-
PDF
- Split View
-
Views
-
Cite
Cite
Damaris Desgarennes, Etzel Garrido, Miryam J. Torres-Gomez, Juan J. Peña-Cabriales, Laila P. Partida-Martinez, Diazotrophic potential among bacterial communities associated with wild and cultivated Agave species, FEMS Microbiology Ecology, Volume 90, Issue 3, December 2014, Pages 844–857, https://doi.org/10.1111/1574-6941.12438
- Share Icon Share
Abstract
Agaves are major biotic resources in arid and semi-arid ecosystems. Despite their ecological, economical and cultural relevance, many aspects of the microbial communities associated with agaves are still unknown. Here, we investigated the bacterial communities associated with two Agave species by 16S rRNA- Denaturing gradient gel electrophoresis fingerprinting and sequencing. We also evaluated the effects of biotic and abiotic factors in the structure of the bacterial communities. In parallel, we isolated and characterized diazotrophic bacteria associated with agaves, as Agave soils are characterized by their low nitrogen content. Our results demonstrate that in Agave, the structure of prokaryotic assemblages was mostly influenced by the community group, where the soil, episphere, and endosphere were clearly distinct. Proteobacteria (γ and α), Actinobacteria, and Acidobacteria were the dominant phyla. Bacterial communities in the episphere of agaves were mainly influenced by the host species, whereas in the endosphere were affected by the season. Fifteen bacterial taxa were common and abundant in the endosphere of both Agave species during the dry season. Notably, some of the confirmed diazotrophic strains belonged to this group, suggesting a possible beneficial role in planta.
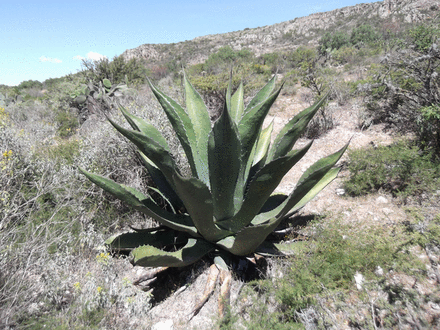
The structure of bacterial communities associated with Agave species is distinct among soil, episphere and endosphere, where diazotrophic bacteria may facilitate adaption of these crassulacean acid metabolism-plants to low-nutrient environments.
Introduction
Agaves are major biotic resources in arid and semi-arid ecosystems, where soils are characterized by limited moisture and low nutrient content. In Mexico, agaves are primarily used in the production of tequila and mezcal, but their potential as bioenergy stocks is currently being discussed (Garcia-Moya et al., 2011; Valenzuela, 2011). Agaves, as other succulents found in deserts, have developed morphological and biochemical strategies to cope with several months of extreme drought and high temperature (up to 61 °C) resulting in the crassulacean acid metabolism (CAM), a water-efficient form of photosynthesis, water-impermeable leaf cuticle waxes, and contractile roots (Nobel, 2003; Boom et al., 2005; Deshmukh et al., 2005; North et al., 2008). Little is known, however, about the associations agaves establish with microorganisms: which biotic and abiotic factors influence the most their bacterial communities and, ultimately, if these interactions affect the survival, performance, and adaptation of the agaves to their environment.
The association of plants with microorganisms is not only ancient [460 Myr in the case of the mycorrhizae (Remy et al., 1994; Redecker et al., 2000)], but has helped plants deal with different abiotic and biotic stresses like salt, drought, and nutrient limitation as well as from the attack of herbivores and pathogens (Arnold et al., 2003; Rodriguez et al., 2008, 2009; Partida-Martinez & Heil, 2011; Hardoim et al., 2012). Well-studied examples of plant–microorganism interactions include rhizobia, the endophytic bacteria of leguminous plants capable of nitrogen fixation (Kiers & Denison, 2008; Oldroyd & Downie, 2008; Martinez-Romero, 2009; Rogel et al., 2011); the vertical transmitted endophytic fungi of grasses, Epichlöe and Neotyphodium, which protect their hosts from herbivores and drought (Cheplick et al., 1989; Clay, 1990) and, arbuscular mycorrhizal fungi (AMF) which enhance nutrition and water transport in their host plants (Brundrett, 2002; Bonfante & Genre, 2008).
Previous studies performed with different Agave species have shown an enhanced capacity in nutrient and water uptake when plants are colonized by mycorrhizae (Cui & Nobel, 1992; Camargo-Ricalde et al., 2003; Pimienta-Barrios et al., 2009). Also, when plants established associations with endophytic fungi or diazotrophic bacteria, their photosynthetic efficiency, sugar content, and growth increased (Obledo et al., 2003; Ruiz et al., 2011). These studies suggest that the interactions agaves maintain with microorganisms play an important role in their health and productivity. Therefore, the goals of this study were the following. First, investigate the bacterial communities associated with wild Agave salmiana and cultivated A. tequilana. These two species grow in different habitats in Central Mexico. Agave salmiana can still be found in natural populations sharing their habitat with cacti and some other xerophytes (Martinez-Salvador et al., 2012), while A. tequilana has been used in the production of tequila since the late 17th century (Colunga-Garcia-Marin et al., 2007) and is now a genetic monoculture. These ecological differences might alter the bacterial communities associated with each species. Second, evaluate the effect of biotic (i.e. host species and community type) and abiotic factors (i.e. season and site) on the structure of the plant-associated bacterial communities. And third, isolate and characterize nitrogen-fixing bacteria associated with agaves to pave the way for increasing agaves' productivity based on the use of compatible bacterial symbionts.
Materials and methods
Study system
Our study system is constituted by two Agave species: Agave salmiana Ottto and Agave tequilana Weber. Although both CAM species are distributed in the Bajio region in central Mexico, they are not sympatric (Fig. 1a). Agave salmiana belongs to the group Salmianae (Gentry, 1982), and it is found in wild populations, while A. tequilana belongs to the group Rigidae (Gentry, 1982) and exists only in cultivated populations (Fig. 1b).
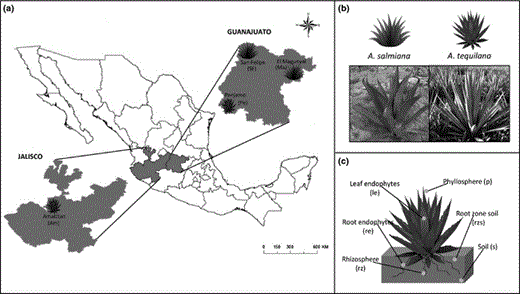
(a) Map showing the four sampling sites selected for this study. (b) Picture and diagram of Agave salmiana (wild) and Agave tequilana (cultivated). (c) The six samples analyzed from each plant.
Sample collection
In 2012, samples were collected at two different times: in late May (dry season D) and in early October (rainy season, R). Agave salmiana samples were collected from the natural populations of El Magueyal (Ma) and San Felipe (SF) (Fig. 1a). Agave tequilana samples were taken from two fields managed by tequila companies: Penjamo (Pe) and Amatitan (Am) (Fig. 1a). Soil characteristics as well as temperature and precipitation data for each site are presented in Table 1. For each species at each location, samples from three healthy plants were collected (Supporting Information, Table S1). Four samples per plant were collected to analyze six different communities (Fig. 1c): two leaves for leaf endosphere (le) and phyllosphere (p); root tissue for root endosphere (re) and rhizosphere (rz); root-zone soil (rzs) which was soil loosely attached to the roots, and bulk soil (s) gathered one meter away of the selected plants. In total, we obtained 144 samples (72 from each species). All samples were stored at 4 °C until processing.
Annual mean temperature, precipitation, and soil characteristics of study sites
Data provided by Comisión Nacional del Agua (CONAGUA), 2012.
Statistically significant difference after Kruskal–Wallis test (H3,32 = 10.445, P= 0.0151).
Statistically significant difference after Kruskal–Wallis test (H3,10 = 7.036, P= 0.0296).
Statistically significant difference after Kruskal–Wallis test (H3,10 = 6.873, P= 0.0329).
