-
PDF
- Split View
-
Views
-
Cite
Cite
Massimiliano Cardinale, João Vieira de Castro, Henry Müller, Gabriele Berg, Martin Grube, In situ analysis of the bacterial community associated with the reindeer lichen Cladonia arbuscula reveals predominance of Alphaproteobacteria, FEMS Microbiology Ecology, Volume 66, Issue 1, October 2008, Pages 63–71, https://doi.org/10.1111/j.1574-6941.2008.00546.x
- Share Icon Share
Abstract
The diversity and spatial pattern of the bacterial community hosted by the shrub-like reindeer lichen Cladonia arbuscula were investigated by general DNA staining and FISH, coupled with confocal laser scanning microscopy (CLSM). Using an optimized protocol for FISH using cryosections of small lichen fragments, we found about 6 × 107 bacteria g−1 of C. arbuscula. Approximately 86% of acridine orange-stained cells were also stained by the universal FISH probe EUB338. Using group-specific FISH probes, we detected a dominance of Alphaproteobacteria (more than 60% of all bacteria), while the abundance of Actinobacteria and Betaproteobacteria was much lower (<10%). Firmicutes were rarely detected, and no Gammaproteobacteria were present. Bacterial cells of different taxonomic groups are embedded in a biofilm-like, continuous layer on the internal surface of the C. arbuscula podetia, mainly occurring in small colonies of a few to a few hundred cells. The other parts of the lichen showed a lower bacterial colonization. α-proteobacterial 16S rRNA genes were amplified using total DNA extracts from C. arbuscula and separated by single-strand conformation polymorphism (SSCP). Sequencing of excised bands revealed the dominance of Acetobacteraceae.
Introduction
Lichens are highly integrated associations of fungi and photoautotrophs (algae and/or cyanobacteria). Suitable photoautotrophs are required by the fungus to develop the characteristic symbiotic phenotypes. The resulting thalli have diverse macroscopic shapes and are in most cases highly structured, with several functionally and anatomically different layers. Some of these fungal-determined morphologies represent what might perhaps be the most complex structures that can be formed by fungi. Lichens are also unique because the physiological integration of organisms provides conditions for persistance under extreme environmental situations that would not favour accumulation of the partners in biological solitude. In contrast to many fungi, which reside inside their substrates, lichens expose their vegetative parts at the surface of the substrates to harvest sunlight. Lichens grow very slowly, in the range of a few millimetres per year, but they can also survive for thousands of years, and represent a reasonably stable ecological niche for further microorganisms.
The presence of nonphotosynthetic bacteria in lichens has been detected some time ago (see references in Cardinale et al., 2006). These early reports were all based on isolated cultures and nonmolecular approaches for further characterization. DNA sequence data or fingerprinting approaches have only recently been used to characterize culturable bacteria in lichens (Cardinale et al., 2006; Liba et al., 2006), and fingerprinting approaches of the unculturable fraction suggested that diverse bacteria are present in lichens (Gonzáles et al., 2005; Cardinale et al., 2006). No study has so far focused on the localization of bacterial populations in the lichen thalli. Few studies included electron microscopic images of bacteria in lichen thalli (e.g. Meier & Chapman, 1983; Souza-Egipsy et al., 2002; De los Ríos et al., 2005), which gave the impression that bacteria are sporadic in the investigated lichens.
In this study, we propose a general DNA-staining technique using acridine orange, for the rapid assessment of bacterial abundances, and apply FISH for more detailed investigation. Using this approach, we illustrate the spatial pattern of bacterial diversity in the lichen Cladonia arbuscula. This lichen produces erect and branched thalli (named podetia) that are several centimetres high. We chose this species because it is a common representative of the shrub-like reindeer lichens, which are of ecological significance in the ground layer of subalpine–alpine, boreal and subarctic habitats of the Northern Hemisphere.
In situ hybridization experiments using fluorescence-labelled nucleic acid probes that are complementary to target signature regions of rRNA have become a golden standard for localization of bacteria in many contexts (DeLong et al., 1989; Amann et al., 1995; Wagner et al., 2003). The complexity of the lichen symbiosis, however, requires special considerations. Autofluorescence of lichen metabolites, of fungal hyphae, or algal chlorophylls can be a major nuisance in FISH experiments. In addition, the strongly conglutinated extracellular matrix of hyphae in lichen thalli interferes with the adherence of cryosectioned samples to the precoated slides. We developed and applied a modified protocol and propose optimized parameters for in situ hybridization experiments for lichens and other complex fungal structures.
