-
PDF
- Split View
-
Views
-
Cite
Cite
Ramachandran Sarojini Santhosh, Shunmugiah Karutha Pandian, Nirmala Lini, Abdul Khader Shabaana, Avuthu Nagavardhini, Kuppamuthu Dharmalingam, Cloning of mce1 locus of Mycobacterium leprae in Mycobacterium smegmatis mc2 155 SMR5 and evaluation of expression of mce1 genes in M. smegmatis and M. leprae, FEMS Immunology & Medical Microbiology, Volume 45, Issue 2, August 2005, Pages 291–302, https://doi.org/10.1016/j.femsim.2005.05.004
- Share Icon Share
Abstract
Plasmid pSET152 is a broad host range mobilizable vector which integrates into streptomyces chromosome utilizing att site and int function of ∅C31. Transformation of this plasmid into Mycobacterium smegmatis mc2 155 SMR5 gave stable transformants carrying the pSET152 as an integrated copy. Integration occurred at the cross over sequence 5′TTG disrupting the gatA gene (Glu-tRNAGln amidotransferase subunitA), which is non-essential under conditions used. Recombinant pSET152 plasmids carrying mce1 locus of Mycobacterium leprae were used to construct M. smegmatis transformants carrying the mce1 locus in their chromosome. RT-PCR analysis revealed specific transcripts of M. leprae mce in M. smegmatis. The transcribed mRNA carried intergenic regions between genes of mce1 locus indicating that mce1 locus is an operon. Examination of M. leprae specific mRNA from lepromatous leprosy patient's biopsy showed that mce locus is transcribed as an operon in the pathogen also.
1 Introduction
Mycobacteria are gram-positive, acid fast staining actinobacteria that includes important human pathogens such as Mycobacterium tuberculosis and Mycobacterium leprae. Leprosy caused by M. leprae is still a major health problem in developing countries such as India (http://www.webspawner.com/usersindiatlmstat/). Identification of immunogenic antigens of these pathogens was pursued to develop immunodiagnostic tools, since vaccination against these intracellular pathogens was not very effective [1–5]. Surface exposed membrane proteins play a major role in host–pathogen interaction since these are the earliest proteins to interact with the host cell [6,7]. M. tuberculosis genome has four loci each containing six genes encoding Mce proteins that show close sequence homology. Of these mce1A gene was examined by many workers [8–11] and found to be a membrane protein capable of enhancing the entry of even Escherichia coli carrying the mce1A gene into HeLa cells that are normally refractory to E. coli. These studies clearly indicated that this protein is involved in cell entry and possibly survival of the pathogen inside macrophages. In addition the structural domains of the Mce protein were also examined [12] and found to have T-cell epitopes. The understanding of the biology of M. leprae has improved significantly due to the availability of whole genome sequence [13] even though this organism is uncultivable. Computational analysis of M. leprae genome showed that there are three mce loci of which two carry pseudogenes [13]. One of the loci, mce1 is complete and the predicted open reading frames in this locus could possibly encode proteins with homology in deduced amino acid sequence and similarity in motif architecture to those of the M. tuberculosis mce1 locus. However, there are no experimental studies of transcription of mce locus of M. leprae except the report of sequencing of this region [13,14].
M. smegmatis is the only fast growing mycobacteria that is being used extensively to study genes of pathogenic mycobacteria such as M. leprae and M. tuberculosis[15]. Since M. leprae is uncultivable in culture media we have examined the expression of the cloned mce locus of M. leprae in the heterologous host M. smegmatis and used these transformants for further analysis of M. leprae mce genes. We have used the E. coli plasmid vector pSET152 carrying Streptomyces phage ∅C31 att int region to integrate a single copy of cloned foreign gene in M. smegmatis chromosome. This approach allowed us to overcome problems associated with expression of multiple copies of foreign genes in a heterologous host. Even though the integration of pSET152 disrupted a gene encoding a subunit of amidotransferase the recombinant M. smegmatis grew normally in routine culture media. Recombinant plasmid carrying full length mce locus and deletion derivatives were also integrated into the chromosome of M. smegmatis. RT-PCR analysis of these transformants showed co-transcription of mce genes. RT-PCR analysis of mRNA extracted from biopsy samples of lepromatous leprosy patients showed that the transcriptional pattern of mce genes in M. leprae is similar to that found in the heterologous host. We also show the absence of M. leprae transcripts in non-lepromatous skin diseases such as psoriasis and eczema [16,17]. These results imply that mce1 locus is an operon and M. smegmatis could be a heterologous host of choice in expressing M. leprae genes to study their biological function.
2 Materials and methods
2.1 Plasmids and bacterial strains
Restriction enzymes, T4 DNA ligase and Calf intestine alkaline phosphatase were from Gibco-BRL (Grand Island, NY.), Promega (Madison, WI) and New England Biolabs (Beverly, USA) respectively. Prime-a-Gene kit for random labeling was from Promega (Madison, WI). α32P-dCTP [Board of Radiation and Isotope Technology (Bombay, India)], DNase I free RNase (Sigma), Molecular weight markers [(Gibco-BRL Life Technologies (Grand Island, NY)] and pBluescript II SK+ (pBSK+) [Stratagene, (Lajolla, CA)] were used for cloning and sequencing. λ DASH II genomic library construction kit from Stratagene (Lajolla, CA). E. coli strains DH5α (lab collection) and XL1 Blue MRF′ (Stratagene, Lajolla, CA) were used as cloning hosts. M. smegmatis mc2 155 SMR5, was kindly provided by Erick C Bottger (Medizinische Hochschule Hannover, Institute für Medizinische Mikrobiologie, Hannover). Armadillo spleen tissue infected with M. leprae was a gift of late M.J. Colston (Laboratory for Leprosy and Mycobacterial Research, National Institute for Medical Research, Mill Hill, London). Plasmid pSET152 (accession number AJ414670) was from Iain S. Hunter, University of Strathclyde, London.
2.2 Media and culture conditions
E. coli strains and M. smegmatis were grown in Luria–Broth (LB) supplemented with antibiotics at the following concentrations: apramycin (50 µg ml−l), ampicillin (100 µg ml−l) and streptomycin (40 µg ml−l). LB medium was supplemented with 0.2% glycerol and 0.05% Tween 80 for the cultivation of mycobacteria. Brain Heart Infusion Broth (BHIB) (Himedia) supplemented with 0.2% glycerol and 0.05% Tween 80 was used for the preparation of competent cells of M. smegmatis.
