-
PDF
- Split View
-
Views
-
Cite
Cite
Isabelly Santos Rosado de Oliveira, Ederson da Conceição Jesus, Thiago Gonçalves Ribeiro, Maura Santos Reis de Andrade da Silva, Jairo de Oliveira Tenorio, Lindete Míria Vieira Martins, Sérgio Miana de Faria, Mimosa caesalpiniifolia Benth. adapts to rhizobia populations with differential taxonomy and symbiotic effectiveness outside of its location of origin, FEMS Microbiology Ecology, Volume 95, Issue 8, August 2019, fiz109, https://doi.org/10.1093/femsec/fiz109
- Share Icon Share
ABSTRACT
Mimosa caesalpiniifolia Benth. is a legume native to the semi-arid region of Brazil, in the Northeast. Its successful adaptation to other locations, such as the Atlantic Forest in the Southeast region, may be related to its ability to establish symbiosis with nitrogen-fixing bacteria, especially β-rhizobia of the genus Paraburkholderia. The objective of this work was to determine whether M. caesalpiniifolia adapted to bacterial symbionts in locals where it was introduced. Bacteria were recovered from nodules of M. caesalpiniifolia and characterized at the genetic level by BOX-PCR and sequencing of the 16S rRNA, recA, nifH, and nodC genes. Their symbiotic effectiveness was assessed under axenic conditions. M. caesalpiniifolia nodulated mainly with Paraburkholderia sabiae and a few strains of Rhizobium in the Southeast. On the other hand, the symbionts found in the Northeast were, predominantly, Paraburkholderia diazotrophica. Regardless of its origin, P. diazotrophica promoted a superior accumulation of plant biomass than other bacterial species. The results presented here demonstrate the ability of M. caesalpiniifolia to adapt to bacterial populations outside its location of origin, and indicate that, in this case, the symbiotic effectiveness was associated with the taxonomical classification of the strains.
INTRODUCTION
Mutualistic symbioses may play a critical role in the successful introduction of a plant species into a new environment. The lack of a compatible symbiont, especially when the symbiosis is highly specific, can be a relevant barrier to the adaptation of the species, as already reported for Pinus spp., which depends on an ectomycorrhizal symbiont (Richardson et al. 2000; Odum 2001).
In the case of legumes, since their relationship with rhizobia is not hereditary (Parker 2001), it is possible that their adaptation to a new environment is due to the joint transfer of the symbiont or that those species are able to nodulate with bacteria present in the area where they were introduced (Richardson et al. 2000). Although the lack of nodulation after the introduction of a legume in a new environment is an exception in the case of tree legumes, recent studies indicate that this may be the case for some species related to β-rhizobia. For example, there is evidence that the co-introduction of the symbiont was necessary for the adaptation of Mimosa species native to South America to several countries (Chen et al. 2005a; Bontemps et al. 2010). It is also known that legumes belonging to the Piptadenia group nodulate preferentially with β-rhizobia (Bournaud et al. 2013). Therefore, it is essential to understand how plants and microorganisms are distributed in different regions, being the study of biogeography fundamental for this purpose.
Currently reclassified within the subfamily Caesalpinioideae, in the clade Mimosoid (LPWG et al. 2017), the genus Mimosa has more than 500 species that can be found in different environments (Simon et al. 2011). Among these is Mimosa caesalpiniifolia Benth., native to Northeast Brazil, where a semi-arid biome known as ‘Caatinga’ predominates. This species is characterized by rapid growth, high regeneration capacity and resistance to dry weather. Due to its rusticity and usefulness—M. caesalpiniifolia can be used as a source of wood and food for cattle, for honey production and for the restoration of degraded areas (Carvalho 2007; Passos, Tavares and Alves 2007; Babic et al. 2008)—it has been introduced in several other regions of Brazil (CNCFlora 2018). Its ability to nodulate with rhizobia, especially β-rhizobia of the genus Paraburkholderia (previously classified in Burkholderia) (Sawana, Adeolu and Gupta 2014), may be directly related to its good adaptation to other regions of Brazil, such as the Southeast region where the Atlantic Forest biome predominates. For this reason, M. caesalpiniifolia is a potential model to understand how the interaction with symbiotic microorganisms influences the adaptation of plant species to new habitats.
The present work aimed to study the phylogenetic relationships between rhizobia isolated from M. caesalpiniifolia introduced in Southeast Brazil and from several locations in the Northeast, where it is native, to unravel the relationship between the symbiont and the successful introduction of this species in other environments.
MATERIALS AND METHODS
Origin of isolates
A total of 95 isolates were obtained from nodules sampled in the field and from experiments under sterile conditions using soil as inoculum and M. caesalpiniifolia as a trap host. Nodules and soils were sampled from different municipalities in the Northeast and Southeast regions of Brazil (Table 1 and Fig. 1). Soil samples were submitted to fertility analysis (Table 2) and used to inoculate M. caesalpiniifolia seedlings used as trap hosts. The isolates were recovered from nodules and purified in yeast-mannitol agar (Fred and Waksman 1928) and stored in the Johanna Dobereiner Biological Resource Center of Embrapa Agrobiology located in Seropédica, Rio de Janeiro, Brazil.
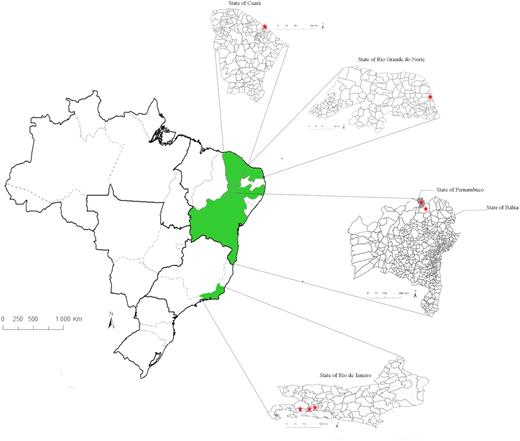
Map of Brazil showing the location of the sampling sites in different states. Ceará, Rio Grande do Norte and Pernambuco are located in the Northeast region, and Rio de Janeiro in the Southeast. The stars indicate the municipalities where soil samples were taken.
