-
PDF
- Split View
-
Views
-
Cite
Cite
Dongying Bai, Ryoma Nakao, Akihiro Ito, Hiroshi Uematsu, Hidenobu Senpuku, Immunoreactive antigens recognized in serum samples from mice intranasally immunized with Porphyromonas gingivalis outer membrane vesicles, Pathogens and Disease, Volume 73, Issue 3, April 2015, ftu006, https://doi.org/10.1093/femspd/ftu006
- Share Icon Share
Outer membrane vesicles (OMVs) of the periodontopathic bacterium Porphyromonas gingivalis contain a wide range of virulence factors including lipopolysaccharide (LPS), fimbriae and gingipains. We have recently reported strong immunogenicity of OMVs using an intranasal vaccine mouse model. In the present study, we performed sub-immunoproteome analysis of OMV-immunized mouse serum samples from six different mice in order to identify immunodominant antigens. The combination of two-dimensional (2D) gel electrophoresis and mass spectrometry analysis identified OMV proteins of 53 spots on a 2D map, and it was notable that OMV proteins were largely distributed within a low pH range, in marked contrast to the ubiquitous distribution of outer membrane proteins. Western blot using the six serum samples after 2D electrophoresis revealed that all showed immunoreactivity to some diffuse signals at extremely low pH, which was similar to the distribution of immunoreactive signals when the A-LPS antibody was used. Mass spectrometry analysis also demonstrated that the signals corresponded to a wide range of virulence factors including A-LPS-modified proteins such as gingipains. Absorption of serum with LPS resulted in a dramatic reduction of immmunoreactivity. We conclude that LPS and A-LPS-modified proteins in OMVs carry immunodominant determinants and eventually elicit P. gingivalis-specific antibodies in mice.
INTRODUCTION
Porphyromonas gingivalis, a black-pigmented Gram-negative anaerobic bacterium, is a major pathogen in periodontal diseases that possesses a diverse repertoire of virulence factors, including fimbriae, cysteine proteinases (gingipains) and lipopolysaccharide (LPS) (Darveau, Hajishengallis and Curtis 2012). Recent reports from epidemiological studies (Ishihara et al., 2004; Desvarieux et al., 2005; Ojima et al., 2005; Mikuls et al., 2014) as well as in vitro and animal model experiments (Dorn, Dunn and Progulske-Fox 1999; Lalla et al., 2000; Li et al., 2002) have shown an association between periodontitis and systemic diseases such as diabetes mellitus, cardiovascular disease, atherosclerosis and rheumatoid arthritis. Therefore, better understanding of the immunopathology of and vaccinology for P. gingivalis infections is highly desired.
Many studies have been conducted to investigate outer membrane vesicles (OMVs) produced by a wide range of Gram-negative bacteria (Beveridge 1999; Deatherage and Cookson 2012; MacDonald and Kuehn 2012). It is now commonly accepted that these small spherical structures (20–250 nm) are extruded from the outer membrane (OM) of bacterial cells and thus contain both OM and periplasmic components. OMVs possess not only an intrinsic inflammatory potential but also highly immunogenic properties due to their composition of cell wall constituents such as LPS, peptidoglycan and OM proteins. Therefore, OMVs as vehicles carrying virulence factors are regarded to be offensive players in microbial pathogenesis (MacDonald and Kuehn 2012), and several reports have shown that virulence factors are associated with OMVs in Gram-negative bacteria (Horstman and Kuehn 2000; Wai et al., 2003) including P. gingivalis (Grenier and Mayrand 1987; Haurat et al., 2011; Nakao et al.2014).
P. gingivalis possesses three different gingipains, two arginine-specific gingipains (RgpA and RgpB) and a lysine-specific gingipain (Kgp). Of these three, RgpA and Kgp are polyproteins comprised of several domains including an N-terminal signal peptide, a propeptide, a catalytic domain, several adhesin/hemagglutinin domains and a conserved C-terminal domain (CTD) (Bhogal, Slakeski and Reynolds 1997; Slakeski et al., 1998, 1999; DeCarlo et al., 1999; Veith et al., 2002; Seers et al., 2006). Grenier and Mayrand were the first to report the presence of gingipains in OMVs (Grenier and Mayrand 1987), after which Haurat et al. reported the selective sorting system of gingipains in OMVs of P. gingivalis (Haurat et al., 2011). We then further expanded available knowledge regarding the composition and functions of OMVs, and showed that P. gingivalis OMVs include multiple forms of gingipains and several gingipain-related proteins, and also that OMVs had strong antigenicity and cell-detachment activities against oral epithelial cells (Nakao et al., 2014). Furthermore, we previously demonstrated that many OMV proteins belong to conserved CTD family, which is comprised of a variety of virulence factors, not only gingipains but also gingipain-related proteins such as zinc carboxypeptidase CPG70 (Chen et al., 2002; Veith, Chen and Reynolds 2004), peptidylarginine deiminase PAD (McGraw et al., 1999) and hemagglutinin HagA (Savett and Progulske-Fox 1995). CTD proteins are usually observed as diffuse spots on an SDS-PAGE (sodium dodecyl sulfate-polyacrylamide gel electrophoresis) gel because of a post-translational modification with a unique LPS containing different lengths of anionic polysaccharides (A-LPS) (Curtis et al., 1999). The presence of a CTD is essential for translocation of the CTD protein across the OM (Seers et al., 2006; Shoji et al., 2011), though the CTD itself is released into culture medium prior to the maturation of CTD proteins (Glew et al., 2012).
OMVs have also been speculated for use in candidate vaccines for infectious diseases. For example, Neisseria meningitidis serogroup B OMV vaccines have been administered to humans in several countries, and demonstrated both safety and efficacy (Sexton et al., 2004). Furthermore, the immunogenic and protective properties of OMVs have been tested and proven for several bacterial species using in vivo assays, e.g. Vibrio cholera (Schild, Nelson and Camilli 2008; Bishop et al., 2010), Salmonella typhimurium (Alaniz et al., 2007), Borrelia burgdorferi (Whitmire and Garon 1993), Bordetella pertussis (Roberts et al., 2008) and P. gingivalis (Kesavalu et al., 1992). Kesavalu et al. have reported that parenteral immunization with 100 μg of P. gingivalis OMVs provided partial protection against both live bacterial and LPS challenges (Kesavalu et al., 1992). We reported that strong antibody responses were elicited in serum and saliva by intranasal immunization of only 1 μg of P. gingivalis OMVs in a mouse model (Nakao et al., 2011). In the present study, we aimed to identify immunoreactive antigens using serum samples of mice intranasally immunized with P. gingivalis OMVs. The scheme of the present study is overviewed in Fig. 1. Using a combination of sub-proteome and western blot (WB) analyses of serum samples from OMV-immunized mice, we found immunodominant antigens in our intranasal vaccine model. We also discuss the significance of the immunoreactive antigens found in OMVs and the feasibility of this vaccine system as a future prophylaxis strategy against periodontal diseases.
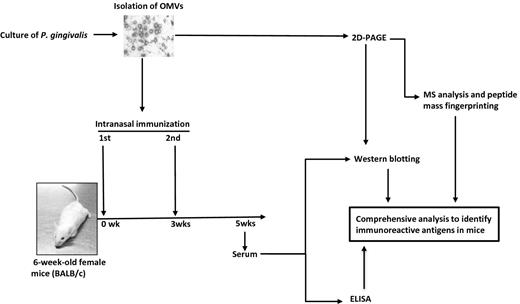
Overview of scheme of the present study. OMV fractions were prepared from culture supernatants of P. gingivalis strain ATCC 33277. The integrity and purity of the OMVs were confirmed by both typical protein profile using SDS-PAGE (data not shown) and spherical morphology shown by TEM. The OMV samples were separated by 2D-PAGE. The gel was stained with CBB, and then 53 spots were excised and used for MS analysis and PMF in order to systemically identify the OMV proteins. In parallel with protein identification, animal experiments were performed to obtain serum after intranasal immunization with OMVs. A strong reactivity of OMV-immunized serum to P. gingivalis was confirmed by ELISA. Finally, we identified the immunoreactive antigen using a combination of WB and MS analysis based on 2D-PAGE.
MATERIALS AND METHODS
Bacterial strains and culture conditions
P. gingivalis strain ATCC 33277 and an isogenic mfa1 mutant (Umemoto and Hamada 2003) were grown in brain heart infusion (BHI) broth supplemented with hemin and menadione (HM) or on BHI-HM blood agar plates in an anaerobic chamber (miniMACS anaerobic workstation; Don Whitley Scientific Ltd, Shipley, UK) in 80% N2, 10% H2 and 10% CO2 (Nakao, Senpuku and Watanabe 2006).
Isolation of OMVs, OM and LPS
P. gingivalis OMVs were isolated by a combination of filtration using a 0.22 μm Durapore (Millipore, Billerica, MA, USA) and ultracentrifugation, as described previously (Nakao et al., 2011). The differential detergent method was used to extract the OM of P. gingivalis, as described previously (Nakao et al., 2008). In this study, we use a Bradford assay and 2D Quant Kit (GE Healthcare Biosciences) as assays for protein-based quantification with bovine serum albumin used as the standard in both. P. gingivalis LPS was isolated using a hot phenol–water extraction method, as described previously (Nakao et al., 2006).
Transmission electron microscopy (TEM)
For TEM analysis, the OMV preparations were allowed to adhere to carbon-coated grids for 1 min at room temperature (15–24ºC), and then negatively stained with 2% uranyl acetate.TEM analysis was performed using an H-7650 (HITACHI, Tokyo, Japan).
Intranasal immunization
Intranasal immunization of mice with OMVs was performed as described previously (Nakao et al., 2011). In brief, female BALB/c mice, aged 6 to 8 weeks at the time of the first immunization, were used in all experiments. Six BALB/c mice were intranasally immunized with 1 μg of OMVs and 10 μg of polyriboinosinic polyribocytidylic acid [Poly (I:C)] (Sigma, St. Louis, MO) as an adjuvant, on day 0 and week 3. For control mice, the adjuvant alone was inoculated into nasal cavities on the same schedule. At 2 weeks after the second immunization, serum samples were collected. The study protocol was reviewed and approved by the animal research committee of the National Institute of Infectious Diseases, and all animal experiments were performed in accordance with our institutional guidelines.
Two-dimensional polyacrylamide gel electrophoresis (2D-PAGE)
After determining OMV amounts using a Bradford assay, OMVs (100 μg) were resuspended in a 1 ml lysis solution containing 1% SDS and a 1% protease inhibitor cocktail (Sigma) in 20 mM Tris-Cl (pH 8.0). Next, the OMVs were disrupted using a FastPrep system (MP Biomedicals, Santa Ana, CA, USA) with standardized conditions (power ‘6’, 30 s ×3 times at 1 min intervals). OMV components were purified by precipitation using 10% (w/v) trichloroacetic acid (TCA) followed by two washes with 80% acetone to remove TCA. The amounts of the resulting OMV proteins were measured again using a 2D Quanti Kit and standardized to 0.8 μg/μl in 50 μl of a solubilization buffer containing 5 M urea, 1 M thiourea, 1% ASB-14 (w/v) and 10 mg/ml dithiothreitol, and incubated for 1 h at room temperature (15–24°C) on a rotator. Isoelectric focusing of OMV samples was performed with a broad range (pH 3–10) or narrow range (pH 3–8) agarose gel containing carrier ampholytes (ATTO, Tokyo, Japan). A constant voltage of 300V was applied for 20 h. Immediately after focusing, the gels were gently shaken for 3 min with distilled water. The gels were then equilibrated for 5 min in 2.5% (w/v) TCA, followed by rinsing with distilled water for 2 h. In the second dimension, SDS-PAGE was employed using a precast 12.5% acrylamide gel (e-pagel, ATTO). After SDS-PAGE, some of the gels were visualized by staining with Bio-Safe Coomassie brilliant blue (CBB, Bio-Rad), while others were electro-transferred to PVDF membranes for WB analysis. Proteins transferred to the membrane were stained and destained using a MemCodeTM Reversible Protein Stain Kit (Thermo, Rockford, IL, USA), according to the manufacturer's instructions. The protein profile on the membrane was digitally recorded using a CanoScan 9000F (Canon, Tokyo, Japan) prior to WB. Densitometric analysis of CBB-stained protein spots on 2D-PAGE gels were performed using ImageJ software (National Institute of Health, Bethesda, MD). First, scanned color images of the 2D-PAGE gels were converted to gray scale. After subtraction of all the background signals, spots sized ≥10 pixel unit were detected. The signal intensity (integrated density) of each spot was eventually quantified using ImageJ algorithm.
