and four Mc-specific antisera showed their ability to induce the complement-dependent killing of homologous isolates. The majority of these antisera demonstrated next different degrees of bactericidal activity against cross-reacting M. catarrhalis isolates. Complement-mediated bactericidal activity of anti-OMPs sera was generally more potent than that of anti-Mc sera. The bactericidal activity of antibodies in anti-OMP sera against heterologous isolates was only from 1.5 to 8.5 times weaker compared to homologous ones. In the case of anti-Mc sera that cross-reactivity was up to nearly 90 times weaker. Only two antisera, namely anti-Mc1 and anti-Mc8, in reaction against Mc5 showed a very low level of cross-reacting bactericidal activity. These results suggest that complement-dependent bactericidal activity of cross-reactive antibodies is driven principally by antibodies elicited in response to OMPs.
Complement-dependent bactericidal activity of pooled mice antisera specific against OMPs or whole bacteria assayed against homologous and heterologous M. catarrhalis isolates.
. | Serum bactericidal activity against . | |||
---|---|---|---|---|
Serum* . | M. catarrhalis isolates . | |||
. | Mc1 . | Mc5 . | Mc6 . | Mc8 . |
Anti-Mc1 | 276 | 3 | 261 | 75 |
Anti-Mc5 | 48 | 218 | 159 | 77 |
Anti-Mc6 | 24 | 234 | 601 | 317 |
Anti-Mc8 | 426 | 10 | 234 | 720 |
Anti-OMPMc1 | 695 | 246 | 105 | 460 |
Anti-OMPMc5 | 161 | 352 | 83 | 220 |
Anti-OMPMc6 | 571 | 720 | 1247 | 313 |
Anti-OMPMc8 | 376 | 103 | 234 | 886 |
Control serum | <3 | <3 | <3 | <3 |
. | Serum bactericidal activity against . | |||
---|---|---|---|---|
Serum* . | M. catarrhalis isolates . | |||
. | Mc1 . | Mc5 . | Mc6 . | Mc8 . |
Anti-Mc1 | 276 | 3 | 261 | 75 |
Anti-Mc5 | 48 | 218 | 159 | 77 |
Anti-Mc6 | 24 | 234 | 601 | 317 |
Anti-Mc8 | 426 | 10 | 234 | 720 |
Anti-OMPMc1 | 695 | 246 | 105 | 460 |
Anti-OMPMc5 | 161 | 352 | 83 | 220 |
Anti-OMPMc6 | 571 | 720 | 1247 | 313 |
Anti-OMPMc8 | 376 | 103 | 234 | 886 |
Control serum | <3 | <3 | <3 | <3 |
*The complement-dependent bactericidal killing was determined using the guinea pig serum as the exogenous complement source. The bactericidal titer was defined as the reciprocal value of the highest dilution of antiserum at which ≥50% killing of the target strain was observed, and is the geometric mean determination of bactericidal assays carried out in triplicate from at least two independent experiments. Bold numbers indicate homologous systems. Control serum refers to non-immunized group of mice.
Complement-dependent bactericidal activity of pooled mice antisera specific against OMPs or whole bacteria assayed against homologous and heterologous M. catarrhalis isolates.