2.3 Electroporation
E. coli cells were electrotransformed using 100 ng of DNA as per the manufacturer's instruction using gene pulser (Bio-Rad, Hercules, CA). The competent cells of M. smegmatis was prepared as described by Snapper et al. [18] and 1 µg of plasmid DNA was used for electroporation of M. smegmatis using the following settings: voltage 1.4 kV, capacitance 25 μF, resistance 1000 Ω.
2.4 DNA techniques
DNA cloning was carried out as described already [19]. M. smegmatis genomic DNA extraction was done according to the procedure described previously [20]. Plasmid DNA extraction was done by alkali lysis method [21] and for sequencing the DNA was precipitated once using polyethylene glycol. For Southern analysis, 3 µg of genomic DNA was digested with BamHI separated electrophoretically in an agarose gel. Southern transfer, hybridization and detection were carried out as per the instructions provided by manufacturer (Hybond, Amersham BioSceinces, Hong Kong). Radiolabeled [32P] probes were prepared as per the manufacturer's instruction (Promega, Madison, WI).
2.5 Cloning of hybrid integration site
The presence of a single BamHI site in the plasmid pSET152 allowed the cloning of one of the phage-host hybrid integration sites. One microgram of genomic DNA from transformant was digested with BamHI, extracted with phenol:chloroform and precipitated with ethanol. DNA was ligated using T4 DNA ligase and used for transforming E. coli DH5α. Apramycin resistant transformants were purified and plasmid DNA was isolated for further studies. One such recombinant plasmid (pSE) was digested with PstI and BamHI. The resulting 2.3 kb fragment (Fig. 1) was cloned into pBSK+. A partial sequencing of 2.3 kb DNA was done with M13 forward and reverse primers from two independent clones.
![Integration of pSET152 and cloning of attR locus of M. smegmatis. (a) Apramycin resistant pSET152 transformants were generated as described under materials and methods. Genomic DNA from four transformants was isolated, digested with BamHI and separated on agarose gels. For the Southern analysis of these digests probe used was radiolabeled [32P] pSET152 plasmid DNA. (b) Schematic diagram showing the integration event and the sizes of various restriction fragments based on the pSET152 sequence data. The regions in the plasmid ∅C31 int attP, and apramycin resistance marker, are shown as grey box and dark arrow. Integration occurred in the attB site generating the right and left attachment sites denoted as attR and attL. (c) Physical map of a recombinant plasmid pSE. Restriction sites, P, PstI; E, EcoRI; H, HindIII; B, BamHI.](https://oup.silverchair-cdn.com/oup/backfile/Content_public/Journal/femspd/45/2/10.1016_j.femsim.2005.05.004/1/m_FIM_291_f1.jpeg?Expires=1750369691&Signature=GyZ7BBt-EjzuFROhtFxyoMmu4J3SNnftH4ENLc1FPOACBeg0XiMtLRGkmSxRrRJOtsaXVaqXMb9NY3~YEkJ7bZm42dkqAdu2Ms72LdrTNRM5l3gRdv0cokSr0naWd-Nq4UOdg79Y9GrU9~mw~6w1u2JvVFpWSBeE0x5I1h54BZQkLinohX-HmLdsZMYHpsO61t1JnWbo9m3ri~5X6Ex7dmI8G1-qND1NTtpy0oUJVGFTkd0UuhOKpaP4bYNrUsVAtjKWmLFtYFAROj4cMmB3dlQNUcyAU8FGvOzcGBz0DyIbnmHdDl3PaOJwPgyjr6VXbuHGxrRABxg13owuyUj5fw__&Key-Pair-Id=APKAIE5G5CRDK6RD3PGA)
Integration of pSET152 and cloning of attR locus of M. smegmatis. (a) Apramycin resistant pSET152 transformants were generated as described under materials and methods. Genomic DNA from four transformants was isolated, digested with BamHI and separated on agarose gels. For the Southern analysis of these digests probe used was radiolabeled [32P] pSET152 plasmid DNA. (b) Schematic diagram showing the integration event and the sizes of various restriction fragments based on the pSET152 sequence data. The regions in the plasmid ∅C31 int attP, and apramycin resistance marker, are shown as grey box and dark arrow. Integration occurred in the attB site generating the right and left attachment sites denoted as attR and attL. (c) Physical map of a recombinant plasmid pSE. Restriction sites, P, PstI; E, EcoRI; H, HindIII; B, BamHI.
A genomic library was constructed using Sau3AI partial digest of genomic DNA in λ DASH II. The lambda clones were screened using attR (2.3 kb) containing fragment as probe. Phage DNA from a positive clone was extracted and digested with BamHI; Southern analysis showed a 5.8 kb fragment hybridizing to the probe. This fragment was subcloned into pBSK+ and named pB3.5.2.1. Southern analysis of EcoRI digest of pB3.5.2.1 showed a 3.7 and 1.2 kb fragments hybridizing with the attR probe. These fragments were subcloned into pBSK+ and PCR analysis of clones (universal forward primer and AMD2; universal reverse primer and AMD2) showed the cross over site is in 3.7 kb fragment. The clone carrying this fragment was sequenced with two primers AMD0 and AMD2 (Fig. 2 (a) and (b)).
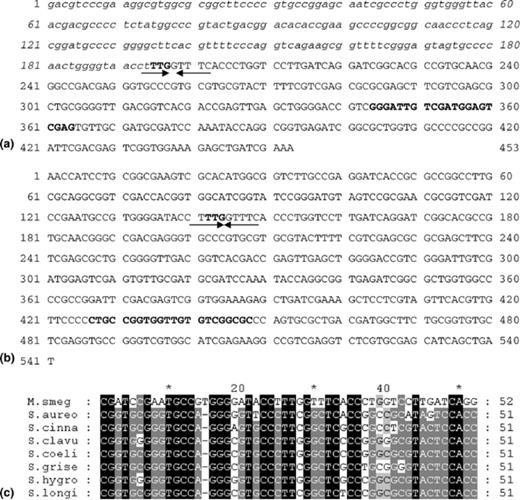
Nucleotide sequence of attB region of M. smegmatis. (a) The sequence of attR region subcloned in pBSK+ vector was determined using M13 primers. The sequence shown in italics is the ∅C31 attP sequence. The putative cross over region TTG is shown in bold. Sequence of mycobacterial genomic DNA sequence follows TTG. Sequence of AMD0 primer is shown in bold. (b) Cloning and sequencing of attB region from genomic clone of M. smegmatis mc2 155 SMR5 was done as described. Sequence of AMD2 primer is shown in bold. The perfect inverted repeat at cross over region is shown as arrows. The putative cross over region 5′TTG is also marked. (c) Alignment of attB sequences of M. smegmatis and several Streptomyces species. M. smeg, M. smegmatis mc2155 SMR5; S. aureo, S. aureofaciens; S. cinna, S. cinnamonensis; S. clavu, S. clavuligerus; S. coeli, S. coelicolor A3(2) strain 145; S. grise, S. griseus ATCC12475; S. hygro, S. hygroscopicus NRRL 5491; S. longi, S. longisporoflavus 83E6. Light shading, 50% conserved; dark sharding, 75% conserved; solid boxes, identical sequences.