Protein identification by MS and peptide mass fingerprinting (PMF), and bioinformatics analysis
CBB-stained protein spots were excised from the 2D-PAGE gels and subjected to in-gel digestion with trypsin. Tryptic peptide samples were analyzed by liquid chromatography–tandem mass spectrometry (LC-MS/MS), LTQ (Thermo) and the MS data were analyzed using the Mascot search engine (v2.4.1, Matrix Science, Inc., Boston, MA, USA) against the protein database of the P. gingivalis strain ATCC 33277. Peptide mass tolerance for Mascot analysis was set at ±1.5 Da and fragment mass tolerance at ±0.8 Da, in consideration of one missing cleavage by trypsin, carbamidomethylation of cysteine and oxidation of methionine. The applications PSORTb ver. 3.0.2, SignalP ver. 4.0, LipoP ver. 1.0 and Compute pI/Mw Tool of ExPASy were used for predicting the subcellular localization, presence and location of signal peptide cleavage sites in the amino acid sequences, and theoretical pI (isoelectric point)/MW (molecular weight), respectively.
ELISA
Whole-cell ELISA
The reactivity of mouse sera to whole cells of P. gingivalis was tested using an ELISA described previously (Nakao et al., 2011). Briefly, freeze-dried whole cells were weighed and used as an antigen for whole-cell ELISA. ELISA plates were coated with 10 μg of freeze-dried bacteria resuspended in 100 μl of 50 mM carbonate/bicarbonate buffer (pH 9.6) per well. After overnight blocking at 4ºC with 1% skim milk in PBS with 0.05% Tween 20 (PBS-T), each serum sample was 4-fold serially diluted with 0.5% skim milk in PBS-T, added to the wells, and incubated for 1 h at 37ºC. The wells were then incubated for 1 h at 37ºC with alkaline phosphatase-conjugated goat anti-mouse IgG (Invitrogen, Carlsbad, CA, USA) at a dilution of 1:1000. Subsequently, the P. gingivalis-specific antibody was detected by chromogenic development using para-nitrophenyl phosphate as the alkaline phosphatase substrate. Absorbance at 405 nm was measured after 15 min. The endpoint titers were defined as the maximum dilution of examined serum samples that showed absorbance greater than 0.05 at 405 nm (A405) as compared to the average absorbance of two mock-immunized mice.
OMV-coating ELISA
ELISA was performed using P. gingivalis OMVs as a coating antigen, as described previously (Nakao et al., 2014). OMVs at 100 ng/well were coated onto ELISA plates. Except for antigen coating, ELISA of OMVs was performed in the same manner as described in the section ‘whole-cell ELISA’.
Western blotting (WB)
After electro-transfer of proteins on the PAGE gels to PVDF membranes, the membranes were blocked with 1% skim milk in PBST overnight at 4ºC and then incubated with mouse sera diluted at different concentrations with PBST containing 0.1% skim milk. Rabbit polyclonal antiserum against both FimA and Mfa1 (a kind gift from Dr Nobushiro Hamada) was used at a dilution of 1:1000. Mouse monoclonal antiserum against A-LPS and rabbit polyclonal antiserum against the catalytic domain of RgpA (RgpAcat) (a kind gift from Dr Michael A. Curtis) were used at dilutions of 1:1000 and 1:200, respectively. HRP-labeled donkey anti-rabbit antiserum IgG (GE Healthcare, Buckinghamshire, UK) and HRP-labeled goat anti-mouse IgG (GE Healthcare) were used as secondary antibodies at 1:200 000 and 1:20 000 dilution, respectively, and visualized using the chemiluminescent reagent ECLplus (Thermo). Signals on developed X-ray films were recorded by a CanoScan 9000F (Canon) and superimposed onto MemCodeTM-stained PVDF membrane images as noted in the section ‘Two-dimensional polyacrylamide gel electrophoresis (2D-PAGE)’ using Photoshop CS4 image editing software (Adobe, San Jose, CA, USA).
LPS absorption assay and LPS ELISA
LPS, unlike protein antigens, is unable to adhere to ELISA plate wells. Hence, for serum absorption by LPS, we used polymyxin B (PMB)-based ELISA (LPS ELISA) to capture LPS via the specific interaction between PMB and the lipid A portion of LPS. We performed the LPS ELISA, as described previously (Nakao et al., 2014) and evaluated the efficacy of serum absorption by LPS. In brief, a PMB solution (100 μl) at a concentration of 50 μg/ml in a carbonate/bicarbonate buffer was added to multiple wells of ELISA plates and incubated for 16 h at 37ºC. After washing with PBST six times to remove unattached PMB, the same volume of P. gingivalis LPS solution at a concentration of 1 mg/ml was added to the wells and incubated for 30 min at 37ºC. After blocking with skim milk, mouse sera were added to the PMB/LPS-coated wells to absorb the LPS-specific antibody and incubated for 1 h at 37ºC. After absorption, mouse sera were collected from the wells and added to other PMB/LPS-coated wells. These absorption/collection steps were repeated four times. Non-absorbed OMV-1 serum and three times absorbed OMV-1 serum were subjected to WB using OMVs resolved by 2D-PAGE in order to determine whether the WB signals decreased after absorption.
Statistical analysis
Statistical analysis was performed using one-way analysis of variance (ANOVA) followed by Dunnett's multiple comparison test or Mann–Whitney's U-test. P values of 0.05 or less were considered to indicate statistical significance.
RESULTS
Wide range of OMV proteins were resolved with 2D-PAGE and mostly focused at low isoelectric points
OMVs were prepared from culture supernatants of P. gingivalis strain ATCC 33277, as described in the section ‘materials and methods’. TEM analysis findings confirmed the structural integrity of the isolated OMVs, i.e. spherical morphology and relatively homogeneous size (approximately 50–80 nm in diameter) (Fig. 2), consistent with our previous observations (Nakao et al., 2011). We also observed the fimbriae as fine structures with a thickness of approximately 5 nm (shown by arrowheads in Fig. 2), which appeared to be associated with the OMVs. The purity of the OMVs was also assessed based on the protein profile on SDS-PAGE gels. The protein profiling was reproducible (data not shown) and identical to our previous report (Nakao et al.2014).
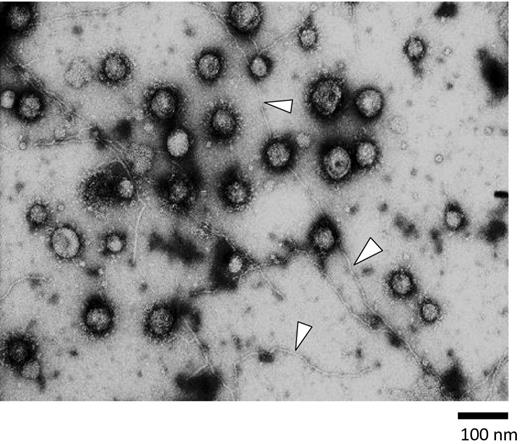
Shown is a representative electron micrograph of a strain ATCC 33277 OMV preparation. Samples were negatively stained with uranyl acetate and visualized at x 50 000. Many OMVs were clearly visible as spherical structures with different sizes ranging from 50 to 150 nm in diameter. Fimbrial structures were also found as long and fine structures approximately 5 nm in thickness (arrowheads). Bar: 100 nm.
First, the purified OMVs were resolved by 2D-PAGE using a broad range (pH 3–10) agarose gel for 1D (isoelectric focusing) and 12% polyacrylamide SDS-PAGE for 2D. Most of the OMV proteins were observed in the low pH range on the 2D map (Fig. 3A). Therefore, we also performed 2D-PAGE using a narrow range (pH 3–8) gel for 1D, resulting in good separation of the proteins with low pI (Fig. 3B). Fifty-three CBB-stained spots on 2D gels were numbered, and then picked from those gels and resolved using both the wide (pH 3–10) and narrower (pH 3–8) range gels. The peptides digested by trypsin were analyzed by LC-MS/MS and PMF. All identified proteins with Mascot score greater than 100 and more than 3 peptide hits are listed in Table S1. The list of the 53 spots with the highest Mascot score for each spot is shown in Table 1. In total, 61 different proteins were successfully identified in the 53 spots on the 2D-PAGE of the OMV sample. Of these 61 proteins, 20 possessed a conserved CTD, of which 4, PGN_0458, PGN_0654, PGN_0693 and PGN_1611, were conserved hypothetical proteins. Sixteen annotated CTD proteins, por secretion system (PorSS) protein PorU (PGN_0022), peptidylarginine deiminase PAD (PGN_0898), lysine-specific gingipain Kgp (PGN_1728), lysyl endopeptidase precursor (PGN_1416), hemin binding protein HBP35 (PGN_0659), arginine-specific gingipains RgpA (PGN_1970) and RgpB (PGN_1466), hemagglutinin HagA (PGN_1733), immunoreactive 47 kDa antigen (PGN_0852), immunoreactive 61 kDa antigen (TapA, PGN_0152), immunoreactive 46 kDa antigen (PGN_1767), immunoreactive 84 kDa antigen (PGN_0509), serine protease (PGN_0561), thiol protease (PGN_0900), zinc carboxypeptidase CPG70 (PGN_0335), and the C-terminal domain of Arg- and Lys-gingipain proteinase (PGN_0295), were identified in this study. Table 1 and Table S1 present the information from the results of several in silico predictions, including cellular localization, presence/length of signal peptides, and theoretical pI and molecular weights, as well as from a recent proteome research report regarding OMVs of strain W83 (Veith et al., 2014). Prediction of cellular localization using the PSORTb program revealed that most of the identified proteins were predicted to be on the OM or in an extracellular location when the prediction was successful.
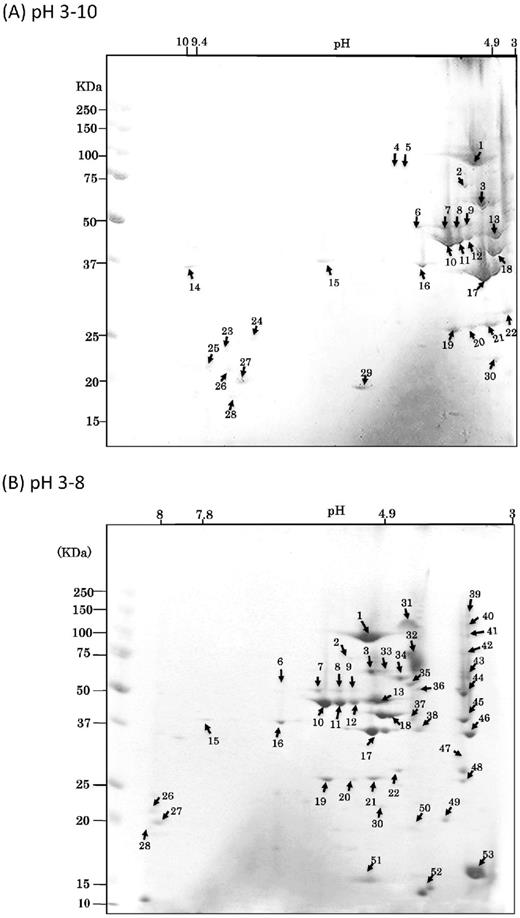
CBB-stained 2D reference maps with pH ranging from 3–10 (A) to 3–8 (B). Analyzed protein spots are noted by numbers that refer to Table 1.
