-
PDF
- Split View
-
Views
-
Cite
Cite
Wietse de Boer, Xiaogang Li, Annelein Meisner, Paolina Garbeva, Pathogen suppression by microbial volatile organic compounds in soils, FEMS Microbiology Ecology, Volume 95, Issue 8, August 2019, fiz105, https://doi.org/10.1093/femsec/fiz105
- Share Icon Share
ABSTRACT
There is increasing evidence that microbial volatile organic compounds (mVOCs) play an important role in interactions between microbes in soils. In this minireview, we zoom in on the possible role of mVOCs in the suppression of plant-pathogenic soil fungi. In particular, we have screened the literature to see what the actual evidence is that mVOCs in soil atmospheres can contribute to pathogen suppression. Furthermore, we discuss biotic and abiotic factors that influence the production of suppressive mVOCs in soils. Since microbes producing mVOCs in soils are part of microbial communities, community ecological aspects such as diversity and assembly play an important role in the composition of produced mVOC blends. These aspects have not received much attention so far. In addition, the fluctuating abiotic conditions in soils, such as changing moisture contents, influence mVOC production and activity. The biotic and abiotic complexity of the soil environment hampers the extrapolation of the production and suppressing activity of mVOCs by microbial isolates on artificial growth media. Yet, several pathogen suppressive mVOCs produced by pure cultures do also occur in soil atmospheres. Therefore, an integration of lab and field studies on the production of mVOCs is needed to understand and predict the composition and dynamics of mVOCs in soil atmospheres. This knowledge, together with the knowledge of the chemistry and physical behaviour of mVOCs in soils, forms the basis for the development of sustainable management strategies to enhance the natural control of soil-borne pathogens with mVOCs. Possibilities for the mVOC-based control of soil-borne pathogens are discussed.
INTRODUCTION
The performance of plants in both natural and man-made terrestrial ecosystems is highly dependent on the functioning of soil microbial communities. This also holds for the ability of plants to deal with root-infecting pathogenic fungi. Perhaps the extensive use of fungicides in agriculture can give the impression that pathogens cannot be restrained without human–chemical intervention. Yet, in reality, there are several natural factors in soils that can counteract pathogen proliferation and infection. A better understanding of the underlying mechanisms of these pathogen-suppressing 'soil forces' is essential to exploit them and allow the transition to sustainable agriculture.
During the period of survival outside the host plants, pathogens reside in the soil and are part of the soil microbial community (Termorshuizen and Jeger 2008). Consequently, they are involved in interactions with other soil microbial inhabitants. A major type of interaction in soil microbial communities is competition for organic energy sources (Schimel and Schaeffer 2012). Competitive interactions can affect pathogens directly or indirectly. For instance, direct competition is occurring when pathogens and saprotrophic microbes compete for root exudates. Both exploitation competition (uptake/withdrawing of energy sources) and interference competition (production of inhibitors) can be involved (Garbeva et al. 2011). Indirect effects of competition can occur when pathogens are inhibited by secondary metabolites that are produced during interference-competition interactions between other members of the soil microbial community (de Boer 2017). Hence, the production of inhibiting compounds within soil microbial communities is not only affecting the competing microbes, but also other microbes, including plant pathogens. Therefore, the production of secondary metabolites in soils can be a major causal factor of suppression of soil-borne plant diseases (Kwak and Weller 2013; Cha et al. 2016; Schlatter et al. 2017).
Volatile organic compounds produced by soil microbes (mVOCs) form an interesting group of chemicals for below-ground interference competition as they can spread via air-filled pores in soils, thereby extending the range over which antagonistic activities can take place. More than 1300 mVOCs have been identified, and this number is continuously increasing (Lemfack et al. 2017; Piechulla et al. 2017). Major chemical classes of mVOCs are alcohols, ketones, aromatic compounds, terpenes, organic acids, esters, aldehydes, sulfur compounds, alkanes and nitrogen compounds (Schenkel et al. 2015; Schmidt et al. 2015). Since suppressive activities of mVOCs can act against plant pathogenic microorganisms, scientists have started to explore the possibility of using mVOCs for controlling root-infecting pathogens.
The extrapolation of the current knowledge of mVOCs towards the application in real soils is hampered by the fact that our knowledge of the production of pathogen-suppressive mVOCs is mostly based on studies using single strains growing on artificial nutrient-rich media (Effmert et al. 2012; Garbeva et al. 2014; de Vrieze et al. 2015; Piechulla et al. 2017). However, it is well known that medium composition has a strong impact on the spectrum of mVOCs produced as well as on the suppressive effects (Fiddaman and Rossall 1994; Garbeva et al. 2014; Lazazzara et al. 2017). Especially, studies using simple synthetic microbial communities do indicate that microbial interactions can strongly influence the production and activity of mVOCs (Tyc et al. 2017). VOC-mediated suppressive activities by soil microbial communities are thus not necessarily the same as those observed for model strains. Yet, VOCs are being produced by microbes in soils, and several of these mVOCs are known from lab incubations. In addition, there is evidence for pathogen-suppressing effects of mVOCs produced in soils (van Agtmaal et al. 2018). Hence, the intention to explore mVOCs as a new tool for biocontrol purposes has definitely a good basis (Bitas et al. 2013; Kanchiswamy et al. 2015a; Bailly and Weisskopf 2017).