Materials and methods
Sampling and fixation conditions
Lichens were collected at the Koralpe mountain ridge (Handalm) in the South of the province Styria, Austria, at an elevation of c. 1700 m. Samples were carefully taken using sterile forceps and immediately placed in sterile bags, at environmental temperature. Several individual thalli were always taken in order to have statistically representative samples. Lichen samples were quickly washed in phosphate-buffered saline (PBS) and fixed within 6 h after collection in 3 : 1 4% paraformaldehyde solution/PBS for 12 h at 4 °C, and then washed three times in ice-cold PBS before storage at −20 °C in 1 : 1 ice-cold PBS/ice-cold 96% ethanol. A subset of the samples was quickly washed in PBS, placed into ice and then directly fixed in 1 : 1 ice-cold PBS/ice-cold 96% ethanol and immediately stored at −20 °C. Nonfixed (fresh) subsamples were quickly washed in PBS and immediately stained with a general dye for fluorescent detection of nucleic acids (see ‘Nucleic acid staining’), in order to compare the bacterial colonization with that of fixed samples. For each of the staining experiments, we analysed at least 20 different lichen sections. Fresh lichen subsamples were also used for the extraction of metagenomic DNA [see ‘Single-strand conformation polymorphism (SSCP) analysis’].
Nucleic acid staining
Cryosections of both fresh and fixed lichen samples were stained with acridine orange, 4,6-diamino-2-phenyl indole (DAPI) and BacLight green, to compare the suitability of the stains. Acridine orange (Molecular Probes Inc., Eugene, OR) was used at 1 mg mL−1 in 1 : 3 permeabilizing solution PS (0.1% Triton X-100, 80 mM HCl, 150 mM NaCl)/staining solution STS (37 mM citric acid, 126 mM Na2HPO4, 150 mM NaCl, 1 mM Na2EDTA) or 1 : 3 : 1 PS/STS/5 M NaCl, as described by Bertaux et al. (2005). DAPI was used at 0.7 μg mL−1 in PBS. BacLight green (Invitrogen, Carlsbad, CA) was used at 0.2 μM, following the manufacturer's instructions. All incubations were performed at room temperature in the dark for 30 min (acridine orange and DAPI) to overnight (BacLight green). The samples were observed with the confocal laser scanning microscope (CLSM).
FISH probes and fluorochromes
Detailed information about the probes used for FISH is provided in Table 1. The stringency conditions for optimal staining are also reported therein. FITC-, Cy3- and Cy5-labelled probes or 6FAM-, Cy3- and Cy5-labelled probes were applied sequentially or simultaneously depending on the required formamide concentrations. Samples stained with multiple probes were simultaneously observed, taking advantage of the nonoverlapping emission wavelengths of the fluorochromes (max. excitation/emission in nm: 6FAM 492/518, FITC 490/525, Cy3 548/562 and Cy5 650/670).
Name | Sequence (5′–3′) | Target | Formamide concentration | Reference |
EUB338 | gctgcctcccgtaggagt | Most bacteria | 10–20 | Amann et al. (1990) |
EUB338II | gcagccacccgtaggtgt | Planctomycetales | 10–20 | Daims et al. (1999) |
EUB338III | gctgccacccgtaggtgt | Verrucomicrobiales | 10–20 | Daims et al. (1999) |
ALF968 | ggtaaggttctgcgcgtt | Alphaproteobacteria, except Rickettsiales | 45 | Neef (1997) |
BET42a | gccttcccacttcgttt | Betaproteobateria | 45 | Manz et al. (1992) |
GAM42a | gccttcccacatcgttt | Gammaproteobacteria | 45 | Manz et al. (1992) |
LGC354A | tggaagattccctactgc | Firmicutes (low G+C Gram-positive bacteria) | 35 | Meier et al. (1999) |
LGC354B | cggaagattccctactgc | Firmicutes (low G+C Gram-positive bacteria) | 35 | Meier et al. (1999) |
LGC354C | ccgaagattccctactgc | Firmicutes (low G+C Gram-positive bacteria) | 35 | Meier et al. (1999) |
HGC236 | aacaagctgataggccgc | Actinobacteria (high G+C Gram-positive bacteria) | 20 | Erhart et al. (1997) |
Burkho | accctctgttccgaccat | Burkholderia spp. | 40 | Hogardt et al. (2000) |
ARCH915 | gtgctcccccgccaattcct | Archaea | >60 | Stahl & Amann (1991) |
Univ-1390 | gacgggcggtgtgtacaa | All organisms | 5 | Zheng et al. (1996) |
NONEUB | actcctacgggaggcagc | – | ‡ | Wallner et al. (1993) |