Spot . | Protein names#2 . | Locus tags in . | Locus tags in . | Mascot . | Peptide . | Subcellular . | Localization . | Presence/length of . | lipoprotein . | Theoretical . |
---|---|---|---|---|---|---|---|---|---|---|
nos.#1 . | . | ATCC 33277 . | W83 . | scores . | numbers . | localization#5 . | in OMVs#6 . | signal peptide#7 . | signal . | pI/Mw#9 . |
. | . | database#3 . | database#4 . | . | . | . | . | . | peptide#8 . | . |
1 | receptor antigen A, RagA | PGN_0293 | PG_0185 | 3404 | 237 | OM | VM | Yes/20 | NO | 5.59 / 112196.92 |
2 | minor componet FimD | PGN_0184 | PG_2135 | 1082 | 30 | U | ND | NO | NO | 6.19 / 75848.09 |
3 | Mfa1 fimbrillin | PGN_0287 | ND | 1291 | 92 | U | ND | NO | Yes/19 | 5.62 / 60786.40 |
4 | TonB-linked receptor Tlr | PGN_0683 | PG_1552 | 1731 | 39 | OM | VM | Yes/19 | NO | 7.31 / 76544.35 |
5 | TonB-linked receptor Tlr | PGN_0683 | PG_1552 | 1315 | 28 | OM | VM | Yes/19 | NO | 7.31 / 76544.35 |
6 | minor compent FimE | PGN_0185 | PG_2136 | 1598 | 43 | U | ND | Yes/24 | Yes/21 | 6.17 / 58060.40 |
7 | hypothetical protein PGN_0477 | PGN_0477 | PG_1626 | 1734 | 54 | OM | VM | Yes/33 | NO | 6.07 / 57581.94 |
8 | lysine-specific cysteine proteinase Kgp | PGN_1728 | PG_1844 | 1366 | 38 | EC | EC-CTD | Yes/21 | NO | 4.95 / 185049.40 |
9 | hypothetical protein PGN_0477 | PGN_0477 | PG_1626 | 1459 | 22 | OM | VM | Yes/33 | NO | 6.07 / 57581.94 |
10 | lysine-specific cysteine proteinase Kgp | PGN_1728 | PG_1844 | 5419 | 126 | EC | EC-CTD | Yes/21 | NO | 4.95 / 185049.40 |
11 | lysine-specific cysteine proteinase Kgp | PGN_1728 | PG_1844 | 1418 | 116 | EC | EC-CTD | Yes/21 | NO | 4.95 / 185049.40 |
12 | lysine-specific cysteine proteinase Kgp | PGN_1728 | PG_1844 | 1326 | 45 | EC | EC-CTD | Yes/21 | NO | 4.95 / 185049.40 |
13 | receptor antigen B, RagB | PGN_0294 | PG_0186 | 2224 | 137 | U | VM(L) | Yes/24 | Yes/19 | 5.30 / 54143.04 |
14 | Por secretion system protein porN/gldN | PGN_1673 | PG_0291 | 186 | 4 | U | UL | Yes/22 | NO | 9.39 / 39290.31 |
15 | Omp41 precursor | PGN_0729 | PG_0695 | 272 | 6 | OM | VM | Yes/20 | NO | 7.88 / 41300.66 |
16 | Omp40 precursor | PGN_0728 | PG_0694 | 1251 | 38 | OM | VM | Yes/21 | Yes/14 | 6.44 / 40370.50 |
17 | FimA type I fimbrillin | PGN_0180 | PG_2132 | 1250 | 164 | EC | VM(L) | Yes/20 | Yes/18 | 5.40 / 39211.97 |
18 | arginine-specific cysteine proteinase RgpA | PGN_1970 | PG_2024 | 1551 | 89 | EC | EC-CTD | Yes/20 | NO | 4.96 / 183282.09 |
19 | hypothetical protein PGN_1557 | PGN_1557 | PG_0409 | 779 | 28 | U | VM | Yes/21 | NO | 5.94 / 27289.94 |
20 | heme-binding protein FetB | PGN_0705 | PG_0669 | 795 | 16 | U | VM(L) | Yes/23 | Yes/18 | 5.58 / 30189.26 |
21 | heme-binding protein FetB | PGN_0705 | PG_0669 | 1449 | 55 | U | VM(L) | Yes/23 | Yes/18 | 5.58 / 30189.26 |
22 | hypothetical protein PGN_0290 | PGN_0290 | PG_0181 | 1361 | 51 | U | VM(L) | NO | NO | 5.32 / 37110.19 |
23 | hypothetical protein PGN_0860 | PGN_0860 | PG_1382 | 587 | 11 | U | VM | Yes/21 | Yes/19 | 8.54 / 36516.32 |
24 | Omp41 precursor | PGN_0729 | PG_0695 | 706 | 16 | OM | VM | Yes/20 | NO | 7.88 / 41300.66 |
25 | hypothetical protein PGN_0156 | PGN_0156 | PG_2106 | 891 | 29 | U | VM | Yes/22 | NO | 9.12 / 22105.45 |
26 | hypothetical protein PGN_1998 | PGN_1998 | PG_2054 | 674 | 24 | OM | VM(L) | Yes/18 | Yes/19 | 8.75 / 20828.41 |
27 | hypothetical protein PGN_1744 | PGN_1744 | PG_1823 | 940 | 36 | U | VM | Yes/43 | NO | 8.82 / 19941.71 |
28 | Omp41 precursor | PGN_0729 | PG_0695 | 650 | 15 | OM | VM | Yes/20 | NO | 7.88 / 41300.66 |
29 | hypothetical protein PGN_1744 | PGN_1744 | PG_1823 | 155 | 4 | U | VM | Yes/43 | NO | 8.82 / 19941.71 |
30 | Omp41 precursor | PGN_0729 | PG_0695 | 816 | 29 | OM | VM | Yes/20 | NO | 7.88 / 41300.66 |
31 | zinc carboxypeptidase CPG70 | PGN_0335 | PG_0232 | 1515 | 76 | U | EC-CTD | Yes/24 | NO | 5.07 / 88849.44 |
32 | arginine-specific cysteine proteinase RgpB | PGN_1466 | PG_0506 | 1372 | 72 | U | EC-CTD | Yes/24 | Yes/19 | 5.34 / 78372.87 |
33 | lysine-specific cysteine proteinase Kgp | PGN_1728 | PG_1844 | 1163 | 36 | EC | EC-CTD | Yes/21 | NO | 4.95 / 185049.40 |
34 | TPR domain protein | PGN_0876 | PG_1385 | 1304 | 31 | U | VL | Yes/21 | NO | 5.17 / 43621.43 |
35 | lysine-specific cysteine proteinase Kgp | PGN_1728 | PG_1844 | 1590 | 49 | EC | EC-CTD | Yes/21 | NO | 4.95 / 185049.40 |
36 | arginine-specific cysteine proteinase RgpA | PGN_1970 | PG_2024 | 873 | 37 | EC | EC-CTD | Yes/20 | NO | 4.96 / 183282.09 |
37 | TPR domain protein | PGN_0876 | PG_1385 | 1579 | 68 | U | VL | Yes/21 | NO | 5.17 / 43621.43 |
38 | Por secretion system protein porV (pg27, lptO) | PGN_0023 | PG_0027 | 1377 | 58 | OM | VM | Yes/26 | NO | 4.76 / 40312.12 |
39 | arginine-specific cysteine proteinase RgpA | PGN_1970 | PG_2024 | 870 | 28 | EC | EC-CTD | Yes/20 | NO | 4.96 / 183282.09 |
40 | arginine-specific cysteine proteinase RgpA | PGN_1970 | PG_2024 | 1144 | 35 | EC | EC-CTD | Yes/20 | NO | 4.96 / 183282.09 |
41 | lysine-specific cysteine proteinase Kgp | PGN_1728 | PG_1844 | 635 | 15 | EC | EC-CTD | Yes/21 | NO | 4.95 / 185049.40 |
42 | arginine-specific cysteine proteinase RgpA | PGN_1970 | PG_2024 | 902 | 38 | EC | EC-CTD | Yes/20 | NO | 4.96 / 183282.09 |
43 | arginine-specific cysteine proteinase RgpA | PGN_1970 | PG_2024 | 1032 | 40 | EC | EC-CTD | Yes/20 | NO | 4.96 / 183282.09 |
44 | arginine-specific cysteine proteinase RgpA | PGN_1970 | PG_2024 | 1163 | 54 | EC | EC-CTD | Yes/20 | NO | 4.96 / 183282.09 |
45 | arginine-specific cysteine proteinase RgpA | PGN_1970 | PG_2024 | 1173 | 60 | EC | EC-CTD | Yes/20 | NO | 4.96 / 183282.09 |
46 | arginine-specific cysteine proteinase RgpA | PGN_1970 | PG_2024 | 608 | 35 | EC | EC-CTD | Yes/20 | NO | 4.96 / 183282.09 |
47 | hemagglutinin protein HagA | PGN_1733 | PG_1837 | 594 | 27 | U | EC-CTD | Yes/24 | Yes/17 | 4.60 / 280580.19 |
48 | hemagglutinin protein HagA | PGN_1733 | PG_1837 | 438 | 40 | U | EC-CTD | Yes/24 | Yes/17 | 4.60 / 280580.19 |
49 | immunoreactive 46 kDa antigen | PGN_1767 | PG_1798 | 797 | 44 | U | EC-CTD | U | NO | 5.66 / 47696.75 |
50 | hypothetical protein PGN_1816 | PGN_1816 | PG_1889 | 481 | 24 | U | VM(L) | NO | Yes/20 | 5.65 / 24995.63 |
51 | hypothetical protein PGN_1129 | PGN_1129 | PG_1341 | 709 | 49 | U | VM(L) | Yes/18 | Yes/19 | 6.38 / 15644.45 |
52 | hemagglutinin protein HagA | PGN_1733 | PG_1837 | 561 | 21 | U | EC-CTD | Yes/24 | Yes/17 | 4.60 / 280580.19 |
53 | lysine-specific cysteine proteinase Kgp | PGN_1728 | PG_1844 | 607 | 20 | EC | EC-CTD | Yes/21 | NO | 4.95 / 185049.40 |
Spot . | Protein names#2 . | Locus tags in . | Locus tags in . | Mascot . | Peptide . | Subcellular . | Localization . | Presence/length of . | lipoprotein . | Theoretical . |
---|---|---|---|---|---|---|---|---|---|---|
nos.#1 . | . | ATCC 33277 . | W83 . | scores . | numbers . | localization#5 . | in OMVs#6 . | signal peptide#7 . | signal . | pI/Mw#9 . |
. | . | database#3 . | database#4 . | . | . | . | . | . | peptide#8 . | . |
