-
PDF
- Split View
-
Views
-
Cite
Cite
William J Landesman, Zachary B Freedman, David M Nelson, Seasonal, sub-seasonal and diurnal variation of soil bacterial community composition in a temperate deciduous forest, FEMS Microbiology Ecology, Volume 95, Issue 2, February 2019, fiz002, https://doi.org/10.1093/femsec/fiz002
- Share Icon Share
ABSTRACT
The temporal dynamics of soil bacterial communities are understudied, but such understanding is critical to elucidating the drivers of community variation. The goal of this study was to characterize how soil bacterial communities vary across diurnal, sub-seasonal and seasonal time-scales in a 5.8 m2 plot and test the hypothesis that bacterial diversity varies on each of these scales. We used 16S rDNA gene amplicon sequencing to quantify the alpha and beta diversity of soil bacteria as well as the Net Relatedness Index and Nearest Taxon Indices to assess the degree of phylogenetic clustering, and the extent to which community shifts were driven by stochastic vs. deterministic limitation. We found that species richness was highest in winter, lowest in fall and that communities were compositionally distinct across seasons. There was no evidence of diurnal-scale shifts; the finest temporal scale over which community shifts were detected using our DNA-based analysis was between sampling dates separated by 6 weeks. Phylogenetic analyses suggested that seasonal-scale differences in community composition were the result of environmental filtering and homogeneous selection. Our findings provide insight into temporal variation of soil bacterial communities across the hourly to seasonal scales while minimizing the potential confounding effect of spatial variation.
INTRODUCTION
Soils are highly diverse and dynamic environments, and soil microbial communities have an important influence on soil biogeochemistry and fertility (van der Heijden, Bardgett and van Straalen 2008). Soil processes, such as decomposition and nitrogen mineralization, are thought to be highly sensitive to ongoing and projected climate change, in part because of the direct and indirect effects of climate on microbial communities (Fierer, Bradford and Jackson 2007; Classen et al. 2015; DeAngelis et al. 2015; Freedman et al. 2016). Recent research has assessed the relative importance of various potential environmental drivers of spatial variation in soil bacterial community composition (e.g. Fierer and Jackson 2006; Zhou et al. 2016; Delgado-Baquerizo et al. 2017). However, microbial communities also vary through time (e.g. Bardgett et al. 1999; Marschner et al. 2002; Lauber et al. 2013; Degrune et al. 2017), and there is a need for field studies that simultaneously measure temporal variation across multiple scales (e.g. hourly to seasonal), while also minimizing potentially confounding variation in communities due to differences in location. Such studies may help improve understanding of the response of soil biogeochemical processes to changing environmental conditions, the attribution of soil ecosystem functions to particular microbial groups (Strickland et al. 2009; Aanderud et al. 2015; Baldrian 2017), and predictions of biogeochemical processes (Kuzyakov and Blagodatskaya 2015; Žifčáková et al. 2017). Furthermore, temporal dynamics of bacterial communities have been reported for other systems, including rivers and estuaries (Fortunato et al. 2012; Graham et al. 2016), lakes (Shade, Jones and McMahon 2008; Eiler, Heinrich and Bertilsson 2012) and nearshore marine communities (Gilbert et al. 2012), and a greater understanding of temporal dynamics in soil bacterial communities can help provide a more general understanding of the patterns and controls of temporal variation in environmental microbial communities.
A variety of factors may influence temporal variation in soil microbial community composition. On long (e.g. decadal or more) time scales, microbial communities are thought to be structured by processes such as genetic drift and dispersal barriers (Martiny et al. 2006; Vellend 2010; Freedman and Zak 2015a), as well as by changes to litter quality, soil fertility, and soil pH associated with plant growth and succession (Wardle et al. 2004; Mitchell et al. 2010; Landesman, Nelson and Fitzpatrick 2014). Recent studies indicate that soil bacterial community composition also varies seasonally (e.g. Lipson and Schmidt 2004; Kaiser et al. 2010; Kuffner et al. 2012; Lauber et al. 2013; López-Mondéjar et al. 2015; Žifčáková et al. 2016), potentially in relation to seasonal differences soil temperature, moisture and/or soil organic matter content or autecological dynamics (e.g. dormancy; Lennon and Jones 2011). Across shorter (e.g. hourly to daily) time scales, compositional shifts in soil microbial communities may be driven by changes in litter and root-derived soil organic matter, whose quantity and composition may vary temporally (Kuzyakov and Blagodatskaya 2015; Baldrian 2017).