Name | Sequence (5′–3′) | Target | Formamide concentration | Reference |
EUB338 | gctgcctcccgtaggagt | Most bacteria | 10–20 | Amann et al. (1990) |
EUB338II | gcagccacccgtaggtgt | Planctomycetales | 10–20 | Daims et al. (1999) |
EUB338III | gctgccacccgtaggtgt | Verrucomicrobiales | 10–20 | Daims et al. (1999) |
ALF968 | ggtaaggttctgcgcgtt | Alphaproteobacteria, except Rickettsiales | 45 | Neef (1997) |
BET42a | gccttcccacttcgttt | Betaproteobateria | 45 | Manz et al. (1992) |
GAM42a | gccttcccacatcgttt | Gammaproteobacteria | 45 | Manz et al. (1992) |
LGC354A | tggaagattccctactgc | Firmicutes (low G+C Gram-positive bacteria) | 35 | Meier et al. (1999) |
LGC354B | cggaagattccctactgc | Firmicutes (low G+C Gram-positive bacteria) | 35 | Meier et al. (1999) |
LGC354C | ccgaagattccctactgc | Firmicutes (low G+C Gram-positive bacteria) | 35 | Meier et al. (1999) |
HGC236 | aacaagctgataggccgc | Actinobacteria (high G+C Gram-positive bacteria) | 20 | Erhart et al. (1997) |
Burkho | accctctgttccgaccat | Burkholderia spp. | 40 | Hogardt et al. (2000) |
ARCH915 | gtgctcccccgccaattcct | Archaea | >60 | Stahl & Amann (1991) |
Univ-1390 | gacgggcggtgtgtacaa | All organisms | 5 | Zheng et al. (1996) |
NONEUB | actcctacgggaggcagc | – | ‡ | Wallner et al. (1993) |
The indicated concentrations of formamide are intended for hybridizations at 41°C.
Used together in equimolar concentration.
Used as negative control at the same formamide concentration as that used for the positive FISH probe.
Name | Sequence (5′–3′) | Target | Formamide concentration | Reference |
EUB338 | gctgcctcccgtaggagt | Most bacteria | 10–20 | Amann et al. (1990) |
EUB338II | gcagccacccgtaggtgt | Planctomycetales | 10–20 | Daims et al. (1999) |
EUB338III | gctgccacccgtaggtgt | Verrucomicrobiales | 10–20 | Daims et al. (1999) |
ALF968 | ggtaaggttctgcgcgtt | Alphaproteobacteria, except Rickettsiales | 45 | Neef (1997) |
BET42a | gccttcccacttcgttt | Betaproteobateria | 45 | Manz et al. (1992) |
GAM42a | gccttcccacatcgttt | Gammaproteobacteria | 45 | Manz et al. (1992) |
LGC354A | tggaagattccctactgc | Firmicutes (low G+C Gram-positive bacteria) | 35 | Meier et al. (1999) |
LGC354B | cggaagattccctactgc | Firmicutes (low G+C Gram-positive bacteria) | 35 | Meier et al. (1999) |
LGC354C | ccgaagattccctactgc | Firmicutes (low G+C Gram-positive bacteria) | 35 | Meier et al. (1999) |
HGC236 | aacaagctgataggccgc | Actinobacteria (high G+C Gram-positive bacteria) | 20 | Erhart et al. (1997) |
Burkho | accctctgttccgaccat | Burkholderia spp. | 40 | Hogardt et al. (2000) |
ARCH915 | gtgctcccccgccaattcct | Archaea | >60 | Stahl & Amann (1991) |
Univ-1390 | gacgggcggtgtgtacaa | All organisms | 5 | Zheng et al. (1996) |
NONEUB | actcctacgggaggcagc | – | ‡ | Wallner et al. (1993) |
Name | Sequence (5′–3′) | Target | Formamide concentration | Reference |
EUB338 | gctgcctcccgtaggagt | Most bacteria | 10–20 | Amann et al. (1990) |
EUB338II | gcagccacccgtaggtgt | Planctomycetales | 10–20 | Daims et al. (1999) |
EUB338III | gctgccacccgtaggtgt | Verrucomicrobiales | 10–20 | Daims et al. (1999) |
ALF968 | ggtaaggttctgcgcgtt | Alphaproteobacteria, except Rickettsiales | 45 | Neef (1997) |
BET42a | gccttcccacttcgttt | Betaproteobateria | 45 | Manz et al. (1992) |
GAM42a | gccttcccacatcgttt | Gammaproteobacteria | 45 | Manz et al. (1992) |
LGC354A | tggaagattccctactgc | Firmicutes (low G+C Gram-positive bacteria) | 35 | Meier et al. (1999) |
LGC354B | cggaagattccctactgc | Firmicutes (low G+C Gram-positive bacteria) | 35 | Meier et al. (1999) |
LGC354C | ccgaagattccctactgc | Firmicutes (low G+C Gram-positive bacteria) | 35 | Meier et al. (1999) |
HGC236 | aacaagctgataggccgc | Actinobacteria (high G+C Gram-positive bacteria) | 20 | Erhart et al. (1997) |
Burkho | accctctgttccgaccat | Burkholderia spp. | 40 | Hogardt et al. (2000) |
ARCH915 | gtgctcccccgccaattcct | Archaea | >60 | Stahl & Amann (1991) |
Univ-1390 | gacgggcggtgtgtacaa | All organisms | 5 | Zheng et al. (1996) |
NONEUB | actcctacgggaggcagc | – | ‡ | Wallner et al. (1993) |
The indicated concentrations of formamide are intended for hybridizations at 41°C.