Annual mean temperature, precipitation, and soil characteristics of study sites
Data provided by Comisión Nacional del Agua (CONAGUA), 2012.
Statistically significant difference after Kruskal–Wallis test (H3,32 = 10.445, P= 0.0151).
Statistically significant difference after Kruskal–Wallis test (H3,10 = 7.036, P= 0.0296).
Statistically significant difference after Kruskal–Wallis test (H3,10 = 6.873, P= 0.0329).
Sample preparation and DNA extraction
To recover microorganisms from the phyllosphere (p) and rhizosphere (rz), leaf and root samples were washed in a flux cabinet with phyllosphere removal buffer (6.75 g KH2PO4, 8.75 g K2HPO4, and 1 mL Triton X-100 per liter) and a 0.9% NaCl solution, respectively. From these solutions, the microbial pellet was obtained by centrifugation and stored at −80 °C prior DNA extraction.
Leaf and root tissues were then disinfected placing them for two min in 70% ethanol, 10 min in 5% sodium hypochlorite, and then rinsed twice with sterile distilled water. To validate our disinfection process, 200 μL of the second rinse was poured into nutrient agar plates and no microbial growth was observed after several days of incubation. Afterward, using a sterilized blade, we made transverse cuts across the leaves and roots, resulting in 5 mm thick sections. 300 g each of leaf and root tissue were placed in sterile plastic bags and kept at −80 °C for further DNA extraction. These surface sterilized leaf and root tissues constituted the endophytic samples (le and re).
DNA was extracted following three different protocols depending on the sample type. Total DNA was extracted from 500 mg of leaf (le) and root (re) tissue with a CTAB-based extraction protocol, as described elsewhere (Edwards, 2001). In the case of phyllosphere (p) and rhizosphere (rz), DNA was extracted, cleaned, and concentrated with Ultra Clean® Water DNA Kit (MO BIO Laboratories, Carlsbad, CA), according to the manufacturer's protocol. Soil (s) and root-zone soil (rsz) DNA was extracted, cleaned, and concentrated from 500 mg of sample using the Power Soil® DNA Isolation Kit (MO BIO Laboratories), according to the manufacturer's protocol. Extracted DNA was quantified with a ND 1000 spectrophotometer (NanoDrop, Thermo Scientific, Wilmington, DE), and its integrity was confirmed by 1% agarose gel electrophoresis.
PCR amplification of 16S rRNA gene fragments
DNA from all samples was used as the template for a nested PCR, targeting first the complete 16S rRNA gene, and secondly, the V6–V8 hypervariable region (Lottmann et al., 2010). These PCRs were performed using primers and conditions as reported by Lottmann et al. (2010). To minimize heteroduplex formation, we reduced the number of cycles to 25 and 20 in the first and second reaction, respectively. Products were checked by electrophoresis in 1% agarose gels.
Denaturing gradient gel electrophoresis (DGGE) fingerprinting of 16S rRNA gene fragments
DGGE analysis was performed with the Dcode System (Bio-Rad Inc., Hercules, CA) based on the protocol of Costa et al. (2006). Previous to the analysis of all samples, reproducibility and validation of the 16S rRNA-DGGE fingerprinting was assessed. For this, 200 ng each of five independent samples from the leaf endosphere of agaves and a self-developed marker of the same amplicon from five known bacterial strains at five different concentrations (25, 50, 75, 100, and 150 ng) were loaded in two separate gels. Consistent quantification (band intensity) and migration (electrophoretic distance/relative front) patterns among samples revealed low intraspecific variability and reproducibility of the method. Once this was carried out, the fingerprinting of the 144 samples was performed in the same way placing the marker at each outer lane in every gel used (Fig. S1). Gels were stained with SYBR Gold (INVITROGEN), according to manufacturer's protocol. The ChemiDoc MP System (Bio-Rad Inc.) was used to capture gel images for further processing and analysis.
Cloning and sequencing
DGGE bands were excised from stained DGGE gels and eluted according to the protocol described by Lottmann et al. (2010). Two microliters of the resulting suspension were used in a PCR to re-amplify the excised 16S rRNA gene fragments (Lottmann et al., 2010). PCR products were ligated into a pJET 1.2 vector (Thermo Scientific) and transformed into competent Escherichia coli DH5α cells, following the manufacturer's protocol. Dilutions were plated onto LB agar plates with ampicillin (100 mg L−1) and incubated overnight at 37 °C. For each treatment, ten white colonies were randomly selected and cultured overnight at 37 °C in LB broth supplemented with ampicillin (100 mg L−1). We extracted plasmid DNA from selected colonies to check the clones for the correct size of inserts after PCR amplification with the primers pJET 1.2F and pJET 1.2R (Thermo Scientific) according to the manufacturer's protocol. Plasmid DNA from clones with correct inserts was sent for Sanger sequencing.
Bacterial isolation and characterization from Agave tissues
Based on the rationale that nitrogen is the most limiting macronutrient in Agave soils (Nobel, 2003), we pursued the isolation of native diazotrophic bacteria. For this, collected samples from the endosphere, rhizosphere, and phyllosphere from both agaves were used to inoculate Rennie and Winogradsky media (N-free media for diazotrophs; Winogradsky, 1935; Rennie, 1981). Bacterial colonies growing on these petri dishes were further diluted and inoculated in solid media until axenic cultures were obtained. Using microbiological methods (macro- and microscopic morphology, growing conditions, pH, oxygen requirements, etc.), 18 strains were identified as distinct. Further confirmation of possible nitrogenase activity was pursued by performing the acetylene reduction assay as described elsewhere (Hardy et al., 1968). Bacteria that were positive in the acetylene reduction assay were also tested for their ability to use fructans, the complex carbon polymers presented in Agave species, as the only carbon source (Mancilla-Margalli & Lopez, 2006; Ravenscroft et al., 2009; Mellado-Mojica & Lopez, 2012). The 18 bacterial strains were subsequently identified by amplifying and sequencing the complete 16S rRNA gene using the procedures described above. Finally, partial and full 16S rRNA gene sequences from isolated bacteria were compared to those obtained from DGGE bands using the cd-hit software (Li & Godzik, 2006; Huang et al., 2010).
Data analysis and statistical treatment of DGGE profiles
Representatives of each operational taxonomic unit (OTU) were cloned and sequenced as previously described. The sequences obtained were depurated, compared, and aligned with data from the silva database (Quast et al., 2013), the Ribosomal Database Project Release 10.32 (Maidak et al., 2001), and the 16S rRNA gene database from the NCBI using the blast algorithm (Altschul et al., 1990). From the DGGE profiles and their sequences, a total of 88 OTUs were determined. From these, 23 were identified as chloroplasts (23.1%) and thus were removed from our analyses. The sequences from the remaining 65 bacterial OTUs were checked for chimeras using the uchime software (Edgar et al., 2011). The sequences of these 65 bacterial OTUs and the 18 isolated bacterial strains were submitted to the NCBI under the accession numbers: KJ659221–KJ659285 and KM232684–KM232701, respectively.
Digitalized DGGE profiles were quantitatively analyzed with the image lab® 4.0 software (Bio-Rad Laboratories, CA). Each band was located and quantified relative to the marker on each gel (Fig. S1). After processing of all samples, a table recording the presence or absence of individual bands in each sample and their relative intensity was generated for different statistical analyses. This matrix was built under the assumptions that bands which shared the same migration pattern (electrophoretic distance/relative front) represented one OTU and that the band intensity densitometrically calculated with the help of the marker and the image lab® 4.0 software could be used as a proxy for the relative abundance of the specific OTU with respect to the others in each sample. Cured data were then log-transformed prior to the analyses. To estimate the dissimilarity between samples, we used the Bray–Curtis measure where the sum of differences between objects (i.e. samples) across variables (i.e. OTUs) is standardized by the sum of the variable values across objects, also summed across variables (Quinn & Keough, 2002). This measure ranges between zero (same variables and values in both objects – completely similar) and one (no variables in common – completely dissimilar), and it is well suited to species abundance data, because it ignores variables with zeros for both objects. The effect of the community type, Agave species, season, site, and all their interactions on the distance matrix was evaluated through a permutational multivariate analysis of variance (permanova; Anderson, 2001). Afterward, those factors with significant effects were plotted using a Kruskal's Nonmetric Multidimensional Scaling (nmds). The aim of this ordination method is to display the dissimilarities between the samples (i.e. objects) graphically, that is, the distance between the objects on the plot represent their relative dissimilarities considering the abundance of the OTUs they share. The measure of the goodness of fit of the final nmds plot is the stress value (S), which indicates the match between interobject distance and dissimilarity. The closest the S value to zero, the better the ordination (Manly, 1986; Quinn & Keough, 2002). All the analyses were performed in r (R Core Team, 2013) using the mass (Venables & Ripley, 2002) and vegan (Oksanen et al., 2013) packages.