Origin of the studied rhizobia isolates in the Brazilian territory, their codes and geographical coordinates.
Soil origin (city and state) . | Geographical region . | Number of isolates . | Strain identity . | Geographic coordinates . |
---|---|---|---|---|
Fortaleza, Ceará | Northeast | 1 | BR 3424 (UFC 813.35) | 9°4′18″ S 40°19′6″ W |
Natal, Rio Grande do Norte | Northeast | 5 | RN | 5°15′28″ S 36°34′49″ W |
Petrolina, Pernambuco | Northeast | 10 | CPATSA | 9°4′16.4″ S 40°19′5.37″ W |
Juazeiro, Bahia | Northeast | 15 | JUA | 9°25′19.76″ S 40°29′16.05″ W |
Itaguaí, Rio de Janeiro | Southeast | 10 | P3 | Not available |
Mangaratiba, Rio de Janeiro | Southeast | 14 | P4 and PM | K 0600256, UTM 7464349 |
Seropédica, Rio de Janeiro | Southeast | 30 | P1 and SAB | 22°45′33″ S 43°40′17″ W |
Soil origin (city and state) . | Geographical region . | Number of isolates . | Strain identity . | Geographic coordinates . |
---|---|---|---|---|
Fortaleza, Ceará | Northeast | 1 | BR 3424 (UFC 813.35) | 9°4′18″ S 40°19′6″ W |
Natal, Rio Grande do Norte | Northeast | 5 | RN | 5°15′28″ S 36°34′49″ W |
Petrolina, Pernambuco | Northeast | 10 | CPATSA | 9°4′16.4″ S 40°19′5.37″ W |
Juazeiro, Bahia | Northeast | 15 | JUA | 9°25′19.76″ S 40°29′16.05″ W |
Itaguaí, Rio de Janeiro | Southeast | 10 | P3 | Not available |
Mangaratiba, Rio de Janeiro | Southeast | 14 | P4 and PM | K 0600256, UTM 7464349 |
Seropédica, Rio de Janeiro | Southeast | 30 | P1 and SAB | 22°45′33″ S 43°40′17″ W |
Origin of the studied rhizobia isolates in the Brazilian territory, their codes and geographical coordinates.
Soil origin (city and state) . | Geographical region . | Number of isolates . | Strain identity . | Geographic coordinates . |
---|---|---|---|---|
Fortaleza, Ceará | Northeast | 1 | BR 3424 (UFC 813.35) | 9°4′18″ S 40°19′6″ W |
Natal, Rio Grande do Norte | Northeast | 5 | RN | 5°15′28″ S 36°34′49″ W |
Petrolina, Pernambuco | Northeast | 10 | CPATSA | 9°4′16.4″ S 40°19′5.37″ W |
Juazeiro, Bahia | Northeast | 15 | JUA | 9°25′19.76″ S 40°29′16.05″ W |
Itaguaí, Rio de Janeiro | Southeast | 10 | P3 | Not available |
Mangaratiba, Rio de Janeiro | Southeast | 14 | P4 and PM | K 0600256, UTM 7464349 |
Seropédica, Rio de Janeiro | Southeast | 30 | P1 and SAB | 22°45′33″ S 43°40′17″ W |
Soil origin (city and state) . | Geographical region . | Number of isolates . | Strain identity . | Geographic coordinates . |
---|---|---|---|---|
Fortaleza, Ceará | Northeast | 1 | BR 3424 (UFC 813.35) | 9°4′18″ S 40°19′6″ W |
Natal, Rio Grande do Norte | Northeast | 5 | RN | 5°15′28″ S 36°34′49″ W |
Petrolina, Pernambuco | Northeast | 10 | CPATSA | 9°4′16.4″ S 40°19′5.37″ W |
Juazeiro, Bahia | Northeast | 15 | JUA | 9°25′19.76″ S 40°29′16.05″ W |
Itaguaí, Rio de Janeiro | Southeast | 10 | P3 | Not available |
Mangaratiba, Rio de Janeiro | Southeast | 14 | P4 and PM | K 0600256, UTM 7464349 |
Seropédica, Rio de Janeiro | Southeast | 30 | P1 and SAB | 22°45′33″ S 43°40′17″ W |
Soil origina (city and state) . | Geographic region . | pH . | P . | K . | Ca . | Mg . | Al . | MO . |
---|---|---|---|---|---|---|---|---|
. | . | . | (mg L−1) . | (mg L−1) . | (cmolc dm−3) . | (cmolc dm−3) . | (cmolc dm−3) . | (%) . |
Natal, Rio Grande do Norte | Northeast | 6.8 | 3.68 | 146.84 | 3.41 | 1.08 | 0 | 0.86 |
Juazeiro, Bahia | Northeast | 7.2 | 318.22 | 154.39 | 6.99 | 1.31 | 0.1 | 1.19 |
Mangaratiba, Rio de Janeiro | Southeast | 5.0 | 18.85 | 126.94 | 2.77 | 0.61 | 0.27 | 2.43 |
Itaguai, Rio de Janeiro | Southeast | 5.7 | 4.94 | 91.32 | 2.43 | 0.61 | 0.42 | 0.51 |
Seropédica, Rio de Janeiro | Southeast | 4.8 | 2.81 | 33.51 | 0.61 | 0.16 | 0.12 | 0.88 |
Fortaleza, Cearáa | NA | NA | NA | NA | NA | NA | NA | NA |
Petrolina, Pernambucoa | NA | NA | NA | NA | NA | NA | NA | NA |
Soil origina (city and state) . | Geographic region . | pH . | P . | K . | Ca . | Mg . | Al . | MO . |
---|---|---|---|---|---|---|---|---|
. | . | . | (mg L−1) . | (mg L−1) . | (cmolc dm−3) . | (cmolc dm−3) . | (cmolc dm−3) . | (%) . |
Natal, Rio Grande do Norte | Northeast | 6.8 | 3.68 | 146.84 | 3.41 | 1.08 | 0 | 0.86 |
Juazeiro, Bahia | Northeast | 7.2 | 318.22 | 154.39 | 6.99 | 1.31 | 0.1 | 1.19 |
Mangaratiba, Rio de Janeiro | Southeast | 5.0 | 18.85 | 126.94 | 2.77 | 0.61 | 0.27 | 2.43 |
Itaguai, Rio de Janeiro | Southeast | 5.7 | 4.94 | 91.32 | 2.43 | 0.61 | 0.42 | 0.51 |
Seropédica, Rio de Janeiro | Southeast | 4.8 | 2.81 | 33.51 | 0.61 | 0.16 | 0.12 | 0.88 |
Fortaleza, Cearáa | NA | NA | NA | NA | NA | NA | NA | NA |
Petrolina, Pernambucoa | NA | NA | NA | NA | NA | NA | NA | NA |
Soil analysis is not available for Fortaleza and Petrolina.