Used together in equimolar concentration.
Used as negative control at the same formamide concentration as that used for the positive FISH probe.
In tube FISH
Small fragments (10–15 mm length) of fixed thalli were embedded in water, rapidly frozen at −25 °C and cut into 30-μm-thick sections with a cryotome. Sections from different thalli were collected separately. Some fragments were embedded in O.C.T.™ Tissue-Tek® (Sakura, Finetech Europe BV, Zoeterwoude, The Netherlands), which prevents tissue damage. Comparison with the latter was used to exclude the possibility of artefacts with water-embedded samples. The cryosections were directly placed in a 1.5-mL microcentrifuge tube (without mounting on slides) and incubated with 1 mg mL−1 lysozyme (Sigma-Aldrich, Steinheim, Germany) for 10 min at room temperature, to increase the bacterial cell wall permeability for the FISH probes. The samples were then rinsed twice with ice-cold PBS. An ethanolic series (50–70–96% ethanol solutions, 3 min each) was carried out in the same tube. The samples were quickly rinsed with ice-cold PBS and washed in ice-cold PBS for 3 min at room temperature. All hybridizations were performed at 41 °C for 2–3 h in a buffer containing 0.9 M NaCl, 0.02 M Tris-HCl, pH 8, 0.01% vol sodium dodecyl sulphate (SDS) and 1.5–2.5 ng μL−1 of each used probe. The concentration of ultrapure formamide™ (Invitrogen) was adjusted individually for the respective FISH probe. Double-distilled sterile water (Fresenius Kabi, Graz, Austria) was added up to an appropriate volume until the lichen sections were completely immersed (usually 100–200 μL). Different concentrations of formamide and incubation temperatures were tested to assess the optimal parameters. The hybridization buffer was immediately removed and the samples were rinsed twice with prewarmed (42 °C) washing buffer, containing 0.02 M Tris-HCl, pH 8, and 30–450 mM NaCl (depending on the concentration of formamide in the hybridization buffer). The washing buffer was adjusted to 5 mM EDTA when formamide concentration exceeded 20%. The samples were incubated in the washing buffer in a water bath for 10–15 min at a temperature 1 °C above the hybridization temperature. The washing buffer was removed and the samples were rinsed with ice-cold water. The sections were incubated with a 5 μM PBS solution of the general nucleic acid stain Sytox Blue (Molecular Probes Inc.; maximum excitation/emission 444/480 nm; no overlap with any of the emission wavelengths of the FISH probe fluorochromes) for 1 h at room temperature in the dark. The samples were then rinsed with ice-cold water. The sections were finally placed on a regular microscopic glass-slide and quickly dried with compressed air. The sections were immediately mounted with ProLong Gold antifadent (Molecular Probes), incubated for 24 h at room temperature as recommended by the manufacturer and finally observed under the CLSM.
CLSM
Stained lichens were observed with a Leica TCS SP confocal laser-scanning microscope (Leica Microsystems, Heidelberg, Germany) equipped with argon and helium/neon lasers. Three confocal light channels were observed simultaneously along with further bright-field channel. Filter settings were adjusted to achieve the maximum signal from each fluorochrome (the detection of some sample autofluorescence was often impossible to avoid). Up to three FISH probe signals were observed simultaneously (with or without the bright-field image) and compared with the image from Sytox Blue staining only. Maximum projections of an appropriate number of 0.8–1-μm-depth optical slices were applied to visualize the lichen sections (confocal stacks). Up to 15 scans per optical slice were averaged to improve the image resolution and to reduce noise. The digital images were further observed and analysed with the NIH imagej software implemented with suitable plugins (http://rsbweb.nih.gov/ij/docs/index.html). Bacterial cells were manually counted using the ‘point picker’ plugin (http://bigwww.epfl.ch/thevenaz/pointpicker/). Images showing a high number of ambiguous objects or a high noise-to-signal ratio were excluded from the analysis. The software imaris 6.0 (Bitplane, Zurich, Switzerland) was used for three-dimensional (3D) rendering of confocal stacks.