2.6 Isolation of M. leprae DNA
Spleen tissue from infected Armadillo was thawed and cut into small pieces and suspended in 5 ml of β-mercaptoethanol (2%). The tissue was mixed with glass beads and incubated at 30 °C for 3 h on a rocking platform. The tissue suspension was centrifuged at 300g and the supernatant was saved. The pellet was again incubated overnight with 2%β-mercaptoethanol as described above and again spun at 300g for 10 min. The supernatants were pooled and centrifuged at 12,000g for 10 min. The pellet containing the bacilli was resuspended in 2 ml resuspension buffer (10 mM Tris–Cl pH 8.0, 0.1 M EDTA pH 8.0, 0.02 M NaCl) and stored at −20 °C.
One milliliter of M. leprae bacterial suspension was mixed with 0.1 mm glass beads in a centrifuge tube and liquid nitrogen was added. The frozen mixture was homogenized. After thawing, 3 ml of lysis buffer (10 mM EDTA pH 8.0, 100 mM NaCl, 150 mM Tris–Cl pH 8.0, 3.5% SDS, proteinaseK 250 µg ml−l) was added and incubated at 55 °C for 2 h on a rocking platform. The lysate was extracted with phenol:chloroform and then 0.6 volume of absolute ethanol was added to the aqueous layer and kept at −20 °C overnight. DNA was precipitated by centrifugation at 12,000g at 4 °C for 15 min. The pellet was washed with 70% ethanol air dried and dissolved in TE buffer (10 mM Tris–Cl pH 8.0, 1 mM EDTA pH 8.0).
2.7 Construction of genomic library of M. leprae and subcloning of mce genes
M. leprae genomic library was constructed as described above and screened with labeled RT-PCR product of mce1A (226 bp) amplified using mRNA from human biopsy samples. Primers for the amplification are shown in Table 1. DNA from a positive lambda clone was digested with SphI and SalI in separate reactions and digests was separated on agarose gel and hybridized with mce1A probe. Fragments hybridizing with the probe were eluted and cloned into pBSK+ and pUC18. Recombinant pBSK+ plasmid carrying SphI fragment (11.4 kb) and pUC18 carrying SalI fragment (2.4 kb) were digested and the inserts released with respective enzymes were end filled using DNA polymeraseI. These end filled fragments were cloned into pSET152 to get pSETMCEFL and pSETMCE1A. Further subcloning from pSETMCEFL is shown in the results. The plasmid pSETMCEFL was digested with BamHI that released two BamHI fragments of sizes 3.3 and 2.1 kb, the remaining 5.8 kb of mce1 locus along with pSET152 backbone was self-ligated to construct pSETMCEDEF. The 2.1 kb BamHI fragment released from pSETMCEFL was cloned into pSET152/BamHI to construct pSETMCE1B. The identity of insert was confirmed by sequence determination. The recombinant plasmids were electrotransformed into M. smegmatis as described earlier.
Primer sequence | Gene | Amplicon size (bp) | Primer set |
YrbEAFP:5′ACTCATCGCTGGCTTGGTC3′ | |||
YrbEARP:5′TAGCGGCTCAGGGCATTAG3′ | yrbE1A/yrbE1B | 327 | J1 |
YrbEBFP:5′TCTGGTCGTTCTTGGAGG3′ | |||
YrbEBRP:5′CATCGTCAACTTGGACTTAG3′ | yrbE1B/mce1A | 359 | J2 |
M1AF:5′ATCAACACATTGTTCCAGACC 3′ | |||
M1AR:5′TTAGCATAAGTGTCCCCCAGG 3′ | mce1A | 226 | G1 |
Ma/bF:5′CATACAACCACTTGGATAGCG3′ | |||
Ma/bR:5′AACAGAGTTGACCTTGCCC3′ | mce1A/mce1B | 286 | J3 |
M1BF:5′CAGGGCGGAACTATAAACGAC3′ | |||
M1BR:5′TAACAGTGCGTGGTCCTCAG3′ | mce1B | 279 | G2 |
Mb/cF:5′TCGTGAACTTCTACTCCTGTG3′ | |||
Mb/cR:5′TTGTCGCCCTTGTTGAGTC3′ | mce1B/mce1C | 282 | J4 |
M1CF:5′TCGGGCTGGAACATTGACAC3′ | |||
M1CR:5′GATGGTAAGAATGCGGCGAC3′ | mce1C | 440 | g3 |
Mce 1c/dFP:5′GGTGATCCAGCTCCGAAC3′ | |||
Mce 1c/dRP:5′CCACCACCAATGACCCTAC3′ | mce1C/mce1D | 282 | J5 |
M1DF:5′TAGCATCACCAAAATCATCTCC3′ | |||
M1DR:5′CAGGTTATTGACATCGGCG3′ | mce1D | 400 | g4 |
M1d/eF:5′TTCCACCACCCATCGGCTTG3′ | |||
M1d/eR:5′CGTTAATCGCCAGCGTGTCC3′ | mce1D/mce1E | 344 | J6 |
M1EF:5′AGCTACCGAAGAACACCAC3′ | |||
M1ER:5′ACCCACATAGGTCAGCAGTC3′ | mce1E | 371 | g5 |
Me/fF:5′CGAAGATAATGAGGGGCGAC3′ | |||
Me/fR:5′AGCGAATACCGACCGATAC3′ | mce1E/mce1F | 348 | J7 |
M1FF:5′AAGACCAACATCACCGACG3′ | |||
M1FR:5′GGAATGAAGCCAGTTAGACAAG3′ | mce1F | 419 | g6 |
RT16SF:5′CGAGCGTTGTCCGGAATT3′ | |||
RT16SR:5′TCCACCGCTACACCAGGAA3′ | 16SRrNA | 151 | 16SrRNA |
Primer sequence | Gene | Amplicon size (bp) | Primer set |
