-
PDF
- Split View
-
Views
-
Cite
Cite
Azhar A Zarkani, Jasper Schierstaedt, Marlies Becker, Johannes Krumwiede, Maja Grimm, Rita Grosch, Sven Jechalke, Adam Schikora, Salmonella adapts to plants and their environment during colonization of tomatoes, FEMS Microbiology Ecology, Volume 95, Issue 11, November 2019, fiz152, https://doi.org/10.1093/femsec/fiz152
- Share Icon Share
ABSTRACT
Humans and animals are considered typical hosts for Salmonella, however, also plants can be colonized. Tomatoes were linked to salmonellosis outbreaks already on several occasions. The aim of this study was, therefore, to establish a comprehensive view on the interaction between Salmonella enterica and tomatoes, and to test the hypothesis that colonization of plants is an interactive process. We assessed the persistence of Salmonella in agricultural soil, the colonization pattern in and on tomatoes, as well as the reciprocal responses of tomatoes to different Salmonella strains and Salmonella to root exudates and tomato-related media. This study revealed that Salmonella can persist in the soil and inside the tomato plant. Additionally, we show that Salmonella strains have particular colonization pattern, although the persistence inside the plant differs between the tested strains. Furthermore, the transcriptome response of tomato showed an up-regulation of several defense-related genes. Salmonella transcriptome analysis in response to the plant-based media showed differentially regulated genes related to amino acid and fatty acid synthesis and stress response, while the response to root exudates revealed regulation of the glyoxylate cycle. Our results indicate that both organisms actively engage in the interaction and that Salmonella adapts to the plant environment.
INTRODUCTION
The last decade has seen a growing trend towards gastroenteritis outbreaks caused by non-typhoidal Salmonella enterica strains. Many of which were linked to fruits and vegetables, including processed and frozen food containing plant products. Contamination with Salmonella resulted in thousands of hospitalizations and noticeable economic losses to the food industry (Mandrell 2009; Barak and Schroeder 2012; CDC 2018; EFSA 2018). Under suitable conditions, human enteric pathogens can colonize and persist within various plant species and plant tissues (Schikora et al. 2008; Noel et al. 2010; Han and Micallef 2016; de Moraes et al. 2017; EFSA 2018; CDC 2019; Jechalke et al. 2019).
Tomatoes (Solanum lycopersicum) are one of the most widely consumed crops and have been frequently associated with salmonellosis outbreaks, some outbreaks were even directly linked to tomatoes (Hedberg et al. 1999; Cummings et al. 2001; Gupta et al. 2007; Gu et al. 2011; Bartz, Marvasi and Teplitski 2014; EFSA 2014; EFSA 2018). Internalization of Salmonella in tomato plants via irrigation water has been postulated to depend on the serovar and the inoculum density (Zheng et al. 2013). Not only the use of contaminated water but also contaminated soil resulted in detectable S. enterica populations in the phyllosphere, which indicates a risk of fruit contamination and subsequent human disease (Barak, Kramer and Hao 2011). Moreover, S. enterica can enter plant leaves through natural openings, such as stomata or hydathodes (Kroupitski et al. 2009; Gu, Cevallos-Cevallos and van Bruggen 2013). Also, type I glandular trichomes were suggested to be a possible colonization and entry site into tomato leaves (Barak, Kramer and Hao 2011; Gu et al. 2011). In addition, it was suggested that Salmonella can reach tomato fruit using internal translocation from leaves through the phloem (Gu et al. 2011). Furthermore, Salmonella serovars introduced on flowers were recovered from within the developing tomato fruit, indicating the possibility of Salmonella translocation into the edible part of the plant once established in the vegetative tissues (Shi et al. 2007). However, access and internal persistence of Salmonella in tomato leaves were shown to be affected by the tomato cultivar (Gu, Cevallos-Cevallos and van Bruggen 2013) and the ripening stage, mature tomatoes were found to be more favorable to the growth of some Salmonella serovars if compared with immature tomatoes (Noel et al. 2010; Marvasi et al. 2013). Thus, it was hypothesized that colonization of tomato surfaces and vegetative and reproductive tissues depends on several factors, such as particular Salmonella genes, the tomato genotype, the physiological state of the plant, and the plant tissues (Shi et al. 2007; Noel et al. 2010; Barak, Kramer and Hao 2011; Barak and Schroeder 2012).
To survive on/in plant hosts, Salmonella must adapt to numerous stresses, including differences in pH, osmolarity or temperature, as well as UV light, oxidative stress and anti-microbial compounds (Foster and Spector 1995; Santamaria and Toranzos 2003). Under such environmental conditions, bacteria activate stress responses that substantially improve their chances of survival. Numerous reports have revealed that different Salmonella serovars utilize various strategies to colonize plants. Those may involve assembly of flagella, the fimbriae curli, O-antigen capsule, biofilm formation and synthesis of cellulose (Barak et al. 2007; Berger et al. 2009; Lapidot and Yaron 2009). Furthermore, similar to phytopathogens, Salmonella seems to require the biosynthesis of amino acids and lipopolysaccharides (LPS) to colonize tomatoes (de Moraes et al. 2017). The expression of genes involved in amino acid biosynthesis and transport, cellulose production, fimbriae, regulators and surface structures was up-regulated after contact with plants (Barak et al. 2005; Zaragoza, Noel and Teplitski 2012). Even though, the interactions between tomatoes and Salmonella were the topic of several publications so far, many of those publications focused on the interactions between bacteria and the tomato fruits, which is indeed the most important issue in the view of the consumers. This study, however, focused on interactions occurring before the bacteria reach the fruit tissues, in the soil and especially in the rhizosphere as well as the plant phyllosphere, as sites of first interaction between the two organisms. Both important ecological niches allow the adaptation and may grant the passage to the fruits in a pre-harvest colonization. Our goal in the present study was to test the hypothesis that Salmonella strains vary in their ability to persist inside tomato plants and to investigate to what extent the manner of contamination would have an impact on the colonization outcome and the translocation to the non-infected plant parts, to this end we used both, qualitative and quantitative methods. For this, we used S. Typhimurium 14028s as a reference strain, while S. Typhimurium LT2 was used because of its inability to elicit a sustained acid tolerance response due to the low level of rpoS expression (Lee et al. 1995), which could be relevant in plant apoplastic environment. On the other hand, Salmonella Senftenberg, a strain that was originally isolated from basil (Pezzoli et al. 2008), was used in our study as a plant-originated strain. The colonization pattern was assessed using GFP-expressing bacteria and different bacterial densities. The transcriptome of Salmonella exposed to tomato root exudates and tomato-based medium was compared to the transcriptome profile in minimal medium (MM), in order to assess the potential adaptation to a plant-related environment. In addition, we monitored the transcriptional response of the tomato plant to different Salmonella strains. Our results indicate that both organisms actively engage into the interaction and that Salmonella adapts to the plant environment.
