-
PDF
- Split View
-
Views
-
Cite
Cite
Marina Luongo, Amalia Porta, Bruno Maresca, Homology, disruption and phenotypic analysis of CaGS Candida albicans gene induced during macrophage infection, FEMS Immunology & Medical Microbiology, Volume 45, Issue 3, September 2005, Pages 471–478, https://doi.org/10.1016/j.femsim.2005.06.007
- Share Icon Share
Abstract
During macrophage infection Candida albicans expresses differentially several genes whose functions are associated with its survival strategy. Among others, we have isolated CaGS gene, which is homologous to SNF3, a glucose sensor of Saccharomyces cerevisiae. To elucidate its potential role during infection, CaGS has been disrupted and the resulting phenotype analyzed on different solid media. The null mutant lost the ability to form hyphae on a medium with low glucose concentration and serum. Furthermore, this mutant does not disrupt macrophage in in vitro infections. We believe that this putative glucose sensor is involved in hyphal development during macrophage infection.
1 Introduction
Candida albicans is a dimorphic fungus that is part of the normal gastrointestinal microbiota of humans. C. albicans is also an important opportunistic pathogen which causes a wide range of infection in the compromised host [1].
In systemic candidosis, macrophages are probably the main effectors involved in the resistance to the fungus [2,3]. Fungal cells withstand the extreme conditions existing inside the macrophage, which is ultimately disrupted by the formation of hyphae. The morphogenetic yeast-to-hyphae transition of C. albicans has been extensively studied: this phenomenon is dependent, among other factors, upon the specific activation of several signal transduction pathways in response to different environmental stimuli [4–6]. So far, only a limited number of signals triggering the morphogenetic changes have been identified, especially those occurring during macrophage infection [7]. C. albicans differentially expresses a number of specific genes during phagocitosis whose products allow Candida to survive or prevent phago-lysosome formation [8].
Here, we report the isolation of a CaGS gene (Candida glucose sensor, previously called CG13 [8]) that is differentially expressed during macrophages infection and homolog to SNF3 of Saccharomyces cerevisiae[9].
In most organisms, glucose is the major source of energy and it has a profound influence on many metabolic processes [10]. Glucose transporters are specialized proteins found in all living organisms to uptake d-glucose. Yeast and mammalian glucose transporters share a common topology, with a central 12-trans-membrane domain and the cytoplasmic N and C termini. Glucose transporters are also of considerable interest as potential targets for the development of antimicrobial and antiparasitic drugs.
A C. albicans glucose transporter, CaHGT1 has been previously described in complementation studies of S. cerevisiae[11] and it was suggested that the C. albicans glucose transporter might be linked to the phenomenon of drug resistance [11].
In this study, we report the first characterization of the upregulation of C. albicans CaGS gene during infection. To understand the role of CaGS gene, mutants were generated by gene disruption and their phenotypes analyzed on different media with high or low glucose concentration and during macrophage infection. All together, our data suggest that CaGS is implicated in the morphogenesis of C. albicans.
2 Materials and methods
2.1 Strains and growth condition
C. albicans strains utilized in this study are listed in Table 1. The strains were routinely cultured in liquid either in YPD (1% yeast extract, 2% bacto peptone and 2% glucose) or in YNB (2% glucose, 0.67% Difco yeast nitrogen base) at 30 °C, with vigorous agitation with a rotary shaker or on solidified media with 2% agar. Filamentation was assessed using various plates: YNB, with 10% fetal calf serum (FCS, Euroclone) using either 0.1%, 2% or 4% glucose concentration; 10% fetal calf serum; Medium 199 containing Earle's salts and glutamine lacking sodium bicarbonate (Gibco-BRL), and containing 150 mM HEPES adjusted to pH 7.5. Plates were spotted with 106 cells in 5 µl of water and incubated at 37 °C for 6 days. In all assays, stationary phase cells grown at 30 °C in YPD were used as inoculum. Media were supplemented with uridine (25 µg ml−1) when necessary. Ura− auxotrophs were selected on medium containing 5′-fluoro-orotic acid (5′-FOA) [12]. The macrophage cell line J774.1 (ATCC TIB-67) was cultured in high-glucose Dulbecco's modified Eagle's medium (DMEM: 4.5 g l−1 glucose; Bio-Wihittaker Europe) supplemented with 10% heat-inactivated fetal calf serum (FCS, Euroclone), 3.024 g l−1 NaHCO3, 2 mM l-glutamine, 100 U ml−1 penicillin and 100 U ml−1 streptomycin as required. To prevent filamentation, all C. albicans strains used for macrophage infection, were preliminarily cultured in YPD, 4% glucose at 37 °C.