YrbEAFP:5′ACTCATCGCTGGCTTGGTC3′ | |||
YrbEARP:5′TAGCGGCTCAGGGCATTAG3′ | yrbE1A/yrbE1B | 327 | J1 |
YrbEBFP:5′TCTGGTCGTTCTTGGAGG3′ | |||
YrbEBRP:5′CATCGTCAACTTGGACTTAG3′ | yrbE1B/mce1A | 359 | J2 |
M1AF:5′ATCAACACATTGTTCCAGACC 3′ | |||
M1AR:5′TTAGCATAAGTGTCCCCCAGG 3′ | mce1A | 226 | G1 |
Ma/bF:5′CATACAACCACTTGGATAGCG3′ | |||
Ma/bR:5′AACAGAGTTGACCTTGCCC3′ | mce1A/mce1B | 286 | J3 |
M1BF:5′CAGGGCGGAACTATAAACGAC3′ | |||
M1BR:5′TAACAGTGCGTGGTCCTCAG3′ | mce1B | 279 | G2 |
Mb/cF:5′TCGTGAACTTCTACTCCTGTG3′ | |||
Mb/cR:5′TTGTCGCCCTTGTTGAGTC3′ | mce1B/mce1C | 282 | J4 |
M1CF:5′TCGGGCTGGAACATTGACAC3′ | |||
M1CR:5′GATGGTAAGAATGCGGCGAC3′ | mce1C | 440 | g3 |
Mce 1c/dFP:5′GGTGATCCAGCTCCGAAC3′ | |||
Mce 1c/dRP:5′CCACCACCAATGACCCTAC3′ | mce1C/mce1D | 282 | J5 |
M1DF:5′TAGCATCACCAAAATCATCTCC3′ | |||
M1DR:5′CAGGTTATTGACATCGGCG3′ | mce1D | 400 | g4 |
M1d/eF:5′TTCCACCACCCATCGGCTTG3′ | |||
M1d/eR:5′CGTTAATCGCCAGCGTGTCC3′ | mce1D/mce1E | 344 | J6 |
M1EF:5′AGCTACCGAAGAACACCAC3′ | |||
M1ER:5′ACCCACATAGGTCAGCAGTC3′ | mce1E | 371 | g5 |
Me/fF:5′CGAAGATAATGAGGGGCGAC3′ | |||
Me/fR:5′AGCGAATACCGACCGATAC3′ | mce1E/mce1F | 348 | J7 |
M1FF:5′AAGACCAACATCACCGACG3′ | |||
M1FR:5′GGAATGAAGCCAGTTAGACAAG3′ | mce1F | 419 | g6 |
RT16SF:5′CGAGCGTTGTCCGGAATT3′ | |||
RT16SR:5′TCCACCGCTACACCAGGAA3′ | 16SRrNA | 151 | 16SrRNA |
Primer sequence | Gene | Amplicon size (bp) | Primer set |
YrbEAFP:5′ACTCATCGCTGGCTTGGTC3′ | |||
YrbEARP:5′TAGCGGCTCAGGGCATTAG3′ | yrbE1A/yrbE1B | 327 | J1 |
YrbEBFP:5′TCTGGTCGTTCTTGGAGG3′ | |||
YrbEBRP:5′CATCGTCAACTTGGACTTAG3′ | yrbE1B/mce1A | 359 | J2 |
M1AF:5′ATCAACACATTGTTCCAGACC 3′ | |||
M1AR:5′TTAGCATAAGTGTCCCCCAGG 3′ | mce1A | 226 | G1 |
Ma/bF:5′CATACAACCACTTGGATAGCG3′ | |||
Ma/bR:5′AACAGAGTTGACCTTGCCC3′ | mce1A/mce1B | 286 | J3 |
M1BF:5′CAGGGCGGAACTATAAACGAC3′ | |||
M1BR:5′TAACAGTGCGTGGTCCTCAG3′ | mce1B | 279 | G2 |
Mb/cF:5′TCGTGAACTTCTACTCCTGTG3′ | |||
Mb/cR:5′TTGTCGCCCTTGTTGAGTC3′ | mce1B/mce1C | 282 | J4 |
M1CF:5′TCGGGCTGGAACATTGACAC3′ | |||
M1CR:5′GATGGTAAGAATGCGGCGAC3′ | mce1C | 440 | g3 |
Mce 1c/dFP:5′GGTGATCCAGCTCCGAAC3′ | |||
Mce 1c/dRP:5′CCACCACCAATGACCCTAC3′ | mce1C/mce1D | 282 | J5 |
M1DF:5′TAGCATCACCAAAATCATCTCC3′ | |||
M1DR:5′CAGGTTATTGACATCGGCG3′ | mce1D | 400 | g4 |
M1d/eF:5′TTCCACCACCCATCGGCTTG3′ | |||
M1d/eR:5′CGTTAATCGCCAGCGTGTCC3′ | mce1D/mce1E | 344 | J6 |
M1EF:5′AGCTACCGAAGAACACCAC3′ | |||
M1ER:5′ACCCACATAGGTCAGCAGTC3′ | mce1E | 371 | g5 |
Me/fF:5′CGAAGATAATGAGGGGCGAC3′ | |||
Me/fR:5′AGCGAATACCGACCGATAC3′ | mce1E/mce1F | 348 | J7 |
M1FF:5′AAGACCAACATCACCGACG3′ | |||
M1FR:5′GGAATGAAGCCAGTTAGACAAG3′ | mce1F | 419 | g6 |
RT16SF:5′CGAGCGTTGTCCGGAATT3′ | |||
RT16SR:5′TCCACCGCTACACCAGGAA3′ | 16SRrNA | 151 | 16SrRNA |
Primer sequence | Gene | Amplicon size (bp) | Primer set |
YrbEAFP:5′ACTCATCGCTGGCTTGGTC3′ | |||
YrbEARP:5′TAGCGGCTCAGGGCATTAG3′ | yrbE1A/yrbE1B | 327 | J1 |
YrbEBFP:5′TCTGGTCGTTCTTGGAGG3′ | |||
YrbEBRP:5′CATCGTCAACTTGGACTTAG3′ | yrbE1B/mce1A | 359 | J2 |
M1AF:5′ATCAACACATTGTTCCAGACC 3′ | |||
M1AR:5′TTAGCATAAGTGTCCCCCAGG 3′ | mce1A | 226 | G1 |
Ma/bF:5′CATACAACCACTTGGATAGCG3′ | |||
Ma/bR:5′AACAGAGTTGACCTTGCCC3′ | mce1A/mce1B | 286 | J3 |
M1BF:5′CAGGGCGGAACTATAAACGAC3′ | |||
M1BR:5′TAACAGTGCGTGGTCCTCAG3′ | mce1B | 279 | G2 |
Mb/cF:5′TCGTGAACTTCTACTCCTGTG3′ | |||
Mb/cR:5′TTGTCGCCCTTGTTGAGTC3′ | mce1B/mce1C | 282 | J4 |
M1CF:5′TCGGGCTGGAACATTGACAC3′ | |||
M1CR:5′GATGGTAAGAATGCGGCGAC3′ | mce1C | 440 | g3 |
Mce 1c/dFP:5′GGTGATCCAGCTCCGAAC3′ | |||
Mce 1c/dRP:5′CCACCACCAATGACCCTAC3′ | mce1C/mce1D | 282 | J5 |
M1DF:5′TAGCATCACCAAAATCATCTCC3′ | |||
M1DR:5′CAGGTTATTGACATCGGCG3′ | mce1D | 400 | g4 |
M1d/eF:5′TTCCACCACCCATCGGCTTG3′ | |||
M1d/eR:5′CGTTAATCGCCAGCGTGTCC3′ | mce1D/mce1E | 344 | J6 |
M1EF:5′AGCTACCGAAGAACACCAC3′ | |||
M1ER:5′ACCCACATAGGTCAGCAGTC3′ | mce1E | 371 | g5 |
Me/fF:5′CGAAGATAATGAGGGGCGAC3′ | |||
Me/fR:5′AGCGAATACCGACCGATAC3′ | mce1E/mce1F | 348 | J7 |
M1FF:5′AAGACCAACATCACCGACG3′ | |||
M1FR:5′GGAATGAAGCCAGTTAGACAAG3′ | mce1F | 419 | g6 |
RT16SF:5′CGAGCGTTGTCCGGAATT3′ | |||
RT16SR:5′TCCACCGCTACACCAGGAA3′ | 16SRrNA | 151 | 16SrRNA |
2.8 Sequence analysis