The aim of the current paper is to give an overview of the current knowledge of pathogen-suppressive activities of mVOCs produced by soil microbial communities. In addition, we will indicate possibilities for management strategies to enhance the control of pathogens via the stimulation of the production of suppressive mVOCs by soil microbial communities. The microbial production of inorganic volatiles, like ammonia and hydrogen cyanide, which can be involved in pathogen suppression, is not dealt with in this review, but a recent update on that topic is given by Piechulla et al. (2017). In this paper, we will use the abbreviation mVOCs when it is likely that microbes are the main producers. For soil samples, we consider produced VOCs as mVOCs when root-derived VOCs can be excluded.
Evidence for the production of fungistatic and pathogen-suppressing mVOCs in soils
The germination and growth of fungi is often negatively affected by direct or indirect contact with soils, a phenomenon named soil fungistasis (Watson and Ford 1972; Garbeva et al. 2011). Since many root-infecting pathogenic fungi appear to be highly sensitive to soil fungistasis, a lot of research has been devoted to elucidate the mechanisms with the aim to develop new disease control strategies. Whereas abiotic soil factors can be involved, the major cause of fungistasis is microbiological as it is mostly strongly reduced after sterilization of soils. It is now generally agreed that intensive competition for nutrients within the soil microbial community is the main mechanism explaining soil fungistasis, and the production of inhibitors coinciding with competitive interactions can play a major role (Garbeva et al. 2011).
Chuankun et al. (2004) made a strong case for the involvement of mVOCs in soil fungistasis by showing a strong correlation between inhibition and germination of fungal spores that are in direct contact with soils and that of germination of spores exposed to mVOCs emitted from those soils. The emission of suppressive mVOCs was stopped after sterilization of soils. In their study, they did not use spores of plant pathogenic fungi but of nematode-parasitizing fungi. Therefore, their results indicate that mVOCs in soils may hamper the application of spores of parasitic fungi for biocontrol purposes.
Effects of soil mVOCs on plant pathogenic fungi were studied by Van Agtmaal et al. (2018). Two pathogenic fungi (Fusarium oxysporum and Rhizoctonia solani) and a plant pathogenic oomycete (Pythium intermedium) growing on nutrient-poor agar were exposed to mVOCs emitted from soil samples obtained from 50 agricultural fields. Most soil samples emitted growth-suppressing VOCs, but the extent of growth inhibition per soil differed strongly for the three pathogens. This variation can be ascribed to differences in sensitivity of fungal species for mVOCs (Garbeva et al. 2014) and to differences in the composition of mVOCs among soils (Chuankun et al. 2004; McNeal and Herbert 2009; Insam and Seewald 2010; Mancuso et al. 2015).
To obtain evidence for the involvement of soil mVOCs in plant disease suppression, only fungistasis tests are not sufficient as fungistasis may help prevent the germination and growth of pathogenic fungi under conditions that are not suitable to find a host plant (Garbeva et al. 2011). Hence, soil fungistasis is not necessarily negative for pathogens. Therefore, effects of soil mVOCs should be studied under conditions where both host plants and pathogen propagules are present.
Evidence for the possible contribution of soil-derived mVOCs to disease suppression was obtained in a study by van Agtmaal et al. (2015). In this study, a strong reduction of the suppressing effects of mVOCs emitted from soil on the in vitro growth of P. intermedium was found as a legacy of a severe soil disturbance (anaerobic soil disinfestation) that had been applied three months earlier. In a simultaneous measurement, it was shown that Hyacinth bulbs planted in these soils were highly sensitive to infection when this oomycete was added. One year later, 15 months after the anaerobic disinfestation treatment, suppressing effects of soil mVOCs on the in vitro growth of P. intermedium had returned to the level of untreated soils and this coincided with a decreased sensitivity of Hyacinth bulbs to diseases. Anaerobic soil disinfestations are applied to eliminate pathogens, but the study of van Agtmaal et al. (2015) shows that such a drastic measure can also reduce the mVOC-mediated suppressive activities of soil microbial communities. This can make the soil more conducive to diseases caused by pathogens that have survived the eliminating treatment or by novel pathogenic invaders.