Although hourly-scale shifts in soil bacterial community structure can occur in response to rainfall and nitrogen availability (Landesman and Dighton 2011; Fierer et al. 2012; Aanderud et al. 2015), few studies have investigated the potential for communities to shift on diel- or diurnal-scales in the absence of such pulsed resources, especially in natural environments. Factors that exhibit diurnal-scale patterns and that could contribute to diurnal-scale variation in soil bacterial community composition include soil temperature (Tang, Baldocchi and Xu 2005; Gunnigle et al. 2017), photosynthetic activity and root exudate production (Neumann and Römheld 2000; Phillips et al. 2008; Högberg et al. 2010; Herron et al. 2013). However, we are unaware of studies that measured diel- or diurnal-scale patterns of bacterial communities in temperate forest soils. A further challenge is that forest soils contain high levels of extracellular DNA (Levy-Booth et al. 2007; Carini et al. 2016), which may interfere with the detection of short-term changes in bacterial community composition. However, DNA-based analyses can improve predictions of biogeochemical process rates (Strickland et al. 2009) and are less expensive than RNA-based analyses, making them well suited for the study of microbial temporal dynamics.
We conducted a one-year study to assess seasonal, sub-seasonal and diurnal variation in the composition of soil microbial communities, and the extent to which different bacterial taxa contribute to such temporal variation. Our first objective was to characterize how soil bacterial richness (i.e. alpha-diversity) and community composition (i.e. beta-diversity) vary over hourly, sub-seasonal and seasonal scales. Our second objective was to determine the shortest time step over which changes in bacterial community composition could be detected with a DNA-based analysis. We performed this study within a small (5.8m2) plot to minimize, as much as possible, the potentially confounding influences of spatially dependent environmental factors on soil bacterial communities. We tested the hypothesis that the richness and composition of soil bacterial communities exhibit distinct seasonal, sub-seasonal and diurnal patterns.
METHODS
This study was conducted within a secondary deciduous forest in the Appalachian Mountains near Frostburg, Maryland (39.666 N, −78.98 W). The canopy is dominated by oak (Quercus rubrum and Quercus alba), cherry (Prunus serotina) and maple (Acer saccharum, Acer rubrum) trees. The organic horizon in the study plot had an average depth of 5 cm and an average pH of 4.63 (± 0.05 SE). The average soil C/N ratio was 16.7. The soils are classified as Meckesville, which are well drained, very stony silt loams with 8%–25% slopes (https://websoilsurvey.sc.egov.usda.gov/), although the precise sampling area had little to no sloping.
We collected 240 soil samples from one 2.4 m x 2.4 m (5.8 m2) plot on 10 different days during 2010. To minimize possible disturbance effects associated with our intensive sampling scheme, sampling locations were predetermined by a random number generator, with each number corresponding to a particular coordinate on a grid. Samples were collected during two or three 24-hour periods per season. In seasons with two sampling periods (winter and fall), the samples were collected 6 weeks apart, and in seasons with three sampling periods (spring and summer) the samples were collected 3 weeks apart (Table S1, Supporting Information). On each sampling period we removed four randomly selected samples every 4 hours, beginning at 8 am and ending at 4 am the following day. To avoid the potentially confounding hourly-scale effects of rainfall on microbial community composition (Clarholm and Rosswall 1980; Zhang and Zak 1998; Cleveland et al. 2006; Landesman and Dighton 2011), we selected sampling dates for which total rainfall in the 2 days prior to the beginning of sampling was less than 5 mm. We selected this time frame because previous work indicated that soil microbial community composition can rapidly shift within the first 48 hours of rainfall events (Landesman and Dighton 2011), potentially confounding detection of the hypothesized diurnal-scale patterns. During one sampling period (September 3–4) a brief rain shower occurred 2.5 hours before the fourth sampling event, but samples from this period were not removed from the analysis. Soils were sampled using a 2.5 cm diameter coring device to a depth of 5 cm. Visible litter and coarse debris were removed in the field and samples were homogenized through a 2 mm sieve prior to laboratory analyses. This sieving approach was expected to retain fine roots, and thus capture the expected diurnal-scale shifts in root exudates. Samples were collected in plastic bags and stored at −20°C within 24 hours of collection.