Soil origina (city and state) . | Geographic region . | pH . | P . | K . | Ca . | Mg . | Al . | MO . |
---|---|---|---|---|---|---|---|---|
. | . | . | (mg L−1) . | (mg L−1) . | (cmolc dm−3) . | (cmolc dm−3) . | (cmolc dm−3) . | (%) . |
Natal, Rio Grande do Norte | Northeast | 6.8 | 3.68 | 146.84 | 3.41 | 1.08 | 0 | 0.86 |
Juazeiro, Bahia | Northeast | 7.2 | 318.22 | 154.39 | 6.99 | 1.31 | 0.1 | 1.19 |
Mangaratiba, Rio de Janeiro | Southeast | 5.0 | 18.85 | 126.94 | 2.77 | 0.61 | 0.27 | 2.43 |
Itaguai, Rio de Janeiro | Southeast | 5.7 | 4.94 | 91.32 | 2.43 | 0.61 | 0.42 | 0.51 |
Seropédica, Rio de Janeiro | Southeast | 4.8 | 2.81 | 33.51 | 0.61 | 0.16 | 0.12 | 0.88 |
Fortaleza, Cearáa | NA | NA | NA | NA | NA | NA | NA | NA |
Petrolina, Pernambucoa | NA | NA | NA | NA | NA | NA | NA | NA |
Soil origina (city and state) . | Geographic region . | pH . | P . | K . | Ca . | Mg . | Al . | MO . |
---|---|---|---|---|---|---|---|---|
. | . | . | (mg L−1) . | (mg L−1) . | (cmolc dm−3) . | (cmolc dm−3) . | (cmolc dm−3) . | (%) . |
Natal, Rio Grande do Norte | Northeast | 6.8 | 3.68 | 146.84 | 3.41 | 1.08 | 0 | 0.86 |
Juazeiro, Bahia | Northeast | 7.2 | 318.22 | 154.39 | 6.99 | 1.31 | 0.1 | 1.19 |
Mangaratiba, Rio de Janeiro | Southeast | 5.0 | 18.85 | 126.94 | 2.77 | 0.61 | 0.27 | 2.43 |
Itaguai, Rio de Janeiro | Southeast | 5.7 | 4.94 | 91.32 | 2.43 | 0.61 | 0.42 | 0.51 |
Seropédica, Rio de Janeiro | Southeast | 4.8 | 2.81 | 33.51 | 0.61 | 0.16 | 0.12 | 0.88 |
Fortaleza, Cearáa | NA | NA | NA | NA | NA | NA | NA | NA |
Petrolina, Pernambucoa | NA | NA | NA | NA | NA | NA | NA | NA |
Soil analysis is not available for Fortaleza and Petrolina.
Four reference strains obtained from the same culture collection were used as a reference in the experiments and molecular analyses.
Authentication assay
An authentication assay (Vincent 1970), following Koch's postulate, was carried out in a greenhouse to confirm the isolate's ability to induce nodulation in M. caesalpiniifolia. Each isolate was inoculated in 350-mL glass bottles containing sterile nutrient solution (Gruzman and Döbereiner 1968) and a pregerminated seed. Two bottles per isolate were used. Strains BR 3405 and BR 3407T (Chen et al. 2005b), both Paraburkholderia sabiae, were used as positive controls and two bottles were left uninoculated as negative controls. The seeds were immersed in 98% sulfuric acid for 4 min to break dormancy. Afterward, they were immersed in ethanol for 30 s and hydrogen peroxide 30% for 2 min, and washed thoroughly in sterile water. The seeds were germinated in a germination chamber under a temperature of 28°C and a photoperiod of 12 h, for 5 days. Once the radicle reached 1 cm in length, seeds were transferred to sterile 350-mL glass bottles. The rootlets were inoculated with 1 mL of inocula grown in yeast-mannitol broth (Fred and Waksman 1928). Each bottle contained two 20 cm × 2.0 cm (length × width) paper strips to support the seed and provide rootlets with the nutrient solution. The bottleneck was sealed to avoid external contamination. Nodulation was evaluated 45 days after inoculation.
DNA extraction
Cell cultures were prepared in yeast-mannitol broth (Fred and Waksman 1928) at 28°C under constant stirring at 150 rpm. Their genomic DNA was extracted with the Wizard Genomic DNA Purification Kit (Promega) according to the manufacturer's instructions. The DNA concentration was evaluated by spectrophotometry in a QUBIT (Invitrogen) apparatus, and the concentration was adjusted to 10 ng μL−1.