Strain | Parental strain | Genotype | References |
SCS314 | Wild type | Gillum et al. [34] | |
CAI4 | Δura3::imm434/Δura3::imm343 | Fonzi and Irwin [12] | |
CAI12 | CAI4 | Δura3::imm434/URA3 | Porta et al. [21] |
CAL1 | CAI4 | Δcags::hisG-URA3-hisG/CaGSΔura3::imm434/Δura3::imm343 | This study |
CAL2 | CAL1 | Δcags::hisG/CaGSΔura3::imm434/Δura3::imm343 | This study |
CAL3 | CAL2 | Δcags::hisG/Δcags::hisG–URA3-hisGΔura3::imm434/Δura3::imm343 | This study |
CAL4 | CAL3 | Δcags::hisG/Δcags::hisGΔura3::imm434/Δura3::imm343 | This study |
CALRE | CAL4 | Δcags::hisG/ΔCaGS::URA3Δura3::imm434/Δura3::imm343 | This study |
CAL2U | CAL2 | Δcags::hisG/CaGSΔura3::imm434Δura3::imm343 | This study |
Δura3::imm434/URA3 | |||
CAL4U | CAL4 | Δcags::hisG/Δcags::hisGΔura3::imm434/Δura3::imm343 | This study |
Δura3::imm434/URA3 |
Strain | Parental strain | Genotype | References |
SCS314 | Wild type | Gillum et al. [34] | |
CAI4 | Δura3::imm434/Δura3::imm343 | Fonzi and Irwin [12] | |
CAI12 | CAI4 | Δura3::imm434/URA3 | Porta et al. [21] |
CAL1 | CAI4 | Δcags::hisG-URA3-hisG/CaGSΔura3::imm434/Δura3::imm343 | This study |
CAL2 | CAL1 | Δcags::hisG/CaGSΔura3::imm434/Δura3::imm343 | This study |
CAL3 | CAL2 | Δcags::hisG/Δcags::hisG–URA3-hisGΔura3::imm434/Δura3::imm343 | This study |
CAL4 | CAL3 | Δcags::hisG/Δcags::hisGΔura3::imm434/Δura3::imm343 | This study |
CALRE | CAL4 | Δcags::hisG/ΔCaGS::URA3Δura3::imm434/Δura3::imm343 | This study |
CAL2U | CAL2 | Δcags::hisG/CaGSΔura3::imm434Δura3::imm343 | This study |
Δura3::imm434/URA3 | |||
CAL4U | CAL4 | Δcags::hisG/Δcags::hisGΔura3::imm434/Δura3::imm343 | This study |
Δura3::imm434/URA3 |
Strain | Parental strain | Genotype | References |
SCS314 | Wild type | Gillum et al. [34] | |
CAI4 | Δura3::imm434/Δura3::imm343 | Fonzi and Irwin [12] | |
CAI12 | CAI4 | Δura3::imm434/URA3 | Porta et al. [21] |
CAL1 | CAI4 | Δcags::hisG-URA3-hisG/CaGSΔura3::imm434/Δura3::imm343 | This study |
CAL2 | CAL1 | Δcags::hisG/CaGSΔura3::imm434/Δura3::imm343 | This study |
CAL3 | CAL2 | Δcags::hisG/Δcags::hisG–URA3-hisGΔura3::imm434/Δura3::imm343 | This study |
CAL4 | CAL3 | Δcags::hisG/Δcags::hisGΔura3::imm434/Δura3::imm343 | This study |
CALRE | CAL4 | Δcags::hisG/ΔCaGS::URA3Δura3::imm434/Δura3::imm343 | This study |
CAL2U | CAL2 | Δcags::hisG/CaGSΔura3::imm434Δura3::imm343 | This study |