Single-strand conformation polymorphism (SSCP) analysis
The SSCP analysis was carried out according to Schwieger & Tebbe (1998). Metagenomic DNA extracted from fresh lichen samples (see ‘Sampling strategy’ above) was used as a template for the PCR using Alphaproteobacteria-specific primers ADF 681F (5′-AGTGTAGAGGTGAAATT-3′) (Blackwood et al., 2005). A semi-nested PCR was performed using the primer pair ADF 681F/1492r (5′-TACGGYTACCTTGTTACGACTT-3′) for the first PCR and the primer pair ADF 681F/927r (5′-CCCGTCAATTYMTTTGAGTT-3′) for the second PCR. Dominant bands were excised from SSCP gels as described by Schwieger & Tebbe (1998). Gel-extracted DNA was reamplified and cloned as described by Opelt & Berg (2004). For phylogenetic analysis and identification of related sequences, the blast algorithm according to Altschul et al. (1997) was used. The sequences obtained from SSCP bands are available in GenBank/EMBL/DDBJ database under accession numbers AM944536–AM944545.
Results
Nucleic acid staining and assessment of bacterial density
Aspecific staining of nucleic acids in sectioned C. arbuscula using acridine orange indicates that the abundance of bacterial colonization of lichens varied among areas of lichen thallus. The quality of the images was better as compared with both DAPI and BacLight green staining. The high cytoplasmic content of bacterial RNA allowed a well-distinguishable staining of the bacterial cells. The lichen medulla mainly showed a green colour, resulting from cytoplasmic autofluorescence, some aspecific DNA staining and weak staining of hyphal walls (Fig. 1). Furthermore, the chlorophyll autofluorescence allowed us to detect algal cells easily (Fig. 1). Dense bacterial colonization is particularly seen on the internal surface of the cylindric thalli of C. arbuscula (Fig. 1). The external surfaces were less abundantly colonized, either by single bacteria or by small clusters of cells attached to fungal hyphae (Fig. 2). In some cases, we detected bacteria inside hyphae, between the outer cell wall and the cytoplasm (Fig. 2b). Different morphotypes were clearly detected (Fig. 2b). We calculated an average density of 6.27±4.37 × 107 bacteria g−1C. arbuscula thallus. The highest density of bacterial colonization (8.9±1.6 × 104 bacteria mm−2, corresponding to 2.1±0.38 × 108 bacteria g−1 thallus) was found on the internal layer on C. arbuscula podetia, where bacterial populations colocalized in a biofilm-like continuous layer (Fig. 1). Other nonprokaryotic microorganisms were also detected on the surfaces, including fungal spores not belonging to the lichen mycobiont and protists (data not shown).
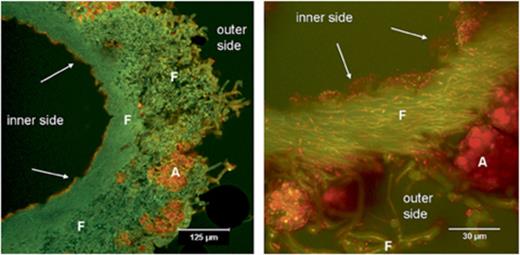
Acridine orange-stained transversal sections of Cladonia arbuscula showing bacterial colonization on the internal surface and also to a lower extent on the outside (CLSM image). Left panel, overview; right panel, detail. A, algal cells; F, fungal hyphae; arrows, bacteria.
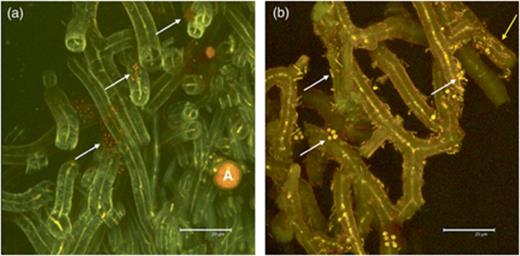
Acridine orange-stained sections of Cladonia arbuscula showing bacteria on hyphae of the external lichen surface (CLSM image). Thin yellow central lines in hyphae represent the cytoplasm of the fungus. (a) Clusters of bacterial colonies. (b) Colonization by different bacterial morphotypes. A, algal cells; white arrows, bacteria adhering to the hyphae; yellow arrow, bacteria inside hyphae.