We measured soil pH on a subset (n = 37) of samples to determine if this soil property varied across time or space, since soil pH is known to correlate strongly with soil bacterial community composition (e.g. Fierer and Jackson 2006; Lauber et al. 2009; Landesman, Nelson and Fitzpatrick 2014). Soil pH of 2 g of air-dried soil mixed with 20 ml of 0.01 M CaCl2 was measured using a Thermo Orion 410A þ pH meter and an Orion 8104BN Ross Combination pH probe. Additionally, we measured gravimetric water content (GWC) on a subset of samples (n = 70), with seven samples per sampling date, by determining mass loss of 30 mg samples that were dried at 105°C for 48 hours. Total soil carbon (C) and nitrogen (N) of dried soil were determined on the same subset of samples used for GWC, with a Carlo Erba NC2500 elemental analyzer (CE Instruments, Milano, Italy), using acetanilide and atropine standards. Due to the small quantities of soil collected, pH measurements were performed on separate samples from those measured for C/N and soil moisture. We obtained daily air temperature and daily precipitation data from NOAA's National Center for Environmental data (station ‘FROSTBURG 2 MD US’ https://www.ncdc.noaa.gov/).
We characterized soil bacterial community composition in each sample via 16S rDNA gene amplicon sequencing using the procedures described in Landesman, Nelson and Fitzpatrick (2014). Briefly, genomic DNA was isolated from 0.25 g soil with the MoBIO Power-Soil® DNA Isolation Kit (MO BIO Laboratories, Inc., Carlsbad, CA, USA) following the manufacturer's instructions. DNA was quantified with the Qubit® dsDNA BR Assay on a Qubit 1.0 fluorometer (Life Technologies, Carlsbad, CA, USA). We used the universal prokaryotic 515f/806r primer pair with 10 bp barcodes for amplification of the V4 region of the 16S rDNA gene (Caporaso et al. 2010; Bergmann et al. 2011). The PCR reactions consisted of 2.5 uM forward and reverse primer, 2 mL template DNA (10 ng/µL), and 18 mL FastStart High Fidelity PCR System master mix (Roche Applied Science, Indianapolis, USA). PCR reactions were performed in duplicate using an Eppendorf Mastercycler® pro thermal cycler. First, DNA was denatured at 94°C for 3 min, followed by 25 cycles of 94°C for 45 sec (denaturation), 50°C for 30 sec (annealing) and 72°C for 90 sec (extension). There was a final extension step at 72°C for 10 min. PCR products were combined and purified with the MoBIO UltraClean® PCR Clean-Up Kit. Equal concentrations of samples were pooled, gel purified and extracted with the Qiagen QIAquick Gel Extraction Kit (Alameda, CA, USA). Sequencing of the pooled samples was performed from the B adapter on the forward primer on the Roche/454 GS FLX Titanium platform at the Roy J. Carver Biotechnology Center at the University of Illinois at Urbana-Champaign.
Sequence reads were processed using QIIME v1.6 and v1.9 (Caporaso et al. 2010), following the recommended filtering steps described in Kuczynski et al. (2012). Operational taxonomic units (OTUs) were selected at the 97% identity threshold by de novo OTU picking with the uclust routine (Edgar 2010) against the Greengenes (v 13.8) reference database (DeSantis et al. 2006). The OTU table was rarefied to 1247 sequences per sample, and phylogenetic relatedness was reconstructed using the FastTree approximate maximum-likelihood algorithm (Price et al. 2010). For alpha diversity, we calculated Faith's Phylogenetic Diversity (FPD), which quantifies species richness by weighting taxa based on their phylogenetic similarity. Samples that contained more distant taxa receive a higher richness score than those for which the taxa are more closely related (Faith 1992; Lauber et al. 2013). For beta diversity we calculated the abundance-unweighted UniFrac distance metric (Lozupone and Knight 2005; Lauber et al. 2009) to determine the degree of similarity among the bacterial communities in the samples. We used indices that emphasize species richness, as opposed to relative abundance, because the 16S rDNA gene copy number is variable among bacterial species (Pei et al. 2010; Kembel et al. 2012). Archaeal sequences were excluded from the dataset.