. | Serum bactericidal activity against . | |||
---|---|---|---|---|
Serum* . | M. catarrhalis isolates . | |||
. | Mc1 . | Mc5 . | Mc6 . | Mc8 . |
Anti-Mc1 | 276 | 3 | 261 | 75 |
Anti-Mc5 | 48 | 218 | 159 | 77 |
Anti-Mc6 | 24 | 234 | 601 | 317 |
Anti-Mc8 | 426 | 10 | 234 | 720 |
Anti-OMPMc1 | 695 | 246 | 105 | 460 |
Anti-OMPMc5 | 161 | 352 | 83 | 220 |
Anti-OMPMc6 | 571 | 720 | 1247 | 313 |
Anti-OMPMc8 | 376 | 103 | 234 | 886 |
Control serum | <3 | <3 | <3 | <3 |
. | Serum bactericidal activity against . | |||
---|---|---|---|---|
Serum* . | M. catarrhalis isolates . | |||
. | Mc1 . | Mc5 . | Mc6 . | Mc8 . |
Anti-Mc1 | 276 | 3 | 261 | 75 |
Anti-Mc5 | 48 | 218 | 159 | 77 |
Anti-Mc6 | 24 | 234 | 601 | 317 |
Anti-Mc8 | 426 | 10 | 234 | 720 |
Anti-OMPMc1 | 695 | 246 | 105 | 460 |
Anti-OMPMc5 | 161 | 352 | 83 | 220 |
Anti-OMPMc6 | 571 | 720 | 1247 | 313 |
Anti-OMPMc8 | 376 | 103 | 234 | 886 |
Control serum | <3 | <3 | <3 | <3 |
*The complement-dependent bactericidal killing was determined using the guinea pig serum as the exogenous complement source. The bactericidal titer was defined as the reciprocal value of the highest dilution of antiserum at which ≥50% killing of the target strain was observed, and is the geometric mean determination of bactericidal assays carried out in triplicate from at least two independent experiments. Bold numbers indicate homologous systems. Control serum refers to non-immunized group of mice.
Correlation between cross-reacting antibodies and bactericidal titer
Relationships between serum subclass-specific/cross-reactive antibodies responsible for complement activation and complement-mediated bactericidal activity were analyzed (Fig. 3). There was a positive medium correlation (P < 0.01, r = 0.638) between the levels of IgG2a + IgG2b, recognizing the whole-cell antigens and the bactericidal titers of antibodies in anti-Mc sera when analyzing both homologous and heterologous responses. A comparable correlation was also demonstrated between the same immunoglobulin subclasses-specific against OMPs and bactericidal titers of anti-OMPMc sera (P < 0.001, r = 0.756). There were no correlations between each single subclass and bactericidal titer, suggesting that both subclasses recompense each other and that total activity of both subclasses is needed to exert their full functionality.
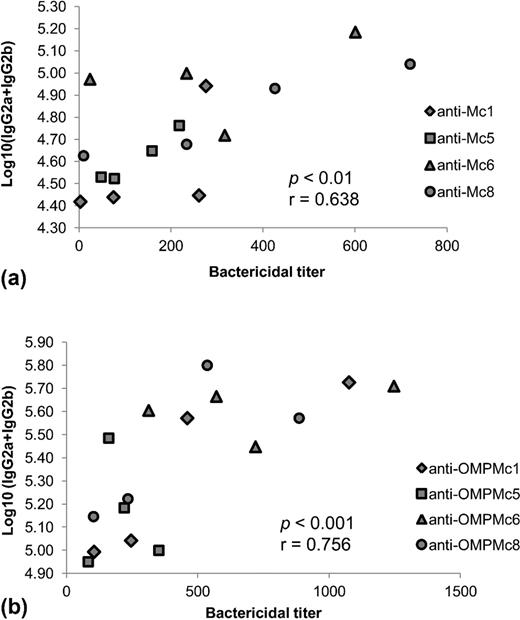
Relationship between serum subclass antibodies responsible for complement activation and complement-mediated bactericidal activity was analyzed by Spearman rank coefficient. Each spot represents a serum with the titer of bactericidal activity ( x-axis) and its corresponding titer of serum IgG2a + IgG2b expressed as mean log10 titer ( y-axis). There was a positive correlation between the total levels of IgG2a + IgG2b present in (a) anti-Mc sera or (b) anti-OMPMc sera and the bactericidal activities of those sera when analyzed both homologous and heterologous responses.