Δura3::imm434/URA3 | |||
CAL4U | CAL4 | Δcags::hisG/Δcags::hisGΔura3::imm434/Δura3::imm343 | This study |
Δura3::imm434/URA3 |
Strain | Parental strain | Genotype | References |
SCS314 | Wild type | Gillum et al. [34] | |
CAI4 | Δura3::imm434/Δura3::imm343 | Fonzi and Irwin [12] | |
CAI12 | CAI4 | Δura3::imm434/URA3 | Porta et al. [21] |
CAL1 | CAI4 | Δcags::hisG-URA3-hisG/CaGSΔura3::imm434/Δura3::imm343 | This study |
CAL2 | CAL1 | Δcags::hisG/CaGSΔura3::imm434/Δura3::imm343 | This study |
CAL3 | CAL2 | Δcags::hisG/Δcags::hisG–URA3-hisGΔura3::imm434/Δura3::imm343 | This study |
CAL4 | CAL3 | Δcags::hisG/Δcags::hisGΔura3::imm434/Δura3::imm343 | This study |
CALRE | CAL4 | Δcags::hisG/ΔCaGS::URA3Δura3::imm434/Δura3::imm343 | This study |
CAL2U | CAL2 | Δcags::hisG/CaGSΔura3::imm434Δura3::imm343 | This study |
Δura3::imm434/URA3 | |||
CAL4U | CAL4 | Δcags::hisG/Δcags::hisGΔura3::imm434/Δura3::imm343 | This study |
Δura3::imm434/URA3 |
2.2 Macrophage infection
Cultivation and infection of macrophages was conducted as described by Prigneau et al. [13]. Co-culture (Candida and macrophages) with non-confluent macrophages were performed onto Lab-Tek chamber Slides (Nalge Nunc International) to monitor phenotypic modification of the different C. albicans strains (CaGS mutants and CAI-12 as a control) during macrophage infection. Infected cultures were then stained using the Hochtkiss–Macmanus method [13,14].
2.3 DNA cloning and sequence analysis
On the basis of the Stanford sequence database (http://www.sequence.stanford.edu/group/candida/search.html) CaGS cDNA fragment has 99% of identity with the contig6-2512 [8]. CaGS was amplified using as template genomic DNA of SC5314 strain of C. albicans and a Fynnzymes DyNAzyme EXT DNA polymerase for a high PCR performance. The primers were RM1 (5′-CTTTGTTCATCTCTTGCTCTGG-3′) and FM2 (5′-CTTAAGGACGATAAACAAAGAGCAG-3′).
The underlined AflII restriction site was added at the 5′ end of the forward primer. The PCR product was cloned into TA cloning vector pCR2.1 to generate a plasmid pCaGS. Homology searches of the predicted protein in the GenBank database, was performed by BLASTP [15]. Multiple sequence alignments were conducted using Clustal W [16]. Sequence was deposited in the GenBank database under Accession No. AF441395.