BOX-PCR analysis
BOX-PCR was performed according to the protocol described by de Bruijn, Rademaker and Schneider (1996) in 25 μL reactions using the primers and conditions described in Table S1 (Supporting Information). The reaction products were analyzed by 2% agarose gel electrophoresis and stained with ethidium bromide and photographed on a Kodak Gel Logic 100 Imaging System® Photodocumentator. The profiles obtained were analyzed with the software Bionumerics 6.6 (Applied Maths BVBA, Belgium), where they were aligned and used for the calculation of similarity matrices based on the Dice index. The dendrogram was built with the UPGMA method (unweighted pair group method using arithmetic averages). In total, 39 distinct profiles were observed, and one isolate of each was chosen for sequencing of selected genes and phylogenetic analysis.
Amplification of 16S rRNA, recA, nifH and nodC genes
The selected isolates were submitted to PCR to amplify fragments of the 16S rRNA (small subunit ribosomal ribonucleic acid), recA (recombinase A protein), nifH (nitrogenase iron protein) and nodC (N-acetylglucosaminyltransferase) genes, with the primers and conditions described in Table S1 (Supporting Information). The amplification products of each gene were analyzed by 1% agarose gel electrophoresis, stained with ethidium bromide and photographed on a Kodak Gel Logic 100 Imaging System Photodocumentator, and subsequently prepared for sequencing. Sequencing by the Sanger method was performed on the ABI3500 equipment (Applied Biosystems) and according to the manufacturer's instructions, at the Genomics Laboratory of Embrapa Agrobiologia (Seropédica, Rio de Janeiro, Brazil). The accession codes of sequences deposited in GenBank are MK 139712–MK 139736 and MK 159711 for 16S rRNA, MK 142239–MK 142264 and MK 174943 for recA, MK 174922–MK 174942 for nifH and MN 019631–MN 019649 for nodC.
Sequence processing, alignment and phylogenetic analysis
After sequencing, the leader and complementary strands of each gene were assembled in contigs with the ChromasPro program (Technelysium).
The search for 16S rRNA sequences of high similarity was carried out with the seqmatch tool made available by the Ribosomal Database Project (Cole et al. 2007). The sequences were aligned with the Infernal aligner (Nawrocki and Eddy 2013) made available by the same platform. recA, nifH and nodC sequences were submitted to the BLASTn algorithm (Altschul et al. 1990) with the Nucleotide collection (nr/nt) to search for sequences of high similarity in the NCBI GenBank® public database. The default BLASTn parameters were used. High identity sequences in the database were selected to serve as reference sequences. The corresponding recA, nifH and nodC protein sequences were aligned with the software MUSCLE (Edgar 2004) within the software MEGA6 (Tamura et al. 2013).
Maximum-likelihood trees of each gene and the concatenated alignments of 16S rRNA and recA were generated with the same software. The best substitution models used for the calculation of distances were the Tamura 3-parameter (1992) for recA and nifH, Tamura 3-parameter (1992) with G for nodC, Tamura–Nei (1993) for the 16S rRNA gene and Tamura–Nei with G + I for the concatenated alignment. The statistical support for different trees was calculated by bootstrap analysis (Swofford et al. 1996). A thousand replicates were used.
Evaluation of symbiotic effectiveness
A completely randomized design experiment, with 31 treatments and 4 replicates per treatment, was carried out to evaluate the symbiotic effectiveness of selected isolates. The treatments were 29 isolates, an uninoculated control and a control that received 5 mg per jar of N in the form of ammonium nitrate weekly.
Pregerminated seeds (as described earlier) were seeded in Leonard jars containing sterile 2:1 (v: v) sand and vermiculite and sterile nutrient solution (Gruzman and Döbereiner 1968). The plants were collected after 3 months, and their total dry mass (shoot, roots and nodules) was evaluated. The data were analyzed with an analysis of variance followed by the Scott–Knott's test (5% probability) (Scott and Knott 1974). The analyses were performed with the easyanova package (Arnhold 2013) in the statistical software R (R Core Team 2018).
The symbiotic effectiveness of each strain was calculated with the following formula: [dry weight of inoculated treatment/dry weight of uninoculated control] × 100.
RESULTS
Soil characterization
Soil pH was above 6 in the soils sampled in the Northeast region, while pH ranged between 4.8 and 5.7 in soils from the Southeast region (Table 2). Al concentration was higher in the Southeast in agreement with the lower pH observed in these soils. P concentration was high only in Juazeiro (Northeast). Soils from the Northeast also had the highest Ca and Mg concentrations; except for Seropédica, the concentration of these two nutrients can be considered satisfactory from an agricultural point of view in soils from the Southeast.
BOX-PCR analysis
Ninety-five isolates were reinoculated on M. caesalpiniifolia. From these, 39 induced nodulation and were analyzed by BOX-PCR fingerprinting. They were divided into five clusters at the level of 30% similarity based on their genotypic profiles (Fig. 2). Generally, these clusters match the origin of the isolates. Cluster 1 is formed only by bacteria isolated from the Southeast region. Cluster 2 is predominantly composed of bacteria isolated from the Northeast region, and within it, there is a subgroup formed by type and reference strains (codes with BR) and isolate PM2-1 from the Southeast region. Cluster 3 has a mixed composition, formed by two bacteria from the Southeast region (13P1 and 9P4) and two from the Northeast region (09-RN and 11-RN), which are the genotypic profiles that are most different from the others. Cluster 4 contains just one isolate, 19AP3, from the Southeast and cluster 5, the outermost cluster, contains only isolates from Rio Grande do Norte in the Northeast (Fig. 2).