Statistical analyses
To elucidate the possible ecological mechanisms that influence temporal dynamics among bacterial communities, we calculated three additional measures of phylogenetic structure: The net relatedness index (NRI), nearest taxon index (NTI) and the β nearest taxon index (βNTI) against the ‘phylogeny shuffle’ null model. The NRI is a metric of phylogenetic clustering across a phylogenetic tree within a sample, whereas the NTI measures the degree of phylogenetic clustering of taxa on the tips of the phylogenetic tree (Webb et al. 2002; Fine and Kembel 2011) and is a metric of phylogenetic evenness (Kembel et al. 2011). Higher values of NRI and NTI (> 2) indicate phylogenetic clustering among samples and are indicative of environmental filtering. Lower values (< 2) indicate over dispersion and suggest the importance of interactions, such as facilitation or competition, in structuring microbial communities (sensu Freedman and Zak 2015b). The βNTI is a metric of phylogenetic turnover; a βNTI < −2 is indicative of less turnover than expected by chance due to consistent selective pressure (i.e. homogeneous selection) and βNTI > +2 is indicative of more turnover than expected by chance due to dynamic selective pressures (i.e. variable selection; sensu Stegen et al. 2012; Dini-Andreote et al. 2015; Graham and Stegen 2017). Under ‘homogenous selection’, environmental conditions are spatially homogeneous across the study area and community turnover is due to the relative fitness of species to those environmental conditions.
Statistical analyses were performed in Primer-E (Version 7; Plymouth, UK), R (Version 3.4.0; R Core Team 2013) and SPSS Statistics Version 24 (SPSS, Inc., Chicago, IL). For NRI, NTI and βNTI we used the picante package in R (Kembel et al. 2010) and other publicly-available custom scripts (Stegen et al. 2012).
To test the influence of temporal scale on FPD (i.e. alpha diversity), we implemented a one-way Analysis of Variance (ANOVA); means were compared with a protected Tukey's honest significant difference test (HSD; Tukey 1949). We then used permutational multivariate analysis of variance (PerMANOVA; Anderson 2001) to measure the impact of temporal scale on bacterial community composition (i.e. beta diversity). This test was performed on the abundance-unweighted UniFrac distance matrix, with season, sub-season, hours and their interactions as factors. We used principal coordinates analysis to visualize similarity among the soil bacterial communities over time. We used a permutational distance-based test for homogeneity of multivariate dispersions (PERMDISP) to determine if observed shifts in bacterial community composition result from differences in assemblage location or degree of dispersion in ordination space (Anderson 2004). Contributions of bacterial taxa to the total dissimilarity between the seasonal or sub-seasonal temporal scales were determined using Similarity Percentage Analysis (SIMPER; Clarke 1993). We used stepwise linear regression to analyze associations between diversity matrices (Faiths, NRI and NTI), the most abundant and influential bacterial orders (as determined by SIMPER) and environmental factors, including average air temperature (30-day and 10-day average) and total precipitation in the seven days and 30 days prior to sampling. This statistical approach allowed us to quantify correlations among environmental variables while controlling for variance attributable to other predictor variables as well as for multiple comparisons.
RESULTS
We obtained 459 455 sequences and 14 424 OTUs. Four samples contained counts below 600 sequences/sample and were removed from the analysis during the rarefaction step (see below). Across all samples, the most dominant phyla were the Acidobacteria (37%), Proteobacteria (30%), Actinobacteria (9.2%), Verrucomicrobia (6.3%), Bacteroidetes (4.9%) and Planktomycetes (3.8%). The most abundant orders (>5% relative abundance) were the Acidobacteriales (14.6%–16.5% by season), an unidentified Acidobacteria (11.2%–13.5%), Solibacterales (6.2%–9.1%), Rhizobiales (6.4%–8.3%), Actinomycetales (4.1%–10.7%) and Xanthomonadales (5.9%–7.2%; Fig. S1, Supporting Information).
Faith's phylogenetic diversity across temporal scales
There was a significant difference in FPD across seasons (Fig. 1; ANOVA; P < 0.01). FPD was highest in the winter (54.2 ± 0.6; HSD P < 0.05), lowest in the fall (47.5 ± 0.5; HSD P < 0.01) and moderate in spring and summer (51.1 ± 0.4 and 52.1 ± 0.6, respectively). At the sub-seasonal scale between the two sampling dates in winter, separated by 6 weeks, the bacterial FPD was significantly higher on the first (55.5 ± 0.7) vs. second (52.9 ± 0.9; HSD P < 0.05) date. Across the three sampling dates in summer, bacterial community FPD was lowest on the first sampling date (50.1 ± 0.6) and highest on the last sampling date (54.2 ± 1.1; HSD P < 0.05), 6 weeks later. The second sub-seasonal sampling date was intermediate and not significantly different from the first and third sampling dates (Fig. 2). Bacterial community FPD was not significantly different across sampling dates during the spring or the fall. There was no significant difference between FPD for samples collected 12 hours apart (8:00 am vs. 8:00 pm, 12:00 am vs. 12:00 pm and 4:00 am vs. 4:00 pm).