2.4 Strain constructions
To determine the role of CaGS in C. albicans, a cags null mutant was constructed by replacing a hisG-URA3-hisG cassette for CaGS coding region [12]. The primers RM2 (5′-GAGCTCAGATGATGGCGATATTGA-3′) and FM2 were used to amplify the 3′ end of CaGS. SacI restriction site added at the 5′ end of the reverse primer is underlined. The PCR product, a fragment of 577 bp, was cloned into TA cloning vector pCR2.1, to generate the plasmid pT2. A fragment SacI–AflII from pT2 was subcloned into like sites of plasmid LITMUS 28 (Biolabs), generating a plasmid named pML2. The primers RM1 and FM1 (5′-AAGCTTCACCAAAAGCAGCAAACATACC-3′) (HindIII restriction site is underlined) were used to amplify a fragment of 827 bp from the 5′ end of CaGS and cloned into TA cloning vector pCR2.1, generating a pT1 plasmid. An EcoRI–HindIII fragment from pT1 was cloned in pML2. A HindIII–SacI fragment containing the hisG-URA3-hisG cassette, from pMB7 [12], was cloned in plasmid pML1-2 generating pL1U2. This plasmid was digested with XhoI and AflII to obtain the transforming DNA. Approximately 8 µg of this linear DNA was used to transform C. albicans strain CAI4 (ura−/−) [17] with Yeast Transformation System kit (Clonthec). The sequentially disruption of both CaGS alleles, performed as described by Fonzi and Irwin [12], generated the following strains: heterozygote ura+ CAL1; heterozygote ura−CAL2; null mutant ura+ CAL3; null mutant ura− CAL4.
Reconstitution of the cags null strain was achieved by integration of plasmid pLGS. This plasmid was constructed by cloning a HindIII–SacI fragment derived from pSMS44 [18], containing URA3, into pML2. In the resulting plasmid, pLU-2 was cloned a fragment AflIII–PstI from pCaGS. After selection of the revertant strains, one of the clones was designated CALRE. Proper integration events were verified in each strain by Southern blot analysis, digesting the genomic DNA with PstI. URA3 gene was re-introduced in its chromosomal locus by transformation of CAL2 and CAL4 strains with a 5kb PstI–BglII fragment containing the full URA3 coding sequence [12]. The generated strains CAL2U and CAL4U did not reveal any phenotypic difference when compared, respectively, to CAL1 and CAL3 on agar plates or onto Lab-Tek Chamber Slides during macrophage infection.
2.5 DNA extraction and analysis
Standard techniques have been used for DNA manipulations. Genomic DNA for Southern blot analysis was prepared as previously described [19]. DNA samples, digested with PstI, were separated by electrophoresis on 0.8% agarose gels, denatured, transferred onto Hybond-N nylon membrane (Amersham-Pharmacia Biotech) and hybridized. The fragment obtained with the primers FM1/RM1 and the fragment obtained with the primers FM2/RM2 were used as probe, labeled with [α-P]dCTP by High Prime kit (Boehringer-Mannheim) and hybridized at 55 °C overnight. Washing condition was 58 °C for 15 min, repeated three times.
2.6 RNA purification and Northern blot analysis
Total RNA was prepared by growing cultures to stationary phase at 30 °C in YPD. Cells from these cultures were used to inoculate 300 ml of pre-warmed (30 or 37 °C) YNB with 0.1%, 2% or 4% of glucose, at a density of 6 × 106 cell ml−1. Cultures were incubate at 30 or 37 °C with vigorous agitation for 2–3 h. RNA was extracted using selective precipitation with LiCl as described by Ramon et al. [20]. Total RNA was quantified on agarose gel and by spectrophotometric reading at λ260. Samples containing 20 µg of RNA were separated by electrophoresis in 1.2% agarose gel containing formaldehyde as previously described [21]. RNA was transferred onto nylon membranes (Hybond N+; Amersham) and fixed by UV irradiation. Blots were hybridized with a HpaI–EarI fragment (707 bp) from pCaGS containing the coding region of CaGS, and labelled with [α-32P]dCTP. A cDNA probe corresponding to 18S ribosomal RNA was used for mRNA quantification using a 445SI PhosphorImager (Molecular Dynamics) and Gel Analyzer.
2.7 RT-PCR
Total RNA was extracted as previously described. For RT-PCR, each RNA samples were treated with DNA-free™ (Ambion Inc.) DNase and reverse-transcribed with Multi Scribe™ reverse transcriptase (PE Biosystems) at 42 °C for 12 min. Primers used to amplify by PCR 800 bp of cDNA were RGS (5′-ATTGGTGCTGGAATACGATG-′3) and FGS (5′-CATCAACTTCAACCATTTCGG-′3). Cycle profiles were initial denaturation at 94 °C for 5 min; 40 cycle of amplification at 94 °C for 45 s, 51 °C for 45 s, and 72 °C for 1 min and final extension at 72 °C for 10 min. PCR includes a positive control using genomic DNA as the template and a negative control without reverse transcriptase.