Opsonophagocytic activity of cross-reactive antibodies
The opsonophagocytosis test was performed in order to assess the functionality of anti-Mc and anti-OMPMc sera in triggering of opsonophagocytic (OPA) killing by PMA-differentiated THP-1 macrophages (Table 4). Opsonophagocytic killing activity of anti-OMP sera was generally more potent than that of anti-Mc sera. As can be seen, the OPA killing titers ranged approximately from 1:30 to 1:600 for the cross-reactive anti-OMPMc sera and from 1:30 to 1:300 for the cross-reactive anti-Mc sera. The stronger potency of homologous anti-OMPMc sera compared to anti-Mc sera was also confirmed by at least twice higher OPA titers found for the former. The opsonophagocytic killing activity of particular antisera within both anti-OMPMc and anti-Mc groups against heterologous isolates in reference to the relevant homologous strain was from 2 to 20 times weaker. The OPA killing activity of various cross-reactive anti-OMPMc and anti-Mc sera was often considerably stronger than that observed for control non-immunized serum. From these experiments, we conclude that the cross-reactive antibodies which recognize the whole-cell surface antigens and even more potent OMPs trigger the effective macrophage-mediated killing of some opsonized strains of M. catarrhalis.
Comparison of opsonophagocytic activities of anti-Mc and anti-OMPMc sera to homologous and heterologous M. catarrhalis isolates.
. | Opsonophagocytic activity against . | |||
---|---|---|---|---|
Serum* . | M. catarrhalis isolates . | |||
. | Mc1 . | Mc5 . | Mc6 . | Mc8 . |
Anti-Mc1 | 113 | 143 | 264 | <30 |
Anti-Mc5 | 150 | 600 | 327 | <30 |
Anti-Mc6 | <30 | 266 | 575 | ND |
Anti-Mc8 | 83 | 186 | <30 | 278 |
Anti-OMPMc1 | 517 | 112 | 569 | <30 |
Anti-OMPMc5 | 199 | 1200 | 300 | 110 |
Anti-OMPMc6 | 286 | 178 | 1200 | ND |
Anti-OMPMc8 | 50 | 283 | 290 | 668 |
Control serum | <30 | <30 | <30 | <30 |
. | Opsonophagocytic activity against . | |||
---|---|---|---|---|
Serum* . | M. catarrhalis isolates . | |||
. | Mc1 . | Mc5 . | Mc6 . | Mc8 . |
Anti-Mc1 | 113 | 143 | 264 | <30 |
Anti-Mc5 | 150 | 600 | 327 | <30 |
Anti-Mc6 | <30 | 266 | 575 | ND |
Anti-Mc8 | 83 | 186 | <30 | 278 |
Anti-OMPMc1 | 517 | 112 | 569 | <30 |
Anti-OMPMc5 | 199 | 1200 | 300 | 110 |
Anti-OMPMc6 | 286 | 178 | 1200 | ND |
Anti-OMPMc8 | 50 | 283 | 290 | 668 |
Control serum | <30 | <30 | <30 | <30 |
*The OPA killing was determined using THP-1 macrophages as effector cells. The OPA titer was determined for pooled antisera as the reciprocal value of the serum dilution at which the macrophage-dependent killing of at least 50% opsonized strain was observed. The presented results come from at least two independent experiments. Bold numbers indicate homologous systems.Control serum refers to non-immunized group of mice.
Comparison of opsonophagocytic activities of anti-Mc and anti-OMPMc sera to homologous and heterologous M. catarrhalis isolates.
. | Opsonophagocytic activity against . | |||
---|---|---|---|---|
Serum* . | M. catarrhalis isolates . | |||
. | Mc1 . | Mc5 . | Mc6 . | Mc8 . |
Anti-Mc1 | 113 | 143 | 264 | <30 |
Anti-Mc5 | 150 | 600 | 327 | <30 |
Anti-Mc6 | <30 | 266 | 575 | ND |
Anti-Mc8 | 83 | 186 | <30 | 278 |
Anti-OMPMc1 | 517 | 112 | 569 | <30 |
Anti-OMPMc5 | 199 | 1200 | 300 | 110 |
Anti-OMPMc6 | 286 | 178 | 1200 | ND |
Anti-OMPMc8 | 50 | 283 | 290 | 668 |
Control serum | <30 | <30 | <30 | <30 |
. | Opsonophagocytic activity against . | |||
---|---|---|---|---|
Serum* . | M. catarrhalis isolates . | |||
. | Mc1 . | Mc5 . | Mc6 . | Mc8 . |
Anti-Mc1 | 113 | 143 | 264 | <30 |
Anti-Mc5 | 150 | 600 | 327 | <30 |
Anti-Mc6 | <30 | 266 | 575 | ND |
Anti-Mc8 | 83 | 186 | <30 | 278 |
Anti-OMPMc1 | 517 | 112 | 569 | <30 |
Anti-OMPMc5 | 199 | 1200 | 300 | 110 |
Anti-OMPMc6 | 286 | 178 | 1200 | ND |
Anti-OMPMc8 | 50 | 283 | 290 | 668 |
Control serum | <30 | <30 | <30 | <30 |
*The OPA killing was determined using THP-1 macrophages as effector cells. The OPA titer was determined for pooled antisera as the reciprocal value of the serum dilution at which the macrophage-dependent killing of at least 50% opsonized strain was observed. The presented results come from at least two independent experiments. Bold numbers indicate homologous systems.Control serum refers to non-immunized group of mice.