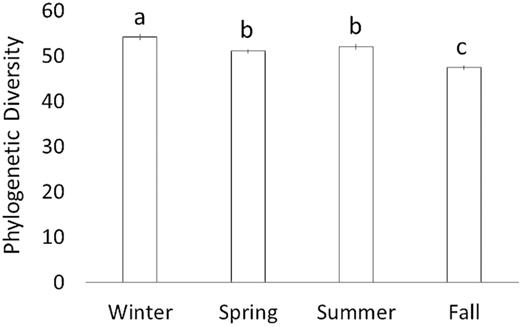
Faith's Index (i.e. phylogenetic diversity) of soil bacterial communities across seasons. The mean ± SE (represented by the vertical bars) of all samplings in each season is presented.
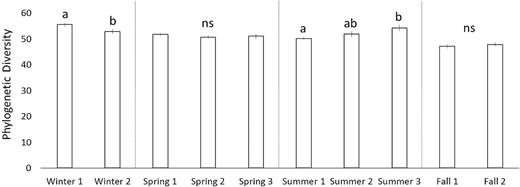
Faith's Index (i.e. phylogenetic diversity) of soil bacterial communities at each sub-seasonal sampling date. The mean ± SE (represented by the vertical bars) is presented.
Bacterial community composition across temporal scales
Soil beta diversity was distinct across seasons (PerMANOVA; P < 0.01; Fig. S2 and Table S2-a, Supporting Information). When compared in a pairwise fashion, the PerMANOVA test revealed that the microbiome of each season was compositionally distinct from the microbiome of other seasons (Table S2-b, Supporting Information). When considered in chronological order, PERMDISP revealed that bacterial community composition differed seasonally by dispersion, with winter and summer having greater average distances to the season centroid than spring and fall (P < 0.05; Table S2-c, Supporting Information). However, when comparing seasons separated by six months bacterial community composition differed by location in ordination space and not by dispersion (PERMDISP; P > 0.20 for the pairwise comparisons between fall/spring and winter/summer).
On a sub-seasonal scale, changes in bacterial community composition were observed across several sampling dates (season × sub-season P < 0.01; Table S2-d, Supporting Information). In the spring, pairwise differences in species composition existed between the first and third sampling day (a 6-week time step, P < 0.05), were borderline significant between the first and second sampling day (a 3-week time step; P = 0.09) and the bacterial communities between the second and third sampling dates were not significantly different. In summer, bacterial community composition was borderline significantly different between sampling days one and two (a 3-week time-step, P = 0.09), significantly different between sampling days one and three (6-week time step, P < 0.01), and not significantly different between sampling days two and three. Only two sub-seasonal dates were sampled in the fall and winter, and the bacterial community composition was different across sampling dates for both seasons (6-week time step; P ≤ 0.05). The effect of time of day was not statistically significant (P = 0.21, data not shown).
Over 99% of the samples had NRI and NTI values greater than two, which indicates greater phylogenetic clustering than expected by chance (Table S3, Supporting Information). However, neither the NRI or NTI changed through time. Similarly, over 99% of ßNTI comparisons were less than −2, indicating that the bacterial phylogenetic turnover was less than expected by chance.
Microbial taxa driving seasonal and sub-seasonal shifts
Although many bacterial phyla consistently contributed to community dissimilarity across the calendar year, members of the Actinobacteria increasingly contributed to total compositional dissimilarity between winter and spring (11%), spring and summer (13%) and summer and fall (22%). Actinobacteria exhibited the greatest amount of dissimilarity of all bacterial phyla for the comparison between fall and winter (the first and last sampling dates), as indicated by SIMPER ( 26%). Among other phyla, members of the Acidobacteria contributed ∼25% of multivariate dissimilarity across the calendar year, which was the greatest contribution to total dissimilarity across seasons of all bacterial phyla. Other highly important contributing bacterial phyla were the Proteobacteria (∼18% of dissimilarity), Actinobacteria (∼18%), Bacteroidetes (∼7%) and Verrucomicrobia (∼7%).