Identity of fungistatic and pathogen-suppressing mVOCs produced in soils
The composition of fungistatic and pathogen-suppressing mVOC blends produced by microbial strains on artificial growth media has triggered investigations to determine the identity of the inhibiting compounds and the mechanisms of inhibition. The most common approach to elucidate the identity of fungistatic mVOCs is by reporting on directly examined or published antifungal effects of pure compounds that are present in suppressing mVOC blends (Fernando et al. 2005; Zou et al. 2007; Minerdi et al.2009; Effmert et al. 2012; Cordovez et al. 2015; de Vrieze et al. 2015; Raza et al. 2015; Kanchiswamy et al. 2015b; Piechulla et al. 2017; Rybakova et al. 2017). In addition, a comparison of mVOC blends of suppressive wild-type strains and those of nonsuppressive mutants can give indications on the identity of suppressive mVOCs (Ossowicki et al. 2017; Carrion et al. 2018)
Mechanisms of inhibiting effects of fungistatic compounds have mainly been examined with pure compounds. From these studies, it has become apparent that several mechanisms such as the disruption of cell membrane integrity or the malfunctioning of cellular antioxidation systems in pathogenic fungi are a common consequence of the exposure to mVOCs (Kim et al. 2011; Giorgio et al. 2015). A next step is to develop protocols to examine the impact of mVOC blends in the soil atmosphere on physiological responses of pathogens and other microbial soil inhabitants (Yuan et al. 2018).
The information on fungistatic mVOCs produced by pure cultures can be used to identify mVOCs that are causing soil fungistasis in the soil atmosphere (Penuelas et al. 2014). The developments on identification and (semi)quantification of VOCs that are present in soil atmospheres are making rapid progress (De Cesare et al. 2011; Penuelas et al. 2014; Veres et al. 2014; Mancuso et al. 2015; Garcia-Alcega et al. 2017; Bourtsoukidis et al. 2018; Rosabbi et al. 2018; Ruiz-Hernandez et al. 2018). Most studies focus on quantification of emission of soil VOCs to the atmosphere and not on the role of VOCs in the soil environment. However, relevant information on the presence of fungistatic mVOCs in soil atmospheres can be obtained by comparing data of these soil VOC emission screenings with a database of mVOCs (Lemfack et al. 2017). For example, benzaldehyde was found to be emitted from several agricultural soils (Zou et al. 2007; McNeal and Herbert 2009; Zhao et al. 2016), and this fungistatic compound can be produced by many soil bacterial species (Effmert et al. 2012).
Obviously, the best way to get information about the identity of soil mVOCs contributing to fungistasis and pathogen suppression in agricultural soils is by doing simultaneous measurements of VOC composition and mVOC-mediated fungal inhibition. This has been done only in a few cases. In the earlier mentioned study of Chuankun et al. (2004), a subset of pure VOCs (e.g. trimethylamine, dimethyl disulfide (DMDS), 3-methyl-2-pentanone, methyl pyrazine, 2,5-dimethyl-pyrazine, N,N-dimethyloctylamine and nonadecane) that were detected only in strong fungistatic soils were tested for antifungal activity. Of these compounds, the two amines had a strong inhibiting effect on fungal spore germination even at low doses. The same was found for benzaldehyde, although this compound was also present in low concentrations in nonfungistatic soils. Similarly, suppressing amines and benzaldehyde were detected in mVOC blends emitted from fungistatic agricultural soils (Zou et al. 2007). In addition, several alkanes, alcohols and ketones were detected in soils that exhibited mVOC suppression as well as disease suppression against Pythium but not in the soils that lost these suppressive activities after a disturbance (van Agtmaal et al. 2015). Three of these compounds (2-octanone, 2-nonanone and 2-undecanone) are known to have fungus-suppressing activity (Fernando et al. 2005; Zou et al. 2007; Weisskopf 2013).
The spectrum of mVOCs produced by cultivated soil microbes is huge (Lemfack et al. 2017), and several of these mVOCs were found to have biocidal or biostatic effects. The implication of this for soil microbial communities is that there can be functional redundancy for fungistasis and pathogen suppression as different mVOCs can result in a similar level of suppression (Hol et al. 2015). Another implication is that mVOCs produced by soil microbial communities can have broad-spectrum effects resulting not only in the inhibition of (pathogenic) fungi but also of other soil inhabitants, including plant roots. The latter has been described as general soil biostasis (Garbeva et al. 2011).
Impact of microbial diversity, composition and interactions on the production of fungistatic and pathogen-suppressing mVOCs
The mVOCs in soils that suppress soil-borne pathogens are produced within soil microbial communities. This implies that knowledge of the functioning of mVOCs in soil microbial communities is essential to understand the production of pathogen-suppressing mVOCs. However, so far, most studies on pathogen suppression by mVOCs have not exceeded the level of suppressing mVOC production in single-species cultivations. Studies on mVOCs that have included community ecological aspects, like population interactions, species diversity and assembly, are mainly restricted to bacterial VOC producers. The most important findings of these pioneer studies are discussed in this section and are outlined in Fig. 1.