Blocking activity of cross-reacting antibodies on bacterial adherence to the A549 cell line
In order to investigate the potency of cross-reactive antibodies in the inhibition of M. catarrhalis adherence to epithelial A549 cells, adhesion blocking assays were performed. Adhesion assays were conducted for isolates Mc1, Mc5 and Mc8, which differed in adhesion ability to the A549 cell line. The differences in adhesion ability for M. catarrhalis have been previously reported by others (Luke et al., 2007; Balder et al., 2009). The adherence of indicated bacteria to epithelial cells in the presence or absence of post-immunized mice sera was measured by the biotin–avidin-HRP ELISA method. This assay is based on the quantitative immunodetection of biotinylated adherent bacteria using avidin-HRP conjugate. To rule out the possibility that the biotin labeling affected the viability of M. catarrhalis strains, the appropriate experiments were performed. Biotin-labeled bacteria showed no marked differences in CFU after plating on agar plates when compared to the untreated control (data not shown). The blocking activities of anti-OMPMc sera were generally stronger than those of corresponding anti-Mc sera. As presented in Fig. 4a, incubation of bacteria with homologous antisera diluted 1:50 significantly reduced bacterial adherence to A549 cells by up to nearly 38% of the control in the presence of anti-OMPMc sera (P < 0.0005) and up to 32% in the presence of anti-Mc sera (P < 0.005) with only one exception related to anti-Mc5 in reaction with Mc5. The blocking activity of antisera on adhesion of heterologous M. catarrhalis strains, which allowed the potency of cross-reacting antibodies to be detected, was weaker, but generally still significant and led to a reduction of approximately 17.9–29.8% of the control bacterial attachment in the case of anti-OMPMc sera (0.005 > P > 0.0001) and from 8.5 to 21.6% attachment in the case of anti-Mc sera (0.05 > P > 0.001). Non-effective blocking was observed for one anti-OMPMc serum and for two anti-Mc sera. The results indicate the greater blocking potency of cross-reactive anti-OMPMc antibodies compared to anti-Mc antibodies. To confirm that blocking of bacterial adhesion is specifically targeted by post-immunized sera antibodies, we performed a series of control experiments. They showed that various control sera or albumin solutions at dilutions 1:20 and 1:100 showed no blocking activity on M. catarrhalis adherence to line A549 (Fig. 4b). Furthermore, to rule out the possibility that the apparent reduction in M. catarrhalis adherence was caused by epithelial cell death, their viability was assessed by trypan blue exclusion. There was no significant difference in the total number of non-viable cells after each treatment as compared to control A549 cells (data not shown). Overall, it was revealed that addition of antisera with cross-reactive antibodies recognizing either OMPs or whole surface antigens of M. catarrhalis led to a marked reduction of binding by M. catarrhalis to the A549 epithelial cell line. Our finding indicates an effective blocking potency of studied cross-reacting antibodies.