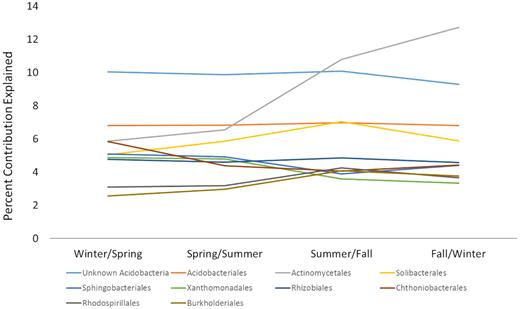
Results of SIMPER analysis indicating the contribution of bacterial orders to differences in community composition across seasons.
At the order level, ∼22% of the compositional dissimilarity across seasons was attributed to orders within the Acidobacteria phylum, namely an Acidobacteria (∼10% of dissimilarity), Acidobacteriales (∼7%) and Solibacteriales (6%; order-level data not shown). The order Actinomycetales (phylum Actinobacteria) contributed a high proportion of compositional dissimilarity across the calendar year for the winter vs. spring (6%), spring vs. summer (7%) and summer vs. fall (11%) comparisons. The Actinomycetales exhibited the most dissimilarity of all bacterial orders for the fall vs. winter comparison (13%; Fig. 3).
Correlations between soil bacterial diversity and environmental factors
For most bacterial taxa and diversity matrices, there were weak and non-significant correlations with environmental factors after controlling for multiple comparisons (Table S4, Supporting Information). However, the Actinomycetales and Xanthomonadales (representing 5.4% and 6.2% of the total community) were significantly correlated with total soil N (R2 = 0.15; P < 0.05) and total soil C (R2 = 0.19; P < 0.05), respectively. Additionally, the Chthoniobacterales (representing 4.4% of the total community) were significantly correlated with the combination of average temperature (30-day average), C and GWC (R2 = 0.46; P < 0.05). Xanthomonadales and Chthoniobacterales were also significantly correlated with the combination of temperature (30-day and 10-day averages) and soil pH (R2 = 0.38 and 0.40, respectively) at the P < 0.05 level.
DISCUSSION
Our results indicated that soil bacterial communities in a temperate deciduous forest varied in their alpha and beta diversity across seasons, and in their beta diversity among sampling dates within seasons. This observed temporal turnover in bacterial communities was driven by environmental filtering, as indicated by a phylogenetically clustered organization (by NRI, NTI and βNTI) as well as correlations between some taxa and environmental parameters, although a significant amount of community variation remains unexplained. Members of the Actinobacteria, a functionally important phylum, accounted for a majority of seasonal shifts in bacterial community composition. Seasonal variation in soil microbial communities has been previously reported (e.g. Lipson and Schmidt 2004; Kaiser et al. 2010; Kuffner et al. 2012; Lauber et al. 2013; López-Mondéjar et al. 2015; Žifčáková et al. 2016). In this study, we demonstrate such variation over a smaller (5.8 m2) area and with greater temporal resolution. This relatively small spatial scale allowed us to minimize the potentially confounding effects of space and was expected to capture a large amount of spatial variation among bacterial communities (O'Brien et al. 2016). The finest temporal scale across which we detected compositional shifts between two sampling events was 6 weeks, and this sub-seasonal variation was detected during all seasons. This sub-seasonal time scale may approximate the shortest time-step across which compositional differences can be detected in a temperate forest soils using rDNA gene sequencing. This estimate could be further refined with more intensive sub-seasonal sampling.
Seasonal-scale variation in soil bacterial communities
We observed that soil bacterial communities were compositionally distinct across seasons. Further, the manner in which they were distinct differed based on whether communities were considered chronologically across a calendar year (i.e. winter vs. spring, summer vs. fall) or across seasons separated by 6 months (i.e. winter vs. summer, fall vs. spring). For the former, community differences were driven by differences in the dispersion of samples, most likely due to variation in relative abundances of phylogenetically-similar taxa (Table S2-c, Supporting Information; Anderson, Ellingsen and McArdle 2006). For the latter, the bacterial community composition differed by location in ordination space and not by dispersion (PERMDISP; P > 0.20 for pairwise comparisons between fall/spring and winter/summer). Similar results have been observed across time in soil mesocosms (Pan et al. 2014) as well as in sponge-associated bacterial communities (Erwin et al. 2012).