In-tube FISH and assessment of bacterial diversity
In-tube FISH was used to overcome the detachment problem of the lichen sections from the precoated slides. All FISH steps were performed in a single 1.5-mL tube by exchanging the solutions with a 20–200-μL micropipette and filter tips. The hybridization temperature of 41 °C proved to be optimal. Higher hybridization temperatures led to the production of autofluorescent aggregates of unknown nature and origin in the gelatinous matrix of the conglutinated hyphal network. The optimal concentration of formamide for each probe is reported in Table 1. The conglutinate nature of the lichen thallus preserved the integrity of the sample during the sectioning and the hybridization/washing steps. In fact, no differences in terms of number of cells and distribution pattern were detected between the fresh samples and fixed samples and between the samples embedded in O.C.T.™ Tissue-Tek® compound before the cryosectioning and the samples embedded in water. With in-tube FISH using universal bacterial probes EUB338MIX and CLSM, we could detect 85.8±16.8% of the acridine orange-stained cells and confirm the biofilm-like structure of the bacterial community (Fig. 3). Negative controls with a nonsense FISH probe (NONEUB, Table 1) were always without positive signals. Using FISH probes targeting different bacteria, we detected the occurrence of distinguishable bacterial cells belonging to different phylogenetic groups (Fig. 4) that can form neighbouring or mixed colonies. The dominant group was Alphaproteobacteria, with a percentage of 75.0±12.0% of the EUB338MIX-stained cells (Fig. 5); other bacterial groups were Actinobacteria (5.7±2.9%, Fig. 3) and Betaproteobacteria (4.4±1.2%). Only in a few cases was stratification of different populations visible (Fig. 6). Firmicutes were only present in small numbers, in a number of images too low to allow a statistically supported count. No Gammaproteobacteria or Archaea were detected in any lichen sample. 13.3±1.4% of EUB338MIX-stained cells remained unidentified.
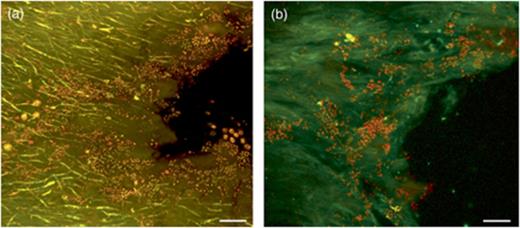
Detection of bacteria by acridine orange and FISH in Cladonia arbuscula (diagonal sections of inner surface, CLSM images). (a) Acridine orange: bacterial cells are yellow-orange particles. (b) EUB338MIX-Cy3 and HGC236-Cy5 bacterial FISH probes: Actinobacteria are yellow objects, and other bacterial cells are red. Scale bars are 15 μm.
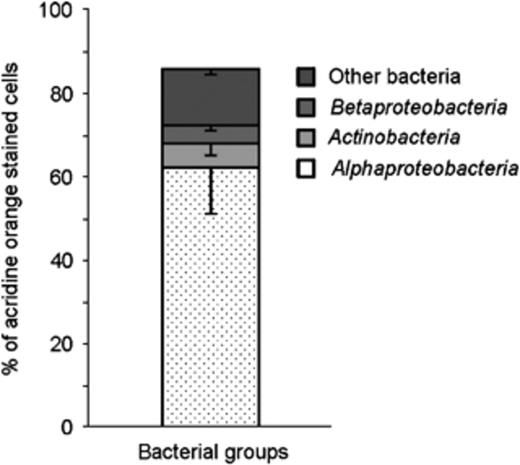
Abundance of bacterial groups in Cladonia arbuscula revealed by FISH and CLSM image analysis of FISH experiments.

Predominance of Alphaproteobacteria in Cladonia arbuscula as detected by simultaneous use of EUB338MIX-Cy3 and ALF968-Cy5 FISH probes (CLSM images). (a) Transversal and (b) tangential sections. Pink, Alphaproteobacteria (resulting from a positive signal with both probes); red, other bacteria (stained by EUB338MIX-Cy3 only); green, chlorophyll autofluorescence; yellow/brown, fungal autofluorescence; A, algal cells; F, fungal hyphae. Scale bars are 10 μm. (c, d) 3D reconstructions (formed by spots and isosurfaces) of the images in (a) and (b), respectively. Red/blue spots, Alphaproteobacteria; red spot, other bacteria; yellowish isosurface, fungi; green isosurface, algal cells.