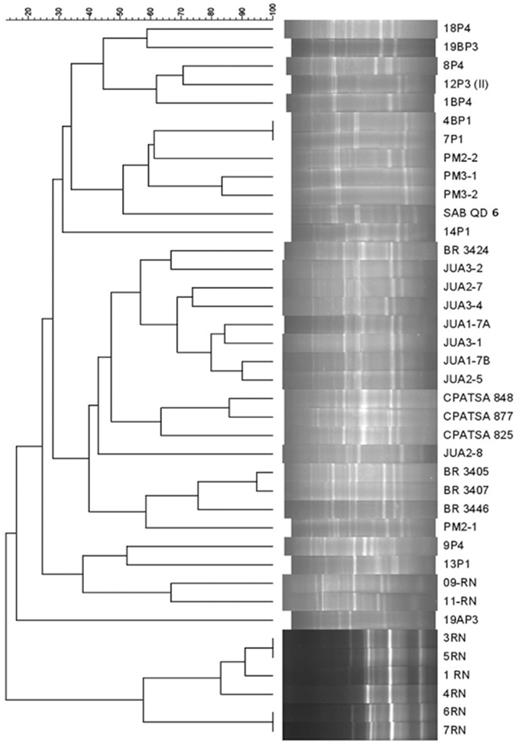
Dendrogram of similarity based on BOX-PCR profiles of 39 rhizobia isolated from nodules of M. caesalpiniifolia Benth. from the Northeast and Southeast regions of Brazil. The dendrogram was built with the Dice index and the UPGMA method. The codes of isolates according to their origin are SAB and P1 (Seropédica, Rio de Janeiro), P3 (Itaguaí, Rio de Janeiro), PM and P4 (Mangaratiba, Rio de Janeiro) in the Southeast, and JUA (Juazeiro, Bahia), CPATSA (Petrolina, Pernambuco) and RN (Natal, Rio Grande do Norte) in the Northeast.
Phylogenies of core genes (16S rRNA and recA)
The phylogenetic trees built with 16S rRNA and recA gene sequences are very similar (Figs S2 and S3, Supporting Information). For this reason, the alignments of these two genes were concatenated and used to build a single phylogeny (Fig. 3 and Fig. S1, Supporting Information). The concatenated phylogenetic tree divides the isolates into two large groups that represent the division of the isolates into β-Proteobacteria and α-Proteobacteria. Within β-Proteobacteria, there are three subgroups that will be named after clades I, II and III.
![Phylogenetic tree based on the concatenated alignment of 16S rRNA and recA genes of rhizobia isolated from nodules of M. caesalpiniifolia Benth. from the Northeast and Southeast regions of Brazil. A maximum-likelihood method with 1000 bootstrap replicates was used to build this tree. The substitution model was the Tamura 3-parameter (1992). The scale bar represents changes per nucleotide. Purple circles represent bootstrap values and the diameter of the circle is proportional to calculated value. Blue circles and yellow squares show the origin of the isolates. The codes of isolates according to their origin are SAB and P1 (Seropédica, Rio de Janeiro), P3 (Itaguaí, Rio de Janeiro), PM and P4 (Mangaratiba, Rio de Janeiro) in the Southeast, and JUA (Juazeiro, Bahia), CPATSA (Petrolina, Pernambuco) and RN (Natal, Rio Grande do Norte) in the Northeast. The bar plot displays the biomass accumulation of inoculated plants, and the percentages indicate their symbiotic effectiveness: [dry weight of inoculated treatment/dry weight of uninoculated control] × 100. Means (three replicates) with different letters show that the treatments differ by the Scott–Knott's test (5% probability).](https://oup.silverchair-cdn.com/oup/backfile/Content_public/Journal/femsec/95/8/10.1093_femsec_fiz109/1/m_fiz109fig3.jpeg?Expires=1749965175&Signature=45F6CociMqzoGwJniY9ZPDYd4aKgZGRc-ncpVwKICzMjLGjAqRjDfzl-JsbT-nhNJYjb-QHw2yUenc-g0TTrtO95XUO~QuxPs~M5MGbgOBjP0ejvwVt2iDdqUsVrjhbIYS~Jy8kiH3liczT8d~bUZZOBHeYOkiWo1~VtP4NuxRt4Ov08zgmAd2Fe7nHI8mF4-sSx1uGBw0~igVLMGOKzwjw-KA6HK1LUbPNF61~x69uHMj1tIXDiBBhOoFD4aatnxjsfa5qJohcJuK4HojgsARJqwoNYbLOBhpAez6H0rr1J0c~IXgzUsmoEa-8ogEu3VP1MwKn8OvJ4L6zGx2Oh0A__&Key-Pair-Id=APKAIE5G5CRDK6RD3PGA)
Phylogenetic tree based on the concatenated alignment of 16S rRNA and recA genes of rhizobia isolated from nodules of M. caesalpiniifolia Benth. from the Northeast and Southeast regions of Brazil. A maximum-likelihood method with 1000 bootstrap replicates was used to build this tree. The substitution model was the Tamura 3-parameter (1992). The scale bar represents changes per nucleotide. Purple circles represent bootstrap values and the diameter of the circle is proportional to calculated value. Blue circles and yellow squares show the origin of the isolates. The codes of isolates according to their origin are SAB and P1 (Seropédica, Rio de Janeiro), P3 (Itaguaí, Rio de Janeiro), PM and P4 (Mangaratiba, Rio de Janeiro) in the Southeast, and JUA (Juazeiro, Bahia), CPATSA (Petrolina, Pernambuco) and RN (Natal, Rio Grande do Norte) in the Northeast. The bar plot displays the biomass accumulation of inoculated plants, and the percentages indicate their symbiotic effectiveness: [dry weight of inoculated treatment/dry weight of uninoculated control] × 100. Means (three replicates) with different letters show that the treatments differ by the Scott–Knott's test (5% probability).