MATERIALS AND METHODS
Bacterial strains, culture conditions and media preparation
The rifampicin resistant mutants of Salmonella enterica serovar Typhimurium strains 14028s (S. Typhimurium 14028s) and LT2 (S. Typhimurium LT2), as well as S. enterica serovar Senftenberg (S. Senftenberg) used in this study, were previously described in Fornefeld et al. (2017). Bacteria were grown in Luria-Bertani (LB) broth (Carl Roth GmbH & Co., KG, Karlsruhe, Germany) or on LB agar. The plant-based medium was prepared as described by Fornefeld et al. (2017) with some modifications. Tomato medium (TM) contained 25% (v/v) tomato leaf extract (cultivar Money Maker) and 1 × M9 salts (5x concentrated) (Sigma-Aldrich Chemie GmbH, München, Germany) and 55% sterile deionized water in addition to 1% agar (if required). Tomato root (cultivar Money Maker) exudates were collected according to Witzel et al. (2017). Briefly, roots of plants were dipped in sterile distilled water for 1 hour and then transferred to fresh-distilled water for another 4 hours. The exudates from approximately 25 plants were pooled into a single sample. The medium was filtered through a cellulose ester membrane filter (pore size 0.22 μm, Carl Roth, Germany) to remove any cellular debris and microbes and concentrated 10-fold by freeze-drying. The lyophilized root exudates were re-suspended in 30 mL of 10 mM MgCl2 before use. In some experiments, a MM was used as a control. The MM consisted of 1 × M9 salts (5x concentrated), 2 mM MgSO4 and 1.23 mM glucose in sterile-deionized water. In all experiments, Xylose–Lysine–Desoxycholate (XLD) agar (Carl Roth GmbH & Co., KG, Karlsruhe, Germany) was used as a selective medium for Salmonella. Antibiotics were used at the following concentrations: rifampicin 50 mg L−1 (S. Typhimurium 14028s and LT2 and S. Senftenberg), gentamicin 10 mg L−1 and streptomycin 50 mg L−1 (Escherichia coli pSM1890), and trimethoprim 20 mg L−1 (E. coli R751). Bacteria were incubated overnight on LB and XLD agar at 37°C or 28°C, while on plant-based media at 25°C–28°C. All bacterial strains used in this study are listed in Table S1 (Supporting Information).
Preparation of GFP-expressing Salmonella
To visualize the colonization of tomato plants, S. Typhimurium 14028s, S. Typhimurium LT2 and S. Senftenberg were GFP-labeled with the plasmid pSM1890 GFP (Haagensen et al. 2002) as described in Jechalke et al. (2019). In brief, a triparental mating was performed between Salmonella strains and E. coli carrying the gfp IncQ plasmid pSM1890 GFP (derived from the IncQ plasmid pIE723 (Richter and Smalla 2007)) and E. coli carrying the helper plasmid R751 (Thorsted et al. 1998). Strains were cultured on LB plates containing the respective antibiotics, then harvested and re-suspended in 1 mL of 10 mM MgCl2 solution. Cell suspensions were combined, mixed and centrifuged (4000 × g, 3 min). After re-suspension in the residual supernatant, cells were placed on a filter disc (0.22 µm, Durapore membrane filters, Merck, Darmstadt, Germany) on LB agar and incubated overnight at 28°C. Cells were re-suspended from the filter by vortexing in 5 mL 10 mM MgCl2 solution. Transconjugants were selected by plating serial dilutions on LB plates containing respective antibiotics and incubated at 28°C. After overnight incubation, green fluorescent colonies were picked and plated on XLD agar and subsequently incubated overnight at 37°C.
Plant cultivation
Tomato (Solanum lycopersicum cultivar Money Maker) seeds were surface sterilized with 70% ethanol for 1 min followed by incubation in 3% sodium hypochlorite (NaClO) solution for 3 min, then vigorously washed with sterile-distilled water. Seeds were germinated for 1 week in Petri dishes on sterile ¼-strength Murashige and Skoog (MS) agar medium (Sigma-Aldrich Chemie GmbH, München, Germany), pH 5.4 including vitamins and 5 g L−1 sucrose. Seedlings were grown under sterile conditions with a light intensity of 150 µmol m2 s−1 (16 hour photoperiod) at 22°C for either additional 2 weeks in sterile glass pots (for spray inoculation) or for 2 days in 15 mL conical tubes, containing 10 mL ¼ MS liquid medium (for CLSM). To evaluate the survival rate of Salmonella inside the plant leaves, tomato plants were cultivated in greenhouse conditions in standard bedding substrate (substrate 1 Klasmann-Deilmann GmbH, Geeste, Germany) at 22°C and 16 hour photoperiod for 4–5 weeks. The plants were watered as needed and special care was taken to avoid washing off bacteria from the leaves. In addition, soil-grown plants were spray inoculated with GFP-tagged strains (for CLSM).
Salmonella persistence in soil and translocation to the phyllosphere
Soil (a standard greenhouse substrate) was inoculated with S. Typhimurium 14028s (106 colony forming units (CFU) g−1 dry soil) and tomatoes were planted at the day of inoculation (day zero). Soil samples were mixed thoroughly before sampling and 1 g of soil was transferred to 50 mL conical tubes, 9 mL 10 mM MgCl2 solution was added to the soil and mixed by vortexing for 1 min. Duplicates of serial dilutions were dropped (10 µL) in two technical replicates on XLD agar containing respective antibiotics. CFUs were enumerated after overnight incubation at 37°C. Additionally, 100 µL and 1000 µL were spread on XLD agar when a low concentration of Salmonella was expected. CFUs were calculated per gram dry soil. All experiments were performed with four technical replicates. Tukey–Kramer Grouping for Group Least Square Means (alpha = 0.05) was performed to test for significant differences. Presence of Salmonella in the phyllosphere was determined using a modified ISO 6579-1 norm for Salmonella detection. The vegetative part was sampled and cut with sterile scissors in 50 mL conical tubes, 10 mL of buffered peptone water (BPW) (Carl Roth GmbH & Co. KG, Karlsruhe, Germany) was added thereafter. After incubation at 37°C overnight under shaking conditions (140 rpm), 10 µL of the suspension was transferred to 190 µL Rappaport Vassiliadis Broth (RVS) (Carl Roth GmbH & Co. KG, Karlsruhe, Germany) and incubated overnight at 42°C. From both enrichments (BPW and RVS), 10 µL were dropped on XLD plates containing respective antibiotics and incubated overnight at 37°C to confirm the presence of Salmonella. BPW with and without Salmonella was used as positive and negative controls, respectively, and incubated at 37°C overnight with shaking. A total of 29 plants were tested per data point.
Persistence and translocation of Salmonella within the plant
To assess the extent of plant colonization by Salmonella, tomato plants were grown for 4–5 weeks in standard bedding substrate and subsequently inoculated with S. Typhimurium strains 14028s and LT2, and S. Senftenberg using syringe infiltration. Alternatively, the impact of the inoculation method on Salmonella persistence was investigated using a dipping method. Here, the leaves were dipped in solution containing Salmonella. Bacteria were pre-cultured on plant-based medium to allow them to adapt to the plant environment. To avoid contamination of the entire plant, the non-inoculated parts of the plants were covered with plastic bags until the inoculated parts became dry. The bacterial suspension was adjusted to a density of 0.01 at OD600nm (107 CFU mL−1) in 10 mM MgCl2. As a control, 10 mM MgCl2 without bacteria was used in addition to non-treated plants (NT). Leaves were sampled after 3 hour (0 day), 7 and 14 days post-inoculation (dpi), cut with sterile scissors and 5 mm leaf discs were obtained using a sterile biopsy bunch (Smithline Beecham Ltd, UK). The plant materials were homogenized in 1 mL 10 mM MgCl2 using a homogenizer (Xenox, Götze, Berlin, Germany). Serial dilutions were prepared in duplicates and 10 µL of each dilution was dropped on XLD agar plates to count the CFUs after overnight incubation at 37°C. The experiment was performed with three (syringe infiltration) or four replicates (dipping). Three plants per replicate (syringe infiltration) and four plants per replicate (dipping) were taken randomly per data point with technical duplicates. Student's t-test was applied and P < 0.05 was considered significant. No colonies were found in the negative control and MgCl2-treated plants.