When considered as alpha- (FPD) or beta-diversity (unweighted UniFrac), the largest pairwise seasonal differences included comparisons against the fall season. For example, the greatest difference in FPD was between winter and fall (Fig. 1) and the largest compositional differences were found in the comparison of fall vs. spring and fall vs. winter (Table S2-b, Supporting Information). A potential driver of these observed seasonal shifts is changes in plant phenology that affect litter inputs, root exudates (Griffiths et al. 1999; Högberg et al. 2001; Högberg et al. 2010; Kaiser et al. 2010; Prescott and Grayston 2013; Žifčáková et al. 2016) and soil temperature, all of which can constrain soil microbial community composition (e.g. Lipson 2007; Zhou et al. 2016; Oliverio et al. 2017). For example, it is plausible that the fall litter input selected for a distinct bacterial community adapted to the labile carbon sources (e.g. bacteria with cellulase and protease activity), and distinct late-summer/fall communities with enzymes that process more recalcitrant organic matter.
Members of the Actinobacteria phylum accounted for a majority of seasonal shifts in bacterial community composition (Fig. 3). Consistent with this result, several other studies demonstrate that the Actinobacteria exhibited seasonal variation in relative abundance (e.g. Kuffner et al. 2012; Žifčáková et al. 2016). We found the largest percentage of actinobacterial sequences in fall, which is consistent with other research showing a fall peak in the activity of manganese peroxidase (Criquet et al. 2000; Baldrian et al. 2013), an enzyme produced by several species within the Actinobacteria (Mercer et al. 1996). Additionally, members of the order Actinomycetales tended to increase in experimentally trenched plots (Brant, Myrold and Sulzman 2006), a condition that excludes root exudates and that would occur in fall and winter. Furthermore, Actinomycetales tended to decrease in response to root exudate substrates (Griffiths et al. 1999), as would occur during spring and summer. Recent analysis in soil mesocosms determined OTUs attributable to the Actinobacteria can be favored by pulses of labile C (i.e. glucose; Morrissey et al. 2017) as well as by recalcitrant C sources (Bugg et al. 2011; Wilhelm et al. 2018). Thus, multiple lines of evidence suggest that turnover of OTUs within the Actinobacteria phylum involved in labile and recalcitrant C decay may drive compositional differences observed in our study site across the calendar year. Actinobacteria have been implicated in several important soil microbial functions, including soil organic C mineralization, antibiotic synthesis, as well as engaging in symbioses with plants (McCarthy and Williams 1992; Větrovský, Steffen and Baldrian 2014; Barka et al. 2016) and we therefore suggest that the observed seasonal changes may have important functional implications for these soils.
Elucidating the ecological processes mediating seasonal responses
Our finding of no change in NRI or NTI over time, and βNTI < −2 for over 99% of the samples, suggests that the soil bacterial community was overwhelmingly influenced by deterministic selection and environmental filtering across the calendar year (sensu Stegen et al. 2013). There was little evidence for influence by biotic interactions (only 0.003% of samples had an NTI of < −2) or stochastic selection, such as dispersal limitation and drift (only 0.87% of the samples had |βNTI| < 2). The relationships between the bacterial community and soil properties further support the interpretation that environmental factors mediated variation in bacterial community composition over time (Table S4, Supporting Information). In our dataset, NRI and NTI scores > 2 across the year indicated that the soil microbiome was phylogenetically clustered. Many assessments of microbial communities, in both aquatic and terrestrial environments, have observed that phylogenetic clustering of microbial communities can be lessened by alleviation of nutrient limitation (i.e. N fertilization; Horner-Devine and Bohannan 2006; Peay et al. 2010; Freedman and Zak 2014; Amend, Matulich and Martiny 2015). Thus, the phylogenetic clustering detected in the current study may suggest that nutrient limitation is a key environmental driver constraining bacterial community composition across seasons. Since stochastic influences (e.g. barriers to dispersal, drift) are thought to mostly influence soil microbial communities across wide temporal and spatial scales (Brown and Jumpponen 2014; Cline and Zak 2014; Freedman and Zak 2015a) it is unsurprising that such mechanisms did not exert strong pressure on the soil bacterial community in our study of a small soil plot throughout one calendar year.