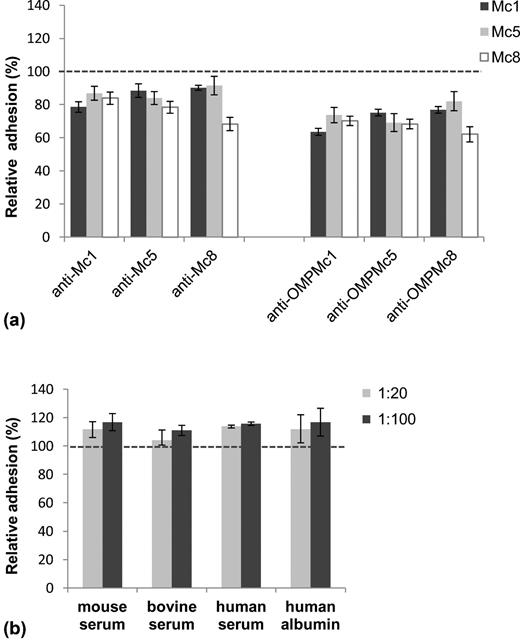
Blocking effect of homologous and cross-reactive antibodies on adhesion of M. catarrhalis clinical strains to A549 epithelial cell monolayer was determined by biotin–avidin-HRP ELISA. Bacterial adhesion in the absence of serum was used to established 100% binding ( dashed line). Relative adhesion was determined by calculating the absorbance at λ = 490 nm, reflecting the adhered bacteria as a proportion of the control adhesion. The error bars indicate SEM. Histogram (a) refers to inhibition of M. catarrhalis adherence by homologous or cross-reactive antibodies. The bacteria (∼ 2 × 108 CFU ml−1) were incubated with post-immunized sera at a 1:50 dilution. Histogram (b) refers to the lack of inhibition of the representative M. catarrhalis Mc1 adhesion in the presence of various non-specific sera and 5% (w/v) human albumin. The human serum contains no specific antibodies against M. catarrhalis as determined by whole-cell ELISA (titer < 1:100). The data are averages of the results from two independent experiments performed at least in triplicate. The differences between control adhesion and treatment condition adhesion were significant if P < 0.05 (one-way ANOVA followed by Tukey's post-hock test) and are given in the text.
Relationship of antibacterial antibodies and their protective functions as a result of homologous and heterologous reactivity
The distribution of protective functions of cross-reacting antibodies derived from anti-Mc and anti-OMPMc sera was further analyzed for all performed functional assays (Fig. 5). The positive threshold of protection for the adhesion assay was defined for all the results which were different from mean control results by more than 2 SD. For bactericidal and opsonophagocytic assays, this positive threshold of protection was arbitrarily accepted if cross-reacting activity exceeded 1:10 and 1:30 dilution of antisera, respectively. For all tested antibody-dependent functional assays, the protective function of cross-reactive antibodies recognizing the OMPs was much stronger than antibodies recognizing whole-cell antigens. However, by analyzing the cross-protective potency of antibodies against whole cells, which should reflect a more natural way of infection, it seems that complement-dependent killing is the more effective mechanism triggered by these antibodies against M. catarrhalis.
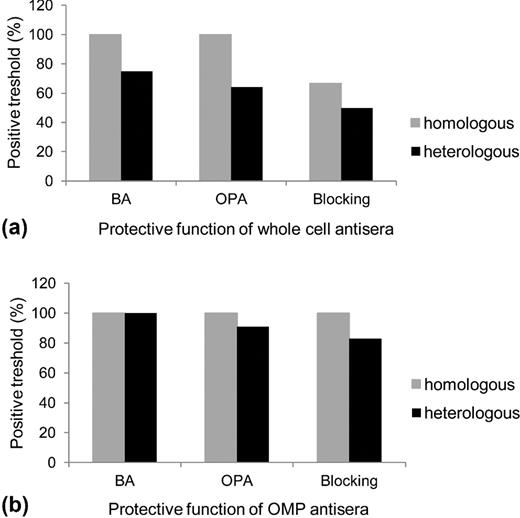
Relationship of antibodies recognizing cross-reactive M. catarrhalis epitopes and their biological functions. BA and OPA refer to complement-dependent bactericidal assay and Opsonophagocytic killing assay, respectively. The positive thresholds of all used pool of antisera for each studied functional assay in reference to homologous and heterologous strains are shown.