3 Results
3.1 Homology study and structure analysis of CaGS gene
The full-length ORF of CaGS gene codes for a putative protein of 526 amino acid with a predicted molecular weight of 52 kDa (PSORTII). Comparison with protein database revealed that CaGSp has 47% identity and 67% similarity to the high affinity glucose sensor Snf3p of S. cerevisiae[9].
CaGSp shows extended similarity to other proteins: 67% similarity and 46% identity with Rgt2p, low affinity glucose sensor of S. cerevisiae; 65% similarity and 47% identity with Rag4p, a glucose sensor of Kluyeromyces lactis[22]; 61% similarity and 44% identity with Rco3p, a glucose sensor of Neurospora crassa[23].
The CaGSp has 12 predicted trans-membrane-spanning domains, but, as Rco3p, does not have a long –COOH terminal tail, with a typical sequence of 25 amino acids [9] present in Rgt2p, Snf3p and Rag4p. Structural study of the protein sequence (http://ca.expasy.org/prosite) showed the presence of signatures characteristics of glucose transporters. The first signature is based on the G-R-[KR] motif (positions 97–100; 184–187; 341–344). The second typical signature is GIILVETWGRRPLLMAGS (position 334–351).
3.2 CaGS expression pattern during macrophage infection
A time course of CaGS gene expression during macrophage infection was performed and RNA was analyzed by Northern hybridization. Quantification of mRNA by Gel Analyzer revealed an increase of ca. 4-fold of CaGS gene expression 30 min after infection. We could not detect any transcript, using RNA purified from C. albicans grown in the absence of macrophages in DMEM as control (Fig. 1). This expression pattern was confirmed with four independent batches of RNA purified during infection, two of which are shown represented. The observation that CaGS gene is down regulated when Candida is grown in DMEM medium in the absence of macrophages, is in agreement with the presence of high glucose concentration in the medium and it strongly supports our conclusion that its overexpression is due to the presence of macrophages.
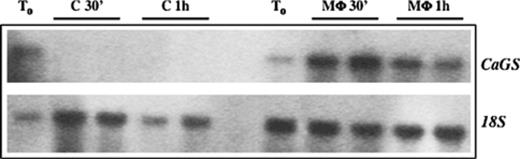
Northern blot analysis of CaGS gene expression during macrophage infection. RNA batches obtained with two independent infections of C. albicans CAI-12. T0 represents the expression before infection; C 30′, represents C. albicans total RNA extracted 30 min after incubation in DMEM (control); M Φ 30′ represents total RNA extracted 30 min after macrophage infection; C 1h represents a control 1 h after incubation in DMEM; M Φ 1h represents total RNA extracted 1 h after macrophage infection. 169 × 53 mm (300 × 300 DPI).
3.3 Expression of CaGS at different glucose concentration
As shown with a database analysis, CaGS gene is homologous to a high affinity glucose receptor (SNF3), which is repressed at high glucose concentration. To analyze the transcriptional regulation of CaGS by RT-PCR, cells were cultured at 30 or 37 °C until logarithmic phase in liquid YNB with different glucose concentrations (0.1%, 2% and 4%). As shown in Fig. 2, CaGS is strongly expressed in low glucose (0.1%).