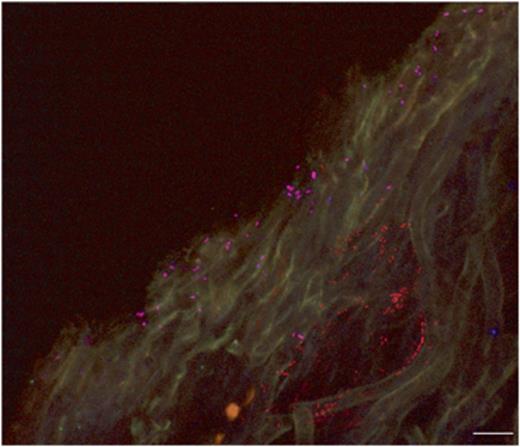
Stratified bacterial colonies in the inner surface of Cladonia arbuscula as detected by simultaneous use of EUB338MIX-Cy3 and ALF968-Cy5 FISH probes (CLSM image of transversal section). Pink, Alphaproteobacteria (stained by the universal bacterial probe EUB338MIX-Cy3 and the specific probe ALF968-Cy5); red, other bacteria (stained by EUB338MIX-Cy3 only). Scale bar is 10 μm.
SSCP analysis
16S-SSCP profiles containing between five and seven bands of different intensity were obtained using specific primers for Alphaproteobacteria (data not shown). Representative samples of the four major bands were excised, reamplified and sequenced. blast searches with three distinct sequences revealed a relationship with members of the family Acetobacteraceae, close to the genera Gluconacetobacter, Acidisphaera, and also to the recently proposed new species Rhodovastum atsumiense (GenBank accession no.: AB381935, unpublished). The remaining distinct sequence was not identified at the genus level, and it matched only with unculturable Alphaproteobacteria of the SAR11 cluster (Stingl et al., 2007). Sequences obtained from dominant SSCP bands of Acetobacteraceae from two different sampling times were identical.
Discussion
Although the presence of bacteria in lichen symbioses has been detected previously, still little is known so far about their diversity and specific localization within the lichen structure. Here, we show that a microscopic approach is suitable to properly interpret data obtained from culture-based approaches or from analyses of unculturable fractions. Cardinale (2006) studied the diversity of culturable and unculturable bacteria associated with several Cladonia spp., including the reindeer lichen Cladonia rangiferina, by sequencing the 16S rRNA gene of pure cultures isolated from both internal and external parts of the lichen thalli. Common phylotypes represented genera of Firmicutes and Betaproteobacteria, whereas Actinobacteria were only found in Cladonia pyxidata. Differences in the structure of the culturable bacterial communities were observed among the lichen species, but some bacterial taxa were retrieved from different lichens sampled at the same or different sites. Strains of Paenibacillus and Burkholderia were particularly common and these bacterial genera are also involved in other fungal–bacterial associations of nonlichenized fungi (Bianciotto et al., 2000; Bertaux et al., 2003; Partida-Martinez & Hertweck, 2005). Further strains were found by sequencing the dominant bands obtained by ribosomal intergenic spacer analysis (RISA) using the DNA extracted from the same lichens (Cardinale et al., 2006). In other recent studies, a number of Gammaproteobacteria were found in lichens (Liba et al., 2006), while fingerprinting techniques also revealed a considerable diversity of Actinobacteria, especially in lichens from tropical habitats (Gonzáles et al., 2005). Lichens certainly represent a promising source of new bacterial species (e.g. Li et al., 2007). However, a thorough assessment of specificity and variation of the associations by considering the diversity of the hosts will provide new clues about the biology of lichens. To achieve this, the relative abundances of bacterial groups and their preferred location need to be studied directly in the host lichens (in situ). Here, a two-step approach elucidated the spatial distribution of bacteria in the reindeer lichen C. arbuscula.
First, we tested which of the aspecific DNA-staining methods is suitable to visualize bacteria and their general abundance in lichens. We found that acridine orange has the optimal properties for rapid general assessment of bacterial colonization. Second, for a more detailed investigation we optimized FISH with lichens. So far, FISH has only rarely been applied in lichens, e.g. when Grube & de los Rios (2001) visualized the presence of parasitic fungi in lichens. In that study, so-called microslides (small rectangular pieces cut out from cover-slips) were used to carry out the in situ hybridization with small volumes of liquids in PCR tubes. Only rather thin sections adhered properly to the microslides, while washing steps were still carried out with greatest care to avoid section detachment. Once transversal sections of more than 10 μm thickness are required, e.g. to obtain a better 3D representation of structures, detachment becomes a serious problem even with precoated slides. We suppose that the adherence is decreased due to the hygroscopic movements of the strongly conglutinated hyphal structures in lichens. The problem cannot be solved by stronger fixation of the material because this apparently decreases the permeability for the probes and makes targets inaccessible. Lichen sections resided on the slide after they were covered by a layer of 1% agarose (dried with compressed air), but this treatment led to the deposition of autofluorescent agarose residues, which cause artefacts in the CLSM analysis (data not shown). We therefore skipped the use of supporting slides during the FISH procedure. Taking advantage of the strong coherence of the lichen structures, we used thick sections (between 15 and 30 μm) in an approach designated here as in-tube FISH. We found that the integrity of the lichen structures and the distribution of the bacterial communities are more easily preserved than in material placed on slides. Furthermore, the possibility of adapting the reaction volume to the sample size allowed us to minimize the amount of chemicals and toxic wastes. This optimized FISH protocol was successfully tested with other lichen species, including those with different macroscopic organizations (M. Cardinale, unpublished data).