Similarly, both inoculation methods were conducted to investigate their impact on Salmonella translocation to the non-inoculated parts. Hence, tomato leaves were inoculated, by infiltration or by dipping, with S. Typhimurium strains 14028s and LT2 and S. Senftenberg at a density of 0.01 at OD600nm (107 CFU mL−1). After 5 hours (0 day), 7 and 14 dpi the non-inoculated leaves were cut with sterile scissors and merged in 5 mL BPW in conical tubes and incubated overnight at 37°C. Next day, 10 µL of the previous culture were dropped on XLD agar plates and another 10 µL were transferred to 190 µL RVS medium. RVS culture was incubated overnight at 42°C and subsequently 10 µL were dropped on XLD agar plates. Both XLD plates were incubated overnight at 37°C to confirm the presence of Salmonella. Concurrently, the same steps were performed with the infected leaves as a positive control. The experiment was performed in 7 replicates (one leaf per plant and replicate) (infiltration) and 8 replicates (one leaf per plant and replicate) (dipping).
Confocal laser scanning microscopy (CLSM)
To visualize the colonization patterns of Salmonella, tomato plants were preliminarily grown under sterile conditions. One-week-old plants were transferred to conical tubes containing ¼-strength MS medium. Following an adaptation of the plants for 24 hours, the medium was inoculated with a final cell number of 108 CFU mL−1 of each: S. Typhimurium 14028s-GFP, S. Typhimurium LT2-GFP and S. Senftenberg-GFP, separately. The plants were sampled, stained with propidium iodide (PI) solution (1 µg mL−1) for 5–10 min and subsequently mounted on a microscope slide in 4′, 6-diamidine-2′-phenylindole dihydrochloride (DAPI) solution (10 µg mL−1). In order to picture the bacterial internalization through leaf surfaces, mature tomato leaves from plants grown on soil in the greenhouse were spray-inoculated and analyzed at 6 dpi. Small squares of the leaf were cut with a sterile scalpel and stained with PI and DAPI as described above. Additionally, an experiment was conducted to investigate whether the pattern of colonization is similar while using different bacterial number. Hence, tomato plants grown under sterile condition were transferred to conical tubes containing ¼-strength MS medium and inoculated with 108, 106, 104 and 102 CFU mL−1 of S. Typhimurium 14028s-GFP. Plants were sampled at 48 hours post-inoculation (hpi) and stained as mentioned above. Confocal laser scanning microscopy was performed using SP8 microscope (Leica Microsystems, Wetzlar, Germany) with excitation 405 nm, emission 430–480 nm (blue), excitation 488 nm, emission 500–550 nm (green), excitation 561 nm, emission 600–680 nm (red) including autofluorescence of chloroplasts.
Quantitative real time PCR analysis
In order to assess the response of tomato to Salmonella presence, S. Typhimurium strains 14028s and LT2, and S. Senftenberg were applied via spray-inoculation on three-week-old tomato plants grown under sterile condition on ¼-strength MS agar medium. Bacteria were grown one day before the inoculation on plant-based medium agar plates at 25–28°C. Plants were spray-inoculated in nine replicates with bacteria suspended in 10 mM MgCl2 at a density of 0.1 OD600nm (108 CFU mL−1). Leaves were sampled at 0 and 24 hpi. Samples were pooled from three plants per treatment. About 100 mg of leaf tissue was homogenized in a TissueLyser (Qiagen, Hilden, Germany) and the total RNA was extracted using TRIzol Reagent (Invitrogen, Darmstadt, Germany) according to the manufacturer protocol. DNase treatments followed by cDNA synthesis were performed from 2 µg of total RNA using qScript cDNA Super Mix kit (Quanta BioSciences, Gaithersburg, MD, USA). Target cDNA was then amplified in 20 µL reaction mixture containing 5 µL of sample DNA and LUNA Master Mix (New England Biolabs, Frankfurt, Germany) according to the manufacturer protocol. Reactions were run for 5 min at 95°C and 40 cycles of 30 s at 95°C, 30 s at 58°C and 60 s at 72°C in the CFX connect System (Bio-Rad, München, Germany). The primers used for the qPCR are listed in Table S2 (Supporting Information). Relative gene expression was normalized to the expression of the Actin gene. Student's t-test was applied and P < 0.05 was considered significant.
Salmonella transcriptome analysis
To determine the transcriptional profiles of S. Typhimurium 14028s in different tomato-related environments, an additional experiment was performed according to Jechalke et al. (2019). Briefly, Salmonella was prepared to a final OD600nm of 1.0 and 2 mL of this suspension was pipetted into cellulose ester dialysis tubes with a pore size of 100 kD (Spectrum Europe, Breda, The Netherlands). The dialysis tubes were knotted at one end and subsequently the other end using dental floss and placed into 50 mL conical tubes containing 30 mL of either MM, TM or medium based on tomato root exudates (TE). All treatments were performed in triplicates. The conical tubes were incubated at 28°C for 24 hours while shaking at 180 rpm. Afterwards, samples from each dialysis tube were mixed with RNAprotect (QIAGEN, Hilden, Germany) and the total RNA from the bacterial samples was extracted using the RNeasy Mini Kit (QIAGEN, Hilden, Germany) according to the manufacturer instructions. Covaris S220 focused-ultra sonicator (Covaris, Wolburn, MA, USA) (180s, 175 W peak incident power, 10% Duty Factor, 200 cycles per burst) was used for RNA fragmentation. Illumina HiSeq sequencing was performed at the ZIEL—Institute for Food & Health, Core Facility Microbiome/NGS, Technische Universität München, Freising, Germany. The library preparation was performed as described in Jechalke et al. (2019) and the significant differences between transcriptional profiles of S. Typhimurium 14028s exposed to TM, TE and to MM were calculated as false discovery rate (FDR)—adjusted P-values of the test statistic (q-value). Values differing by more than 2-fold change and q-values < 0.05 were considered significant. Significantly enriched GO terms among the significantly up-regulated genes were identified using the web-based tool PANTHER (http://geneontology.org). The Euler diagrams were created using the R package Eulerr. The raw sequences were deposited in the Gene Expression Omnibus database (NCBI) with the accession numbers GSE132634 (GSM3884784 -GSM3884786 for MM, GSM3497449-GSM3497451 for TE and GSM3497455-GSM3497457 for TM).