Clade I is composed of bacteria isolated from municipalities in the Southeast region of Brazil (Seropédica, Itaguaí and Mangaratiba), with codes PM, P, BP and SAB QD6. All of them were grouped with BR 3407T, the type strain of P. sabiae, which is also originally from Southeast Brazil (Chen et al. 2005b), with a bootstrap support of 99%. Isolate PM3-1 was grouped with Paraburkholderia phymatum in the 16S rRNA gene tree (Fig. S2, Supporting Information), with low support of 42%, and with P. sabiae, in the recA gene tree (Fig. S3, Supporting Information), with support of 99%. It was included with P. sabiae in the tree based on the concatenated alignments.
Clade II is predominantly composed of bacteria isolated from Northeast Brazil identified by codes containing JUA (for Juazeiro, Bahia), CPATSA (for Fortaleza, Ceará) and RN (for Natal, Rio Grande do Norte). This clade contains strains of Paraburkholderia diazotrophica, including the type strain of this species, with bootstrap values ranging from 60% to 95%. Within this group, there is also a single isolate from the Southeast region, 14P1, more specifically from Seropédica, Rio de Janeiro. Clade III is formed only by isolate 11-RN, which was grouped with the Trinickia symbiotica type strain (Sheu et al. 2012; Estrada-de los Santos et al. 2018), with a bootstrap value of 99%.
Clade IV is formed by three bacteria isolated from municipalities in the Southeast region, which were identified as Rhizobium (α-Proteobacteria). This cluster also contains type strains of the species Rhizobium mongolense, Rhizobium multihospitium, Rhizobiumfreirei, Rhizobiumtropici and Rhizobium hainanense, with a bootstrap value of 100%. Strain 13P1 was grouped with the type strain of R. hainanense, with a bootstrap value of 79%.
Phylogenies of symbiotic genes (nifH and nodC)
The phylogenies based on sequences of the nifH (Fig. 4) and nodC (Fig. 5) genes include only strains of β-Proteobacteria since the attempts to amplify this gene for Rhizobium (13P1, 19AP3 and 9P4) were not successful. Besides, the nifH sequences of strains PM2-1 and 4BP1 were not included due to their poor quality. The nodC gene could not be amplified for strains 11-RN, CPATSA 848 and BR 3424. The strains were subdivided into two and three clades in the nodC and nifH phylogenies, respectively.

Phylogenetic tree based on sequences of the nifH gene of rhizobia isolated from nodules of M. caesalpiniifolia Benth. from the Northeast and Southeast regions of Brazil. A maximum-likelihood method with 1000 bootstrap replicates was used to build this tree. The substitution model was the Tamura–Nei (1993). The scale bar represents changes per nucleotide. Purple circles represent bootstrap values and the diameter of the circle is proportional to calculated value. Blue circles and yellow squares show the origin of the isolates. The codes of isolates according to their origin are SAB and P1 (Seropédica, Rio de Janeiro), P3 (Itaguaí, Rio de Janeiro), PM and P4 (Mangaratiba, Rio de Janeiro) in the Southeast, and JUA (Juazeiro, Bahia), CPATSA (Petrolina, Pernambuco) and RN (Natal, Rio Grande do Norte) in the Northeast.
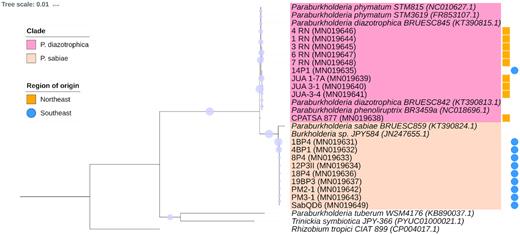
Phylogenetic tree based on sequences of the nodC gene of rhizobia isolated from nodules of M. caesalpiniifolia Benth. from the Northeast and Southeast regions of Brazil. A maximum-likelihood method with 1000 bootstrap replicates was used to build this tree. The substitution model was the Tamura 3-parameter (1992). The scale bar represents changes per nucleotide. Purple circles represent bootstrap values and the diameter of the circle is proportional to calculated value. Blue circles and yellow squares show the origin of the isolates. The codes of isolates according to their origin are SAB and P1 (Seropédica, Rio de Janeiro), P3 (Itaguaí, Rio de Janeiro), PM and P4 (Mangaratiba, Rio de Janeiro) in the Southeast, and JUA (Juazeiro, Bahia), CPATSA (Petrolina, Pernambuco) and RN (Natal, Rio Grande do Norte) in the Northeast.
For nifH (Fig. 4), clade I was formed by isolates from the Northeast region (with codes JUA, CPATSA and RN), isolate 14P1 from the Southeast and strains of P. phymatum, P. phenoliruptrix and the type strain of P. diazotrophica, with bootstrap support between 62% and 100%. Clade II was formed by isolates of the Southeast region (18P4, 8P4, 1BP4, BR 3407T, PM3-1, 12P3II, 19BP3 and SAB QD6) and the type strain of P. sabiae, with bootstrap support varying between 44% and 99%. Clade III was formed by the isolate 11-RN and a strain of T. symbiotica with support of 99%.
For nodC (Fig. 5), two main clades were formed, and their composition corresponded to those of clades I and II in nifH phylogeny. Clade I was formed by isolates from the Northeast region, isolate 14P1 from the Southeast and the type strains of P. phymatum, P. phenoliruptrix and P. diazotrophica, with a bootstrap support of 71%. Clade II was formed by isolates of the Southeast region and the type strain of P. sabiae, with bootstrap support of 99%.