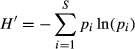

Results
Bacterial communities in wild and cultivated agaves
From the 65 bacterial OTUs identified in this study, 51 were present in both Agave species. That is, 78.5% of the DGGE detected taxa were shared among the two species. Only eleven and three OTUs were exclusively identified in A. salmiana and A. tequilana, respectively (Table S2). The phylum Proteobacteria was the most abundant in both Agave species, being the γ- and α-Proteobacteria the predominant classes (Fig. 2). Actinobacteria and Acidobacteria were the second and third more represented phyla, while Firmicutes, Nitrospirae, and Gemmatimonadetes represented together < 9% of the taxa in these agaves (Fig. 2). Sequences of OTUs classified only as Bacteria made up around 6% of the detectable bacterial community in A. salmiana and A. tequilana (Fig. 2). Distribution of detected OTUs and their relative abundance according to the community type revealed that the bacterial communities in the soil, which included soil and root-zone soil samples, were most similar among agaves than the plant-associated communities (Fig. 2). Especially, the root and leaf endospheres displayed the greater variation among agaves and among each other. Clearly, members of the γ-Proteobacteria increased their relative abundance in the episphere, which includes the rhizosphere and phyllosphere, compared to the soil. Members of the α-Proteobacteria were more abundant in the endosphere relative to the episphere, especially in the root endosphere of the wild A. salmiana (Fig. 2).

Taxonomic distribution of bacterial groups associated with Agave species based on DGGE profiles. The first row of stacked bar shows the overall structure of detected bacterial taxa in each Agave species. The following bars show the structure by community group immediately followed by the two community types integrating each group. Soil integrated by s – soil and rzs – root-zone soil; Episphere integrated by p – phyllosphere and rz – rhizosphere; and Endosphere integrated by le – leaf endosphere and re – root endosphere.
Although we are aware that DGGE had a low resolution compared to NGS technologies, we used DGGE profiles for estimating diversity of the bacterial communities to establish a comparative baseline and guide further work. For this, we tested if the number of OTUs, as well as the Shannon and Berger–Parker indexes were significantly influenced by the plant species and/or the community type (Table 2). These analyses showed that on average A. salmiana had a higher number of OTUs associated with its communities than A. tequilana and that the number of OTUs was influenced by the community group (anova, F2,6 = 23.46, P≤ 0.001). Moreover, the lower Berger–Parker values associated with A. salmiana indicated that its communities were dominated by fewer bacterial species than the cultivated A. tequilana (anova, F1,6 = 23.67, P≤ 0.01), which was congruent with the observed composition shown in Fig. 2. The observable differences in the values of the Shannon index for each community type and species were not significant. Interestingly, diversity values calculated for both agaves were similar between the rhizosphere and the phyllosphere; while in the endosphere, a clear distinction between the root and leaf endosphere was evident in the cultivated Agave (Table 2).
Estimated diversity based on DGGE profiles for the six different community types in both Agave species
Community group | Community type | A. salmiana | A. tequilana | ||||||||||||||||||||||||||||||||
Number of OTUs | Shannon index (H′) | Berger–Parker index | Number of OTUs | Shannon index (H′) | Berger–Parker index | ||||||||||||||||||||||||||||||
All | Overall | 62 | 4.127 | 1.751 | 54 | 3.989 | 2.309 | ||||||||||||||||||||||||||||
Soil | Soil | 34 | 3.555 | 1.213 | 34 | 3.526 | 1.811 | ||||||||||||||||||||||||||||
Root-zone-soil | 41 | 3.714 | 1.489 | 30 | 3.401 | 1.465 | |||||||||||||||||||||||||||||
Episphere | Rhizosphere | 23 | 3.135 | 0.937 | 28 | 3.332 | 1.700 | ||||||||||||||||||||||||||||
Phyllosphere | 27 | 3.296 | 1.159 | 27 | 3.296 | 1.771 | |||||||||||||||||||||||||||||
Endosphere | Root endosphere | 35 | 3.555 | 1.537 | 31 | 3.434 | 2.483 | ||||||||||||||||||||||||||||
Leaf endosphere | 34 | 3.526 | 1.571 | 16 | 2.773 | 2.089 |
Community group | Community type | A. salmiana | A. tequilana | ||||||||||||||||||||||||||||||||
Number of OTUs | Shannon index (H′) | Berger–Parker index | Number of OTUs | Shannon index (H′) | Berger–Parker index | ||||||||||||||||||||||||||||||
All | Overall | 62 | 4.127 | 1.751 | 54 | 3.989 | 2.309 | ||||||||||||||||||||||||||||
Soil | Soil | 34 | 3.555 | 1.213 | 34 | 3.526 | 1.811 | ||||||||||||||||||||||||||||
Root-zone-soil | 41 | 3.714 | 1.489 | 30 | 3.401 | 1.465 | |||||||||||||||||||||||||||||
Episphere | Rhizosphere | 23 | 3.135 | 0.937 | 28 | 3.332 | 1.700 | ||||||||||||||||||||||||||||
Phyllosphere | 27 | 3.296 | 1.159 | 27 | 3.296 | 1.771 | |||||||||||||||||||||||||||||
Endosphere | Root endosphere | 35 | 3.555 | 1.537 | 31 | 3.434 | 2.483 | ||||||||||||||||||||||||||||
Leaf endosphere | 34 | 3.526 | 1.571 | 16 | 2.773 | 2.089 |
Estimated diversity based on DGGE profiles for the six different community types in both Agave species
Community group | Community type | A. salmiana | A. tequilana | ||||||||||||||||||||||||||||||||
Number of OTUs | Shannon index (H′) | Berger–Parker index | Number of OTUs | Shannon index (H′) | Berger–Parker index | ||||||||||||||||||||||||||||||
All | Overall | 62 | 4.127 | 1.751 | 54 | 3.989 | 2.309 | ||||||||||||||||||||||||||||
Soil | Soil | 34 | 3.555 | 1.213 | 34 | 3.526 | 1.811 | ||||||||||||||||||||||||||||
Root-zone-soil | 41 | 3.714 | 1.489 | 30 | 3.401 | 1.465 | |||||||||||||||||||||||||||||
Episphere | Rhizosphere | 23 | 3.135 | 0.937 | 28 | 3.332 | 1.700 | ||||||||||||||||||||||||||||
Phyllosphere | 27 | 3.296 | 1.159 | 27 | 3.296 | 1.771 | |||||||||||||||||||||||||||||
Endosphere | Root endosphere | 35 | 3.555 | 1.537 | 31 | 3.434 | 2.483 | ||||||||||||||||||||||||||||
Leaf endosphere | 34 | 3.526 | 1.571 | 16 | 2.773 | 2.089 |
Community group | Community type | A. salmiana | A. tequilana | ||||||||||||||||||||||||||||||||
Number of OTUs | Shannon index (H′) | Berger–Parker index | Number of OTUs | Shannon index (H′) | Berger–Parker index | ||||||||||||||||||||||||||||||
All | Overall | 62 | 4.127 | 1.751 | 54 | 3.989 | 2.309 | ||||||||||||||||||||||||||||
Soil | Soil | 34 | 3.555 | 1.213 | 34 | 3.526 | 1.811 | ||||||||||||||||||||||||||||
Root-zone-soil | 41 | 3.714 | 1.489 | 30 | 3.401 | 1.465 | |||||||||||||||||||||||||||||
Episphere | Rhizosphere | 23 | 3.135 | 0.937 | 28 | 3.332 | 1.700 | ||||||||||||||||||||||||||||
Phyllosphere | 27 | 3.296 | 1.159 | 27 | 3.296 | 1.771 | |||||||||||||||||||||||||||||
Endosphere | Root endosphere | 35 | 3.555 | 1.537 | 31 | 3.434 | 2.483 | ||||||||||||||||||||||||||||
Leaf endosphere | 34 | 3.526 | 1.571 | 16 | 2.773 | 2.089 |
Factors affecting the bacterial communities associated with agaves
permanova analyses revealed that the detected bacterial composition was mostly influenced by the community type, that is, soil, root-zone-soil, rhizosphere, phyllosphere, root, and leaf endosphere (F5,120 = 16.94, R2 = 0.29, P≤ 0.001), followed by the Agave species (F1,120 = 12.05, R2 = 0.04, P≤ 0.001) and the interaction between community type and Agave species. Together they explained 42% of the observed variation. In general, the factor season only explained 1.9% of the total variance, but the interaction community type and season were the second most relevant, after community type, when permanova was performed on separate datasets from each Agave species. In addition, nmds graphics showed that for A. salmiana, clustering pattern relied on the community group indistinctively of sampling site and season (Fig. 3), while in A. tequilana, a clearer clustering was observed only if samples from each site (Am and Pe) were plotted independently (Fig. S2). Nonetheless, the nmds plots generated revealed that in both Agave species three major community groups could be observed as depicted in Fig. 3 and Fig. S2: (1) the soil integrated by samples from soil and root-zone-soil (s-rzs), (2) the episphere which included the rhizosphere and phyllosphere (rz-p), and (3) the endosphere composed by samples from the interior of leaves and roots (le-re). These results and the similar patterns observed in the structure of the bacterial communities among samples within each group (Fig. 2, Figs S1 and S2), prompted us to separately analyze the influence of all evaluated factors on the prokaryotic assemblages.