Hourly and sub-seasonal scale variation in bacterial communities
The finest temporal scale at which we detected significant community shifts was across sample dates separated by 6 weeks, which was detected in all seasons (i.e. at the sub-seasonal scale). A similar temporal limit-of-detection was found for planktonic bacterial communities in the hyporheic zone studied by Graham et al. (2016). Underlying our hypothesis of diurnal-scale community changes were the observations that shifts in microbial biomass can be detected at the hourly-scale (e.g. Clarholm and Rosswall 1980; Zhang and Zak 1998; Landesman and Dighton 2011). However, in the absence of a pulsed resource such as rainfall, a 6-week timeframe may represent an approximate limit in the time scale across which community shifts can be detected with DNA-based approaches in soils not severely limited by critical environmental factors (i.e. moisture in mediterranean grasslands; Barnard, Osborne and Firestone 2015). Furthermore, we may be limited in our ability to detect mechanisms of community change, particularly with respect to plant-soil-fungal-bacterial interactions and fine-scale changes in environmental factors. For example, in soil, upwards of 80% of microbial cells and 50% of microbial OTUs may be inactive or dormant (Lennon and Jones 2011), thus not engaging in full-genome replication that would be detected by our analysis. An additional potentially confounding factor in detecting diurnal-scale variation in soil bacterial communities in our study may be extracellular DNA that may compete with cellular DNA for primer binding (Carini et al. 2016). Follow-up studies using stable-isotope-probing based methods (Neufeld et al. 2007; Hungate et al. 2015) across several experimental plots with varying plant species composition are warranted to advance a mechanistic understanding of temporal dynamics of soil microbiomes. Despite this potential limitation, the current study makes an important contribution to the understanding of the temporal scales across which bacterial communities change detectably with 16S rDNA data.
Correlational analyses (Table S4, Supporting Information) provide insight into the environmental drivers of the observed temporal patterns in bacterial community composition. Among the orders that were most influential to seasonal variation in bacterial community composition (>2.5% of community dissimilarity explained), the Actinomycetales, Chthoniobacterales and Xanthomonadales exhibited significant correlations with some soil properties and temperature. However, these taxa were either weakly or moderately correlated with environmental properties. Furthermore, none of the other influential taxa (Acidobacteriales, Acidobacteria-2, Solibacterales, Rhizobiales, Sphingobacteriales, Rhodospirillales, Chthoniobacterales and Burkholderiales) were correlated with the measured environmental properties. Together, these other taxa represented, on average, 55% of the sequences obtained per sample. Thus, there remains a large amount of unexplained temporal variation in the observed bacterial community composition.
Conclusions
Our results demonstrated that deterministic selection by variation in environmental factors drove differences in soil bacterial community composition among and between seasons in a temperate deciduous forest. Stochastic processes (i.e. barriers to dispersal) did not significantly influence bacterial diversity across seasons. Further, OTUs related to Actinomycetales, which are thought to be associated with organic matter decay, contributed most to variation in bacterial community composition throughout the year. Although diurnal-scale variation in abiotic factors and plant phenology may affect soil bacterial community composition, no such diurnal-scale shifts were detected in our experiment. Dates separated by 6 weeks may represent an approximate limit on the temporal resolution for detecting shifts in bacterial community composition and species richness using rDNA-based sequence analysis. Thus, our results may be used to inform sampling designs for microbial community analyses, and we recommend that the limit of DNA-based tests should be at the interface between the seasonal and sub-seasonal scales.
ACKNOWLEDGEMENTS
We thank Jim Garlitz and Robin Paulman for laboratory support, Kenneth Mulder for advice on statistical analyses and Lydia and Abigail Nelson for field assistance. We thank Chris Wright and Dr. Alvaro Hernandez of the Roy J. Carver Biotechnology Center for sequencing services. We thank two anonymous reviewers whose suggestions greatly improved this manuscript.
FUNDING
This project was funded by the National Geospatial Intelligence Agency grant HM1582-09-1-0010 to D. Nelson. W. Landesman was supported by an Institutional Development Award (IDeA) from the National Institute of General Medical Sciences of the National Institutes of Health under grant number P20GM103449. Z. Freedman was supported by the USDA National Institute of Food and Agriculture Hatch project 1011670 and the West Virginia Agricultural and Forestry Experiment
Conflict of interest. None declared.