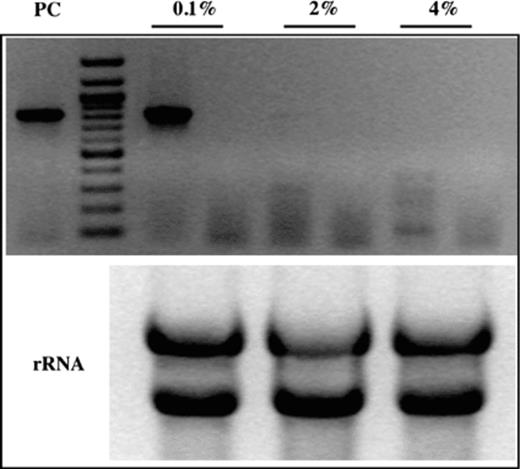
Transcription of CaGS gene in response to different glucose concentration in the growth medium. RT-PCR of total RNA extract from yeast grown in the YNB with different glucose concentration. Lanes: (0.1%), RT-PCR at 0.1% glucose concentration and non-RT-PCR as a negative control; (2%), RT-PCR at 2% glucose concentration and non-RT-PCR as a negative control; (4%), RT-PCR at 4% glucose concentration and non-RT-PCR as a negative control; (PC), PCR using genomic DNA template as a positive control; (rRNA), control of RNA using for RT-PCR. 80 × 70 mm (300 × 300 DPI).
3.4 CaGS gene knock-outs
To determine the function of CaGS a null mutant was constructed in CAI4 strain replacing 1390 bp of the coding region with hisG-URA3-hisG cassette as shown in Fig. 3(a). DNA was digested with PstI and the products analyzed by Southern blot gels and then hybridized with probe 1 (a PCR product of 827 bp obtained using FM1/RM1 primers). Since by PstI digestion CaGS allele of the heterozygous ura+ has the same size of CaGS in the heterozygous ura−, a twin Southern blot was hybridized with probe 2 (a 577-bp fragment obtained by PCR using FM2/RM2 primers).
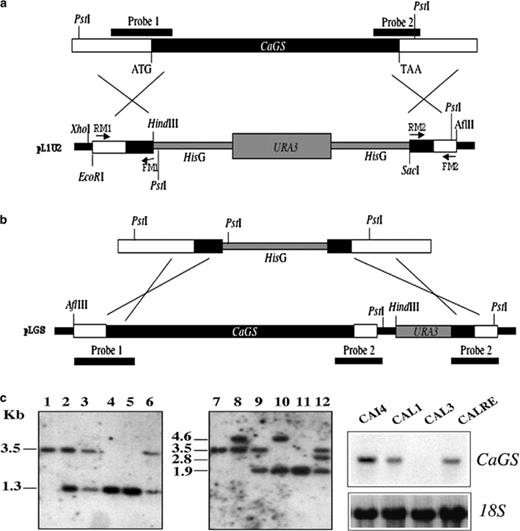
Disruption strategy for CaGS gene. (a) CaGS open reading frame is indicated in black bar. A 1390 bp fragment of the coding region was replaced by hisG-URA3-hisG cassette. Probe 1 and probe 2 (solid lines) were used to confirm the disruption of CaGS. (b) Revertant construct obtained by replacement of the remaining hisG by CaGS gene followed by URA3 selector gene. (c) Southern blot analysis of PstI digested C. albicans genomic DNA. Lanes 1 and 7, parental strain CAI4; 2–3 and 8–9, CaGS/cags strains CAL1 and CAL2, Ura+ and Ura−, respectively; 4 and 10, null mutant strain CAL3; 5 and 11, null mutant Ura−, CAL4; 6 and 12, one representative revertant strain, CALRE. (d) Northern blot analysis of CaGS expression in CAI4, CAL1, CAL3 and CALRE grown in YNB medium with 0.1 used to hybridize C. albicans total RNA (upper panel) and the C. albicans 18S was used as control for quantification (lower panel).
Southern blot analysis of a representative Ura+ transformant (strain CAL1) demonstrated the presence of a 3471-bp PstI fragment characteristic of the wild type CaGS allele, the predicted 1311-bp fragment (hybridization with probe 1) and 4635-bp fragment (hybridization with probe 2) representing the disrupted allele. After 5′-FOA selection, the latter fragment was reduced to 1916 bp (hybridization with probe 2) as shown in the representative heterozygous mutant CAL2. Transformation of CAL2 yielded a homozygous null mutant, CAL3, in which a 4635-bp fragment (hybridization with probe 2; Fig. 3(c)) replaced the 3471-bp fragment. A wild type CaGS allele was introduced into cags null mutant by targeting integration of plasmid pLGS in CAL4 strain (Fig. 3(b)). CALRE showed a fragment of approximately 3467 bp (hybridization with probe 1) and a fragment of 2772 bp (hybridization with probe 2) corresponding to the reconstructed allele (Fig. 3(c)).