RESULTS
Salmonella persists in soil and is able to colonize the phyllosphere via the root system
Soil is one of the potential contamination sources with Salmonella in food production systems. Soil might be easily contaminated through irrigation water or organic fertilizers. In a first step, we wondered whether Salmonella can persist in soil and if so, whether this allows the bacteria to colonize the plants through the root system. To answer those questions, the soil was inoculated with the reference strain S. enterica serovar Typhimurium strain 14028s (S. Typhimurium 14028s; 106 CFU g−1 dry soil) and pre-grown tomato plants were transferred to the soil at the day of inoculation (day zero). The persistence of Salmonella in soil was monitored thereafter for 49 days (Fig. 1A). Salmonella Typhimurium 14028s persisted in soil throughout the growing period. To assess whether Salmonella is able to colonize the plant and to translocate between the tissues, we tested to which extent the plant phyllosphere can be colonized by bacteria present in the soil. Growing tomato plants on Salmonella-contaminated soil revealed that between 4% and 13% of the plants were colonized and Salmonella was detected in the upper phyllosphere (Fig. 1B). Our next step was to gain more knowledge about the persistence and translocation of Salmonella inside the plant.
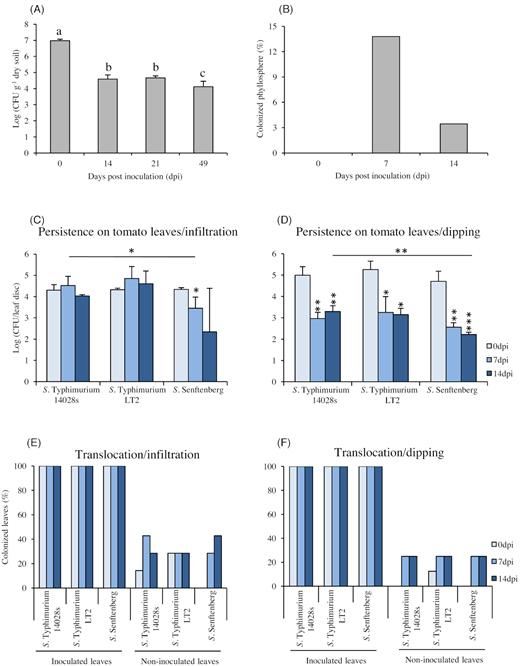
Salmonella persistence and translocation in soil, phyllosphere, and leaves of tomato plants. Salmonella enterica serovar Typhimurium strain 14028s (S. Typhimurium 14028s) was inoculated into the soil (106 CFU g−1 dry soil) and tomatoes were planted after inoculation (day zero). The persistence in soil was monitored during the entire experiment (A) and the presence of Salmonella in phyllosphere (shoots) was determined using a modified ISO 6579–1 norm for Salmonella detection. Data in (A) represent the mean of four technical replicates, the Tukey–Kramer grouping test for group least square means (alpha = 0.05) was performed to test for significant differences; in (B), percentage of Salmonella positively tested plants, n = 29 plants per data point. Persistence of Salmonella inside the leaves was monitored over a period of 14 days after infiltration (C), or dipping (D), inoculation methods. After inoculation of two leaves per plant and time point with (107 CFU mL−1) bacterial suspension, leaf discs were homogenized in 10 mM MgCl2 and serial dilutions were dropped on XLD plates to enumerate CFU counts. The data in (C) and (D) represent the mean of three and four replicates, respectively. Error bars indicate SD and * represents P < 0.05, ** represents P < 0.005 and *** represents P < 0.0005 in Student's t-test, compared to either 0 hpi or S. Typhimurium 14028s. The graph represents the translocation efficiency after infiltration (E), or dipping (F), inoculation, as revealed after enrichment in RVS medium. The bars represent the percentage of Salmonella positively tested plants from an average of 7 replicates (E) and 8 replicates (F), respectively (one leaf per plant and replicate).
The impact of inoculation manner and Salmonella genetic background on the persistence inside the plant
Based on the results obtained from the previous experiment, we raised two questions: (i) To what extent different Salmonella strains are able to survive inside the plant and whether various strains would differ in persistence; (ii) Does the entry route into a plant production system influence the Salmonella survival. Consequently, greenhouse-grown tomato plants were syringe-infiltrated, this inoculation simulated leaf colonization via the root system, with the Salmonella strains: S. Typhimurium 14028s (the reference strain), S. Typhimurium LT2 (the attenuated virulence strain) and S. Senftenberg (originally isolated from basil). As shown in Fig. 1C, all Salmonella strains were able to persist inside the plant leaves up to 14 days post-inoculation (dpi) without showing an obvious impact on the plant phenotype (Fig. S3, Supporting Information). Nevertheless, different patterns of persistence were observed for the various strains. Salmonella Typhimurium strain 14028s and LT2 had similar persistence levels, while the S. Senftenberg strain showed significantly lower persistence (Fig. 1C). On the other hand, by using the dipping inoculation method, which simulated the contamination via an overhead irrigation, all tested strains showed significant reduction in the CFU counts after 7 days (Fig. 1D). Even though, all strains exhibited a drop in CFU number at the beginning, the CFU counts for the S. Senftenberg strain dropped even lower at later time points, similarly to the previous observations. These results indicate that the inoculation method has no impact on Salmonella persistence. Additionally, different strains may differently persist within or on tomato plants.
These results raised the question, whether the inoculation method would have an impact on the effectiveness of Salmonella translocation into the non-inoculated parts of the plant. Hence, another experiment was designed to test how Salmonella translocates within the plant after either infiltration or dipping inoculation. Plants grown under greenhouse conditions were inoculated as described above and both the inoculated and non-inoculated leaves were sampled and tested for the presence of Salmonella using enrichment in BPW and RVS media. Comparing the translocation after infiltration (Fig. 1E) to that after the dipping inoculation method (Fig. 1F), it seems that infiltration may have an impact on the translocation of S. Typhimurium 14028s only during the first day after inoculation, in which Salmonella was detected in part of the non-inoculated leaves. This impact, however, seems to be restricted only to the first observed time point. After 7 or even 14 days, Salmonella strains behave similarly, regardless the used inoculation method and colonized about 20% of the non-inoculated leaves (Fig. 1E–F).
Salmonella strains colonize different plant tissues
The site of colonization and its pattern may influence the bacterial ability to establish in a new environment. We, therefore, examined the colonization site of S. Typhimurium 14028s on tomato plants grown under sterile conditions using green fluorescent protein (GFP)-expressing bacteria and confocal laser scanning microscopy (CLSM). We found that 2 hours post-inoculation (hpi), S. Typhimurium 14028s cells were attached to root hairs (Fig. 2A and B). Remarkable was the following colonization of roots by Salmonella and its pattern (Fig. 2C and D). Here, the bacteria seem to form micro-colonies. In addition, Salmonella was observed in the cavity between the primary and the emerging secondary root (Fig. 2E and F). Leaves are another entry point into plant tissues for many pathogens, including human pathogens. While examining tomato leaves 2 days after inoculation with the GFP-expressing S. Typhimurium 14028s, we detected Salmonella cells attached to the cuticle as well as inside the parenchyma tissue and within the stomatal cavities (Fig. 3A–D and Movie S5, Supporting Information). Additionally, Salmonella was observed inside hydathodes (Fig. 3E and F).