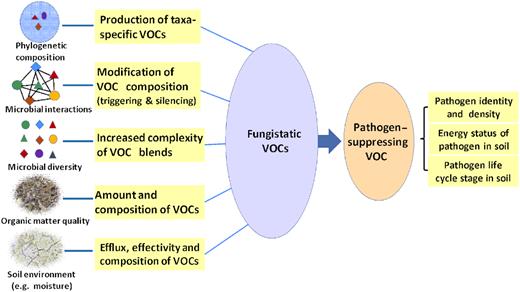
Soil microbial communities (interactions, diversity and composition) and abiotic factors (e.g. organic matter quality and moisture) determining the quantity and composition of fungistatic mVOCs and associated pathogen-suppressing mVOCs. The actual suppression is also dependent on the density and life-history characteristics of the pathogens.
The suppressing activity of mVOCs produced by bacterial mixtures can be different from that of mVOCs produced by single species. Co-culturing of bacterial species was shown to affect the composition and suppressing activities of mVOCs (Schulz-Bohm et al. 2015; Tyc et al. 2015, 2017; Kai et al. 2018). Simple synthetic bacterial communities of two to five species produced different mVOC blends than either of the single species that the communities were composed of. For example, co-culturing of two soil bacterial isolates (Burkholderia sp. and Paenibacillus sp.) resulted in a significant increase in the production of a pyrazine volatile by the Paenibacillus strain (Tyc et al. 2015). The pyrazine volatile was found to suppress the growth of the Burkholderia strain, pointing at the role of this compound in interference competition. Interestingly, pyrazine volatile compounds can have a broad spectrum of inhibiting activities, including the suppression of plant pathogenic fungi (Schmidt et al. 2015). In competitive interactions, mVOCs themselves may also act as signals to trigger the production of other mVOCs (Schmidt et al. 2017). Another possibility for the importance of interactions was given by Kai et al. (2018), who showed that structurally complex mVOCs could be produced by precursors formed by different bacteria. Taken together, these studies indicate that competitive interactions within soil bacterial communities can trigger the production of mVOCs that contribute to the suppression of pathogens. However, interactions can also result in a reduced production of suppressive mVOCs (Tyc et al. 2017).
It is important to consider the role of microbial diversity for the production of pathogen-suppressing mVOCs, but this has been addressed in only a few studies. For instance, Hol et al. (2015) measured the effect of species richness of cultivable soil bacterial communities on the production of pathogen-suppressive mVOCs. Differences in species richness were established by using inoculums from dilution series of filtered soil suspensions, and the bacteria were grown on nutrient-poor agar to resemble the energy-limiting conditions in soil. A consistent increase in the intensity of the mVOC suppression of the fungal pathogen (F. oxysporum) was seen with an increasing bacterial species richness. This could point at the importance of low-abundant bacteria for the production of suppressive mVOCs in bacterial communities. Volatiles with known antifungal effects that were mainly restricted to blends produced by species-rich communities were 2-methylfuran, 2-furaldehyde, 2-(methylthio)benzothiazole and muurolol. The absence of these mVOCs from blends produced by species-poor bacterial communities coincided with the loss of bacteria belonging to the genera Stenotrophomonas, Luteibacter, Dyella and Pedobacter. The possible importance of low-abundant bacteria in the composition and activity of mVOCs emitted by soil bacterial communities was also observed by Schulz-Bohm et al. (2015), who examined mVOC production and activity for small synthetic soil bacterial communities. Low-abundant bacteria might contribute directly, as mVOC producer, or indirectly, via triggering mVOC production by abundant bacteria, to a more complex blend of inhibiting mVOCs. In addition, synergistic suppressive effects of mVOCs present in a mixture of mVOCs have been reported (Cornelison et al. 2014, 2016).
When comparing emissions of pathogen-suppressing mVOCs of 50 agricultural soils with the bacterial diversity of those soils, no positive correlation was observed (van Agtmaal et al. 2018). In contrast, for Rhizoctonia solani, even a significant negative correlation was found for the suppressive effects of soil VOCs on fungal growth and the Shannon diversity index for soil bacteria. Hence, at this stage, it is not clear if the positive effects of diversity of manipulated soil bacterial communities on suppressive mVOC production do have a meaning for mVOC production by real soil bacterial communities. In the study by van Agtmaal et al. (2018), diversity was based on extracted soil DNA, which includes the DNA of inactive microbes. Hence, it would be interesting to examine whether differences in diversity-suppressive VOC relationships between soils and experiments still exist when only the active microbes in soils are considered.