1 | receptor antigen A, RagA | PGN_0293 | PG_0185 | 3404 | 237 | OM | VM | Yes/20 | NO | 5.59 / 112196.92 |
2 | minor componet FimD | PGN_0184 | PG_2135 | 1082 | 30 | U | ND | NO | NO | 6.19 / 75848.09 |
3 | Mfa1 fimbrillin | PGN_0287 | ND | 1291 | 92 | U | ND | NO | Yes/19 | 5.62 / 60786.40 |
4 | TonB-linked receptor Tlr | PGN_0683 | PG_1552 | 1731 | 39 | OM | VM | Yes/19 | NO | 7.31 / 76544.35 |
5 | TonB-linked receptor Tlr | PGN_0683 | PG_1552 | 1315 | 28 | OM | VM | Yes/19 | NO | 7.31 / 76544.35 |
6 | minor compent FimE | PGN_0185 | PG_2136 | 1598 | 43 | U | ND | Yes/24 | Yes/21 | 6.17 / 58060.40 |
7 | hypothetical protein PGN_0477 | PGN_0477 | PG_1626 | 1734 | 54 | OM | VM | Yes/33 | NO | 6.07 / 57581.94 |
8 | lysine-specific cysteine proteinase Kgp | PGN_1728 | PG_1844 | 1366 | 38 | EC | EC-CTD | Yes/21 | NO | 4.95 / 185049.40 |
9 | hypothetical protein PGN_0477 | PGN_0477 | PG_1626 | 1459 | 22 | OM | VM | Yes/33 | NO | 6.07 / 57581.94 |
10 | lysine-specific cysteine proteinase Kgp | PGN_1728 | PG_1844 | 5419 | 126 | EC | EC-CTD | Yes/21 | NO | 4.95 / 185049.40 |
11 | lysine-specific cysteine proteinase Kgp | PGN_1728 | PG_1844 | 1418 | 116 | EC | EC-CTD | Yes/21 | NO | 4.95 / 185049.40 |
12 | lysine-specific cysteine proteinase Kgp | PGN_1728 | PG_1844 | 1326 | 45 | EC | EC-CTD | Yes/21 | NO | 4.95 / 185049.40 |
13 | receptor antigen B, RagB | PGN_0294 | PG_0186 | 2224 | 137 | U | VM(L) | Yes/24 | Yes/19 | 5.30 / 54143.04 |
14 | Por secretion system protein porN/gldN | PGN_1673 | PG_0291 | 186 | 4 | U | UL | Yes/22 | NO | 9.39 / 39290.31 |
15 | Omp41 precursor | PGN_0729 | PG_0695 | 272 | 6 | OM | VM | Yes/20 | NO | 7.88 / 41300.66 |
16 | Omp40 precursor | PGN_0728 | PG_0694 | 1251 | 38 | OM | VM | Yes/21 | Yes/14 | 6.44 / 40370.50 |
17 | FimA type I fimbrillin | PGN_0180 | PG_2132 | 1250 | 164 | EC | VM(L) | Yes/20 | Yes/18 | 5.40 / 39211.97 |
18 | arginine-specific cysteine proteinase RgpA | PGN_1970 | PG_2024 | 1551 | 89 | EC | EC-CTD | Yes/20 | NO | 4.96 / 183282.09 |
19 | hypothetical protein PGN_1557 | PGN_1557 | PG_0409 | 779 | 28 | U | VM | Yes/21 | NO | 5.94 / 27289.94 |
20 | heme-binding protein FetB | PGN_0705 | PG_0669 | 795 | 16 | U | VM(L) | Yes/23 | Yes/18 | 5.58 / 30189.26 |
21 | heme-binding protein FetB | PGN_0705 | PG_0669 | 1449 | 55 | U | VM(L) | Yes/23 | Yes/18 | 5.58 / 30189.26 |
22 | hypothetical protein PGN_0290 | PGN_0290 | PG_0181 | 1361 | 51 | U | VM(L) | NO | NO | 5.32 / 37110.19 |
23 | hypothetical protein PGN_0860 | PGN_0860 | PG_1382 | 587 | 11 | U | VM | Yes/21 | Yes/19 | 8.54 / 36516.32 |
24 | Omp41 precursor | PGN_0729 | PG_0695 | 706 | 16 | OM | VM | Yes/20 | NO | 7.88 / 41300.66 |
25 | hypothetical protein PGN_0156 | PGN_0156 | PG_2106 | 891 | 29 | U | VM | Yes/22 | NO | 9.12 / 22105.45 |
26 | hypothetical protein PGN_1998 | PGN_1998 | PG_2054 | 674 | 24 | OM | VM(L) | Yes/18 | Yes/19 | 8.75 / 20828.41 |
27 | hypothetical protein PGN_1744 | PGN_1744 | PG_1823 | 940 | 36 | U | VM | Yes/43 | NO | 8.82 / 19941.71 |
28 | Omp41 precursor | PGN_0729 | PG_0695 | 650 | 15 | OM | VM | Yes/20 | NO | 7.88 / 41300.66 |
29 | hypothetical protein PGN_1744 | PGN_1744 | PG_1823 | 155 | 4 | U | VM | Yes/43 | NO | 8.82 / 19941.71 |
30 | Omp41 precursor | PGN_0729 | PG_0695 | 816 | 29 | OM | VM | Yes/20 | NO | 7.88 / 41300.66 |
31 | zinc carboxypeptidase CPG70 | PGN_0335 | PG_0232 | 1515 | 76 | U | EC-CTD | Yes/24 | NO | 5.07 / 88849.44 |
32 | arginine-specific cysteine proteinase RgpB | PGN_1466 | PG_0506 | 1372 | 72 | U | EC-CTD | Yes/24 | Yes/19 | 5.34 / 78372.87 |
33 | lysine-specific cysteine proteinase Kgp | PGN_1728 | PG_1844 | 1163 | 36 | EC | EC-CTD | Yes/21 | NO | 4.95 / 185049.40 |
34 | TPR domain protein | PGN_0876 | PG_1385 | 1304 | 31 | U | VL | Yes/21 | NO | 5.17 / 43621.43 |
35 | lysine-specific cysteine proteinase Kgp | PGN_1728 | PG_1844 | 1590 | 49 | EC | EC-CTD | Yes/21 | NO | 4.95 / 185049.40 |
36 | arginine-specific cysteine proteinase RgpA | PGN_1970 | PG_2024 | 873 | 37 | EC | EC-CTD | Yes/20 | NO | 4.96 / 183282.09 |
37 | TPR domain protein | PGN_0876 | PG_1385 | 1579 | 68 | U | VL | Yes/21 | NO | 5.17 / 43621.43 |
38 | Por secretion system protein porV (pg27, lptO) | PGN_0023 | PG_0027 | 1377 | 58 | OM | VM | Yes/26 | NO | 4.76 / 40312.12 |
39 | arginine-specific cysteine proteinase RgpA | PGN_1970 | PG_2024 | 870 | 28 | EC | EC-CTD | Yes/20 | NO | 4.96 / 183282.09 |
40 | arginine-specific cysteine proteinase RgpA | PGN_1970 | PG_2024 | 1144 | 35 | EC | EC-CTD | Yes/20 | NO | 4.96 / 183282.09 |
41 | lysine-specific cysteine proteinase Kgp | PGN_1728 | PG_1844 | 635 | 15 | EC | EC-CTD | Yes/21 | NO | 4.95 / 185049.40 |
42 | arginine-specific cysteine proteinase RgpA | PGN_1970 | PG_2024 | 902 | 38 | EC | EC-CTD | Yes/20 | NO | 4.96 / 183282.09 |
43 | arginine-specific cysteine proteinase RgpA | PGN_1970 | PG_2024 | 1032 | 40 | EC | EC-CTD | Yes/20 | NO | 4.96 / 183282.09 |
44 | arginine-specific cysteine proteinase RgpA | PGN_1970 | PG_2024 | 1163 | 54 | EC | EC-CTD | Yes/20 | NO | 4.96 / 183282.09 |
45 | arginine-specific cysteine proteinase RgpA | PGN_1970 | PG_2024 | 1173 | 60 | EC | EC-CTD | Yes/20 | NO | 4.96 / 183282.09 |
46 | arginine-specific cysteine proteinase RgpA | PGN_1970 | PG_2024 | 608 | 35 | EC | EC-CTD | Yes/20 | NO | 4.96 / 183282.09 |
47 | hemagglutinin protein HagA | PGN_1733 | PG_1837 | 594 | 27 | U | EC-CTD | Yes/24 | Yes/17 | 4.60 / 280580.19 |
48 | hemagglutinin protein HagA | PGN_1733 | PG_1837 | 438 | 40 | U | EC-CTD | Yes/24 | Yes/17 | 4.60 / 280580.19 |
49 | immunoreactive 46 kDa antigen | PGN_1767 | PG_1798 | 797 | 44 | U | EC-CTD | U | NO | 5.66 / 47696.75 |
50 | hypothetical protein PGN_1816 | PGN_1816 | PG_1889 | 481 | 24 | U | VM(L) | NO | Yes/20 | 5.65 / 24995.63 |
51 | hypothetical protein PGN_1129 | PGN_1129 | PG_1341 | 709 | 49 | U | VM(L) | Yes/18 | Yes/19 | 6.38 / 15644.45 |
52 | hemagglutinin protein HagA | PGN_1733 | PG_1837 | 561 | 21 | U | EC-CTD | Yes/24 | Yes/17 | 4.60 / 280580.19 |
53 | lysine-specific cysteine proteinase Kgp | PGN_1728 | PG_1844 | 607 | 20 | EC | EC-CTD | Yes/21 | NO | 4.95 / 185049.40 |
#1: The corresponding spot number on the reference on 2D-PAGE protein map in Fig. 3. The proteins with highest mascot score were shown in the list.
#2: The names of proteins in bold represent CTD proteins.
#3: The accession number in the genome sequence of P. gingivalis ATCC 33277.
#4: The accession number in the genome sequence of P. gingivalis W83.ND; not detected.
#5: Cellular localization predicted by PSORTb program. EC; extracellular, OM; outer membrane, PP; periplasm, IM; inner membrane, CP; cytoplasm, U; unknown.
#6: Localization in OMVs which was predicted and reported by Veith P.D. et al. in 2014. VM; vesicle membrane, VM(L); vesicle membrane (lipoproteins), VL; vesicle lumen, EC-CTD; Extracellular CTD-containing proteins, UL; uncertain location, ND; not detected.
#7: Presence/length of signal peptides predicted by SignalP program.
#8: Lipoprotein signal peptides predicted by LipoP program.
#9: Theoretical pI/molecular weight predicted by ExPaSy.