DNA sequence analysis was done in the FIST (Funds for Improvement of Science and Technology Infrastructure) facility of the School of Biotechnology, Madurai Kamaraj University, India using ABI PRISM™ 310 automated sequencer. The DNA sequence was analyzed using the Genetics Computer Group (GCG) package and sequence comparisons were done using the BLAST programme from the National Center for Biotechnology Information World Wide Web server [22]. Multiple sequence analysis was done using the GCG package and BioEdit [23]. The sequence was edited and shaded using GeneDoc program (http://www.cris.com~ketchupgendoc.shml).
2.9 RT-PCR analysis of M. smegmatis RNA
Total RNA was extracted using the protocol described earlier [24] with modifications. Cells from 250 ml of culture was mixed with 25 ml of liquid nitrogen and homogenized with 6 ml of denaturing solution [guanidinium isothiocyanate (25 g), water (29.3 ml), 0.75 M sodium acetate pH 7.0 (1.76 ml), 10% Sarkosyl (2.64 ml), β-mercaptoethanol (410 µl)] in a centrifuge tube using syringe and needle and incubated for 5 min at 4 °C. Resulting clear suspension was mixed with 600 µl of 2 M sodium acetate pH 4.0, 6 ml of water saturated phenol and 800 µl of chloroform:iso-amyl alcohol (49:1) and kept for 5 min at 4 °C. Aqueous layer was separated by centrifugation at 12,000g for 15 min. RNA was precipitated using isopropanol. Precipitated RNA was mixed with 2 ml of denaturing solution and reprecipitated with isopropanol. The pellet was washed with 70% ethanol and resuspended in 200 µl of RNase free Milli-Q water and treated with DNase I. RNA concentration and purity were determined by measuring the absorbance at 260 nm and 280 nm.
Reverse transcription was carried out in a reaction mix containing the following: 3 µl of random primer (1.0 µg µl−1) or (oligo dT 2.0 µg µl−1), 1 µg RNA template and DEPC treated water was added to make the final reaction volume to 12.75 µl. The reaction mixture was incubated at 70 °C for 10 min and chilled on ice. At the end of the incubation period the master mix containing the following: 5 µl of 5×reaction buffer (250 mM Tris–HCl pH 8.3, 375 mM KCl and 15 mM MgCl2), 1 µl of RNAsin (40 U µl−l), 1.25 µl of dNTPs (10 mM each) and 200 U (1.25 µl) of M-MuLV reverse transcriptase enzyme were added and incubated at 37 °C for 1 h. The enzyme was heat inactivated at 92 °C for 2 min and the cDNA was stored at −20 °C. Aliquots of cDNA (equivalent to 40 ng of total RNA) were used as template for PCR with the cycling parameters of initial denaturation 95 °C, 3 min; after that 40 cycles of 95 °C for 1 min; 55 °C for 45 s; 72 °C for 45 s with final extension at 72 °C for 5 min. All primers were used at a final concentration of 300 nM. PCR reaction was performed in a reaction mix containing 1 µl of cDNA, 1.0 U of Taq polymerase, 1 mM MgCl2, and 100 mM dNTPs. Finally, PCR products were electrophoresed on a 2% agarose gel. Control experiments using 1 µg of total RNA without reverse transcriptase did not show amplification for any set of primers.
2.10 RT-PCR Analysis of bacterial mRNA from biopsy tissue
Punch biopsies were collected as per the Ethical norms certified by Indian Council of Medial Research, India and Institutional Ethical Committee (Madurai Kamaraj University, India). Six biopsies from lepromatous leprosy patients were used. Fixation, embedding in paraffin and extraction of mRNA was as described by Shabaana et al. [25]. RNA preparations were treated with DNase I and also a reverse transcriptase minus control was always included to confirm the absence of DNA contamination in the template. Total RNA (3 µg) was used for reverse transcription using random primer and oligodT primers in a total volume of 50 µl. PCR amplification using 1 µl cDNA was performed with the following parameters: Denaturation 3 min at 94 °C, annealing 1 min at 55–58 °C depending on the primer sequence and elongation at 72 °C for 1 min with a final extension for 7 min at 72 °C for 36 cycles. PCR products were analysed in 2% agarose gel and visualized by ethidium bromide staining.
2.11 Nucleotide sequence accession number
The attR and attB sequences have been submitted to the GenBank database under Accession Nos. AF199359 and AF542511, respectively.