Lack of expression of CaGS in the null mutant and detection of a hybridizing band in the revertant strain, determined by Northern blot analysis, confirmed the validity of the Southern blot analyses (Fig. 3(d)).
3.5 Effect of CaGS gene disruption on macrophage infection
The infection of macrophages was conducted as previously described [13]. Ingested CAI-12 blastospores, began to germinate 1 h after infection and disrupted phagocytes approximately 2 h later. While CAI-12 strain yields long filaments and disrupt macrophages, the null mutant (CAL3) produces hyphae of reduced size. However, both the heterozygote CAL1 and revertant CALRE showed intermediate hyphae development compared to the control CAI-12 (Fig. 4). Testing CAL2U (CaGS/cags, URA+/−) and CAL4U (cags/cags, URA+/−) we obtained similar results (data not shown).
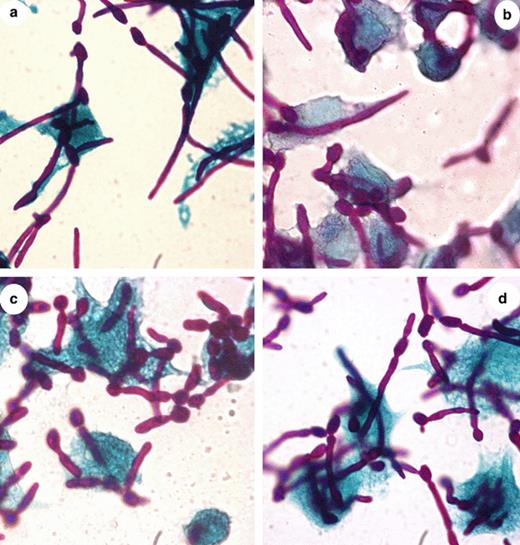
Macrophage infected with CAI12 and CaGS mutants. Yeast cells were resuspended in DMEM and added to the monolayer of macrophages in approximate moi 5/1. After incubation at 37 °C for 1 h, cells were fixed and stained with the Hochtkiss–MacManus coloration. Magnification 100×. Photos a, b, c and d correspond, respectively, to the CAI12, CAL1, CAL3 and CALRE. 123 × 129 mm (300 × 300 DPI).
3.6 Phenotypic analysis of CaGS mutant grown in different glucose concentration
The effect of deletion of CaGS was analyzed spotting yeast cells on plates containing high or low concentration of glucose, with or without 10% of serum after 6 days of incubation at 37 °C. In YNB with 0.1% glucose and 10% serum, the null mutant (CAL4U) lacked the ability to form lateral hyphae at 37 °C and the heterozygous (CAL2U) produced very short hyphae compared to the control CAI-12. The same effect was observed in plates with H2O and 10% serum. On medium 199 the null mutant and the heterozygous showed the formation of hyphae of reduced size. Re-introduction of the wild-type gene into the null mutant reverted (CALRE) all associated phenotypes (Fig. 5). These results were reproduced with a set of two independently constructed mutants. When strains grew as yeast no differences were observed (data not shown).
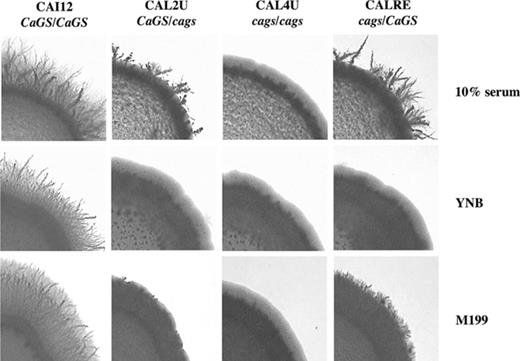
Effect of CaGS disruption on filamentation. Control strain (CAI12), heterozygous mutant (CAL2U), homozygous mutant (CAL4U), and heterozygous revertant (CALRE) strains were spotted on agar plates made with 10% serum, YNB (containing 0.1% glucose and 10% serum) and M199 (pH 7.0) and incubated at 37 °C for 5–6 days. 159 × 107 mm (300 × 300 DPI).