Spot . | Protein names#2 . | Locus tags in . | Locus tags in . | Mascot . | Peptide . | Subcellular . | Localization . | Presence/length of . | lipoprotein . | Theoretical . |
---|---|---|---|---|---|---|---|---|---|---|
nos.#1 . | . | ATCC 33277 . | W83 . | scores . | numbers . | localization#5 . | in OMVs#6 . | signal peptide#7 . | signal . | pI/Mw#9 . |
. | . | database#3 . | database#4 . | . | . | . | . | . | peptide#8 . | . |
1 | receptor antigen A, RagA | PGN_0293 | PG_0185 | 3404 | 237 | OM | VM | Yes/20 | NO | 5.59 / 112196.92 |
2 | minor componet FimD | PGN_0184 | PG_2135 | 1082 | 30 | U | ND | NO | NO | 6.19 / 75848.09 |
3 | Mfa1 fimbrillin | PGN_0287 | ND | 1291 | 92 | U | ND | NO | Yes/19 | 5.62 / 60786.40 |
4 | TonB-linked receptor Tlr | PGN_0683 | PG_1552 | 1731 | 39 | OM | VM | Yes/19 | NO | 7.31 / 76544.35 |
5 | TonB-linked receptor Tlr | PGN_0683 | PG_1552 | 1315 | 28 | OM | VM | Yes/19 | NO | 7.31 / 76544.35 |
6 | minor compent FimE | PGN_0185 | PG_2136 | 1598 | 43 | U | ND | Yes/24 | Yes/21 | 6.17 / 58060.40 |
7 | hypothetical protein PGN_0477 | PGN_0477 | PG_1626 | 1734 | 54 | OM | VM | Yes/33 | NO | 6.07 / 57581.94 |
8 | lysine-specific cysteine proteinase Kgp | PGN_1728 | PG_1844 | 1366 | 38 | EC | EC-CTD | Yes/21 | NO | 4.95 / 185049.40 |
9 | hypothetical protein PGN_0477 | PGN_0477 | PG_1626 | 1459 | 22 | OM | VM | Yes/33 | NO | 6.07 / 57581.94 |
10 | lysine-specific cysteine proteinase Kgp | PGN_1728 | PG_1844 | 5419 | 126 | EC | EC-CTD | Yes/21 | NO | 4.95 / 185049.40 |
11 | lysine-specific cysteine proteinase Kgp | PGN_1728 | PG_1844 | 1418 | 116 | EC | EC-CTD | Yes/21 | NO | 4.95 / 185049.40 |
12 | lysine-specific cysteine proteinase Kgp | PGN_1728 | PG_1844 | 1326 | 45 | EC | EC-CTD | Yes/21 | NO | 4.95 / 185049.40 |
13 | receptor antigen B, RagB | PGN_0294 | PG_0186 | 2224 | 137 | U | VM(L) | Yes/24 | Yes/19 | 5.30 / 54143.04 |
14 | Por secretion system protein porN/gldN | PGN_1673 | PG_0291 | 186 | 4 | U | UL | Yes/22 | NO | 9.39 / 39290.31 |
15 | Omp41 precursor | PGN_0729 | PG_0695 | 272 | 6 | OM | VM | Yes/20 | NO | 7.88 / 41300.66 |
16 | Omp40 precursor | PGN_0728 | PG_0694 | 1251 | 38 | OM | VM | Yes/21 | Yes/14 | 6.44 / 40370.50 |
17 | FimA type I fimbrillin | PGN_0180 | PG_2132 | 1250 | 164 | EC | VM(L) | Yes/20 | Yes/18 | 5.40 / 39211.97 |
18 | arginine-specific cysteine proteinase RgpA | PGN_1970 | PG_2024 | 1551 | 89 | EC | EC-CTD | Yes/20 | NO | 4.96 / 183282.09 |
19 | hypothetical protein PGN_1557 | PGN_1557 | PG_0409 | 779 | 28 | U | VM | Yes/21 | NO | 5.94 / 27289.94 |
20 | heme-binding protein FetB | PGN_0705 | PG_0669 | 795 | 16 | U | VM(L) | Yes/23 | Yes/18 | 5.58 / 30189.26 |
21 | heme-binding protein FetB | PGN_0705 | PG_0669 | 1449 | 55 | U | VM(L) | Yes/23 | Yes/18 | 5.58 / 30189.26 |
22 | hypothetical protein PGN_0290 | PGN_0290 | PG_0181 | 1361 | 51 | U | VM(L) | NO | NO | 5.32 / 37110.19 |
23 | hypothetical protein PGN_0860 | PGN_0860 | PG_1382 | 587 | 11 | U | VM | Yes/21 | Yes/19 | 8.54 / 36516.32 |
24 | Omp41 precursor | PGN_0729 | PG_0695 | 706 | 16 | OM | VM | Yes/20 | NO | 7.88 / 41300.66 |
25 | hypothetical protein PGN_0156 | PGN_0156 | PG_2106 | 891 | 29 | U | VM | Yes/22 | NO | 9.12 / 22105.45 |
26 | hypothetical protein PGN_1998 | PGN_1998 | PG_2054 | 674 | 24 | OM | VM(L) | Yes/18 | Yes/19 | 8.75 / 20828.41 |
27 | hypothetical protein PGN_1744 | PGN_1744 | PG_1823 | 940 | 36 | U | VM | Yes/43 | NO | 8.82 / 19941.71 |
28 | Omp41 precursor | PGN_0729 | PG_0695 | 650 | 15 | OM | VM | Yes/20 | NO | 7.88 / 41300.66 |
29 | hypothetical protein PGN_1744 | PGN_1744 | PG_1823 | 155 | 4 | U | VM | Yes/43 | NO | 8.82 / 19941.71 |
30 | Omp41 precursor | PGN_0729 | PG_0695 | 816 | 29 | OM | VM | Yes/20 | NO | 7.88 / 41300.66 |
31 | zinc carboxypeptidase CPG70 | PGN_0335 | PG_0232 | 1515 | 76 | U | EC-CTD | Yes/24 | NO | 5.07 / 88849.44 |
32 | arginine-specific cysteine proteinase RgpB | PGN_1466 | PG_0506 | 1372 | 72 | U | EC-CTD | Yes/24 | Yes/19 | 5.34 / 78372.87 |
33 | lysine-specific cysteine proteinase Kgp | PGN_1728 | PG_1844 | 1163 | 36 | EC | EC-CTD | Yes/21 | NO | 4.95 / 185049.40 |
34 | TPR domain protein | PGN_0876 | PG_1385 | 1304 | 31 | U | VL | Yes/21 | NO | 5.17 / 43621.43 |
35 | lysine-specific cysteine proteinase Kgp | PGN_1728 | PG_1844 | 1590 | 49 | EC | EC-CTD | Yes/21 | NO | 4.95 / 185049.40 |
36 | arginine-specific cysteine proteinase RgpA | PGN_1970 | PG_2024 | 873 | 37 | EC | EC-CTD | Yes/20 | NO | 4.96 / 183282.09 |
37 | TPR domain protein | PGN_0876 | PG_1385 | 1579 | 68 | U | VL | Yes/21 | NO | 5.17 / 43621.43 |
38 | Por secretion system protein porV (pg27, lptO) | PGN_0023 | PG_0027 | 1377 | 58 | OM | VM | Yes/26 | NO | 4.76 / 40312.12 |
39 | arginine-specific cysteine proteinase RgpA | PGN_1970 | PG_2024 | 870 | 28 | EC | EC-CTD | Yes/20 | NO | 4.96 / 183282.09 |
40 | arginine-specific cysteine proteinase RgpA | PGN_1970 | PG_2024 | 1144 | 35 | EC | EC-CTD | Yes/20 | NO | 4.96 / 183282.09 |
41 | lysine-specific cysteine proteinase Kgp | PGN_1728 | PG_1844 | 635 | 15 | EC | EC-CTD | Yes/21 | NO | 4.95 / 185049.40 |
42 | arginine-specific cysteine proteinase RgpA | PGN_1970 | PG_2024 | 902 | 38 | EC | EC-CTD | Yes/20 | NO | 4.96 / 183282.09 |
43 | arginine-specific cysteine proteinase RgpA | PGN_1970 | PG_2024 | 1032 | 40 | EC | EC-CTD | Yes/20 | NO | 4.96 / 183282.09 |
44 | arginine-specific cysteine proteinase RgpA | PGN_1970 | PG_2024 | 1163 | 54 | EC | EC-CTD | Yes/20 | NO | 4.96 / 183282.09 |
45 | arginine-specific cysteine proteinase RgpA | PGN_1970 | PG_2024 | 1173 | 60 | EC | EC-CTD | Yes/20 | NO | 4.96 / 183282.09 |
46 | arginine-specific cysteine proteinase RgpA | PGN_1970 | PG_2024 | 608 | 35 | EC | EC-CTD | Yes/20 | NO | 4.96 / 183282.09 |
47 | hemagglutinin protein HagA | PGN_1733 | PG_1837 | 594 | 27 | U | EC-CTD | Yes/24 | Yes/17 | 4.60 / 280580.19 |
48 | hemagglutinin protein HagA | PGN_1733 | PG_1837 | 438 | 40 | U | EC-CTD | Yes/24 | Yes/17 | 4.60 / 280580.19 |
49 | immunoreactive 46 kDa antigen | PGN_1767 | PG_1798 | 797 | 44 | U | EC-CTD | U | NO | 5.66 / 47696.75 |
50 | hypothetical protein PGN_1816 | PGN_1816 | PG_1889 | 481 | 24 | U | VM(L) | NO | Yes/20 | 5.65 / 24995.63 |
51 | hypothetical protein PGN_1129 | PGN_1129 | PG_1341 | 709 | 49 | U | VM(L) | Yes/18 | Yes/19 | 6.38 / 15644.45 |
52 | hemagglutinin protein HagA | PGN_1733 | PG_1837 | 561 | 21 | U | EC-CTD | Yes/24 | Yes/17 | 4.60 / 280580.19 |
53 | lysine-specific cysteine proteinase Kgp | PGN_1728 | PG_1844 | 607 | 20 | EC | EC-CTD | Yes/21 | NO | 4.95 / 185049.40 |
Spot . | Protein names#2 . | Locus tags in . | Locus tags in . | Mascot . | Peptide . | Subcellular . | Localization . | Presence/length of . | lipoprotein . | Theoretical . |
---|---|---|---|---|---|---|---|---|---|---|
nos.#1 . | . | ATCC 33277 . | W83 . | scores . | numbers . | localization#5 . | in OMVs#6 . | signal peptide#7 . | signal . | pI/Mw#9 . |
. | . | database#3 . | database#4 . | . | . | . | . | . | peptide#8 . | . |
1 | receptor antigen A, RagA | PGN_0293 | PG_0185 | 3404 | 237 | OM | VM | Yes/20 | NO | 5.59 / 112196.92 |
2 | minor componet FimD | PGN_0184 | PG_2135 | 1082 | 30 | U | ND | NO | NO | 6.19 / 75848.09 |
3 | Mfa1 fimbrillin | PGN_0287 | ND | 1291 | 92 | U | ND | NO | Yes/19 | 5.62 / 60786.40 |
4 | TonB-linked receptor Tlr | PGN_0683 | PG_1552 | 1731 | 39 | OM | VM | Yes/19 | NO | 7.31 / 76544.35 |
5 | TonB-linked receptor Tlr | PGN_0683 | PG_1552 | 1315 | 28 | OM | VM | Yes/19 | NO | 7.31 / 76544.35 |
6 | minor compent FimE | PGN_0185 | PG_2136 | 1598 | 43 | U | ND | Yes/24 | Yes/21 | 6.17 / 58060.40 |
7 | hypothetical protein PGN_0477 | PGN_0477 | PG_1626 | 1734 | 54 | OM | VM | Yes/33 | NO | 6.07 / 57581.94 |
8 | lysine-specific cysteine proteinase Kgp | PGN_1728 | PG_1844 | 1366 | 38 | EC | EC-CTD | Yes/21 | NO | 4.95 / 185049.40 |
9 | hypothetical protein PGN_0477 | PGN_0477 | PG_1626 | 1459 | 22 | OM | VM | Yes/33 | NO | 6.07 / 57581.94 |
10 | lysine-specific cysteine proteinase Kgp | PGN_1728 | PG_1844 | 5419 | 126 | EC | EC-CTD | Yes/21 | NO | 4.95 / 185049.40 |
11 | lysine-specific cysteine proteinase Kgp | PGN_1728 | PG_1844 | 1418 | 116 | EC | EC-CTD | Yes/21 | NO | 4.95 / 185049.40 |
12 | lysine-specific cysteine proteinase Kgp | PGN_1728 | PG_1844 | 1326 | 45 | EC | EC-CTD | Yes/21 | NO | 4.95 / 185049.40 |
13 | receptor antigen B, RagB | PGN_0294 | PG_0186 | 2224 | 137 | U | VM(L) | Yes/24 | Yes/19 | 5.30 / 54143.04 |
14 | Por secretion system protein porN/gldN | PGN_1673 | PG_0291 | 186 | 4 | U | UL | Yes/22 | NO | 9.39 / 39290.31 |
15 | Omp41 precursor | PGN_0729 | PG_0695 | 272 | 6 | OM | VM | Yes/20 | NO | 7.88 / 41300.66 |
16 | Omp40 precursor | PGN_0728 | PG_0694 | 1251 | 38 | OM | VM | Yes/21 | Yes/14 | 6.44 / 40370.50 |
17 | FimA type I fimbrillin | PGN_0180 | PG_2132 | 1250 | 164 | EC | VM(L) | Yes/20 | Yes/18 | 5.40 / 39211.97 |