The role of the phylogenetic composition of soil microbes has to be taken into account when addressing impacts of diversity. Different species have the potential to have specific contributions to the blend of mVOCs produced by microbial communities (Kai et al. 2009; Effmert et al. 2012). For example, different mVOC profiles are produced by different bacterial and fungal strains when cultivated on similar agar media (Insam and Seewald 2010; Penuelas et al. 2014). In addition, the phylogenetic composition of soil microbial communities is indicated as a major factor determining the production of pathogen-suppressing mVOCs in real soils or in communities extracted from real soils (van Agtmaal et al. 2015, 2018; Hol et al. 2015; Schulz-Bohm et al. 2015). Furthermore, changes in the composition of mVOCs emitted from Salix litter were found to coincide with changes in the composition of litter-degrading bacterial communities (Svendsen et al. 2018).
Environmental conditions and mVOCs
Abiotic conditions of soils can have a major impact on the quantity, composition and functioning of mVOCs (Fig. 1). In particular, temperature, pH, moisture content and soil texture were shown to influence the rate and composition of produced (m)VOCs (Bastos and Magan 2007; Insam and Seewald 2010; Gray et al. 2014; Wang et al. 2015; Zhao et al. 2016; Potard et al. 2017; Raza et al. 2017; Som, Willett and Alborn 2017; Kramshøj et al. 2019). In most cases, studies on effects of abiotic soil factors were done to estimate the consequences of climate-related changes (temperature and moisture) on the efflux of VOCs from the soil into the atmosphere. For instance, Raza et al. (2017) reported that the emission of VOCs for an agricultural soil was higher in a soil with a decreased moisture content and an increased soil temperature. Strong fluctuations of soil moisture may be of particular interest, as this will coincide with fluctuations in the availability of oxygen for microbes (Tecon and Or 2017). Anaerobic conditions result in shifts of the abundance of populations within the microbial community and in an altered production of mVOCs (Seewald et al. 2010; Redeker et al. 2018). The composition of mVOCs produced by non-saturated soils that have previously been exposed to anaerobic conditions can remain present for a considerable time (van Agtmaal et al. 2015). Such legacy effects of temporary anaerobiosis on mVOC composition can be due to changes within the microbial community or to an ongoing increased presence of anaerobic microsites (Seewald et al. 2010; van Agtmaal et al. 2015; Tecon and Or 2017; Redeker et al. 2018). Since climate change is expected to coincide with extreme rainfall events in many geographic areas, a more frequent occurrence of temporary anaerobiosis events may take place. For arable soils, this may have serious consequences for the intensity of mVOC-mediated suppression of plant pathogens (Meisner and de Boer 2018).
Next to abiotic conditions, the availability and quality of organic energy sources have a strong impact on the amount and composition of mVOCs produced in soils. Organic matter quality affects the composition of VOCs emitted from soils (Gray et al. 2010; Svendsen et al. 2018). For example, VOCs emitted from litter have higher emission rates and a higher diversity than VOCs emitted from mineral soils, due to differences in organic carbon quality and microbial biomass (Leff and Fierer 2008).
For arable soils, the impact of organic amendments on the amount and composition of emitted mVOCs has been examined in several studies (Potard et al. 2017; Abis et al. 2018; Zhang et al. 2018). The purpose of most of these studies is to determine the effects of organic amendments on VOC fluxes from the soil into the atmosphere and the potential impact thereof for weather and climate development, an area that is underexplored (Penuelas et al. 2014; Brenzinger et al. 2018). Therefore, a lot of attention is being paid to the effect of organic amendments on the emission of greenhouse gases (Ho et al. 2015, 2017). Yet, when the whole spectrum of emitted VOCs is determined, these studies do also reveal important information on the stimulation of potential fungistatic or plant-growth promoting mVOCs by different organic amendments (Raza et al. 2017). As will be discussed in the next section, such information can also be used to design criteria for applying organic amendments that stimulate the mVOC-mediated suppression of soil-borne plant diseases.
Management options for using pathogen-suppressing mVOCs in soil
mVOCs may be important as novel weapons in the control of soil-borne pathogens (Kanchiswamy et al. 2015a; Chung et al. 2016). An overview of possibilities of applying mVOCs in agricultural settings is given in Fig. 2. One of the options is to chemically produce mVOCs with pathogen-suppressing activity and use these as fumigants by injection or dripping into the soil (Wilson et al. 1999; Choi et al. 2014; Chung et al. 2016; Gómez-Tenorio et al. 2015; Badawy, Taktak and El-Aswad 2018). The screening of mVOCs that can be used as safe fumigants has strongly increased after the use of known chemical fumigants, like methyl bromide, was banned because of negative impacts on the environment and human health (Gerik 2005). Many of the mVOCs that are tested as fumigants are commonly found in the soil atmosphere or are produced by soil bacterial communities. Examples are given in Table 1.