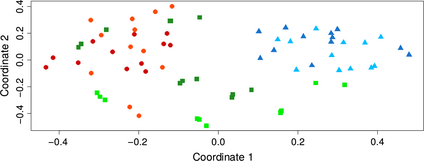
nmds clustering of the six bacterial communities associated with Agave salmiana (S= 0.27). Leaf endosphere (light green squares), root endosphere (dark green squares), phyllosphere (light blue triangles), rhizosphere (dark blue triangles), root-zone soil (orange circles), and soil (red circles).
Bacterial communities associated with the soil (s-rzs) were mainly affected by the season (F1,40 = 11.86, R2 = 0.15, P≤ 0.001) and the interaction between season and species (F1,40 = 6.63, R2 = 0.08, P≤ 0.001). These two factors explained 23% of the total variance in this subset. Acidobacteria was more abundant in soils associated with A. salmiana, while Firmicutes were more abundant in the soils associated with A. tequilana. The phylum Nitrospirae was not detected in the soil samples of the cultivated Agave, but major differences among soils of cultivated and wild agaves were not revealed in our study.
Communities from the episphere (rz-p) were affected by the plant species (F1,40 = 35.01, R2 = 0.31, P≤ 0.001; Fig. 4), the season (F1,40 = 12.85, R2 = 0.11, P≤ 0.001) and their interaction (F1,40 = 15.58, R2= 0.14, P≤ 0.001). Together they explained 56% of the total variance in this group. The dominant bacterial phylum in the episphere was the γ-Proteobacteria, being the OTU40, an Enterobacter, the dominant taxon in A. salmiana, while in A. tequilana the most abundant taxon was Stenotrophomonas (OTU38).
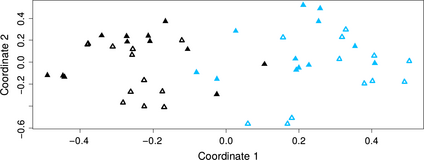
nmds clustering of bacteria associated with the episphere (phyllosphere and rhizosphere) of both Agave species (S= 0.24). Agave salmiana: phyllosphere (empty black triangles) and rhizosphere (full black triangles); Agave tequilana: phyllosphere (empty light blue triangles) and rhizosphere (full light blue triangles).
Finally, bacterial communities associated with the endosphere (re-le) were affected by the season (F1,40 = 11.24, R2 = 0.16, P≤ 0.001), the community type (F1,40 = 4.8, R2 = 0.07, P≤ 0.001) and the host species (F1,40 = 4.49, R2 = 0.06, P≤ 0.001). Together, these factors explained 29% of the total variance in the subgroup. Only in this group, significant differences among the two community types, that is, root and leaf endosphere, were found. Interestingly, root and leaf endosphere samples of A. salmiana and A. tequilana from Penjamo collected during the dry season were similar between them and formed a unique cluster, leaving samples from A. tequilana from Amatitan out of it (Fig. 5). Thus, we investigated whether such differences could be explained by different rainfall patterns among sampling sites (Table 1). The Kruskal–Wallis test supported that accumulated precipitation during the 8 months of the dry season was different between the sites in Guanajuato (Pe, Ma, and SF) and Amatitan (Am) (H3,32 = 10.445, P= 0.0151, Table 1). This suggests that different levels of rainfall could promote changes in the endophytic bacterial communities in these plants. In the endosphere of A. salmiana, the dominant taxon was the α-Proteobacteria: Reyranella (OTU68), while in A. tequilana, there was not a dominant taxon, but several OTUs belonging to the γ-Proteobacteria accounted for the majority of its endophytic communities. We also found that, compared with the episphere group, some bacterial taxa in the endosphere such as the Acidobacteria Gp4, Corynebacteriales, Mycobacterium, Streptomyces, Bacillaceae, Clostridium, Devosia, Xanthobacteraceae, Reyranella, δ-Proteobacteria, and some members from Enterobacteriaceae were enriched (Fig. 2).
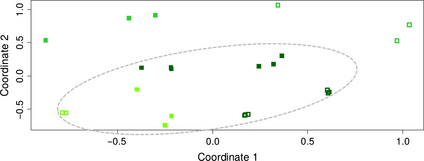
nmds clustering of bacteria associated with the endosphere of Agave salmiana (leaf: empty dark green squares, root: full dark green squares) and Agave tequilana (Amatitan: leaf: empty green squares, root: full green squares; Penjamo: leaf: empty light green squares, root: full light green squares), during the dry season (S= 0.19). The gray ellipse comprises the cluster formed by samples from Guanajuato.
The endophytic dry core of agaves
Despite differences in cultivation status and rainfall patterns, we identified a group of fifteen OTUs shared between the root and leaf endosphere of A. salmiana and A. tequilana in all sites during the dry season (Table 3). From this finding, we were interested in using the definition of ‘core microbiome’ for further exploring functional hypotheses. A core microbiome is defined as members of the microbial community that are found in all the assemblages associated with a habitat (Shade & Handelsman, 2012). Thus, we denominated this group of fifteen OTUs as the endophytic dry core of agaves, and it is composed by members of the γ-Proteobacteria (6/15), α-Proteobacteria (2/15), Actinobacteria (2/15), Acidobacteria (2/15), Firmicutes (1/15), δ-Proteobacteria (1/15), and an unclassified bacterium (1/15) (Table 3). In both Agave species, endophytic dry core OTUs represented the major part of their community during the dry season (> 65%), compared with the rainy season (< 33%) (Fig. S3).