18 | arginine-specific cysteine proteinase RgpA | PGN_1970 | PG_2024 | 1551 | 89 | EC | EC-CTD | Yes/20 | NO | 4.96 / 183282.09 |
19 | hypothetical protein PGN_1557 | PGN_1557 | PG_0409 | 779 | 28 | U | VM | Yes/21 | NO | 5.94 / 27289.94 |
20 | heme-binding protein FetB | PGN_0705 | PG_0669 | 795 | 16 | U | VM(L) | Yes/23 | Yes/18 | 5.58 / 30189.26 |
21 | heme-binding protein FetB | PGN_0705 | PG_0669 | 1449 | 55 | U | VM(L) | Yes/23 | Yes/18 | 5.58 / 30189.26 |
22 | hypothetical protein PGN_0290 | PGN_0290 | PG_0181 | 1361 | 51 | U | VM(L) | NO | NO | 5.32 / 37110.19 |
23 | hypothetical protein PGN_0860 | PGN_0860 | PG_1382 | 587 | 11 | U | VM | Yes/21 | Yes/19 | 8.54 / 36516.32 |
24 | Omp41 precursor | PGN_0729 | PG_0695 | 706 | 16 | OM | VM | Yes/20 | NO | 7.88 / 41300.66 |
25 | hypothetical protein PGN_0156 | PGN_0156 | PG_2106 | 891 | 29 | U | VM | Yes/22 | NO | 9.12 / 22105.45 |
26 | hypothetical protein PGN_1998 | PGN_1998 | PG_2054 | 674 | 24 | OM | VM(L) | Yes/18 | Yes/19 | 8.75 / 20828.41 |
27 | hypothetical protein PGN_1744 | PGN_1744 | PG_1823 | 940 | 36 | U | VM | Yes/43 | NO | 8.82 / 19941.71 |
28 | Omp41 precursor | PGN_0729 | PG_0695 | 650 | 15 | OM | VM | Yes/20 | NO | 7.88 / 41300.66 |
29 | hypothetical protein PGN_1744 | PGN_1744 | PG_1823 | 155 | 4 | U | VM | Yes/43 | NO | 8.82 / 19941.71 |
30 | Omp41 precursor | PGN_0729 | PG_0695 | 816 | 29 | OM | VM | Yes/20 | NO | 7.88 / 41300.66 |
31 | zinc carboxypeptidase CPG70 | PGN_0335 | PG_0232 | 1515 | 76 | U | EC-CTD | Yes/24 | NO | 5.07 / 88849.44 |
32 | arginine-specific cysteine proteinase RgpB | PGN_1466 | PG_0506 | 1372 | 72 | U | EC-CTD | Yes/24 | Yes/19 | 5.34 / 78372.87 |
33 | lysine-specific cysteine proteinase Kgp | PGN_1728 | PG_1844 | 1163 | 36 | EC | EC-CTD | Yes/21 | NO | 4.95 / 185049.40 |
34 | TPR domain protein | PGN_0876 | PG_1385 | 1304 | 31 | U | VL | Yes/21 | NO | 5.17 / 43621.43 |
35 | lysine-specific cysteine proteinase Kgp | PGN_1728 | PG_1844 | 1590 | 49 | EC | EC-CTD | Yes/21 | NO | 4.95 / 185049.40 |
36 | arginine-specific cysteine proteinase RgpA | PGN_1970 | PG_2024 | 873 | 37 | EC | EC-CTD | Yes/20 | NO | 4.96 / 183282.09 |
37 | TPR domain protein | PGN_0876 | PG_1385 | 1579 | 68 | U | VL | Yes/21 | NO | 5.17 / 43621.43 |
38 | Por secretion system protein porV (pg27, lptO) | PGN_0023 | PG_0027 | 1377 | 58 | OM | VM | Yes/26 | NO | 4.76 / 40312.12 |
39 | arginine-specific cysteine proteinase RgpA | PGN_1970 | PG_2024 | 870 | 28 | EC | EC-CTD | Yes/20 | NO | 4.96 / 183282.09 |
40 | arginine-specific cysteine proteinase RgpA | PGN_1970 | PG_2024 | 1144 | 35 | EC | EC-CTD | Yes/20 | NO | 4.96 / 183282.09 |
41 | lysine-specific cysteine proteinase Kgp | PGN_1728 | PG_1844 | 635 | 15 | EC | EC-CTD | Yes/21 | NO | 4.95 / 185049.40 |
42 | arginine-specific cysteine proteinase RgpA | PGN_1970 | PG_2024 | 902 | 38 | EC | EC-CTD | Yes/20 | NO | 4.96 / 183282.09 |
43 | arginine-specific cysteine proteinase RgpA | PGN_1970 | PG_2024 | 1032 | 40 | EC | EC-CTD | Yes/20 | NO | 4.96 / 183282.09 |
44 | arginine-specific cysteine proteinase RgpA | PGN_1970 | PG_2024 | 1163 | 54 | EC | EC-CTD | Yes/20 | NO | 4.96 / 183282.09 |
45 | arginine-specific cysteine proteinase RgpA | PGN_1970 | PG_2024 | 1173 | 60 | EC | EC-CTD | Yes/20 | NO | 4.96 / 183282.09 |
46 | arginine-specific cysteine proteinase RgpA | PGN_1970 | PG_2024 | 608 | 35 | EC | EC-CTD | Yes/20 | NO | 4.96 / 183282.09 |
47 | hemagglutinin protein HagA | PGN_1733 | PG_1837 | 594 | 27 | U | EC-CTD | Yes/24 | Yes/17 | 4.60 / 280580.19 |
48 | hemagglutinin protein HagA | PGN_1733 | PG_1837 | 438 | 40 | U | EC-CTD | Yes/24 | Yes/17 | 4.60 / 280580.19 |
49 | immunoreactive 46 kDa antigen | PGN_1767 | PG_1798 | 797 | 44 | U | EC-CTD | U | NO | 5.66 / 47696.75 |
50 | hypothetical protein PGN_1816 | PGN_1816 | PG_1889 | 481 | 24 | U | VM(L) | NO | Yes/20 | 5.65 / 24995.63 |
51 | hypothetical protein PGN_1129 | PGN_1129 | PG_1341 | 709 | 49 | U | VM(L) | Yes/18 | Yes/19 | 6.38 / 15644.45 |
52 | hemagglutinin protein HagA | PGN_1733 | PG_1837 | 561 | 21 | U | EC-CTD | Yes/24 | Yes/17 | 4.60 / 280580.19 |
53 | lysine-specific cysteine proteinase Kgp | PGN_1728 | PG_1844 | 607 | 20 | EC | EC-CTD | Yes/21 | NO | 4.95 / 185049.40 |
#1: The corresponding spot number on the reference on 2D-PAGE protein map in Fig. 3. The proteins with highest mascot score were shown in the list.
#2: The names of proteins in bold represent CTD proteins.
#3: The accession number in the genome sequence of P. gingivalis ATCC 33277.
#4: The accession number in the genome sequence of P. gingivalis W83.ND; not detected.
#5: Cellular localization predicted by PSORTb program. EC; extracellular, OM; outer membrane, PP; periplasm, IM; inner membrane, CP; cytoplasm, U; unknown.
#6: Localization in OMVs which was predicted and reported by Veith P.D. et al. in 2014. VM; vesicle membrane, VM(L); vesicle membrane (lipoproteins), VL; vesicle lumen, EC-CTD; Extracellular CTD-containing proteins, UL; uncertain location, ND; not detected.
#7: Presence/length of signal peptides predicted by SignalP program.
#8: Lipoprotein signal peptides predicted by LipoP program.
#9: Theoretical pI/molecular weight predicted by ExPaSy.
Of the proteins listed in Table 1, RgpA and Kgp were frequently identified and widely distributed with different molecular weights and in a low pH range. As both RgpA and Kgp are polyproteins comprising several domains, we searched the domains of RgpA (Table S2, Fig. S1) and Kgp (Table S3, Fig. S2) using distribution analysis of tryptic peptides in the amino sequences of the corresponding proteins. As expected, only a few tryptic peptides were found in the propeptide domains of both RgpA and Kgp, while many tryptic peptides in the catalytic and adhesin/hemagglutinin domains were detected in both RgpA and Kgp (Tables S2 and S3). Results of distribution analysis showed that 2D-PAGE could successfully discriminate between the catalytic and adhesin/hemagglutinin domains in RgpA and Kgp by virtue of the difference in isoelectric points between the catalytic and adhesin/hemagglutinin domains in both RgpA and Kgp. All 20 identified CTD proteins including RgpA and Kgp were predicted by SignalP program to possess N-terminal signal peptides; however, no tryptic peptide was detected in the sequences of N-terminal signal peptides in any of the CTD proteins (data not shown). With the exception of the CTD of the immunoreactive 61 kDa antigen (TapA, PGN_0152) and the C-terminal domain of Arg- and Lys-gingipain proteinase (PGN_0295), no tryptic peptide was detected in the CTD sequence (data not shown). In the CTD of TapA (PGN_0152), three peptides and one peptide were detected in spot nos. 34 and 35, respectively. Also, three peptides were detected in the CTD of the C-terminal domain of Arg- and Lys-gingipain proteinase (PGN_0295) in spot no. 48.
We also performed 2D-PAGE and WB using antibodies against the catalytic domain of RgpA (RgpAcat) (Fig. S3[A]) and A-LPS (Fig. S3[B]). By superimposing the developed X-ray film after WB using the corresponding antibodies (α-RgpAcat and α-A-LPS) onto the original membrane stained with MemCodeTM, we found that α-RgpAcat strongly reacted with nos. 32 and 18, and weakly reacted with nos. 31, 34 and 35. On the other hand, α-A-LPS reacted with many diffuse spots at a low pH range including no. 32, but not with no. 18. Therefore, the WB results (Fig. S3[A], [B]) were found to be consistent with the MS analysis results (Table 1, S1), i.e. the diffuse spot no. 32 corresponded to RgpB modified with different length of A-LPS, and the relatively sharp spot no. 18 was unmodified form of RgpAcat. Several spots with extremely low pI (nos. 39–46) were also diffusely immunorecognized with α-A-LPS but not with α-RgpAcat (Fig. S3[A], [B]), in good agreement with the results of MS analysis, which these spots had signals identified as adhesin/hemagglutinin domains but not catalytic domains of RgpA and Kgp (Figs S1 and S2; Tables S2 and S3).
Spot nos. 17 and 3 were identified as major and minor fimbrial proteins (FimA and Mfa1), respectively. By superimposing the developed X-ray film after WB using the specific antibodies against FimA and Mfa1 onto the original membrane stained with MemCodeTM, we confirmed that no. 17 corresponded to FimA (Fig. S3[C]) and no. 3 to Mfa1 (Fig. S3[D]).
We also compared the protein profile of OMVs with that of the OM using 2D-PAGE (Fig. 4). Seven major proteins commonly observed in both OMVs and OM samples were selected, and the signal densities of the selected spots were measured. We found that the integrated densities of Kgp and FimA in the OMVs were much higher than those of corresponding proteins in the OM, while the signal ratio of Omp40/41 to total proteins was nearly 10-fold weaker in the OMVs. In addition, it is notable that the OMV proteins were mostly distributed within a low pH range, in marked contrast to their ubiquitous distribution of the OM proteins.