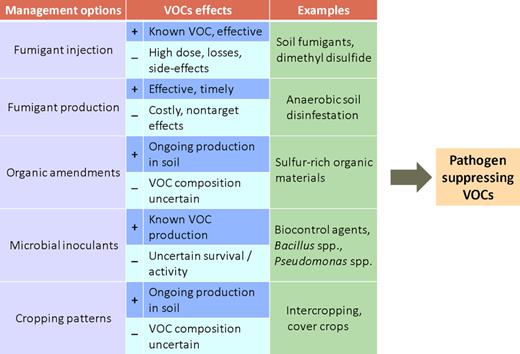
Different management options to use pathogen-suppressing mVOCs for disease control in agriculture.
Examples of existing/potential fumigant VOCs produced by cultivated soil microbes and detected in arable soil atmospheres.
Volatile . | Target pest organism . | Occurring in arable soil atmosphere . | Single-strain producers* . | Possibility for in situ stimulation . |
---|---|---|---|---|
Dimethyldisulfide | Nematodes, pathogenic fungi (Gómez-Tenorio et al. 2015; Papazlatani et al. 2016) | Yes (Chuankun et al. 2004; Zou et al. 2007; Veres et al. 2014; Zhao et al. 2016) | Many bacteria (Bacilli, Gammaproteobacteria, Actinomycetes),several fungi | Sulfur-rich plant residues and manure (Wang et al. 2009; Arnault et al. 2013; Raza et al. 2017) |
Benzaldehyde | Pathogenic fungi and oomycetes (Wilson et al. 1999) | Yes (Chuankun et al. 2004; Zou et al. 2007; Zhao et al. 2016) | Many bacteria (Bacilli, Gammaproteobacteria), few fungi | Incorporation of plant materials containing precursors (Guo et al. 1998; Martens 2002) |
Furfural | Nematodes, pathogeni fungi and oomycetes (Gerik 2005; Crow and Luc 2014) | Yes (Leff and Fierer 2008) | Few bacteria, several fungi | May be stimulated via materials rich in hemi-cellulose (Eseyin and Steele 2015). |
2-Undecanone | Nematodes, insects, pathogenic fungi and oomycetes (Kai et al. 2009; Ntalli et al. 2016) | Yes (Zou et al. 2007; van Agtmaal et al. 2015; Zhao et al. 2016) | Many bacteria (Bacilli, Gammaproteobacteria, Actinomycetes), few fungi | Intercropping with onions (Antonious 2013) |
Volatile . | Target pest organism . | Occurring in arable soil atmosphere . | Single-strain producers* . | Possibility for in situ stimulation . |
---|---|---|---|---|
Dimethyldisulfide | Nematodes, pathogenic fungi (Gómez-Tenorio et al. 2015; Papazlatani et al. 2016) | Yes (Chuankun et al. 2004; Zou et al. 2007; Veres et al. 2014; Zhao et al. 2016) | Many bacteria (Bacilli, Gammaproteobacteria, Actinomycetes),several fungi | Sulfur-rich plant residues and manure (Wang et al. 2009; Arnault et al. 2013; Raza et al. 2017) |
Benzaldehyde | Pathogenic fungi and oomycetes (Wilson et al. 1999) | Yes (Chuankun et al. 2004; Zou et al. 2007; Zhao et al. 2016) | Many bacteria (Bacilli, Gammaproteobacteria), few fungi | Incorporation of plant materials containing precursors (Guo et al. 1998; Martens 2002) |
Furfural | Nematodes, pathogeni fungi and oomycetes (Gerik 2005; Crow and Luc 2014) | Yes (Leff and Fierer 2008) | Few bacteria, several fungi | May be stimulated via materials rich in hemi-cellulose (Eseyin and Steele 2015). |
2-Undecanone | Nematodes, insects, pathogenic fungi and oomycetes (Kai et al. 2009; Ntalli et al. 2016) | Yes (Zou et al. 2007; van Agtmaal et al. 2015; Zhao et al. 2016) | Many bacteria (Bacilli, Gammaproteobacteria, Actinomycetes), few fungi | Intercropping with onions (Antonious 2013) |
Examples of existing/potential fumigant VOCs produced by cultivated soil microbes and detected in arable soil atmospheres.