Symbiotic effectiveness
Strains 14P1, 3-RN, JUA3-1, 6-RN, JUA-7A, 7-RN, 5-RN, 1-RN, 4-RN and JUA3-4 promoted a biomass accumulation ranging from 2437 to 3986 mg upon inoculation on M. caesalpiniifolia (Fig. 3). These biomasses were not statistically different from the nitrogen control, which received a total of 65 mg of nitrogen in the form of ammonium nitrate for 3 months. These isolates are all from the Northeast region of Brazil, with the exception of 14P1, which was isolated from Seropédica, Rio de Janeiro. They belong to P. diazotrophica, indicating that this species is the most efficient in fixing nitrogen in comparison to the others tested in greenhouse.
Strains 4BP1, JUA2-8, 1BP4, 12P3II, BR 3407T and 19BP3 did not differ among themselves and showed an intermediate performance (Fig. 3). All these bacteria were identified as P. sabiae and were from the Southeast region of Brazil, except for JUA2-8, which is a P. diazotrophica that was isolated of soil sampled in Juazeiro, Bahia.
Strains 11-RN, 18P4 and PM3-1 showed the worst performance among all tested strains. Nodulation was not observed with SAB QD6, CPATSA 848, 13P1, 9P4, 19AP3, PM2-1 and BR 3424 despite the fact that these strains were able to induce nodulation on M. caesalpiniifolia in the authentication experiment (Table S3, Supporting Information). Strain CPATSA 877 was not included in this analysis.
DISCUSSION
In the present study, we studied the biogeography of rhizobial symbionts of M. caesalpiniifolia from the ‘Caatinga’ biome in the Northeast of Brazil, where this species is native, and from the Atlantic Forest in the Southeast, where it was introduced. We aimed at unveiling the relationship between the distribution of these symbionts and the successful introduction of this nitrogen-fixing legume in other biomes.
The symbiotic character is considered to be generalized in Mimosa spp., and in Brazil, Paraburkholderia predominates as the rhizobial symbiont in the two major centers of diversification of this genus, the ‘Cerrado’ and ‘Caatinga’ biomes (Bontemps et al. 2010; dos Reis et al. 2010). We noticed the almost exclusive presence of P. diazotrophica in the Northeast and of P. sabiae in the Southeast. The classification of our strains into these two species was supported by core and symbiotic genes, pointing to the ability of M. caesalpiniifolia to nodulate with rhizobial populations outside of its native environment. In fact, P. sabiae was reported as one of the major species of Paraburkholderia in soils from the Atlantic Forest (Dall'Agnol et al. 2017).
Even though we did not find P. sabiae in our Northeast sites, this species was previously isolated from M. caesalpiniifolia in this region by Martins et al. (2015). This study was restricted to sites within a range of 300 km in the state of Pernambuco. Among their isolates, 9 out of 23 formed a clade with P. sabiae, and from these 9, 6 were from the same location. The other 14 isolates were grouped with P. diazotrophica and were isolated from several other locations pointing to a predominance and a broader geographical distribution of P. diazotrophica as well. This may indicate that even though P. sabiae is present in ‘Caatinga’, for some unknown reason M. caesalpiniifolia is nodulating preferentially with P. diazotrophica in this biome. Some authors point to soil pH and fertility as factors affecting the distribution and nodulation of legumes by different rhizobia (Pires et al. 2017; Dall'Agnol et al. 2017), and this may be a possible explanation to the predominance of P. diazotrophica as will be discussed further. Silva et al. (2018) also found P. diazotrophica and P. sabiae in the Northeast region of Brazil, in the state of Bahia, within a range of about 500 km, but these bacteria were nodulating species of Calliandra rather than M. caesalpiniifolia. Two P. diazotrophica and six P. sabiae isolates were recovered; these were restricted, respectively, to Calliandra depauperata in a specific location of the ‘Caatinga’ and Calliandra leutzelburgii in a transitional region between rupestrine and grassland vegetations.
Another evidence of the adaptation of M. caesalpiniifolia to native rhizobial populations is the isolation of Rhizobium from some of the nodules. The Rhizobium strains that we isolated were able to nodulate this legume in the authentication experiment; however, their symbiotic effectiveness could not be assessed since, for an unknown reason, the plants did not nodulate in the experiment aiming to evaluate this character. Also, we were not able to amplify the nifH and nodC genes, so the confirmation that M. caesalpiniifolia is nodulating with Rhizobium is inconclusive. There are no reports of nodulation of M. caesalpiniifolia with Rhizobium in the Brazilian semi-arid region, indicating a ‘symbiotic plasticity’ of M. caesalpiniifolia that favors its adaptation to new environments. Nevertheless, reports of nodulation with Rhizobium in the ‘Caatinga’ and ‘Cerrado’ were made for Mimosa xanthocentra Mart. (Bontemps et al. 2010; dos Reis et al. 2010) and indicated the preference of this species for these symbionts in soils where it is native. According to Bournaud et al. (2013), the ability of legumes to form a symbiosis with Paraburkholderia is not antagonistic to the presence of α-rhizobia, since they also found the two types of symbionts in six species of the Piptadenia group. Additionally, the genus Mimosa is also able to nodulate with Rhizobium in Central Mexico, and this was attributed to the geographical separation between Mexico and Brazil, and factors such as differences in soil fertility (Bontemps et al. 2016).
Some studies reported soil fertility and pH as major determinants of the distribution of symbionts of Mimosa. Pires et al. (2017) compared the predominance of α- and β-rhizobia in soils with different attributes and found out that Mimosa nodulates mainly with Paraburkholderia in soils with low fertility and low pH (4.2 and 4.3). On the other hand, Rhizobium was the primary symbiont in soil characterized as fertile and with pH close to neutral (6.3). A single strain of Paraburkholderia was identified in the most neutral and fertile soil. Other studies corroborate the idea that the genus Rhizobium prefers less acidic and more fertile soils (Baraúna et al. 2016; Bontemps et al. 2016).