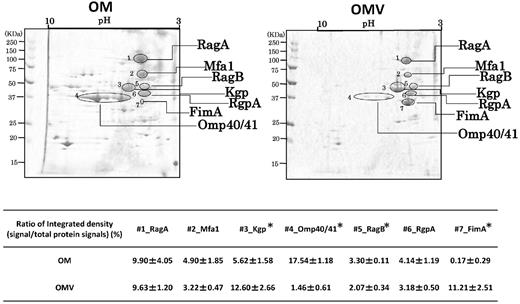
Comparison of protein profiles between OMVs and OM resolved by 2D-PAGE. Scanned images of MemCodeTM-stained membranes of OMVs and OM are shown in the left and right columns, respectively. Selected major signals (RagA, Mfa1, RagB, RgpAcat, FimA, Kgp, Omp40/41) are denoted by lines with the corresponding names. Images shown are representative of the results of three independent experiments using three OMV and three OM samples prepared from different batch cultures, each of which showed similar. Signals from the protein spots of both OMVs and OM proteins were quantified by densitometry with ImageJ. In the lower tables, ratios of signal intensity of each protein to total signal intensity are shown as the mean ± SD from three independent experiments. Statistical analysis was performed using Mann–Whitney's U-test. Asterisks indicate that the ratio was significantly different between OMV and OM.
Identification of spots showing immunoreaction with sera from mice intranasally immunized with OMVs
In parallel with protein identification, animal experiments were performed using our previously established OMV immunization model (Nakao et al., 2011). Serum samples were collected from mice and examined for immunoreactivity to P. gingivalis LPS as well as whole cells by ELISA (Table 2). We found that all tested OMV-immunized mice (OMV-1, -2, -3, -4, -5 and -6) strongly elicited P. gingivalis-specific IgG in serum with titers of not less than 160 against the whole cells and titers of not less than 1600 against the OMVs, when compared with two sham-immunized mice. Immune responses of mouse sera to the OMVs of P. gingivalis were also confirmed by WB after ordinary SDS-PAGE. As expected, strong immunoreactivity was found in sera from all six OMV-immunized mice, but not in sera from two sham-immunized mice (data not shown).
Endpoint ELISA titers of six OMV-immunized mouse antisera used in this study
Mouse ID . | OMV-1 . | OMV-2 . | OMV-3 . | OMV-4 . | OMV-5 . | OMV-6 . |
---|---|---|---|---|---|---|
Coating antigen: whole cells of P. gingivalis#1 | >160 | >160 | >640 | >640 | >160 | >160 |
Coating antigen: OMVs of P. gingivalis#1 | >6400 | >6400 | >6400 | >6400 | >1600 | >1600 |
Mouse ID . | OMV-1 . | OMV-2 . | OMV-3 . | OMV-4 . | OMV-5 . | OMV-6 . |
---|---|---|---|---|---|---|
Coating antigen: whole cells of P. gingivalis#1 | >160 | >160 | >640 | >640 | >160 | >160 |
Coating antigen: OMVs of P. gingivalis#1 | >6400 | >6400 | >6400 | >6400 | >1600 | >1600 |
#1: Four-time serially diluted samples were used for ELISAs. The endpoint titers were detemined as the maximum dilution of sample which showed more than 0.05 higher A405 than the average A405 of sera of two mock-immunized mice.
Endpoint ELISA titers of six OMV-immunized mouse antisera used in this study
Mouse ID . | OMV-1 . | OMV-2 . | OMV-3 . | OMV-4 . | OMV-5 . | OMV-6 . |
---|---|---|---|---|---|---|
Coating antigen: whole cells of P. gingivalis#1 | >160 | >160 | >640 | >640 | >160 | >160 |
Coating antigen: OMVs of P. gingivalis#1 | >6400 | >6400 | >6400 | >6400 | >1600 | >1600 |
Mouse ID . | OMV-1 . | OMV-2 . | OMV-3 . | OMV-4 . | OMV-5 . | OMV-6 . |
---|---|---|---|---|---|---|
Coating antigen: whole cells of P. gingivalis#1 | >160 | >160 | >640 | >640 | >160 | >160 |
Coating antigen: OMVs of P. gingivalis#1 | >6400 | >6400 | >6400 | >6400 | >1600 | >1600 |
#1: Four-time serially diluted samples were used for ELISAs. The endpoint titers were detemined as the maximum dilution of sample which showed more than 0.05 higher A405 than the average A405 of sera of two mock-immunized mice.
After performing 2D-PAGE analysis of OMVs using the broad range (pH 3–10) agarose gel, WB analysis was done to examine the immunoreactivity of OMV-immunized serum samples from the six mice (Fig. 5). Strong signals were always detected in the low pH range. All six serum samples consistently reacted with the diffuse signals from no. 32, while four of six reacted with signals from no. 3. WB signals were also detected from nos. 17, 18 and 21, though the signal strength was weaker and detection rates less frequent as compared to those from nos. 3 and 32. Since these WB signals were mainly detected in a low pH range, we performed WB using the same antibody after 2D-PAGE using the narrow range gel (pH 3–8, Fig. 6). In addition to nos. 3 and 32, we could resolve two strong/diffuse signals diffusely distributed in different molecular weights (nos. 31–36). Other diffuse signal was also detected (nos. 39–48). Spot 53 was also immunoreactive with two of the OMV-immunized mouse serum samples (OMV-3 and -6). Notably, MS analysis identified these immunoreactive spots as a range of CTD proteins including CPG70, RgpA, RgpB, Kgp and HagA (Table 1, S1), and their immunoreaction profiles were similar to the distribution of the WB signals when the A-LPS antibody was used.
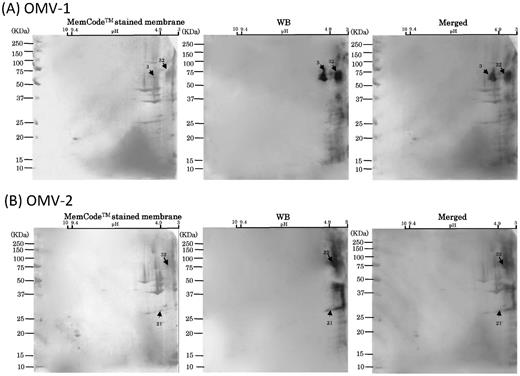
WB analysis of six mouse serum samples with 2D-PAGE of OMVs using broad pH range (3–10) gels for 1D. Scanned images of MemCodeTM-stained membranes and WB are shown in the left and right columns, respectively. Merged images are shown in the right columns. Different mouse serum samples, (A) OMV-1, (B) OMV-2, (C) OMV-3, (D) OMV-4, (E) OMV-5 and (F) OMV-6, were subjected to WB. OMV samples prepared from the same batch cultures were used to obtain OMV-1, -2 and -3 by immunization. Other OMV samples prepared from the same batch cultures were used to obtain OMV-4, 5 and -6 by immunization. Immunoreactive signals are denoted by arrows with spot numbers. Serum samples of OMV-1, -2, -3 and -4 were used at a dilution of 1:1000. Serum samples of OMV-5 and -6 were used at a dilution of 1:500.
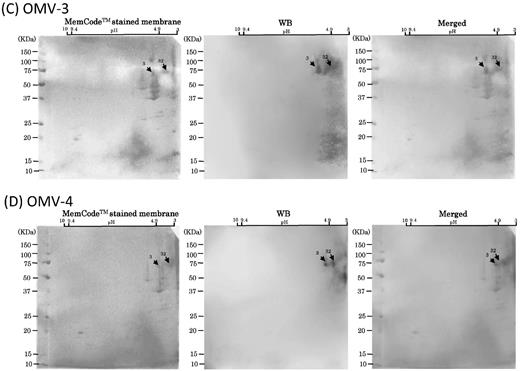

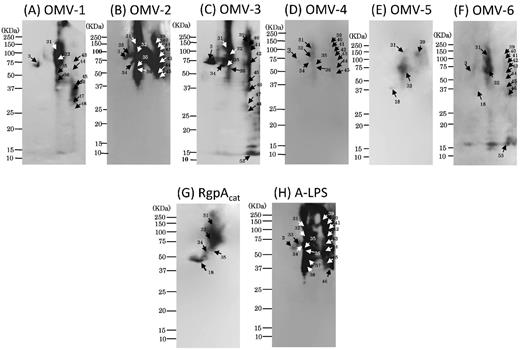
WB analysis of six mouse serum samples with 2D-PAGE of OMVs using narrow pH range (3–8) gels for 1D. WB signals from six different immunized serum samples in low pH areas are shown in the upper panels. Different mouse serum samples, (A) OMV-1, (B) OMV-2, (C) OMV-3, (D) OMV-4, (E) OMV-5 and (F) OMV-6, were subjected to WB. OMV samples prepared from the same batch cultures were used to obtain OMV-1, -2 and -3 by immunization. Other OMV samples prepared from the same batch cultures were used to obtain OMV-4, 5 and -6 by immunization. Immunoreactive signals are denoted by arrows with spot numbers. For comparison, WB results using antibodies against (G) A-LPS and (H) RgpAcat in the same low pH area are shown in the lower panels. Serum samples of OMV-1, -2, -3, -4, -5 and -6 were used at dilutions of 1:1000, 1:500, 1:400, 1:10,000, 1:1000, 1:1000, respectively.
Regarding the signals observed at no. 3, MS analysis identified the no. 3 spot as Mfa1 with the highest mascot score of 1291 (Table 1). However, Kgp protein-derived tryptic peptides were also detected with a high score of 1019 (Table S1). To determine whether Mfa1 is an immunoreactive protein, we performed WB using OMVs isolated from the isogenic mfa1 mutant as an antigen (Fig. 7). The protein profile of the mfa1 mutant OMVs on 2D-PAGE gels was different from that of the wild-type OMVs, e.g. FimA was overexpressed in the mfa1 mutant. A combination of LC-MS/MS and WB using OMV-immunized serum from OMV-1 identified three major immunoreactive proteins of the mfa1 mutant OMVs, M1, M2 and M3, as mixture of Kgp and RgpA, mixture of immunoreactive 84 kDa antigen (PGN_0509) and RgpB, and TPR domain protein (PGN_0876), respectively (Fig. S4, Table S4). However, the serum did not react with any signal at the expected position of Mfa1 when mfa1 mutant-derived OMVs were used as an antigen (Fig. 7). These results indicated that Mfa1 was one of the immunodominant antigens.
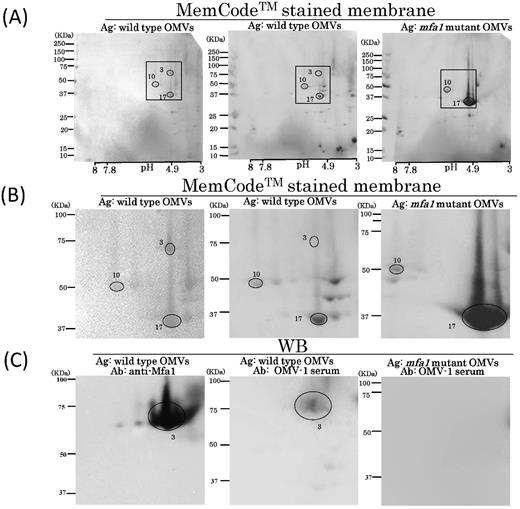
WB analysis using OMV-immunized serum samples with 2D-PAGE of OMVs purified from the wild type and mfa1 mutant. (A) Scanned images of MemCodeTM-stained membranes of wild-type OMVs (left and center) and mfa1 mutant OMVs (right) are shown. (B) Insets in top columns were expanded and are shown in the columns. (C) Scanned images of WB findings using OMV-1 serum (center and right) and Mfa1 antibody (left) (control). Immunoreactive signals are circled with spot numbers. OMV-1 serum and Mfa1 antibody were used at a dilution of 1:1000.