Volatile . | Target pest organism . | Occurring in arable soil atmosphere . | Single-strain producers* . | Possibility for in situ stimulation . |
---|---|---|---|---|
Dimethyldisulfide | Nematodes, pathogenic fungi (Gómez-Tenorio et al. 2015; Papazlatani et al. 2016) | Yes (Chuankun et al. 2004; Zou et al. 2007; Veres et al. 2014; Zhao et al. 2016) | Many bacteria (Bacilli, Gammaproteobacteria, Actinomycetes),several fungi | Sulfur-rich plant residues and manure (Wang et al. 2009; Arnault et al. 2013; Raza et al. 2017) |
Benzaldehyde | Pathogenic fungi and oomycetes (Wilson et al. 1999) | Yes (Chuankun et al. 2004; Zou et al. 2007; Zhao et al. 2016) | Many bacteria (Bacilli, Gammaproteobacteria), few fungi | Incorporation of plant materials containing precursors (Guo et al. 1998; Martens 2002) |
Furfural | Nematodes, pathogeni fungi and oomycetes (Gerik 2005; Crow and Luc 2014) | Yes (Leff and Fierer 2008) | Few bacteria, several fungi | May be stimulated via materials rich in hemi-cellulose (Eseyin and Steele 2015). |
2-Undecanone | Nematodes, insects, pathogenic fungi and oomycetes (Kai et al. 2009; Ntalli et al. 2016) | Yes (Zou et al. 2007; van Agtmaal et al. 2015; Zhao et al. 2016) | Many bacteria (Bacilli, Gammaproteobacteria, Actinomycetes), few fungi | Intercropping with onions (Antonious 2013) |
Volatile . | Target pest organism . | Occurring in arable soil atmosphere . | Single-strain producers* . | Possibility for in situ stimulation . |
---|---|---|---|---|
Dimethyldisulfide | Nematodes, pathogenic fungi (Gómez-Tenorio et al. 2015; Papazlatani et al. 2016) | Yes (Chuankun et al. 2004; Zou et al. 2007; Veres et al. 2014; Zhao et al. 2016) | Many bacteria (Bacilli, Gammaproteobacteria, Actinomycetes),several fungi | Sulfur-rich plant residues and manure (Wang et al. 2009; Arnault et al. 2013; Raza et al. 2017) |
Benzaldehyde | Pathogenic fungi and oomycetes (Wilson et al. 1999) | Yes (Chuankun et al. 2004; Zou et al. 2007; Zhao et al. 2016) | Many bacteria (Bacilli, Gammaproteobacteria), few fungi | Incorporation of plant materials containing precursors (Guo et al. 1998; Martens 2002) |
Furfural | Nematodes, pathogeni fungi and oomycetes (Gerik 2005; Crow and Luc 2014) | Yes (Leff and Fierer 2008) | Few bacteria, several fungi | May be stimulated via materials rich in hemi-cellulose (Eseyin and Steele 2015). |
2-Undecanone | Nematodes, insects, pathogenic fungi and oomycetes (Kai et al. 2009; Ntalli et al. 2016) | Yes (Zou et al. 2007; van Agtmaal et al. 2015; Zhao et al. 2016) | Many bacteria (Bacilli, Gammaproteobacteria, Actinomycetes), few fungi | Intercropping with onions (Antonious 2013) |
Of the mVOCs with known pathogen-suppressing properties, DMDS is probably the most studied for application as a fumigant in greenhouses and open fields. DMDS is patented for use as a fumigant (Paladin Technical EPA Reg. No. 55050–3) and is mostly used as a nematicide, but it can also reduce the number of propagules of plant pathogenic fungi in soils (Li et al. 2014; Gómez-Tenorio et al. 2015; Papazlatani et al. 2016). However, the use of mVOCs as fumigants can also have disadvantages because of high initial concentrations with often rapid evaporation rates, lack of stability and side-effects on nontarget organisms, including phytotoxicity (Choi et al. 2014; Dangi et al. 2014; Kanchiswamy, Malnoy and Maffei 2015b; Han et al. 2018).
As an alternative to the direct injection or dripping of chemically produced mVOCs, organic amendments can be considered that promote the production of suppressive mVOCs during decomposition (Potard et al. 2017; Raza et al. 2017). An obvious advantage of adding organic material instead of the mVOC compound itself is that the release of suppressive mVOCs in the soil atmosphere can continue for a prolonged period, namely as long as the decomposition of the added material is ongoing. Also for this option, DMDS is an interesting compound to zoom in further. Addition of sulfur-containing organic materials to soils often results in the production of organosulfur volatiles, e.g. DMDS (Banwart and Bremner 1976; Wang et al. 2009; Raza et al. 2017; Abis et al. 2018). Disease-suppressing activities against soil-borne fungal pathogens in which DMDS is involved were carried out with the incorporation of sulfur-rich Allium and Brassicaresidues (Wang et al. 2009; Arnault et al. 2013). Whereas disease-suppressive effects of incorporated Brassica material are often attributed to enzymatic conversions of glucosinolates into isothiocyanates, other factors, including the production of organosulfur volatiles by microbes, can be more important (Stapleton and Banuelos 2009; Larkin 2013; Vervoort et al. 2014; Larkin and Lynch 2018).