DISCUSSION
Cross-reacting antibodies are capable of eradicating pathogens primarily while cooperating with other pivotal effector mechanisms of innate immunity. In the present study, in response to immunization with M. catarrhalis antigens, we determined the broad-spectrum effectiveness of cross-reactive antibodies through evaluation of complement activation, opsonophagocytic-dependent killing and blocking of bacterial adhesion. We compared the immunogenicity of multivalent immunogens in order to better mimic the natural response of the host. Each murine antiserum was highly cross-reactive. The titers of cross-reactive antibodies from anti-OMPMc sera were much higher than those from anti-Mc sera, indicating that IgG antibodies recognizing exclusively OMPs of M. catarrhalis were more efficient. These antibodies possessed also significantly stronger and more homogeneous avidities compared to antibodies which recognized whole-cell surface-exposed epitopes. The similar avidity threshold of anti-OMPMc IgG against homo- and heterologous isolates may be the result of the following: (1) by definition the high degree of epitope conservation between tested strains, (2) similar accessibility of surface antigens for antibodies since differences in accessibility of some OMPs encoded by loci with high sequence conservation have been reported (Arenas et al., 2008), (3) a low level of variation in surface antigen expression.
On the other hand, the observed increase in avidity for anti-OMPMc antibodies also indicates the high quality of produced antibodies as a result of affinity maturation. We can speculate, therefore, that the induction of immune memory and preexisting protective antibodies engaged in recognition of cross-reactive epitopes may be in part an essential component of the defense strategy towards M. catarrhalis infection. The weaker immunogenicity of the antigenically more complex whole Moraxella cells may be the result of antigenic competition between highly immunogenic OMPs and LOS epitopes and in turn the generation of competitive highly polyvalent groups of antibodies.
The presence of a high number of cross-reacting antibodies for M. catarrhalis is not surprising considering the high number of common conserved surface antigens within M. catarrhalis strains.
Importantly, the antigen-binding abilities of antibodies per se and even quite high antibody level for cross-reactive antigens may not be sufficient to confer the relevant defense mechanism (Nahm, Olander and Magyarlaki 1997). Moreover, according to the data and conclusions of Verhaegh et al. (2008), it appears that vaccines directed against single immunogenic OMP of M. catarrhalis such as UspA1, UspA2, Hag and OMPCD as well as LOS type may be in part unsuitable for preventing infection in both children and adults. Therefore, only antibodies with defined functionality, produced in response to a strictly immunogenic and multivalent antigenic formulation and at a significant threshold, seem to be essential for widely understood protection of the host. In this study, we confirmed in vitro the protective efficacy of cross-reactive antibodies by performing three antibody-dependent antimicrobial assays. The broad functionality of cross-reactive anti-OMPMc and to a lesser degree anti-Mc antibodies was found since they (1) triggered killing of most heterologous isolates in a complement-dependent bactericidal assay, (2) enhanced in vitro opsonophagocytic killing activity of human THP-1 macrophages and (3) significantly blocked adhesion of heterologous isolates to the A549 alveolar epithelial cell line. The aforementioned antibodies are believed to play a quite different protective biological function during Moraxella infection. Serum bactericidal or opsonophagocytic antibodies are generally oriented to eradicate the pathogen as their production is correlated for example with otitis media resolution (Faden, Hong and Pahade 1994; Sun et al., 2000). Prevention rather than elimination is the hallmark of antibodies with anti-adhesive activity which are designed to prevent bacterial colonization on mucosal surfaces. Moreover, while the leading role of secretory IgA is here incontestable, significant amounts of IgG also appear to reach these surfaces (Twigg III 2005).
The presence of blocking activity of anti-OMPMc and anti-Mc cross-reactive antibodies could have clinical significance, since many M. catarrhalis adhesins including UspA1, UspA2, MID/Hag, OMPCD (LaFontaine et al., 2000; Forsgren et al., 2003; Holm et al., 2004) as well as LOS (Hu et al., 2001) could be anti-adhesive targets for both systemic and mucosal antibodies in adults with COPD (Murphy et al., 2005; LaFontaine et al., 2009). Since bacterial adhesion is multifactorial and dynamic in nature, its effective blockage by cross-reactive antibody molecules may confer partial protection and inhibit or at least make more difficult the colonization strategy of new M. catarrhalis strains.