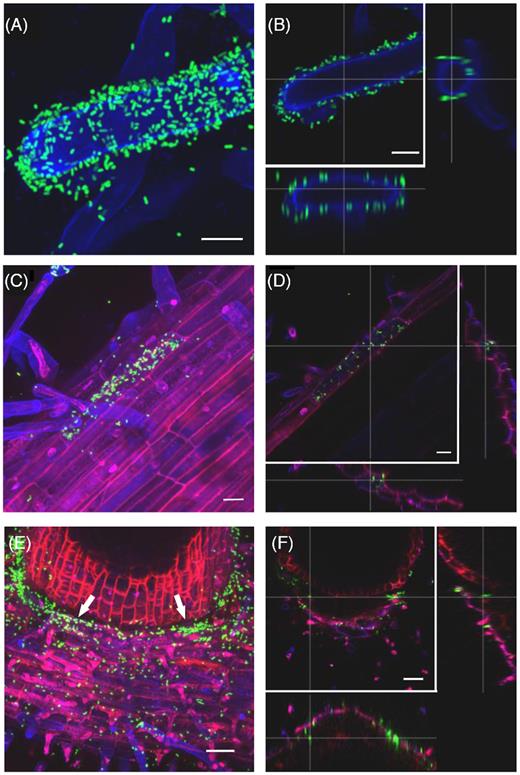
Salmonella Typhimurium 14028s colonizes tomato root hairs, primary and secondary roots in a particular pattern. Maximum projection images (A, C, E), and orthogonal scaling (B, D, F), show Salmonella enterica serovar Typhimurium strain 14028s expressing the green fluorescent protein (GFP) (S. Typhimurium 14028s-GFP) (in green) on different root structures. Root hair surfaces (A, B) are shown in blue. The scale bars indicate 10 µm. Root surface and epidermal cells (C, D) are shown in blue, cell walls in red. Scale bars indicate 20 µm. The cavity between primary (bottom) and secondary (top) root (arrows) is shown in (E, F). Scale bars indicate 50 µm. The root colonization patterns by S. Typhimurium LT2 and S. Senftenberg are shown in Fig. S4 (Supporting Information). Cross lines in orthogonal scaling are pointing to corresponding areas.
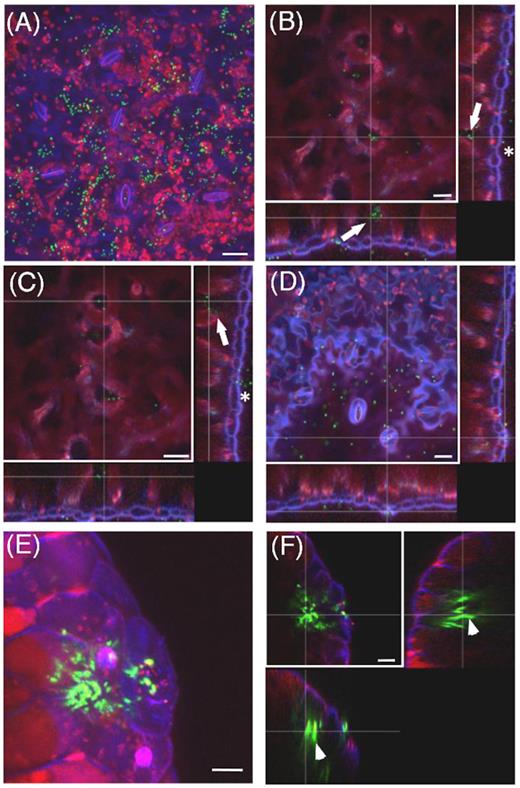
Salmonella Typhimurium 14028s colonizes tomato leaves. Maximum projection image (A), and orthogonal scaling (B–D), show stomata openings and cuticle in blue, auto fluorescence of chloroplasts in red and GFP-labeled Salmonella (S. Typhimurium 14028s-GFP) cells in green. Orthogonal scaling (B–D) shows S. Typhimurium 14028s cells attached to the cuticle (asterisk) and also inside of spongy parenchyma and in stomata cavities (arrows). Scale bars indicate 20 µm. Maximum projection image (E), and orthogonal scaling (F), show hydathode opening, nucleus and cuticle in blue, auto fluorescence of chloroplasts in red and GFP-labeled Salmonella cells in green. Orthogonal scaling (F) shows S. Typhimurium 14028s cells inside the hydathodes (arrowheads). Scale bars indicate 10 µm. The 3D representation of region presented in (A) is shown in Movie S5 (Supporting Information). Leaf colonization patterns of S. Typhimurium LT2 and S. Senftenberg are shown in the Fig. S4 (Supporting Information). Cross lines in orthogonal scaling are pointing to corresponding areas.
In order to simulate the colonization in a plant production-related situation, we assessed the colonization patterns on tomato plants grown on standard substrate soil under greenhouse conditions. In this case, the presence of GFP-expressing Salmonella was observed 6 days after the spray-inoculation. Interestingly, the observed colonization patterns (Fig. 4) were similar to those observed on in vitro grown plants (Fig. 3). Salmonella was present throughout the parenchyma tissue, especially inside hydathodes and below stomata openings (Fig. 4 and Movie S6, Supporting Information). These results suggest that the colonization is not coincidental. In order to investigate whether the pattern of colonization depends on bacterial density, we inoculated tomato plants with different concentrations of the GFP-expressing S. Typhimurium 14028s and assessed the colonization patterns after 48 hours. The analysis revealed similar pattern of colonization in leaves or roots, respectively, regardless of the bacterial density used to inoculate plants (Fig. 5).
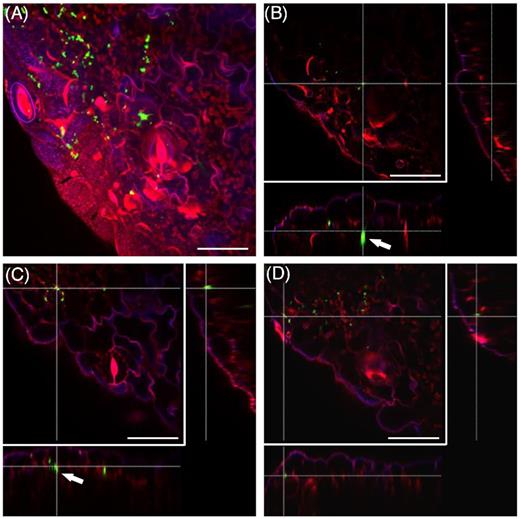
Salmonella Typhimurium 14028s colonizes stomata and hydathodes from soil-grown tomato plants. Maximum projection image (A), and orthogonal scaling (B–D), show stomata openings and cuticle in blue, auto fluorescence of chloroplasts in red and GFP-labeled Salmonella (S. Typhimurium 14028s-GFP) cells in green. Salmonella was found in the parenchyma tissue and cavities below the stomata (arrows). Scale bars indicate 30 µm. Cross lines in orthogonal scaling are pointing to corresponding areas. The 3D representation of the presented region is shown in Movie S6 (Supporting Information).