3 Results
3.1 Integration of plasmid vector pSET152 in the chromosome of M. smegmatis
Plasmid vector pSET152 could be mobilized into several streptomyces species and the mobilized vector integrated mostly at a specific locus in the host chromosome using the att int functions of the Streptomyces phage ∅C31. Since this plasmid does not replicate in Streptomyces the recovered recombinants must harbor the plasmid only as an integrated copy [26,27]. This intergeneric conjugation was used effectively to integrate a single copy of the cloned foreign gene into the chromosome of the recipient and the integrated copy was shown to be stable in all cases of streptomyces [27–30] reported so far. It has been reported earlier [31] that the IncQ plasmid RSF1010 could be mobilizable by the plasmid (RP4) from E. coli to M. smegmatis and the transferred plasmid was shown to be stable. Therefore, we attempted the mobilization of pSET152 (derivative of RK2 plasmid [32]) from E. coli ET12567 (pUZ8002) to M. smegmatis. Under the conditions tested, we could not mobilize the vector by conjugation. However, apramycin resistant transformants could be obtained by electroporation. Integration of the vector into the chromosome is likely to have occurred since pSET152 is unlikely to replicate in M. smegmatis. Chromosomal DNA digested with BamHI, from four transformants were analysed by Southern hybridization. Radioactively labeled pSET152 plasmid DNA was used as probe. Results in Fig. 1 (a) show that two fragments of 6.2 and 5.4 kb hybridized to the probe in all cases. There is a single BamHI site in the plasmid (Fig. 1(b)) and hence the two fragments must have chromosomal region flanking the integrated plasmid DNA sequences. Fig. 1 (b) is a schematic representation of integration and arbitrarily defined attR and attL. Transformation of self ligated BamHI digest of total chromosomal DNA allowed the cloning of the attR region. Restriction analysis of two recombinant plasmids showed that the rescued plasmids are of 6.2 kb size, indicating that attR fragment constitutes 2.9 kb chromosomal DNA and 3.3 kb of plasmid. Physical map of one such plasmid, pSE is shown in Fig. 1 (c).
3.2 Sequence analysis of the attachment site and identification of the cross over point
The fragment carrying the attR region was cloned from pSE into pBSK+ and the nucleotide sequence was determined as described under materials and methods (Fig. 2(a)). Based on the published sequence of phage ∅C31, we could deduce that the cross over region is 5′TTG. In order to obtain the entire sequence of the attB site, a genomic library of M. smegmatis was constructed using λ DASH II and the recombinant clone carrying attB region was isolated and sequenced as described. Fig. 2 (b) shows the entire attB sequence. Blast analysis of this sequence against M. smegmatis genomic sequence showed that the integration site is in the gatA gene encoding Glu-tRNAGln amidotransferase subunitA [33–35]. Alignment of attB sequences of Streptomyces and M. smegmatis (Fig. 2(c)) showed significant homology between Streptomyces and M. smegmatis attB sequences. This result also confirms that the int gene is expressed in M. smegmatis and Int protein and attP sequence are necessary and sufficient for chromosomal integration of pSET152.
3.3 Chromosomal cloning of mce locus of M. leprae
Component genes of the mce locus of M. leprae as derived from the genome sequence [13] are shown in Fig. 3 (a). Various segments of mce1 of M. leprae cloned in pSET152 are described in materials and methods. Plasmid pSETMCE1A (Fig. 3(b)) was introduced into M. smegmatis and a few transformants were screened for the presence of the integrated recombinant plasmid DNA. Total chromosomal DNA was isolated and cleaved with BamHI and the digested DNA was separated on agarose gels and Southern analysis was performed using labeled pSET152 DNA as probe. Results are shown in Fig. 3 (c). Two fragments of 7.5 and 5.4 kb hybridized with the probe. Four independent transformants showed fragments of similar size hybridizing to the probe. Southern analysis using the 2.4 kb mce fragment as probe showed fragments of 7.5 and 1.1 kb hybridizing with the probe (Fig. 3(d)). These results show that the insertion location is same in all four transformants. Plasmids carrying the entire mce1 locus and parts of it were also transformed as above. Southern analysis of those transformants using labeled pSET152 and appropriate mce fragment confirmed that integration was in the same location for all constructs (data not shown).
![Construction of pSET152 recombinant plasmids carrying mce locus of M. leprae and chromosomal cloning of recombinant plasmids. (a) Schematic representation of the organization of mce locus and the conserved yrbE1A and yrbE1B. Restriction fragments used for cloning are shown (PS1-PS2, SphI end filled; PS3-PS4, SalI end filled; B, BamHI; S, SalI; B-PS2, BamHI and SphI end filled). (b) Integration of pSETMCE1A in the chromosome of M. smegmatis. Integration at the attB region generated the right and left attachment sites denoted as attR and attL respectively. The restriction sites shown are P, PstI; B, BamHI and H, HindIII. (c) and (d) Southern analysis of chromosomal DNA of M. smegmatis transformants carrying pSETMCE1A. Radiolabeled [32P] probes used were pSET152 plasmid (c) and 2.4 kb SalI fragment carrying mce1A (d).](https://oup.silverchair-cdn.com/oup/backfile/Content_public/Journal/femspd/45/2/10.1016_j.femsim.2005.05.004/1/m_FIM_291_f3.jpeg?Expires=1750369692&Signature=Mh9q~rdA4iKRDTm-8Ra6GHRINXlbxpGvNeRKpLxGhvzvS-rFO7QbrbIbdYnT3X5~nQvJdFd6W1ybPypzEkG74sKjfU6pgUBT9CI54GmJ7OkBykJUDaGemHu-gO9WacAi-KqpRZEdg2eVPH9t2xVsGmrd04QR5xvFEyKGsnrnFS1VxURDPCpMK8KrOQXJq2IXDKxs76lSuI7Rl6z~7XzB9zuQJq7eHg0WaNzWN9NffUuqnOULFYx4iHxGL0SRY12s9vxqLNR-QLb8harxYVH3lvZ4t2~IR7RRrhw0ZxHcoyaDkfDdzD7qb1jsaOueBRkz43wNORk5-zm7LS0NMvFanA__&Key-Pair-Id=APKAIE5G5CRDK6RD3PGA)
Construction of pSET152 recombinant plasmids carrying mce locus of M. leprae and chromosomal cloning of recombinant plasmids. (a) Schematic representation of the organization of mce locus and the conserved yrbE1A and yrbE1B. Restriction fragments used for cloning are shown (PS1-PS2, SphI end filled; PS3-PS4, SalI end filled; B, BamHI; S, SalI; B-PS2, BamHI and SphI end filled). (b) Integration of pSETMCE1A in the chromosome of M. smegmatis. Integration at the attB region generated the right and left attachment sites denoted as attR and attL respectively. The restriction sites shown are P, PstI; B, BamHI and H, HindIII. (c) and (d) Southern analysis of chromosomal DNA of M. smegmatis transformants carrying pSETMCE1A. Radiolabeled [32P] probes used were pSET152 plasmid (c) and 2.4 kb SalI fragment carrying mce1A (d).