The selective stimulation of fungistatic and pathogen-suppressing organosulfur volatiles by S-rich organic material indicates that managing organic amendments in agricultural soils offers an opportunity to steer mVOC-mediated biocontrol (Bitas et al. 2013; Larkin 2015). However, in many cases, reports on the biocontrol stimulation by organic amendments have not included the possibility of mVOC involvement (Bailey and Lazarovits 2003; Bonanomi et al. 2010; Heck, Ghini and Bettiol 2019).
Although the biocontrol stimulation via organic amendments does not receive much attention, the analysis of mVOCs that are produced after the incorporation of organic residues in soils is receiving increasing attention (Zhao et al. 2016; Potard et al. 2017; Raza et al. 2017; Abis et al. 2018; Zhang et al. 2018). These kinds of studies will give information on the quantity and identity of pathogen-suppressing mVOCs with different organic amendments under different environmental conditions (soil texture, soil abiotic properties, etc.). Such information is required to make reliable predictions on the relationship between physicochemical characteristics of organic residues and the mVOCs produced during their decomposition in soil. In this respect, the increasing insights into the metabolic pathways leading to certain groups of mVOCs are very important (Schulz and Dickschat 2007; Penuelas et al. 2014). Such information can indicate which kind of precursor compounds should be present in organic materials in order to provide the conditions that will actually lead to the production of desired pathogen-suppressing VOCs (Kanchiswamy, Malnoy and Maffei 2015a; de Boer 2017).
Instead of using fresh or partly decomposed organic amendments, the use of living plants can also be used as a source of suppressive VOCs. This could be realized via intercropping. So far, most attention has been paid to suppressive VOCs emitted by the plant roots themselves, but microbes may play an important role here as well (Zhang et al. 2013; Li et al. 2018).
The inoculation of biocontrol microbes into soil is a commonly used strategy to suppress soil-borne fungal crop diseases. However, the results are mostly inconsistent, which can be explained, at least partly, by difficulties that biocontrol strains experience to establish themselves within indigenous soil microbial communities (de Boer 2017). Substrate-niche overlap between invader microbes and resident microbes is considered as a major constraining factor for the invader to be competitively successful and colonize ‘occupied territories’ (Mallon et al. 2015, 2018). The same holds true for biocontrol bacteria. One of the ideas to overcome the competitive exclusion of invader microbes by resident microbes is to provide the former with substrates that are not easily degraded by the latter (Mallon et al. 2015; Wei et al. 2015). This could also be an interesting option for applying strains that suppress pathogens via the production of mVOCs.
Whatever, the method will be that gives most success for the application of pathogen-suppressive microbial volatiles (Fig. 2), studies on possible negative side-effects, including phytotoxicity, mycorrhizal development and increase in other harmful soil organisms, should be done as well (Vesperman et al. 2007; Bitas et al. 2013; Dangi et al. 2014; Werner, Polle and Brinkmann 2016).
CONCLUSIONS AND PERSPECTIVES
It is well known that soil microbes can produce pathogen-suppressing mVOCs on artificial growth media, and several of these suppressive mVOCs occur in soil atmospheres, indicating their production in situ. Although it is recognized that these observations show the potential for application of mVOCs in controlling soil-borne pathogens, progress in that direction is limited. A major reason for this is the knowledge gap on mVOC production and activities under natural conditions in soils, i.e. within soil microbial communities. Yet, developments are promising as progress is being made on the identification of suppressive mVOCs in soil atmospheres and factors influencing mVOC production in soil microbial communities. This knowledge is essential for developing management strategies that result in the stimulation of mVOC-mediated suppression of soil-borne pathogens.
For further development towards application, it will be important to consider which life-cycle phase of the pathogens will be targeted by mVOCs. For instance, when preventing the infection of roots, crops are present, and the influence of root exudates on the production and activity of mVOCs should be taken into account (Fig. 1). Hence, this situation is different from the use of mVOCs in reducing the number of survival structures of pathogens. With respect to the latter, it is evident that in situ pathogen densities should be recorded when examining the effects of mVOC. The production and activity of mVOCs in soils involves microbial, chemical and physical aspects. Therefore, a collaboration between scientists from these disciplines will be important to exploring the possibilities of using mVOCs for enhancing the control of soil-borne diseases.
ACKNOWLEDGEMENTS
This work was supported by the National Key Research and Development Program of China (2017YFD0200604) and the National Natural Science Foundation of China (41671306). This is publication No. 6756 of the Netherlands Institute of Ecology (NIOO-KNAW).
Conflicts of interest. None declared.
REFERENCES
Author notes
The authors contributed equally to this work.