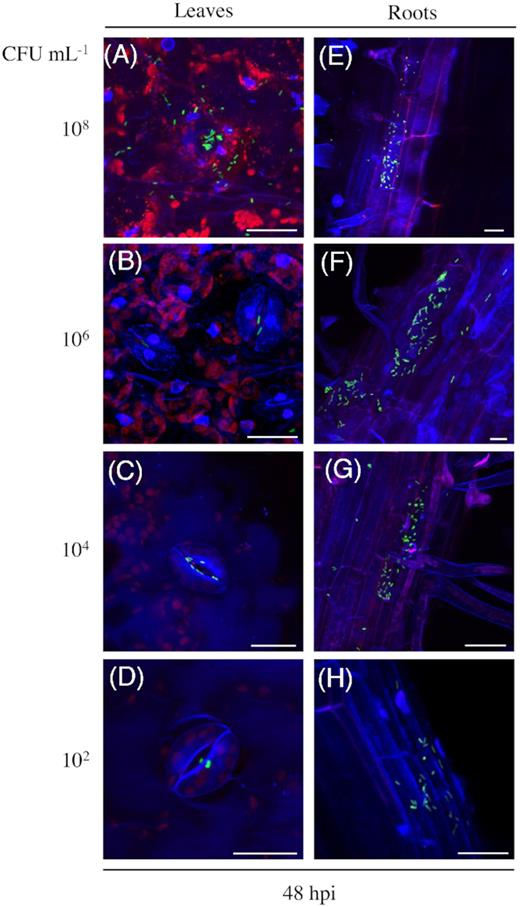
Colonization pattern does not depend on bacterial density. Maximum projection images (A-D), show stomata openings and cuticle in blue, auto fluorescence of chloroplasts in red and GFP-labeled Salmonella (S. Typhimurium 14028s-GFP) cells in green. Maximum projection images (E-H), show root surfaces and epidermal cells in blue, cell walls partially in red. Samples were examined 48 hours post-inoculation (hpi) with the indicated bacterial densities, colony forming units (CFU) mL−1. Scale bars indicate 10 µm.
In order to test whether S. Typhimurium LT2 and S. Senftenberg would colonize tomato plants in similar manner, we performed the experiment using GFP-expressing strains. The observed colonization pattern of S. Typhimurium LT2 on the root tissue and in the leaf parenchyma tissue as well as inside the stomatal cavities (Fig. S4A and B, Supporting Information) was similar to the patterns observed for S. Senftenberg (Fig. S4C and D, Supporting Information). Both strains exhibited colonization patterns similar to those observed for S. Typhimurium 14028s ( Fig. 3 and 4). This suggests that the three strains have similar colonization pattern, although they persist differently inside the plant.
Tomato plants respond to Salmonella colonization
Based on these results we wondered how tomato plants react to the presence of Salmonella. Thus, we monitored the gene expression patterns during the interaction with Salmonella. Tomato plants were grown for 3 weeks under sterile conditions and were spray-inoculated with the three Salmonella strains. We examined the transcriptional response of several plant defense-related genes: GlucA and GlucB (encoding for Glucanase A and B), CHI3 and CHI9 (encoding for Chitinase 3 and 9) and PR1A (Pathogenicity Related-1A). We observed that expression of those genes depends on the used Salmonella strain (Fig. 6). Comparing expression levels of tested genes in response to different Salmonella strains, we noticed that the induction was enhanced 24 hpi, when plants were inoculated with S. Typhimurium LT2 or S. Senftenberg, the response to S. Typhimurium LT2 seems to be the strongest (Fig. 6). This indicates the ability of the tomato plant to recognize and respond to the inoculation with Salmonella, particularly to the inoculation with the partially attenuated strain S. Typhimurium LT2. In contrary, S. Typhimurium 14028s, and to lesser extent S. Senftenberg, induced weaker transcriptional response of the plant.
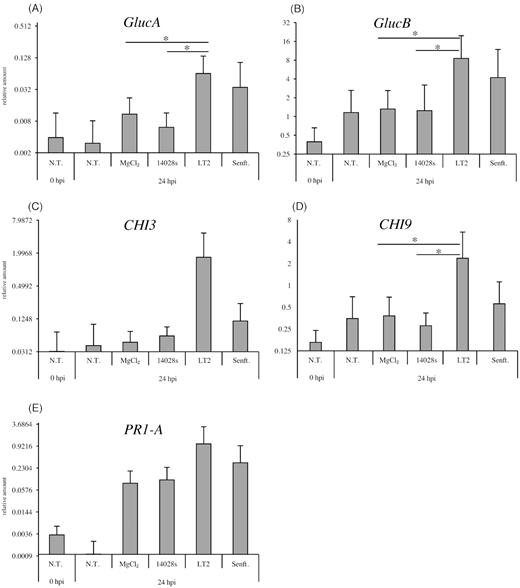
Transcriptional response of tomato to the different Salmonella strains. Tomato plants were grown in sterile ¼-strength MS medium for 3 weeks and subsequently spray-inoculated with 108 CFU mL−1 of Salmonella strains. In addition, non-treated (N.T.) plants and MgCl2 (mock)-treated plants were used as controls. The response was assessed 24 hours post-inoculation (hpi). The expression of Glucanase A and B (GlucA and GlucB) are shown in (A-B), the expression of Chitinase 3 and 9 (CHI3 and CHI9) in (C-D) and Pathogenicity Related 1-A (PR1-A) in (E). Relative expression levels were calculated after normalization with the expression level of the Actin gene, used as an internal control. Results represent means from nine independent experiments. Error bars indicate the SD. * represents P < 0.05 in Student's t-test.
Response of Salmonella to tomato environment
Aiming to test how well Salmonella adapts to the plant host and utilizes nutrients available in the plant environment, S. Typhimurium 14028s and LT2, as well as S. Senftenberg, were grown in liquid tomato-based medium (TM). LB medium was used as a control. All strains showed similar growth on LB medium (data not shown) as well as in TM, respectively (Fig. 7). This suggests that the plant-based medium supports the growth of the bacteria and that Salmonella can use plant material as a nutrient source.
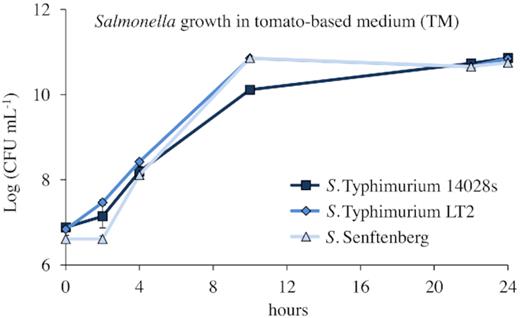
Growth of S. enterica strains in tomato medium. Pre-cultures of Salmonella were prepared in LB medium and incubated overnight at 37°C with shaking at 150 rpm. Cells were pelleted, washed and re-suspended in 10 mM MgCl2. The TM medium was inoculated with a bacterial solution adjusted to a density of 0.01 at OD600nm (107 CFU mL−1) and incubated at 28°C overnight with shaking at 150 rpm. Samples were taken at the indicated time, and serial dilutions were made using 10 mM MgCl2. Duplicated dilution series were dropped in technical duplicates on Xylose–Lysine–Desoxycholate (XLD) plates with respective antibiotics and CFUs were counted after overnight incubation at 37°C. Error bars represent SD from three replicates.