The effective elimination of M. catarrhalis isolates by cross-reactive bactericidal or opsonophagocytic antibodies hypothetically could be important from the clinical point of view, particularly if we consider that the acquisition of new M. catarrhalis strains plays a central role in the pathogenesis of bacterial exacerbation in COPD patients (Sethi et al., 2002), and may be associated with the development of otitis media in children (Faden et al., 1997). One of the virulence strategy of M. catarrhalis is resistance to complement action, in part via its interplay with complement regulators. The Moraxella OMPs such as UspA2, OmpCD, OmpE and Cop B involved in that process (Su, Singh and Riesbeck 2012) are important antibody targets. Thus, it seems possible that the effective opsonization of at least a few of the proteins directly engaged in complement component resistance by cross-reactive bactericidal antibodies could lead to more effective complement activation. Next, since diminished/defective bacterial phagocytosis of macrophages has been documented in COPD patients (Taylor et al., 2010), the antibody-dependent enhancement of neutrophilic arm of this innate mechanism hypothetically should not be excluded. We documented recently the cooperation between humoral adaptive and humoral innate factors in neutrophilic phagocytic uptake of M. catarrhalis (Augustyniak et al., 2012).
Another M. catarrhalis virulence strategy is the release of outer membrane vesicles (OMVs) which can facilitate interbacterial transfer of periplasmic and outer membrane components and may have an impact on the establishment or duration of interspecies as well as intraspecies bacterial infection (Ünal, Schaar and Riesbeck 2011). A good example may be the promotion of Streptococcus pneumoniae or H. influenzae growth by M. catarrhalis-produced OMVs loaded with beta-lactamases. The enzyme, easily gained that way by the neighboring bacteria, promotes next their survival in the presence of relevant antibiotics (Schaar et al., 2011). It is worth adding that OMVs elicit a stable immune response against carried immunogenic components (Ünal, Schaar and Riesbeck 2011). In this context, the highly probable cross-reactive-antibody-dependent OMV neutralization could also have a beneficial influence on elimination or growth inhibition of other respiratory pathogens coexisting with M. catarrhalis. Moreover, OMVs in general are capable of inducing a proinflammatory response (Ünal, Schaar and Riesbeck 2011). We can speculate therefore that, similarly to the herein documented opsonization potential of cross-reactive antibodies which led to adhesion blocking, these antibodies should block OMVs, which in turn could hypothetically prevent the respiratory epithelium from undergoing Moraxella OMV internalization and an OMV-induced proinflammatory response (Schaar et al., 2011). Moreover, the release of M. catarrhalis, OMVs in the nasopharyngeal site of patients with M. catarrhalis infection have been observed (Tan et al., 2007). It would be interesting to find out whether antibodies directed against OMVs released by other heterologous isolates could have a cross-protective function in terms of faster depletion of OMVs from the environment in which they are formed or disseminated.
In summary, the observed quantitative and qualitative differences in immunogen-dependent (OMPMc versus Mc) induction of M. catarrhalis specific IgG antibodies with cross-reactive potency led to differences in protective potential between them. We found that antibodies designed to recognize exclusively OMPs had stronger bactericidal, opsonophagocytic and blocking activity. The immunoproteomic studies on specificity of OMPs that are targeted by these cross-reactive antibodies and give the protective effect are in progress using 2D electrophoresis and MALDI-TOF mass spectrometry analysis. An evaluation of their protective potency using a relevant animal model such as for example Fcγ receptor knockout mice or C1q / C3 deficient mice is further needed. While it remains to be established whether experiments with laboratory mice such as those presented here can be fully extrapolated to the human immune response, it appears that studying the protective potential of antibodies to multivalent immunogens, including those reflecting a more natural way of infection, together with the contribution of various mechanisms of action generated by these antibodies, may help to understand the role of cross-effective immunity to M. catarrhalis. The functional cross-reactive antibodies against M. catarrhalis may be an essential additional source to control host–microbe interaction.
FUNDING
This work was supported by research grant of University of Wroclaw (1016/S/IGiM/T-20).
Conflict of interest statement. None declared.
REFERENCES