OTUs from the endosphere of Agave salmiana and Agave tequilana postulated as the endophytic dry core of agaves
OTU ID | Lineage | A. salmiana | A. tequilana | |||||||||||||||||
Leaf endophyte | Root endophyte | Leaf endophyte | Root endophyte | |||||||||||||||||
41 | Bacteria | x | x | x | x | |||||||||||||||
28 | Acidobacteria Gp4 | x | x | x | ||||||||||||||||
65 | Acidobacteria Gp6 | x | x | x | x | |||||||||||||||
47 | Streptomyces | x | x | x | x | |||||||||||||||
35 | Solirubrobacterales | x | x | x | ||||||||||||||||
56 | Clostridium | x | x | x | ||||||||||||||||
54 | Xanthobacteraceae | x | x | x | ||||||||||||||||
68 | Reyranella | x | x | x | x | |||||||||||||||
71 | Deltaproteobacteria | x | x | x | ||||||||||||||||
48 | Enterobacteriaceae | x | x | x | x | |||||||||||||||
59 | Enterobacteriaceae | x | x | x | ||||||||||||||||
40 | Enterobacter | x | x | |||||||||||||||||
61 | Enterobacter | x | x | x | x | |||||||||||||||
57 | Xanthomonadales | x | x | x | x | |||||||||||||||
38 | Stenotrophomonas | x | x | x |
OTU ID | Lineage | A. salmiana | A. tequilana | |||||||||||||||||
Leaf endophyte | Root endophyte | Leaf endophyte | Root endophyte | |||||||||||||||||
41 | Bacteria | x | x | x | x | |||||||||||||||
28 | Acidobacteria Gp4 | x | x | x | ||||||||||||||||
65 | Acidobacteria Gp6 | x | x | x | x | |||||||||||||||
47 | Streptomyces | x | x | x | x | |||||||||||||||
35 | Solirubrobacterales | x | x | x | ||||||||||||||||
56 | Clostridium | x | x | x | ||||||||||||||||
54 | Xanthobacteraceae | x | x | x | ||||||||||||||||
68 | Reyranella | x | x | x | x | |||||||||||||||
71 | Deltaproteobacteria | x | x | x | ||||||||||||||||
48 | Enterobacteriaceae | x | x | x | x | |||||||||||||||
59 | Enterobacteriaceae | x | x | x | ||||||||||||||||
40 | Enterobacter | x | x | |||||||||||||||||
61 | Enterobacter | x | x | x | x | |||||||||||||||
57 | Xanthomonadales | x | x | x | x | |||||||||||||||
38 | Stenotrophomonas | x | x | x |
OTUs from the endosphere of Agave salmiana and Agave tequilana postulated as the endophytic dry core of agaves
OTU ID | Lineage | A. salmiana | A. tequilana | |||||||||||||||||
Leaf endophyte | Root endophyte | Leaf endophyte | Root endophyte | |||||||||||||||||
41 | Bacteria | x | x | x | x | |||||||||||||||
28 | Acidobacteria Gp4 | x | x | x | ||||||||||||||||
65 | Acidobacteria Gp6 | x | x | x | x | |||||||||||||||
47 | Streptomyces | x | x | x | x | |||||||||||||||
35 | Solirubrobacterales | x | x | x | ||||||||||||||||
56 | Clostridium | x | x | x | ||||||||||||||||
54 | Xanthobacteraceae | x | x | x | ||||||||||||||||
68 | Reyranella | x | x | x | x | |||||||||||||||
71 | Deltaproteobacteria | x | x | x | ||||||||||||||||
48 | Enterobacteriaceae | x | x | x | x | |||||||||||||||
59 | Enterobacteriaceae | x | x | x | ||||||||||||||||
40 | Enterobacter | x | x | |||||||||||||||||
61 | Enterobacter | x | x | x | x | |||||||||||||||
57 | Xanthomonadales | x | x | x | x | |||||||||||||||
38 | Stenotrophomonas | x | x | x |
OTU ID | Lineage | A. salmiana | A. tequilana | |||||||||||||||||
Leaf endophyte | Root endophyte | Leaf endophyte | Root endophyte | |||||||||||||||||
41 | Bacteria | x | x | x | x | |||||||||||||||
28 | Acidobacteria Gp4 | x | x | x | ||||||||||||||||
65 | Acidobacteria Gp6 | x | x | x | x | |||||||||||||||
47 | Streptomyces | x | x | x | x | |||||||||||||||
35 | Solirubrobacterales | x | x | x | ||||||||||||||||
56 | Clostridium | x | x | x | ||||||||||||||||
54 | Xanthobacteraceae | x | x | x | ||||||||||||||||
68 | Reyranella | x | x | x | x | |||||||||||||||
71 | Deltaproteobacteria | x | x | x | ||||||||||||||||
48 | Enterobacteriaceae | x | x | x | x | |||||||||||||||
59 | Enterobacteriaceae | x | x | x | ||||||||||||||||
40 | Enterobacter | x | x | |||||||||||||||||
61 | Enterobacter | x | x | x | x | |||||||||||||||
57 | Xanthomonadales | x | x | x | x | |||||||||||||||
38 | Stenotrophomonas | x | x | x |
Diazotrophic potential among bacterial communities
We isolated 18 bacterial strains in N-free culture media. From these, eight strains were recovered from the root endosphere and rhizosphere of A. tequilana, and ten were recovered from the root endosphere, rhizosphere, and phyllosphere of A. salmiana (Table 4). Molecular characterization of the isolated strains revealed that bacteria associated with cultivated A. tequilana were all members of the γ-Proteobacteria, mainly Enterobacteriaceae (7 out of 8, 87.5%) and one characterized as Stenotrophomonas. Isolates from A. salmiana included not only members of the γ-Proteobacteria (60%), but also members of the α-Proteobacteria, Firmicutes, and Actinobacteria (Table 4). Remarkably, ten of these bacterial strains were grouped at ≥ 98% similarity with five distinct DGGE OTUs (Table 4, Table S2), being eight of them similar to OTU 61 and OTU 38, which belonged to the endophytic dry core of agaves (Table 4, Table S2). Finally, we found that the acetylene reduction assay was positive for eleven strains, confirming their diazotrophic activity (Table 4).