The immunoreaction profiles on WB membranes using six different OMV-immunized serum samples shared several common signals and more or less resembled data obtained by WB using the A-LPS antibody (Fig. 6). These findings led us to examine the contribution of LPS as an immunodominant antigen. We used a serum absorption assay with LPS purified from P. gingivalis with four randomly selected OMV-immunized and four mock-immunized serum samples. In all four of the OMV-immunized samples, the serum absorption assay was successful because reactivity to P. gingivalis LPS was drastically decreased after absorption with P. gingivalis LPS, whereas the four samples from mock-immunized mice did not show altered reactivity to the whole cells regardless of treatment with LPS (Fig. 8). After performing absorption with LPS three times, the reduction rate of the reactivity was estimated to be 87.5 ± 4.0% (mean ± SD) in the OMV-immunized mice and 10.4 ± 19.1% in the mock-immunized mice. We also compared the immunoreaction of OMV-1 mouse serum before and after absorption with LPS using WB analysis with OMVs resolved by 2D-PAGE (Fig. 9). Two blotted membranes were prepared for OMV-1 mouse serum before and after absorption. Three OMV proteins, nos. 27, 30 and 50, were used as loading controls (denoted with circles in Fig. 9). On the 2D maps of the two MemCodeTM-stained membranes, each control protein showed an equivalent amount of protein at the same position. The absorption of serum with LPS resulted in complete loss of LPS ladder bands, spot nos. 35 and 36, and undetermined signals at a high molecular weight (shown by an asterisk with arrow in Fig. 9). We also observed reduced signals at spot nos. 3, 31, 32, 45, 46, 47 and 48, though they remained after absorption.
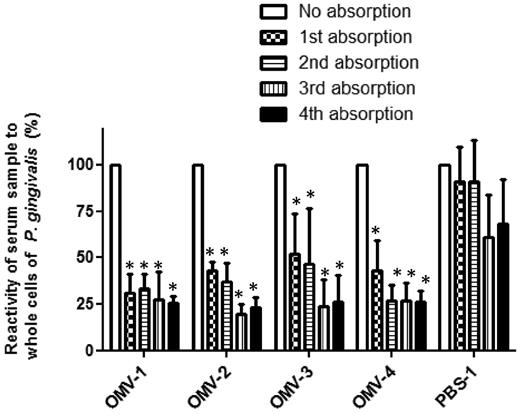
Serum absorption with purified P. gingivalis LPS. Four OMV-immunized serum samples (OMV-1, -2, -3, -4) and 1 mock-immunized serum sample were subjected to absorption assays. Before and after absorption for one to four times with LPS, 100 μl samples after dilution at 1:100 were pipetted into the wells of ELISA plates pre-coated with PMB and P. gingivalis LPS. P. gingivalis LPS-specific IgG was examined. The results are expressed as percent of absorbance at 405 nm after 15 min of incubation with alkaline phosphatase substrate as compared to the same samples without absorption. Absorbance ratios are shown as the mean ± SD from three independent experiments. Statistical analysis was performed using ANOVA followed by the Dunnett's multiple comparison test. Asterisks indicate that the ratio significantly was decreased.
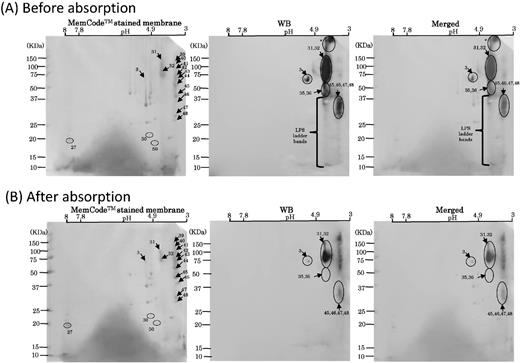
WB analysis using LPS-absorbed and unabsorbed OMV-1 serum. (A) Scanned images of MemCodeTM-stained membranes and WB using OMV-1 serum before absorption with LPS are shown in the left and center columns, respectively. Merged images are shown in the right panel. (B) Scanned images of MemCodeTM-stained membranes and WB using OMV-1 serum after the third absorption with LPS are shown in the left and center columns, respectively. Merged images are shown in the right panel. Three proteins denoted with circles (nos. 27, 30 and 50) were used as loading controls. Immunoreactive signals are denoted by arrows with spot numbers.
DISCUSSION
In the present study, P. gingivalis OMV proteins were resolved on 2D-PAGE gels and transferred to PVDF membranes. Prior to WB, membrane staining was performed to produce a 2D reference map using membranes stained with the MemCodeTM, while the gels remained unstained. This method provided exact identification of the immunoreactive proteins on a 2D map without needing to consider gel contortion, as we could easily superimpose developed X-ray film onto a MemCodeTM-stained membrane. We performed 2D-PAGE analyses using gels with two different pH ranges for 1D, resulting in comprehensive identification of the spots on the reference maps. In addition, LC-MS/MS identified several different proteins from most of the spots by virtue of excellent detection sensitivity. We recommend that this strategy of combined LC-MS/MS analysis with membrane staining followed by WB is a powerful tool for immunoproteome research.
P. gingivalis is an asaccharolytic bacterium and dependent on nitrogenous substrates such as proteins or peptides as its nutrients. In order to degrade proteins from the host or other microorganisms, P. gingivalis can produce a wide range of proteolytic and related enzymes. Surprisingly, we detected a variety of enzymes in the OMVs, including gingipains RgpA (PGN_1970), RgpB (PGN_0294), and Kgp (PGN_1728), zinc carboxypeptidase CPG70 (PGN_0303), trypsin-like proteinase PrtT (PGN_0561), endopeptidase PepO (PGN_0271), alkaline protease AprF (PGN_2041), zinc protease (PGN_0303), lysyl endopeptidase precursors (PGN_1416), thiol protease (PGN_0900) and peptidylarginine deiminase PAD (PGN_0898). In addition, we also frequently identified several proteins involved in hemin or iron acquisition, such as HBP35 (PGN_0659), HagA (PGN_1733) and heme-binding protein FetB (PGN_0705), as well as different variants of gingipains, which contain several hemagglutinin domains. Very recently, a similar report was presented regarding OMVs of Bacteroides, which is close to P. gingivalis, in which acidic glycosidases and proteases were preferentially packed into the OMVs (Elhenawy, Debelyy and Feldman 2014). Our results suggest that OMVs are equipped with many important molecules for acquisition of essential nutrients for P. gingivalis and have a critical role for its survival in periodontal pockets.
It is generally recognized that bacterial OMVs are feasible immunogens for current vaccine strategies, as they can provide ideal antigenic determinants for the host immune system. On the other hand, it is important to consider the presence of virulence factors such as LPS in OMVs for clinical usage. Direct application of P. gingivalis OMVs into periodontal pockets may be harmful to humans. However, in our previous study, both systemic and mucosal immunity in mice were efficiently elicited by application of very small amounts of OMVs to nasal cavities (Nakao et al., 2011). In our opinion, the risk of adverse reactions due to P. gingivalis LPS as a constituent of OMVs must be estimated lower than that in case of E. coli LPS, because P. gingivalis LPS have shown much lower levels of endotoxin activity (Takada and Galanos 1987; Ogawa and Uchida 1996; Tanamoto et al., 1997).
It is now recognized that the CTD proteins contain a variety of virulence factors and are translocated through the OM by a Bacteroidetes-specific secretion system called Por secretion system (PorSS) or type IX secretion system (Sato et al., 2013), or combined attachment and secretion system (Veith et al., 2013). CTD family proteins were reported to be glycosylated with a unique LPS with anionic polysaccharides, termed A-LPS (Curtis et al., 1999). We previously found an unusual surface property of P. gingivalis OMVs, as they bound to epoxy group on magnetic beads in a species-specific manner (Nakao et al., 2014). Another report showed that application of nanoparticles generated from anionic liposomes as vehicles of antigens in a nasal immunization mouse model efficiently elicited mucosal immunity as well as systemic immunity as compared with nanoparticles generated from other liposomes with a different surface chemistry (Chen et al., 2013). Therefore, we speculated that the surface chemical property of P. gingivalis OMVs conferred by A-LPS may be essential for elicitation of antibody production in the context of interaction with host cells.
Recently, the proteome of OMVs of strain W83 was determined by LC-MS/MS analyses of the tryptic peptide library prepared from an ordinary SDS-PAGE gel, resulting in identification of a total of 151 proteins (Veith et al., 2014). In the present study, using OMVs of strain ATCC 33277, 53 spots were resolved by 2D-PAGE with both broad and narrow pH range 1D gels, and subjected to LC-MS/MS analyses and PMF, resulting in identification of 61 proteins. Of 20 CTD proteins identified in this study, a conserved hypothetical protein PGN_0458, serine protease PGN_0561 and C-terminal domain of Arg- and Lys-gingipain proteinase (PGN_0295) were found to be present in ATCC 33277 but not in W83. All three proteins likely conserve the integral CTD. It is therefore expected that the gene products will be translocated to the OM or extracellular milieu, though their biological functions remain unclear.
Both FimA and Mfa1 fimbriae have been found to be involved in the immunopathology of P. gingivalis infection as well as adhesion to organic and inorganic surfaces in the oral cavity. While W83 expresses neither the major fimbrillin FimA nor minor fimbrillin Mfa1, ATCC 33277 expresses both. We previously reported that FimA fimbriae are major constituents of OMVs of ATCC 33277 (Nakao et al., 2014). In the present study, both MS and WB assays identified the location of FimA on the 2D reference map of OMVs (Fig. S3[C]). Furthermore, not only FimA but also Mfa1 was identified in the OMV preparations by MS analysis and 2D-PAGE (Fig. S3[D]), and we also noted that Mfa1 rather than FimA was frequently and strongly immunorecognized by OMV-immunized sera (Figs 5 and 6). The OMV-1 serum immunoreacted with wild-type OMVs, but not with the isogenic mfa1 mutant OMVs (Fig. 7). These results suggest that Mfa1 is an immunodominant antigen in the present mouse model, and may function as an adhesion factor as well as an antigen when Mfa1 proteins interact with the host.
As shown in Fig. 9, a serum absorption assay revealed that LPS highly contributed to induction of immune responses in the present mouse model. A previous in vivo study reported that subcutaneous immunization of P. gingivalis OMVs successfully protected nearly half of mice challenged by a lethal dose of P. gingivalis LPS, while immunization of whole cells provided no such protection (Kesavalu et al., 1992), thus indicating the advantage and applicability of OMVs as an immunogen. LPS is localized at the outer leaf of the OM and OMV. Notably, the location of the polysaccharide portion of LPS places it at the interface between the bacterium and its environment. Hence, we consider that LPS is a potential target molecule in an antibody-directed vaccine system. Although it is generally known that production of LPS antibody is not easy in current available vaccine system, the potential of OMVs as a huge complex antigen containing LPS for induction of LPS antibody is attractive and may be applicable for vaccine system against not only P. gingivalis but also other Gram-negative pathogens that possess LPS as their virulence factors, such as pathogenic E. coli and Salmonella.
Our study characterized the immunoproteome of OMV-immunized mouse serum using a 2D reference map. We demonstrated that intranasal immunization with OMVs systemically elicited immune responses that produced antibodies against LPS as well as a range of proteins including Mfa1 and A-LPS-modified proteins. We propose the possible applicability of the system for development of a periodontal vaccine, though challenge experiments and clinical studies must be done prior to practical usage of such a vaccine system.
We are grateful to Dr Minoru Yoshida for the generous support in our study. We thank Ms Michiyo Kataoka and Ms Yoshiko Obara for their technical assistance with electron microscopy analysis and in silico analysis, respectively. We also thank Dr Yoshio Nakano, Dr Nozomu Obana and Dr Shunsuke Minakuchi for the fruitful discussion. We also thank Dr Nobushiro Hamada and Dr Michael A. Curtis for kindly providing important materials. We also thank the Support Unit for Bio-Material Analysis, RIKEN BSI Research Resources Center, for technical help with the LC-MS/MS analysis. This study was supported in part by JSPS KAKENHI (Grant nos. 22791822, 24659821, 12840634 and 26462866), a grant from the Ministry of Health, Labor and Welfare (H24-Iryo-Shitei-014) and The Naito Foundation.
SUPPLEMENTARY DATA
REFERENCES