Considering that S. Typhimurium 14028s was, among the tested strains, the most successful in persistence inside the plant, this strain was used in the further experiment. To determine the adaptation of Salmonella to conditions in the rhizosphere and the phyllosphere of tomato plants, we monitored the transcriptional changes in response to the two plant-related media. Bacterial cells were exposed either to tomato root exudates-based medium (TE) or to TM and changes in gene expression were compared to Salmonella exposed to MM. In total, expression level of 598 and 151 genes was significantly up-regulated and down-regulated if compared to MM, respectively (Fig. 8, Table S7, Supporting Information). Regarding the genes that were significantly up-regulated in response to both plant media compared to MM, a GO-term enrichment analysis revealed two GO-categories: glycolytic processes, which are mostly related to ATP, and translation, which included peptide metabolism. Intriguingly, genes regulated only in response to TM belonged to the tRNA aminoacetylation and translation categories. While in response to TE, only genes belonging to the glyoxylate cycle were enriched. We detected no enriched GO-categories in genes, which were down-regulated in response to either TM or TE. List of differentially expressed genes and their functions is available in the supporting information (Table S8, Supporting Information). We detected different clusters of differentially expressed genes, including stress response-related genes, genes related to cell division as well as iron homeostasis. The indicated clusters were up-regulated in response to both TM and TE media. Other genes which were differentially expressed in response to both media were involved in O-antigen biosynthetic process, cellular homeostasis, regulators and ribosome binding. Furthermore, several genes were expressed in either TM or TE but not expressed in MM, resulting in ‘infinity’ expression values (inf. values), those genes are shown in Table S9 (Supporting Information). They cluster, among others, in a group related to biofilm and motility, and represent such genes as recA, which is involved in the positive chemotaxis and regulation of bacterial-type flagellum-dependent cell motility. This gene was regulated in response to TM together with yddX, which is involved in biofilm-dependent modulation. In addition, this gene cluster contains celC, which was regulated in response to TE and luxS that was regulated in response to both media. Other, previously mentioned gene clusters, such as amino acid- related and stress response-related as well as iron-related genes were also present in the group of genes expressed in the response to plant-related media. Furthermore, several genes which were not related to a specific cluster, such as, cell wall organization, translation, metal ion binding and genes involved in nitrogen compounds metabolic processes, all those genes are listed in the Table S9 (Supporting Information).
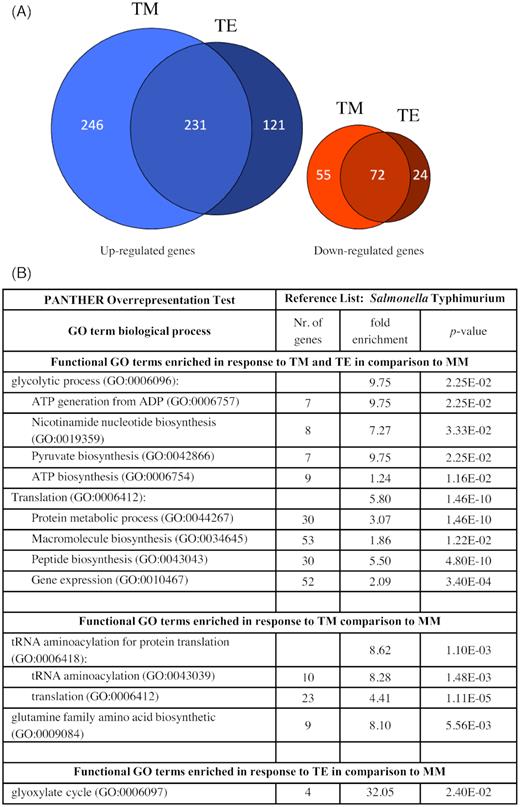
Transcriptional response of S. Typhimurium 14028s to tomato-related environment. Euler diagrams represent S. Typhimurium 14028s genes with up-regulated or down-regulated expression levels (A), in response to either tomato root exudates (TE) or tomato medium (TM) compared to expression levels in minimal medium (MM). Indicated are genes with at least two-fold higher or lower expression levels (P < 0.05). Included are also genes for which the expression was detected in only one of the treatments. Significantly enriched GO-terms of Salmonella genes that were enriched in their expression in TM and TE compared to MM, as a control, are shown in (B). Different clusters of genes and their respective P-values and corresponding functions are listed in the Tables S7–S9 (Supporting Information).
DISCUSSION
The link between salmonellosis outbreaks and fresh produce contaminated with Salmonella is nowadays widely accepted. Unfortunately, even though the number of registered disease cases is stable, the proportion of disease outbreaks associated to plant-originated products or raw plant produce increased in recent years (EFSA and ECDC 2017). Others and our study show the ability of S. Typhimurium to persist in the soil and within the tomato plants for a long period, increasing the potential risk of disease outbreaks. Additionally, when plants grew on contaminated soil, we detected the bacteria in the phyllosphere of a part of the tomato population. These results suggest that Salmonella translocates from the contaminated soil to the plant phyllosphere. Similar results were obtained in the case of lettuce (Lactuca sativa), corn salad (Valerianella locusta) and a leaf-to-fruit colonization in tomato (Gu et al. 2011; Jechalke et al. 2019), suggesting that Salmonella may use plant tissues as ecological niches and actively colonize the entire plant.
Our report shows that the colonization pattern of Salmonella on tomato plants was similar in both sterile- and greenhouse-grown tomato plants regardless the used inoculation method, the conditions of plant cultivation, the bacterial strain and the concentration of the bacteria. These observations indicate that the colonization is not an aleatory event. Salmonella was shown already to move towards open stomata, moreover, this movement was dependent on the photosynthetic activity of the leaves (Kroupitski et al. 2009). The movement of S. Typhimurium from inoculated leaves-to-fruits of tomato was also reported by (Gu et al. 2011). Similarly, some Salmonella serovars inoculated on tomato flowers were recovered from the tomato fruit (Shi et al. 2007). Our results also very strongly support previous observations, e.g. (Barak, Kramer and Hao 2011; Gu et al. 2011; Gu, Cevallos-Cevallos and van Bruggen 2013) suggesting that stomata and hydathodes are potential colonization sites.
In addition, differences in the persistence and translocation of different Salmonella strains could result from the inoculation method. Hence, we used also another inoculation method, dipping, where no force was applied. The results were, to some extent, similar in both methods, indicating that the inoculation method has no impact on Salmonella persistence and that the tested strains are able to survive in the plant environment for a long time. However, the inoculation method seems to have an impact on the translocation of S. Typhimurium 14028s during the early time of inoculation (5 hpi), where the non-inoculated parts were colonized if force was applied during the inoculation (infiltration).
It is important to mention that Salmonella seems to colonize only part of the tomato population. Possible explanation might be individual differences in the strength of the plant defense mechanism. Since plants respond to human pathogens, it appears that some of the defense and hormone-related pathways (ethylene and jasmonic acid) are involved in the interaction between plants and Salmonella (Schikora et al. 2008; Schikora et al. 2011; Marvasi et al. 2014). Quite a lot of studies have indicated that plant hosts are able to recognize human pathogens and initiate defense mechanisms, e.g. the up-regulation of PR1 gene orthologs (Iniguez et al. 2005; Klerks et al. 2007). Numerous biotic factors are inducing the expression of chitinases, which are involved in defense responses against phytopathogens (Kasprzewska 2003; Saker et al. 2008; Maruthachalam et al. 2011). Constitutive expression of both tobacco glucanase and chitinase genes led to enhanced resistance against fungal pathogens in tomatoes (Jongedijk et al. 1995). In our study, we tested the expression levels of PR1, Glucanases (GlucA and GlucB) and Chitinases (CHI3 and CHI9). All tested genes showed a higher response to inoculation with S. Typhimurium LT2. Whether the observed increase in the expression of plant defense-related genes after contact with S. Typhimurium LT2 is linked to the attenuated expression of rpoS (Lee et al. 1995) is a very intriguing question and requires further investigation. The relatively low gene expression level 24 hours after inoculation with S. Typhimurium 14028s could be due to different reasons, the virulence factors of this strain, e.g. effector proteins, could be one of them, the different response time another.