3.4 mce locus is co-transcribed in M. smegmatis
To detect expression of mce genes in M. smegmatis, mce specific mRNA was analyzed by RT-PCR with primers designed from available sequence data (http://genolist.pasteur.fr/Leproma). Fig. 4 (a) shows organization of mce locus and primer positions. Plasmid pSETMCEFL carries the entire mce1 locus including yrbE1A and yrbE1B. For RT-PCR analysis RNA was isolated from the transformants that carry chromosomally integrated plasmid. Results in Fig. 4 (b) show that all primers that span intragenic regions of mce1A to mce1F amplified expected sized products. This shows all the genes in the locus is transcribed. The absence of amplification in RT minus control shows that the template preparation was not contaminated with genomic DNA. RT-PCR analysis of transformants carrying mce1A alone showed amplification only when the mce1A specific primers are used (Fig. 4(b)). Absence of amplification with other primer sets confirmed absence of genomic DNA and that amplicons obtained are from transcripts of cloned mce locus and not from transcripts of mce like conserved regions of M. smegmatis. All primer pairs gave expected size amplicons if the plasmid DNA carrying the entire mce locus was used as template (see Fig. S1).
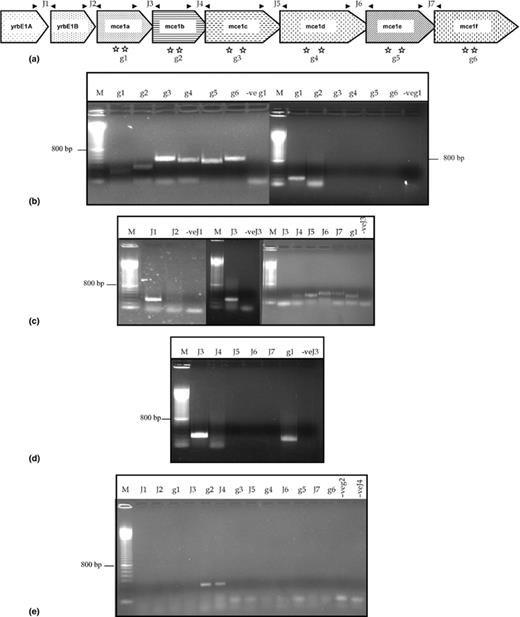
mce genes are co-transcribed in M. smegmatis. (a) Organization of mce1 genes in M. leprae genome deduced from the genome sequence. Primer positions are also shown. (b) RT-PCR amplification of mRNA isolated from transformants carrying integrated full length mce and mce1A using intragenic primers (g1–g6). In Fast migrating bands are the primer dimers. (c) RT-PCR amplification using intergenic junction primers (J1 to J7) using RNA from transformants carrying full length mce (mce1A–mce1F). In all cases except for the middle pan 40 ng equivalent of cDNA was used as template. In the middle pan (primer set J3) the template concentration used is 60 ng cDNA equivalent. (d) and (e) RNA from transformants carrying mce1A and mce1B respectively was isolated and used for RT-PCR analysis. For figures (b)–(e), name of primer sets used are shown above the lanes. M, molecular weight markers; 100 bp ladder. (The molecular weight marker bands are separated by 100 bp increment. Bands stain uniformly with ethidium bromide, except for the 800 bp band, which is approximately twice as intense as the other bands.) −ve is RT minus control.
The organization of mce locus shows that the ORFs overlap. This implied that the mce genes might be co-transcribed. Northern analysis of RNA showed a high molecular weight band hybridizing with mce probes (data not shown) only in the transformants. Therefore, we examined the amplification using intergenic primers that could amplify if the genes are co-transcribed as a single mRNA. Results in Fig. 4 (c) show that the primers spanning junction of yrbE1B and mce1A do not give any amplicon. However, all the other primer pairs amplified expected sized products indicating the presence of RNA spanning the gene junctions also. RNA from transformants carrying integrated copy of pSETMCE1A showed amplification with mce1A mce1B (J3) primers only (Fig. 4(d)). The absence of amplification with yrbE1B mce1A (J2 primer set) indicates that the RNA start point is down stream of the yrbEBFP (Table 1) primer locus. YrbEBFP site is 209 bp upstream of mce1A start codon. RNA from the transformants carrying mce1B alone shows that there was amplification from mce1B using intragenic (g2) as well as intergenic (J4) primers (Fig. 4(e)). Transformants carrying pSETMCEDEF did not show any transcript of M. leprae mce1 locus.
3.5 Analysis of mce genes transcripts in human biopsy samples
We have shown earlier that M. leprae mRNA could be isolated from biopsy material fixed and embedded in paraffin [25]. The same methodology was used to extract and analyze mRNA from lepromatous leprosy cases and from infected Armadillo spleen tissue. Intragenic primers and junction primers used for the PCR analysis of M. smegmatis transformants were used in these experiments also. Fig. 5 shows the RT-PCR results using mRNA from six LL biopsy and Armadillo tissue. In all cases inter and intragenic primer pairs amplified the expected size amplicons. As has been shown in M. smegmatis (Fig. 4(b)), primers spanning yrbE1B and mce1A (J2) did not give any amplification product in biopsy samples too. This result clearly shows similarity of transcription profile in cloned mce1 locus and in biopsy sample. RT-PCR analysis of skin biopsies from eczema and psoriasis patients did not show mce1A and mce1E transcripts indicating the specificity of the primers (see Fig. S2).

RT-PCR analysis of mRNA from biopsy samples and Armadillo spleen tissue. Sequences of primers used are described in Table 1. Lanes are marked with names of primer sets used for amplification. −ve is RT minus control. M, molecular weight marker. Row A, mRNA from Armadillo infected spleen tissue. Rows B to G, mRNA from biopsy material of LL patients. (B) male; bacterial index (BI), 3.7 (C) female; BI 4.2 (D) male; BI, 5.0 (E) male; BI, 5.0 (F) female; BI, 3.0 and (G) female; BI, 3. 0. A, C, D, E, F and G is having 100 bp ladder and B is having 50 bp ladder (each band is separated by 50 bp increment. Bands stain uniformly with ethidium bromide except for 250 bp band which is seen as very intense than other bands) as molecular weight markers.