Strain ID | Agave species | Community type | Confirmed ARA activity | SILVA Taxonomic lineage (NCBI best hit accession number) | DGGE cluster | |||||||||||||||
MJ02 | A. tequilana | Root endosphere (re) | No | Bacteria; Proteobacteria; Gammaproteobacteria; Enterobacteriales; Enterobacteriaceae. (NR_117704) | – | |||||||||||||||
MJ03 | A. tequilana | Root endosphere (re) | No | Bacteria; Proteobacteria; Gammaproteobacteria; Enterobacteriales; Enterobacteriaceae. (NR_117704) | – | |||||||||||||||
MJ04 | A. tequilana | Root endosphere (re) | Yes | Bacteria; Proteobacteria; Gammaproteobacteria; Enterobacteriales; Enterobacteriaceae. (NR_104933) | 1 | |||||||||||||||
MJ05 | A. tequilana | Root endosphere (re) | Yes | Bacteria; Proteobacteria; Gammaproteobacteria; Enterobacteriales;Enterobacteriaceae. (NR_104933) | 1 | |||||||||||||||
MJ06 | A. tequilana | Root endosphere (re) | Yes | Bacteria; Proteobacteria; Gammaproteobacteria; Enterobacteriales;Enterobacteriaceae. (NR_104933) | 1 | |||||||||||||||
MJ07 | A. tequilana | Root endosphere (re) | No | Bacteria; Proteobacteria; Gammaproteobacteria; Enterobacteriales;Enterobacteriaceae. (NR_104934) | 1 | |||||||||||||||
MJ08 | A. tequilana | Rhizosphere (rz) | Yes | Bacteria; Proteobacteria; Gammaproteobacteria; Xanthomonadales; Xanthomonadaceae; Stenotrophomonas. (NR_041577) | 3 | |||||||||||||||
MJ09 | A. tequilana | Rhizosphere (rz) | Yes | Bacteria; Proteobacteria; Gammaproteobacteria; Enterobacteriales;Enterobacteriaceae. (NR_044977) | 1 | |||||||||||||||
MJ10 | A. salmiana | Rhizosphere (rz) | No | Bacteria. (NR_116033) (partial sequence) | – | |||||||||||||||
MJ11 | A. salmiana | Rhizosphere (rz) | Yes | Bacteria; Firmicutes; Bacilli; Bacillales; Bacillaceae; Bacillus (NR_115953) | 2 | |||||||||||||||
MJ13 | A. salmiana | Rhizosphere (rz) | No | Bacteria. (NR_117704) | – | |||||||||||||||
MJ14 | A. salmiana | Rhizosphere (rz) | Yes | Bacteria; Actinobacteria; Actinobacteria; Micrococcales; Microbacteriaceae; Microbacterium. (NR_044937) | – | |||||||||||||||
MJ15 | A. salmiana | Rhizosphere (rz) | No | Bacteria; Proteobacteria; Gammaproteobacteria; Enterobacteriales;Enterobacteriaceae. (NR_117704) | – | |||||||||||||||
MJ17 | A. salmiana | Root endosphere (re) | Yes | Bacteria; Proteobacteria; Gammaproteobacteria; Xanthomonadales; Xanthomonadaceae; Stenotrophomonas. (NR_041577) | 3 | |||||||||||||||
MJ18 | A. salmiana | Root endosphere (re) | Yes | Bacteria; Proteobacteria; Gammaproteobacteria; Enterobacteriales;Enterobacteriaceae. (NR_117704) (partial sequence) | – | |||||||||||||||
MJ19 | A. salmiana | Root endosphere (re) | Yes | Bacteria; Proteobacteria; Gammaproteobacteria; Enterobacteriales;Enterobacteriaceae. (NR_104933) | 1 | |||||||||||||||
MJ20 | A. salmiana | Rhizosphere (rz) | No | Bacteria; Proteobacteria; Gammaproteobacteria; Enterobacteriales;Enterobacteriaceae. (NR_044977) | 1 | |||||||||||||||
MJ22 | A. salmiana | Phyllosphere (p) | Yes | Bacteria; Proteobacteria; Alphaproteobacteria; Rhodospirillales; Acetobacteraceae. (NR_109297) | – |
Strain ID | Agave species | Community type | Confirmed ARA activity | SILVA Taxonomic lineage (NCBI best hit accession number) | DGGE cluster | |||||||||||||||
MJ02 | A. tequilana | Root endosphere (re) | No | Bacteria; Proteobacteria; Gammaproteobacteria; Enterobacteriales; Enterobacteriaceae. (NR_117704) | – | |||||||||||||||
MJ03 | A. tequilana | Root endosphere (re) | No | Bacteria; Proteobacteria; Gammaproteobacteria; Enterobacteriales; Enterobacteriaceae. (NR_117704) | – | |||||||||||||||
MJ04 | A. tequilana | Root endosphere (re) | Yes | Bacteria; Proteobacteria; Gammaproteobacteria; Enterobacteriales; Enterobacteriaceae. (NR_104933) | 1 | |||||||||||||||
MJ05 | A. tequilana | Root endosphere (re) | Yes | Bacteria; Proteobacteria; Gammaproteobacteria; Enterobacteriales;Enterobacteriaceae. (NR_104933) | 1 | |||||||||||||||
MJ06 | A. tequilana | Root endosphere (re) | Yes | Bacteria; Proteobacteria; Gammaproteobacteria; Enterobacteriales;Enterobacteriaceae. (NR_104933) | 1 | |||||||||||||||
MJ07 | A. tequilana | Root endosphere (re) | No | Bacteria; Proteobacteria; Gammaproteobacteria; Enterobacteriales;Enterobacteriaceae. (NR_104934) | 1 | |||||||||||||||
MJ08 | A. tequilana | Rhizosphere (rz) | Yes | Bacteria; Proteobacteria; Gammaproteobacteria; Xanthomonadales; Xanthomonadaceae; Stenotrophomonas. (NR_041577) | 3 | |||||||||||||||
MJ09 | A. tequilana | Rhizosphere (rz) | Yes | Bacteria; Proteobacteria; Gammaproteobacteria; Enterobacteriales;Enterobacteriaceae. (NR_044977) | 1 | |||||||||||||||
MJ10 | A. salmiana | Rhizosphere (rz) | No | Bacteria. (NR_116033) (partial sequence) | – | |||||||||||||||
MJ11 | A. salmiana | Rhizosphere (rz) | Yes | Bacteria; Firmicutes; Bacilli; Bacillales; Bacillaceae; Bacillus (NR_115953) | 2 | |||||||||||||||
MJ13 | A. salmiana | Rhizosphere (rz) | No | Bacteria. (NR_117704) | – | |||||||||||||||
MJ14 | A. salmiana | Rhizosphere (rz) | Yes | Bacteria; Actinobacteria; Actinobacteria; Micrococcales; Microbacteriaceae; Microbacterium. (NR_044937) | – | |||||||||||||||
MJ15 | A. salmiana | Rhizosphere (rz) | No | Bacteria; Proteobacteria; Gammaproteobacteria; Enterobacteriales;Enterobacteriaceae. (NR_117704) | – | |||||||||||||||
MJ17 | A. salmiana | Root endosphere (re) | Yes | Bacteria; Proteobacteria; Gammaproteobacteria; Xanthomonadales; Xanthomonadaceae; Stenotrophomonas. (NR_041577) | 3 | |||||||||||||||
MJ18 | A. salmiana | Root endosphere (re) | Yes | Bacteria; Proteobacteria; Gammaproteobacteria; Enterobacteriales;Enterobacteriaceae. (NR_117704) (partial sequence) | – | |||||||||||||||
MJ19 | A. salmiana | Root endosphere (re) | Yes | Bacteria; Proteobacteria; Gammaproteobacteria; Enterobacteriales;Enterobacteriaceae. (NR_104933) | 1 | |||||||||||||||
MJ20 | A. salmiana | Rhizosphere (rz) | No | Bacteria; Proteobacteria; Gammaproteobacteria; Enterobacteriales;Enterobacteriaceae. (NR_044977) | 1 | |||||||||||||||
MJ22 | A. salmiana | Phyllosphere (p) | Yes | Bacteria; Proteobacteria; Alphaproteobacteria; Rhodospirillales; Acetobacteraceae. (NR_109297) | – |
ARA, Acetylene reduction assay as described in Hardy et al. (1968).
Cluster 1: strains grouped with OTUs 60, 61, and 62; Cluster 2: strains grouped with OTU 31; Cluster 3: strains grouped with OTU 38.