Similar to the interaction with plant pathogens, interaction between Salmonella and tomato depends not only on the plant factors but also on the properties of the bacterial strains (Marvasi et al. 2013). For instance, infiltration with S. Senftenberg, among other strains, that belong to the serogroup E4 (O: 1, 3, 19) possessing the O-antigen resulted in rapid wilting and chlorosis in Arabidopsis leaves. In contrast, infiltration with the same strains lacking the O-antigen did not provoke any symptoms (Berger et al. 2011). In our study, the inoculated tomato leaves showed however no changes in their phenotype. Correspondingly, another report indicated the missing disease symptoms on tomatoes that were inoculated with Salmonella (Gu et al. 2011). Our results revealed that all tested serovars persisted in the plant environment during the tested period (14 days) although, S. Senftenberg showed significantly lower CFU counts in comparison to S. Typhimurium 14028s or S. Typhimurium LT2 at the later stages. This raises the question whether S. Senftenberg is less adapted to tomatoes or whether the plant is reacting differently to S. Senftenberg? Previous reports suggested that S. Senftenberg is only partially recognized by the plant immune system, due to its specific flagellin sequence (Garcia et al. 2014). How well the LeFLS2 receptor recognizes the S. Senftenberg flagellin and which proportion this recognition takes in the response to S. Senftenberg in tomatoes is currently unknown.
In order to reveal the adaptation to plant-related environment, we assessed the transcriptional profile in response to root exudates and to plant-based medium. Very interesting was the up-regulation of genes related to amino acid synthesis and glyoxylate pathway in response to TM or TE media, respectively. Both patterns suggest rather nutrient-poor environment for Salmonella. However, the up-regulation of the glyoxylate pathway in response to TE may be correlated with the presence of sugars, among others, in the root exudates. The glyoxylate cycle is known to be conserved in protists, archaea, plants, bacteria, fungi and nematodes but is absent in mammals (Chew et al. 2019). Additionally, the glyoxylate cycle was reported to be up-regulated in response to oxidative stress and plays an important role in pathogenesis of P. aeruginosa in humans (Ahn et al. 2016). Interestingly, a recent study on the response of Salmonella to cabbage leaves revealed a particular pattern. Although genes associated with energy production, amino acid metabolism and motility were differentially regulated in this system. The study revealed also up-regulated SPI-1-associated genes and down-regulated SPI-2-associated genes when Salmonella was in contact with cabbage (Lee et al. 2018). None of those genes was differentially expressed in response to tomato-based media in our study, implying differences in plants and/or experimental systems and maybe different colonization strategies used by Salmonella in different plants. Together with other reports, this might explain a certain overlap between the invasion strategies in animal and plant hosts (Goudeau et al. 2013). Our results showed up- and down-regulation of several genes, which may be involved in Salmonella interactions with animals. An example of these genes is cigR, a known T3SS-2 effector. Product of this gene was suggested to play a role in the biofilm formation and have a negative impact on Salmonella virulence in the mammalian system (Yin et al. 2016). This gene was significantly up-regulated in response to TM but down-regulated in response to TE. Another example is the rpoS which was significantly up- regulated in response to both media. This gene is a very well-known master regulator of the stationary phase and a general stress response. In addition, rpoS is required for virulence of S. Typhimurium in the mammalian system (Coynault, Robbe-Saule and Norel 1996; Nickerson and Curtiss 1997). The up-regulation of this gene in S. Typhimurium 14028s makes it very interesting especially, if compared to its low expression in the S. Typhimurium LT2 strain. Moreover, rpoS was recently reported to be up-regulated also in response to medium based on lettuce root exudates (Jechalke et al. 2019).
Our data revealed also significant up-regulation of genes, such as glgP and glgX. Both are known to be involved in the glycogen degradation process. This indicates the importance of sugar metabolism in plant media and the necessity of the carbon and energy source that would be acquired from glycogen degradation (Wilson et al. 2010). glgS is another gene that raised our attention since it was significantly down-regulated in response to TM. This gene is involved in the negative regulation of motility, adhesion and biofilm exopolysaccharides synthesis. Another example is feoB that is known as ferrous transporter (Kim, Lee and Shin 2013) and was significantly up-regulated in response to TM (this study). Finally, celC was up-regulated in response to TE, which was in accordance to the results of a previous study with lettuce-based media (Jechalke et al. 2019), celC encodes gene similar to E. coli's phosphoenolpyruvate-dependent sugar phosphotransferase system and may be involved in polysaccharide catabolic processes. It is, howeve,r not yet clear, how well Salmonella may use plant cell wall polymers (e.g. cellulose) as a carbon source (Rodionov, Gelfand and Hugouvieux-Cotte-Pattat 2004; Abbott and Boraston 2007; Karmakar et al. 2018). Hence, it was suggested that Salmonella might be attracted to rather simple sugars and organic acid exudates in tomato plants (Ahmad et al. 2016). Interestingly, the amount of these metabolites differs among cultivars and depends also on the maturity stage of the fruit (Carrari et al. 2006; Mathieu et al. 2009). Composition of fruit exudates was shown to determine the outcome of the colonization, suggesting that their content determine the susceptibility of particular tomato cultivars to S. enterica (Han and Micallef 2014; Han and Micallef 2016).
The differential regulation and the type of genes regulated in response to different plants (tomatoes in our study and lettuce in Jechalke et al. (2019)) may indicate different strategies used by Salmonella. However, the similarities regarding the response of some genes highlight that similar genes may be of great importance for Salmonella in order to adapt to plant environment. Worth to mention is that the vast majority of differentially expressed genes in our study was also identified in the study from de Moraes et al. (2017). The authors screened a transposon insertion library to identify genes involved in the colonization of tomato fruits, highlighting the importance of genes identified in both studies for Salmonella, while in contact with tomato plants.
Taken together, our report strengthens the opinion that tomato plants are suitable hosts for Salmonella and that bacteria can persist and adjust to this environment. Different Salmonella strains however, seem to behave differently on or in tomatoes, as well as other crop plants. Consequently, strategies undertaken to prevent contamination of crop with human pathogens cannot be generalized and would require adaptation to particular plant species.
ACKNOWLEDGEMENTS
We thank Nicola Holden and John Coia for providing the Salmonella enterica serovar Senftenberg strain. The authors would like to thank Klaus Neuhaus for his support in the Salmonella RNA-Seq approach. We thank Lena Rauch for developing the minimal medium.
AUTHOR CONTRIBUTIONS
AZ, JS, SJ, RG, AS are responsible for the design and concept. AZ, JS, MB, JK, MG, SJ performed the experiments and laboratory work. SJ and JS processed the Salmonella transcriptome sequences. AZ, JS, SJ, RG, AS analyzed the data. AZ wrote the first draft of the manuscript. AZ, JS, SJ, RG, AS contributed to the manuscript revision.
FUNDING
AZ would like to thank the DAAD for the fellowship support. SJ and JS were funded by the Federal Office for Agriculture and Food (Bundesanstalt für Landwirtschaft und Ernährung, BLE), Grants 13HS026 and 13HS029.
Conflict of interest
The authors declare that the research was conducted in the absence of any commercial or financial relationships that could be construed as a potential conflict of interest.