4 Discussion
M. leprae genome has undergone several mutational changes resulting in the accumulation of an estimated 1116 pseudogenes, including genes involved in essential biosynthetic pathways [13,36]. In the case of mce loci there are two pseudogenes and one functional mce1 locus. Pseudogenes mce4 and mce1 are truncated and have only one of the six genes, namely ψmce4a and ψ mce1a respectively. This shows that ψmce1A might have duplicated from the complete functional mce1 locus. Six genes of functional mce1 locus, mce1A to mce1F has overlapping open reading frames in M. leprae genome. Our experimental results show that there is a promoter for mce locus within yrbE1B structural gene. Identification of a promoter internal to yrbE1B of M. tuberculosis[37] shows that yrbE1A and yrbE1B might have co-evolved with mce genes in M. tuberculosis and M. leprae. Even in S. coelicolor and S. avermitilis, the mce like loci have yrbE1A and yrbE1B homologs upstream of mce genes (J.J. Maheshwari and K. Dharmalingam, unpublished data). In this report, we examined the expression of mce genes in the heterologous host M. smegmatis and extended the observations to M. leprae encoded products in human biopsies and Armadillo spleen tissues.
Cloning a single copy of foreign gene as an integrated stable entity would represent the in vivo conditions that exist in the native organism from which the gene was cloned. Cloning vector pSET152 has been demonstrated to be a versatile integrative vector with an added advantage of mobilization by conjugation from E. coli to Streptomyces[27,32]. Mobilized plasmid integrated at a specific locus in majority of cases and in some cases they also integrated at pseudo attachment sites [26]. Earlier report demonstrating the mobilization of the IncQ plasmid RSF1010 from E. coli to M. smegmatis[31] prompted us to examine if pSET152 could be mobilized from E. coli ET12567 carrying the mobilization functions on a non-transferable plasmid pUZ8002. Repeated attempts did not yield any exconjugants. However, electroporation of M. smegmatis with pSET152 yielded transformants carrying integrated copy of pSET152. First the sequence of the hybrid site attR was determined by cloning the attR site as described earlier. The cross over site deduced from the attB and attR sequence is 5′TTG, which is the canonical cross over site in streptomyces as well. These results imply that the att site and int encoded functions are sufficient for integrations in M. smegmatis chromosome. In most cases, phage attachment and integration of horizontally transferred genes occur either within or near the tRNA genes [38–40]. However, in this case, pSET152 integration occurred within gene encoding amidotransferase subunitA of putative transamidase. The transformants are viable in standard culture media indicating that the amidase gene is not needed for the growth of the organism. However, other possibilities can not be ruled out.
Initial Northern analysis of mRNA isolated from M. smegmatis transformants carrying the entire mce1 locus showed a diffuse high molecular weight band hybridizing to mce1A probe indicating the possibility of a longer transcript from mce locus. RT-PCR analysis showed that mRNA encoding mce genes is present only in the transformant carrying recombinant plasmid as an integrated copy. This data shows that the primer sets used are M. leprae mce mRNA specific and M. smegmatis genome does not carry any functional mce like genes. Primers specific to 3′ end of preceding gene and 5′ end of the succeeding gene allowed us to confirm the presence of mRNA spanning intergenic junction. These results show that the entire mce locus must be transcribed as a single mRNA. In pSETMCEFL the transcript of yrbE1A is not continued beyond yrbE1B since there was no amplification of the junction region of yrbE1B and mce1A. The transcript from promoter upstream of yrbE1A possibly terminate ahead of J2 reverse primer binding site. It also indicate that the promoter should be located inside the 209 bp region upstream of mce1A start codon. This promoter in yrbE1B transcribe mce1 locus to synthesize full length polycistronic mce transcript. Analysis of the RNA encoded by deletion derivatives shows the presence of another promoter internal to the mce1A gene, because pSETMCE1B (Fig. 3(a)) which carries only 3′ part of mce1A is also transcribed. The absence of amplification using junction primer pair (J3) indicates that the promoter is located about 60 bp region upstream of mce1B start codon. Sequence analysis of attR region shows that the mce locus is integrated in the gatA gene in the opposite transcriptional orientation. Therefore, transcription of mce gene from gatA promoter is unlikely. The absence of any mRNA in transformants carrying integrated copy of pSETMCEDEF indicates that there is no other promoter downstream of mce1B.
Transcription of mce genes in M. leprae has not been studied. Therefore, we extended the studies to M. leprae infected human biopsy and Armadillo spleen tissues using the same primer pairs. Results clearly show that mce1 locus is an operon in M. leprae also. Taken together both sets of observations indicate that mce genes are co-regulated and M. smegmatis is a suitable heterologous host for the cloning and expression of M. leprae genes. Interestingly, the mce genes of M. leprae are different from those of M. tuberculosis. For example, there are five rare arginine codons in M. leprae mce1A gene. Our unpublished observations show that mce1A is poorly translated in E. coli and M. smegmatis indicating the difference in regulation of genes in mce1 operon present in M. tuberculosis and M. leprae even though there is significant homology in amino acid sequence of Mce proteins present in both mce1 operon.
Acknowledgements
R.S.S. acknowledges the Council of Scientific and Industrial Research, New Delhi, India for the award of a Senior Research Fellowship. N.L. thanks University Grants Commission, New Delhi for the award of Junior Research Fellowship. This research was funded by a Centre for Genetic Engineering and Strain Manipulation grant from the Department of Biotechnology, Government of India, New Delhi, India to K. Dharmalingam (BT/03/002/87-Vol. IV). Grant from Heiser program for Research in Leprosy and Tuberculosis, New York, USA is acknowledged.
We thank E.C. Bottger, M.J. Colston and Iain S. Hunter for providing bacterial strains, Armadillo spleen tissues and pSET152 respectively. We thank Ranjan Prasad for his suggestions on the manuscript.
Appendix A Supplementary data
Supplementary data associated with this article can be found, in the online version, at doi:10.1016/j.femsim.2005.05.004.
References
Author notes
Present address: Department of Biotechnology, Alagappa University, Karaikudi, 630 003, India.
present-address: Trudeau Institute Inc., Saranac Lake, NY 12983, USA.