Strain ID | Agave species | Community type | Confirmed ARA activity | SILVA Taxonomic lineage (NCBI best hit accession number) | DGGE cluster | |||||||||||||||
MJ02 | A. tequilana | Root endosphere (re) | No | Bacteria; Proteobacteria; Gammaproteobacteria; Enterobacteriales; Enterobacteriaceae. (NR_117704) | – | |||||||||||||||
MJ03 | A. tequilana | Root endosphere (re) | No | Bacteria; Proteobacteria; Gammaproteobacteria; Enterobacteriales; Enterobacteriaceae. (NR_117704) | – | |||||||||||||||
MJ04 | A. tequilana | Root endosphere (re) | Yes | Bacteria; Proteobacteria; Gammaproteobacteria; Enterobacteriales; Enterobacteriaceae. (NR_104933) | 1 | |||||||||||||||
MJ05 | A. tequilana | Root endosphere (re) | Yes | Bacteria; Proteobacteria; Gammaproteobacteria; Enterobacteriales;Enterobacteriaceae. (NR_104933) | 1 | |||||||||||||||
MJ06 | A. tequilana | Root endosphere (re) | Yes | Bacteria; Proteobacteria; Gammaproteobacteria; Enterobacteriales;Enterobacteriaceae. (NR_104933) | 1 | |||||||||||||||
MJ07 | A. tequilana | Root endosphere (re) | No | Bacteria; Proteobacteria; Gammaproteobacteria; Enterobacteriales;Enterobacteriaceae. (NR_104934) | 1 | |||||||||||||||
MJ08 | A. tequilana | Rhizosphere (rz) | Yes | Bacteria; Proteobacteria; Gammaproteobacteria; Xanthomonadales; Xanthomonadaceae; Stenotrophomonas. (NR_041577) | 3 | |||||||||||||||
MJ09 | A. tequilana | Rhizosphere (rz) | Yes | Bacteria; Proteobacteria; Gammaproteobacteria; Enterobacteriales;Enterobacteriaceae. (NR_044977) | 1 | |||||||||||||||
MJ10 | A. salmiana | Rhizosphere (rz) | No | Bacteria. (NR_116033) (partial sequence) | – | |||||||||||||||
MJ11 | A. salmiana | Rhizosphere (rz) | Yes | Bacteria; Firmicutes; Bacilli; Bacillales; Bacillaceae; Bacillus (NR_115953) | 2 | |||||||||||||||
MJ13 | A. salmiana | Rhizosphere (rz) | No | Bacteria. (NR_117704) | – | |||||||||||||||
MJ14 | A. salmiana | Rhizosphere (rz) | Yes | Bacteria; Actinobacteria; Actinobacteria; Micrococcales; Microbacteriaceae; Microbacterium. (NR_044937) | – | |||||||||||||||
MJ15 | A. salmiana | Rhizosphere (rz) | No | Bacteria; Proteobacteria; Gammaproteobacteria; Enterobacteriales;Enterobacteriaceae. (NR_117704) | – | |||||||||||||||
MJ17 | A. salmiana | Root endosphere (re) | Yes | Bacteria; Proteobacteria; Gammaproteobacteria; Xanthomonadales; Xanthomonadaceae; Stenotrophomonas. (NR_041577) | 3 | |||||||||||||||
MJ18 | A. salmiana | Root endosphere (re) | Yes | Bacteria; Proteobacteria; Gammaproteobacteria; Enterobacteriales;Enterobacteriaceae. (NR_117704) (partial sequence) | – | |||||||||||||||
MJ19 | A. salmiana | Root endosphere (re) | Yes | Bacteria; Proteobacteria; Gammaproteobacteria; Enterobacteriales;Enterobacteriaceae. (NR_104933) | 1 | |||||||||||||||
MJ20 | A. salmiana | Rhizosphere (rz) | No | Bacteria; Proteobacteria; Gammaproteobacteria; Enterobacteriales;Enterobacteriaceae. (NR_044977) | 1 | |||||||||||||||
MJ22 | A. salmiana | Phyllosphere (p) | Yes | Bacteria; Proteobacteria; Alphaproteobacteria; Rhodospirillales; Acetobacteraceae. (NR_109297) | – |
Strain ID | Agave species | Community type | Confirmed ARA activity | SILVA Taxonomic lineage (NCBI best hit accession number) | DGGE cluster | |||||||||||||||
MJ02 | A. tequilana | Root endosphere (re) | No | Bacteria; Proteobacteria; Gammaproteobacteria; Enterobacteriales; Enterobacteriaceae. (NR_117704) | – | |||||||||||||||
MJ03 | A. tequilana | Root endosphere (re) | No | Bacteria; Proteobacteria; Gammaproteobacteria; Enterobacteriales; Enterobacteriaceae. (NR_117704) | – | |||||||||||||||
MJ04 | A. tequilana | Root endosphere (re) | Yes | Bacteria; Proteobacteria; Gammaproteobacteria; Enterobacteriales; Enterobacteriaceae. (NR_104933) | 1 | |||||||||||||||
MJ05 | A. tequilana | Root endosphere (re) | Yes | Bacteria; Proteobacteria; Gammaproteobacteria; Enterobacteriales;Enterobacteriaceae. (NR_104933) | 1 | |||||||||||||||
MJ06 | A. tequilana | Root endosphere (re) | Yes | Bacteria; Proteobacteria; Gammaproteobacteria; Enterobacteriales;Enterobacteriaceae. (NR_104933) | 1 | |||||||||||||||
MJ07 | A. tequilana | Root endosphere (re) | No | Bacteria; Proteobacteria; Gammaproteobacteria; Enterobacteriales;Enterobacteriaceae. (NR_104934) | 1 | |||||||||||||||
MJ08 | A. tequilana | Rhizosphere (rz) | Yes | Bacteria; Proteobacteria; Gammaproteobacteria; Xanthomonadales; Xanthomonadaceae; Stenotrophomonas. (NR_041577) | 3 | |||||||||||||||
MJ09 | A. tequilana | Rhizosphere (rz) | Yes | Bacteria; Proteobacteria; Gammaproteobacteria; Enterobacteriales;Enterobacteriaceae. (NR_044977) | 1 | |||||||||||||||
MJ10 | A. salmiana | Rhizosphere (rz) | No | Bacteria. (NR_116033) (partial sequence) | – | |||||||||||||||
MJ11 | A. salmiana | Rhizosphere (rz) | Yes | Bacteria; Firmicutes; Bacilli; Bacillales; Bacillaceae; Bacillus (NR_115953) | 2 | |||||||||||||||
MJ13 | A. salmiana | Rhizosphere (rz) | No | Bacteria. (NR_117704) | – | |||||||||||||||
MJ14 | A. salmiana | Rhizosphere (rz) | Yes | Bacteria; Actinobacteria; Actinobacteria; Micrococcales; Microbacteriaceae; Microbacterium. (NR_044937) | – | |||||||||||||||
MJ15 | A. salmiana | Rhizosphere (rz) | No | Bacteria; Proteobacteria; Gammaproteobacteria; Enterobacteriales;Enterobacteriaceae. (NR_117704) | – | |||||||||||||||
MJ17 | A. salmiana | Root endosphere (re) | Yes | Bacteria; Proteobacteria; Gammaproteobacteria; Xanthomonadales; Xanthomonadaceae; Stenotrophomonas. (NR_041577) | 3 | |||||||||||||||
MJ18 | A. salmiana | Root endosphere (re) | Yes | Bacteria; Proteobacteria; Gammaproteobacteria; Enterobacteriales;Enterobacteriaceae. (NR_117704) (partial sequence) | – | |||||||||||||||
MJ19 | A. salmiana | Root endosphere (re) | Yes | Bacteria; Proteobacteria; Gammaproteobacteria; Enterobacteriales;Enterobacteriaceae. (NR_104933) | 1 | |||||||||||||||
MJ20 | A. salmiana | Rhizosphere (rz) | No | Bacteria; Proteobacteria; Gammaproteobacteria; Enterobacteriales;Enterobacteriaceae. (NR_044977) | 1 | |||||||||||||||
MJ22 | A. salmiana | Phyllosphere (p) | Yes | Bacteria; Proteobacteria; Alphaproteobacteria; Rhodospirillales; Acetobacteraceae. (NR_109297) | – |
ARA, Acetylene reduction assay as described in Hardy et al. (1968).
Cluster 1: strains grouped with OTUs 60, 61, and 62; Cluster 2: strains grouped with OTU 31; Cluster 3: strains grouped with OTU 38.
Discussion
In this study, we used 16S rRNA-DGGE fingerprinting to investigate for the first time the external and internal bacterial communities associated with two CAM plant species: wild A. salmiana and cultivated A. tequilana. We also evaluated the diazotrophic potential of their native bacteria as Agave soils are characterized by their low nitrogen content. Despite the low resolution and the intrinsic limitations of DGGE (Nocker et al., 2007) in comparison to next-generation sequencing technologies, DGGE fingerprinting proved useful for identifying major bacterial players in both external and internal plant-associated communities and the factors that affect their assemblage in Agave species. Moreover, DGGE profiles, as also used in other studies (Bondoso et al., 2014), suggested that bacterial diversity may be higher in the wild A. salmiana than in the cultivated Agave. Additionally, comparable Shannon diversity values among the phyllosphere and rhizosphere of both agaves support the idea that bacterial diversity in the phyllosphere and rhizosphere could be similar, although significant differences in the dominance of such communities were found between species. It would be ideal to test whether these patterns hold truth by employing metagenomics or other high-throughput sequencing technologies.