Our experiments revealed an abundant bacterial colonization in lichens, which range from single cells attached to fungal hyphae to dense biofilm-like aggregations. Group-specific probes showed that Alphaproteobacteria form a common component in the bacterial community associated with C. arbuscula. Interestingly, Alphaproteobacteria were hardly found in previous culture-dependent approaches (only Inquilinus, Cardinale et al., 2006, and Methylobacterium, unpublished data). This discrepancy could indicate that certain, not yet cultured Alphaproteobacteria require the lichen symbiosis for growth, whereas culturable bacteria could belong to a minor and perhaps less specific fraction in vital parts of the lichen. Moreover, sequences obtained after PCR amplification of excised major bands of SSCP gels using specific primers revealed that the dominant strains belong to Acetobacteraceae. The SSCP-derived sequences are not long enough for a thorough phylogenetic analysis, but their alignment with reference sequences indicated low sequence diversity. Lichen-associated strains could form a monophyletic cluster inside the Acetobacteraceae family, which suggests a possibly specific association between bacteria and lichen partners. Several examples of highly specific associations between Eukaryota and Alphaproteobacteria strains (e.g. Beier et al., 2002; Bertaux et al., 2005; Andam & Parker, 2007) and even members of Acetobacteraceae (Favia et al., 2007) are known. Further investigations are under way to assess the diversity of bacterial groups in other lichens collected from both the same or different sampling sites. These future works will use additional methods, including 16S rRNA gene clone library construction, which will more precisely represent the composition of the whole bacterial community, including the faction that was not identified here.
Our data and published images of bacteria in lichen thalli always show their presence in intercellular spaces and on surfaces (see e.g. Meier & Chapman, 1983; Souza-Egipsy et al., 2002; De los Ríos et al., 2005), but not inside the vital cells of the lichen symbionts. Bacteria inside hyphal cells have been described already in Ascomycota [Cytophaga—Flexibacter–Bacterioides phylogroup (CFB) bacterium in Tuber borchii, Barbieri et al., 2000], Basidiomycota (Paenibacillus in Laccaria bicolor, Bertaux et al., 2003), Glomeromycota (Burkholderia in Gigaspora and Scutellospora, Bianciotto et al., 2000) and Zygomycota (Burkholderia in Rhizopus, Partida-Martinez & Hertweck, 2005). We do not exclude the possibility of endocytoplasmatic bacteria in vital hyphae, but so far, we only have evidence for bacterial colonization within hyphal walls, external of the cytoplasm.
In this work, we present results for a shrub-like species that does not form an exploiting mycelium in the soil substrate. However, initial acridine orange staining experiments with lichen species that are tightly attached to the underlying substrates show a likewise considerable bacterial colonization (preliminary data). It will be interesting to explore differences of bacterial communities in a variety of lichens and their substrates, and to study how the abundance of bacterial colonization is controlled in lichens. One of several possible factors will be secondary lichen compounds with antibiotic effects. Peripheric parts of C. arbuscula thalli, with extracellular, crystallized usnic acid deposits on the surface of hyphae, harbour significantly fewer bacteria. Because usnic acid is well known for its antibiotic potential (Boustie & Grube, 2005), the lower abundance of bacteria is not surprising. Highly structured lichen thalli provide a variety of chemically distinct microniches for bacteria, and varying hydrophobicity of mycelial surfaces in lichens (Büdel & Scheidegger, 1996) may additionally influence the composition of associated bacterial communities.
We hypothesize that associated bacteria contribute significantly to the integrity and robustness of lichen biology. This contribution is perhaps not restricted to one particular function in the lichen system. We hypothesize that bacteria in lichens are parts of a complex functional network, which remains to be studied in the future.
Acknowledgements
We are grateful to Walter Obermayer for an introduction to confocal microscopy and technical support. The F.W.F. is acknowledged for financial support of G.B. and M.G.
References
Author notes
Editor: Jan Dirk van Elsas