4 Discussion
Eukaryotic cells can use as energy sources alternative to glucose, a sugar that is the preferred carbon source for many organisms. Cells monitor the presence or absence of glucose in the environment by receptor/sensor, which transduce the signal into the cells [10]. In particular, yeasts can change the metabolic activity in accordance with the presence or absence of nutrients [24].
In systemic candidosis, macrophages are probably the main effectors involved in the resistance to the fungus [2,3]. During phagocytosis C. albicans survive in the adverse conditions present inside the macrophage and prevent phagolysosome fusion by formation of hyphae [8]. The Candida yeast-hyphae transition is considered as one of the major virulence factor, a process that requires energy. Within the macrophage environment, C. albicans presumably faces nutrient starvation such as glucose deprivation. Thus, among other factors, the ability of Candida to adapt rapidly to sudden environmental changes is also due to the efficiency of its metabolism. Moreover, previous results have indicated that genes coding for proteins related to glyoxylate cycle and lipid metabolism are overexpressed by C. albicans during macrophages infection [8,13].
We have studied CaGS gene whose expression is induced during macrophage infection [8]. CaGS codes for a putative protein of 526 amino acids that is a homolog of glucose sensor proteins such as Snf3p, Rgt2p of S. cerevisiae, Rag4p of K. lactis, and Rco3 which is not clear if is a sensor or a glucose transporter of N. crassa[23].
In S. cerevisiae, there are 20 genes coding for hexose transport (HXT1 to HXT17, GAL2, SNF3 and RGT2) that carry their substrate by facilitate diffusion. Snf3 and Rgt2, are required for proper transcription of several HXT (hexose transporter) genes in response to low (Snf3p, a high affinity glucose sensor) or high (Rgt2p, a low affinity glucose sensor) external glucose concentration [9,25–29].
Sequence alignment showed that CaGSp, as all sugar transporter/sensor proteins, has several defined regions of high amino acid conservation, such as residues GRR/K, and sugar transport protein signature 1 (Prosite database). Moreover, as a glucose sensor, CaGSp has 12 trans-membrane domains but, like Rco3, lacks the long C-terminal tail of 200 bp, present in Snf3 and Rgt2 genes. The C-terminal segment of Snf3 and Rgt2, which is believed to be a cytoplasmic protein, is unusually long (341 amino acids in Snf3 and 218 in Rgt2). In this respect, they differ from all other members of the hexose transporter gene family, none of which has a predicted cytoplasmic C-terminal tail longer than 60 amino acids [9]. The carboxyl-terminal extension of Snf3p and Rgt2p is important in transmitting glucose-induced signals; they transduce the signal inside the cell inducing the HXT genes [9,30,31].
The identification of a high affinity glucose transporter CaHGT1 in C. albicans[11] suggests that, depending on glucose concentration, there is a specific transcriptional regulation and that it changes from a low affinity system transport to a high affinity system which is linked to the yeast–mycelium transition [32,33].
Our results imply that CaGSp expression is correlated to the extracellular glucose concentration and to hyphae formation. Moreover, when extracellular glucose concentration is low, cags strain null mutant produces shorter hyphae (on M199, pH 7.5) or totally absent (on 10% serum; YNB 0.1% glucose plus 10% serum) compared to the parental strain. Furthermore, a filamentation defect of the null mutant has been observed during macrophages infection, confirming that, in vitro, CaGS is probably involved in the hyphae growth.
Thus, the overexpression of CaGS and the hyphae formation are two related phenomena and they can be considered as the fungus' answer to a glucose nutritional limitation.
Acknowledgment
The work was supported by a contract by the Italian Ministero dell'Universitã (MIUR), Stress Proteins and by a grant (ex 60%) by the University of Salerno.
References