Agave salmiana and A. tequilana shared 78.5% of the bacterial OTUs detected by DGGE. This result suggests possible common mechanisms of recruitment and selection of their bacterial communities. In general, bacterial communities associated with both Agave species were dominated by γ-Proteobacteria (mainly Enterobacteraceae and Xanthomonadaceae) and α-Proteobacteria, followed by Actinobacteria and Acidobacteria. In studies with Arabidopsis thaliana, a C3 metabolism plant (Lundberg et al., 2012; Bodenhausen et al., 2013), and maize, a C4 metabolism plant (Peiffer et al., 2013), Proteobacteria was reported as a dominant phylum too. However, distinct proteobacterial taxa were associated with each plant species (Lundberg et al., 2012; Bodenhausen et al., 2013; Peiffer et al., 2013). This selection for different taxa could be promoted by the host genotype, the environmental pool of microorganisms, and particularly by specific plant–microorganism interactions. Additionally, the presence of 6% unclassified bacterial taxa in Agave suggests the existence of yet uncharacterized bacteria, which may play a role in the Agave ecosystem. This idea is supported by the fact that some diazotrophic bacteria from A. salmiana were identified only at the bacterial level using the silva database (strains MJ10 and MJ13, Table 4).
Our results showed that despite their cultivation status and taxonomical position, the main factor affecting bacterial community structure in agaves was the community type, where three major groups were formed: soil (s-rzs), episphere (rz-p), and endosphere (le-re). This finding is in agreement with other studies like those made in arid regions with Capsicum annunm L. (Marasco et al., 2012), and with medicinal plants (Köberl et al., 2011) as well as with those made with wild populations of Populus deltoides (Gottel et al., 2011), where distinct bacterial communities in the soil, rhizosphere, and root endosphere were clearly identified. Moreover, as in most of the previous studies, a significant effect of the host species was also revealed in Agave, especially at the epiphytic level.
Bacterial communities from the soil (s-rzs) were dominated by γ- and α-Proteobacteria, Acidobacteria and Actinobacteria in both Agave species. This finding is consistent with the bacterial composition reported in soils from the Sonoran desert, where the same taxa were the major bacterial groups too (Andrew et al., 2012). In the episphere group, bacterial communities were influenced by the Agave species. This result suggests a possible plant selection effect on these communities. In a recent study in soybean crop, Mendes et al. (2014) found that the rhizosphere is assembled following the niche-based theory, pointing out that selection plant effect and some environmental variables are the main drivers in this assemblage. The plant selection effect on bacterial communities may be related to specific plant exudates that can vary among different species or cultivars (Costa et al., 2006). In this way, plants provide diverse nutrient sources, influencing composition and diversity of bacterial communities in the episphere (Bever et al., 2012). In addition, it should be considered that differences in plant morphological characteristics could provide distinct microhabitats, like the one around the stomata, influencing bacterial community composition too. In agaves, further work is necessary to understand whether differences in major bacterial groups in their episphere are due to the chemistry of the exudates, or if the morphology of the surface tissues plays a role on this phenomenon.
The endosphere of agaves (re-le) was primarily influenced by the season. However, data clustering was not as clear as in the epiphytic communities. This is consistent with the results found by Gottel et al. (2011), where bacterial rhizosphere samples were highly clustered compared with the more variable endosphere samples. The endosphere of the wild A. salmiana was dominated by a single OTU, the α-Proteobacteria: Reyranella (OTU 68), a novel genus with only three species described to date (Pagnier et al., 2011; Kim et al., 2013; Lee & Whang, 2014). This taxon was also present in A. tequilana, but in this domesticated Agave, some members of the γ-Proteobacteria accounted for the majority of the endophytic community. Despite the higher variability of the endophytic communities, we identified a group of fifteen OTUs shared among the endosphere of the two Agave species during the dry season. At this sampling time, plants had been submitted to c. 8 months of drought, where high temperatures and low levels of rainfall prevailed. This suggests that extreme environmental conditions, like those in arid regions, could play a role in shaping the structure and composition of the internal bacterial communities (Marasco et al., 2012). Moreover, we consider that the drought response of the endophytic bacterial communities could be similar among Agave species – explained by the same abundant taxa – and it is possible that these microorganisms may play a role in their fitness.
The endophytic dry core of agaves was mainly constituted by Enterobacteriaceae, which includes taxa that are known for promoting plant growth and fixing atmospheric nitrogen even in the phyllosphere (Kneip et al., 2007; Fürnkranz et al., 2008). This core also includes less-investigated members such as Reyranella, Acidobacteria, and even an unclassified bacterium, but also better studied genera such as Streptomyces and Stenotrophomonas. Unraveling core microorganisms has been proposed as an essential step to understand the microbial community ecology, as microorganisms that are shared between different communities are likely playing a key role in the ecosystem function (Shade & Handelsman, 2012; Shakya et al., 2013). After identifying the endophytic dry core by our molecular analyses, we were able to confirm that some of the native diazotrophic bacteria we had characterized and which mainly belong to Enterobacteriaceae and to Stenotrophomonas are indeed members of the endophytic dry core. This suggests that these bacterial strains may help the plants acquiring nitrogen in the poor soils they grow in. Furthermore, it is also likely that these strains may have some other functional traits that could benefit plants under harsh environmental conditions.
Native diazotrophic bacteria associated with Agave included also α-Proteobacteria, Firmicutes, and Actinobacteria. This finding is similar to studies in the gramineae Lolium multiflorum (Castanheira et al., 2014) where the same taxa were isolated too. Notably, the diazotrophic bacteria associated with agaves are able to use the complex fructans as the only carbon source, which indicates a degree of adaptation to these plant species. The confirmed diazotrophic strains recovered from A. salmiana samples (re, rz and p) were constituted by four distinct bacterial classes, while native diazotrophic bacteria from A. tequilana (re and rz) belonged only to one. This may suggest a higher bacterial diversity in this wild Agave. Remarkably, we also confirmed the diazotrophic activity of a Belnapia-like bacterium obtained only from the phyllosphere of A. salmiana. This fact is relevant for two reasons: first, it suggests that biological nitrogen fixation in Agave may take place not only in the roots, but also in the aerial parts of the plant; and second, reports on closely related strains have demonstrated that Belnapia spp., which occur in soil crusts, may be responsible for carbon cycling in nutrient poor arid soils, as some of their members are anoxygenic aerobic phototrophs (Csotonyi et al., 2010).
All together, we consider that these native diazotrophs and endophytic dry core microorganisms represent promising candidates for further functional studies in planta. Such studies, which are currently in progress in our research group, will allow us evaluate the impact of such microorganisms in the development, productivity, and overall health of agaves in the first place. Moreover, genome sequencing of some of these microorganisms will provide a baseline to explore and understand the molecular mechanisms they may have and use for a successful interaction with Agave species.
Acknowledgements
This project was funded by CONACyT Grant CB-2010-01-151007 to L.P.P.-M. We also thank CONACyT for the Postdoctoral and PhD fellowships awarded to E.G. and D.D., respectively. We thank M.C. Alejandro Nava Cedillo, who help us identified most suitable sampling sites and also trained us in the identification of the Agave species. We acknowledge the support provided by Tequila Real de Penjamo and the support and financial aid provided by Casa Herradura-Brown Forman, as well as the technical assistance of María Nélida Vázquez Sánchez and José Antonio Vera Núñez. We are also grateful to Prof. Dr Luis E. Eguiarte for his critical comments on an early version of the manuscript and to the two anonymous reviewers and editor for their constructive comments on our manuscript. Authors declare no conflict of interest.
References
Author notes
Present address: Etzel Garrido, Department of Entomology, Cornell University, Ithaca, NY, USA
Editor: Angela Sessitsch
CONACyT
CB-2010-01-151007
Casa Herradura-Brown Forman