Nevertheless, Dall'Agnol et al. (2017) isolated P. sabiae and Cupriavidus spp., both β-rhizobia, from soils with a pH close to neutrality (pH = 6.8) in the Atlantic Forest of Rio de Janeiro. P. sabiae was isolated from Phaseolus vulgaris (soil pH = 6.4) and Mimosa pudica (soil pH = 5.1), demonstrating the ability of this symbiont to adapt to other hosts and different soil conditions. Still, Mesorhizobium plurifarum was the most abundant symbiont in these soils.
In the present work, soils from the Northeast region, from where P. diazotrophica was isolated in greater number, were close to neutrality and showed higher fertility than soils from the Southeast, from where P. sabiae was the most frequently isolated species. pH in these soils was above 6, while pH in the Southeast ranged between 4.8 and 5.7. It is also important to note that, in the present study, α-rhizobia were also isolated from the Southeast, even though with a lower frequency. Although it seems that a distribution pattern linked to soil pH exists, our and other studies such as that of Pires et al. (2017) lack replication to test the hypothesis of the link between pH and soil fertility and rhizobial diversity. Dall'Agnol et al. (2017) were able to test this hypothesis with a canonical analysis; however, only six soils trapped with two legume species were examined. Based on these findings, it can be said that there is a need to study more deeply the relationship between soil attributes and the distribution of rhizobial species.
Bacteria classified as P. diazotrophica in the 16S rRNA and recA phylogenies were grouped with P. phymatum, P. diazotrophica and P. phenoliruptrix in the nifH and nodC phylogenies. In addition, a more external group contained a strain of Burkholderia vietnamiensis, which is part of the Burkholderia cepacia complex considered as pathogenic (Estrada-de los Santos, Bustillos-Cristales and Caballero-Mellado 2001), and a nitrogen-fixing bacterium found in the rhizospheres of rice, maize and coffee (Gillis et al. 1995; Estrada-de los Santos, Bustillos-Cristales and Caballero-Mellado 2001). It should be noted that P. phymatum was evolutionarily closer to P. sabiae than to P. diazotrophica in the trees of the concatenated genes but closer to P. diazotrophica in the nifH gene tree, which may indicate a horizontal gene transfer between these two species. Bontemps et al. (2010) also identified exceptions in the phylogeny of nifH genes, but they claimed that they were not sufficient to support events of horizontal gene transfer. They also reported that the generally high congruence of these molecular markers indicates a reasonably stable genetic structure with few transfers between the different species evaluated. Our data also support this high congruence as we found out that both nifH and nodC phylogenies agree with the phylogeny based on the core genes (16S rRNA and recA), supporting a vertical transmission of nifH and nodC genes within P. diazotrophica and P. sabiae. The consistent classification of our isolates into these two species raises evidence to the hypothesis that M. caesalpiniifolia adapted to P. sabiae populations in the Southeast. As already stated, M. caesalpiniifolia is able to nodulate with P. sabiae in its region of origin (Martins et al. 2015); however, the abundance of the P. sabiae associated with this species in the Southeast may indicate the better adaptation of this bacterium to these soils.
The highest biomass accumulation of M. caesalpiniifolia in response to inoculation was promoted by strains of the species P. diazotrophica, including the only isolate from the Atlantic Forest belonging to this species (isolate 14P1). This indicates that the symbiotic effectiveness of the bacterial strains may not be related to their origin, but their taxonomy. This is even more evident when one observes that 14P1 is one of the most effective isolates. Although it is an exception among the bacteria from the Atlantic Forest, this isolate proved to be more efficient than P. sabiae isolated from the same soils. The consequences of the lower effectiveness of P. sabiae on the performance of M. caesalpiniifolia in its new environment remain to be determined. Although less effective than P. diazotrophica, P. sabiae may have an adaptive advantage over the other species in the conditions found in the soils of the Atlantic Forest. The variation of the symbiotic effectiveness has been known for a long time (Moreira, Nobrega and de Carvalho 2010) and the results obtained herein highlight the importance not only of the process of taxonomic identification and classification but also of selection to ensure that efficient and competitive strains are used, for example, as inoculants in environmental restoration programs.
CONCLUSIONS
M. caesalpiniifolia nodulated mainly with P. diazotrophica in soils of the ‘Caatinga’ biome, where it is native, and mainly with P. sabiae in soils of the Atlantic Forest, outside its region of origin. Additionally, M. caesalpiniifolia has the ability to associate with α-rhizobia in soils of the Atlantic Forest. These observations denote the ability of this legume species to adapt to different symbionts. Our data indicate that the symbiotic effectiveness of our strains is primarily related to their taxonomic classification rather than to their origin.
ACKNOWLEDGEMENTS
The authors thank the Brazilian National Council for Scientific and Technological Development (CNPq) and the Carlos Chagas Filho Foundation for the Support of Research in the State of Rio de Janeiro (FAPERJ) for their financial support (projects 475168/2012-7 and E-26/111.683/2012, respectively); the Coordenação de Aperfeiçoamento de Pessoal de Nível Superior (CAPES) for the scholarships provided to Isabelly Santos Rosado de Oliveira, Thiago Gonçalves Ribeiro and Maura Santos Reis de Andrade da Silva; and CNPq for the fellowship provided to Ederson da Conceição Jesus (project 475168/2012-7). The authors also thank Felipe Ferreira da Silva for providing nodules for the isolation of rhizobia, Fernando Cunha and Marcelo Antoniol Fontes for their assistance with the Leonard jar essay, Roberto Carlos da Silva Ramos for helping to collect the experiments, Cândido Barreto de Novais for giving support with the edition of figures and Fernanda dos Santos Dourado for the support given with sequencing.
FUNDING
This work was supported by the Brazilian National Council for Scientific and Technological Development (project 475168/2012-7) and the Carlos Chagas Filho Foundation for the Support of Research in the State of Rio de Janeiro (project E-26/111.683/2012).
Conflict of interest. None declared.