-
PDF
- Split View
-
Views
-
Cite
Cite
Leo van Overbeek, Fedor Gassner, Carin Lombaers van der Plas, Pieter Kastelein, Ulisses Nunes–da Rocha, Willem Takken, Diversity of Ixodes ricinus tick-associated bacterial communities from different forests, FEMS Microbiology Ecology, Volume 66, Issue 1, October 2008, Pages 72–84, https://doi.org/10.1111/j.1574-6941.2008.00468.x
- Share Icon Share
Abstract
Nymphal Ixodes ricinus ticks (n=180) were collected from three different areas in the Netherlands to investigate the effect of forest composition on tick-associated microbial communities. Sampled habitats differed in thickness of leaf litter and humus layers and vegetation associations and were located near Amsterdam (Beech-Oak), Ede (Birch-Oak) and Veldhoven (Birch-Oak). Analysis of nine 16S rRNA gene clone libraries made from individual ticks showed nearest matches with presumed pathogens Candidatus Neoehrlichia mikurensis and Rickettsia australis and arthropod endosymbionts Wolbachia pipientis and Candidatus Midichloria mitochondrii. Total bacterial species diversity (Shannon index) and Borrelia species infections were determined in I. ricinus by, respectively, PCR-denaturing gradient gel-electrophoresis and PCR-reverse line blot with probes specific for Borrelia burgdorferi sensu stricto, Borrelia afzelii, Borrelia garinii, Borrelia valaisiana, Borrelia lusitaniae and Borrelia ruski. Bacterial diversity differed significantly per area and was lowest in Ede. In contrast, Borrelia species-infected ticks were more abundant in Ede, Candidatus Neoehrlichia mikurensis-infected ticks in Ede and Veldhoven, and R. australis-infected ticks in Amsterdam. Borrelia afzelii was the most common Borrelia species found in all three areas. Bacterial tick diversity was influenced by local differences in forest structure, which is proposed to modulate animal populations that are commonly parasitized by I. ricinus.
Introduction
Lyme borreliosis is a widespread phenomenon occurring in many different countries, especially in the Northern Hemisphere. The causative agents of Lyme borreliosis belong to a group of related Borrelia species within the class of Spirochaetes that are transmitted mainly via ticks of the genus Ixodes. Major agents causing disease in humans are Borrelia burgdorferi sensu stricto (ss), Borrelia afzelii and Borrelia garinii. Other Borrelia species closely related to this group are Borrelia valaisiana, Borrelia lusitaniae, Borrelia spielmanii and Borrelia bisettii. Currently, this group consists of at least 11 different species. Some of these species are also suspected to be human pathogens. The principal vector of Borrelia species in Europe is Ixodes ricinus (Rauter & Hartung, 2005). The prevalence of Borrelia species infections as well as the species composition in I. ricinus ticks are highly variable in time and space. Borrelia afzelii, for example, is often the most prevalent species found in I. ricinus (Rijpkema et al., 1995; Schouls et al., 1999; Jenkins et al., 2001; Fraenkel et al., 2002; Maetzel et al., 2005; Kipp et al., 2006; Wielinga et al., 2006). However, in some studies prevalence is lower or this species is even absent (Nohlmans et al., 1995; Kirstein et al., 1997; Kurtenbach et al., 1998a, b; De Michelis et al., 2000; Lenčková, 2006; Fingerle et al., 2007).
The location and presence of vertebrate animals that are parasitized by I. ricinus determine which Borrelia species prevails (Kurtenbach et al., 2006). Borrelia garinii and B. valaisiana were more common in I. ricinus ticks feeding on birds (Kurtenbach et al., 1998a, b; Comstedt et al., 2006; Kipp et al., 2006) and B. lusitaniae in ticks feeding on lizards (Richter & Matuschka, 2006; Amore et al., 2007). Small rodents play an important role in the transmission of B. afzelii, and birds and rodents in the transmission of B. burgdorferi ss from and to I. ricinus (Gray, 1998; Kurtenbach et al., 2002; Ginsberg et al., 2005; Rauter & Hartung, 2005). The geographic distribution of different Borrelia species in I. ricinus largely depends on local environmental factors. In particular, variations in populations of vertebrate animals that are parasitized by I. ricinus play an important role (Kurtenbach et al., 2006; Gassner & Van Overbeek, 2007).
Other bacterial species are also commonly found in I. ricinus, some of which are pathogenic to humans as well. Ehrlichia species were found in I. ricinus ticks collected in Germany (Hildebrandt et al., 2002), Norway (Jenkins et al., 2001; Stuen et al., 2006), Poland (Stanczak et al., 2004), the Netherlands (Schouls et al., 1999; Wielinga et al., 2006), Tunisia and Morocco (Sarih et al., 2005) and Switzerland (Leutenegger et al., 1999). The taxonomy of Ehrlichia and Anaplasma species has been changed (Dumler et al., 2001) and many species previously classified as Ehrlichia nowadays belong to Anaplasma. Anaplasma (Ehrlichia) phagocytophilum is the most commonly found Anaplasma species in I. ricinus. An Ehrlichia-like species (Schotti variant; Pan et al., 2003) was found in I. ricinus in the Netherlands (Schouls et al., 1999) and in rats and I. ovatus ticks in Japan and was renamed as Candidatus Neoehrlichia mikurensis (Kawahara et al., 2004). Other species detected in Ixodes ticks are Bartonella species (Schouls et al., 1999), Coxiella burnetii (Movila et al., 2006), Francisella tularensis (Wicki et al., 2000), Pasteurella species (Stojek & Dutkiewicz, 2004), Rickettsia species (Benson et al., 2004), Wolbachia pipientis (O'Neill et al., 1992; Benson et al., 2004; Sarih et al., 2005) and Candidatus Midichloria mitochondrii (Beninati et al., 2004; Sassera et al., 2006). Bacteria belonging to the group of Gammaproteobacteria were isolated on semi-selective media from I. ricinus ticks collected in Poland (Stojek & Dutkiewicz, 2004). These culturable bacteria taxonomically differ from the species commonly observed with molecular techniques. It is likely that different taxonomical groups in ticks can be accessed by application of different techniques.
Many bacteria present in I. ricinus are difficult to culture or are even unculturable. This makes characterization of bacteria present in ticks a daunting task. Molecular detection techniques are most suitable to determine which species are present in ticks. PCR-reverse line blot (RLB) hybridization of Borrelia species-specific oligonucleotides with Borrelia– genus-specific PCR amplicons made from total tick extracts is a commonly applied method to screen for the presence of different Borrelia species in ticks (Rijpkema et al., 1995). Later, probes specific for other species were included in the PCR-RLB set-up to allow screening for other pathogens as well (Gubbels et al., 1999; Schouls et al., 1999; Georges et al., 2001; Christova et al., 2003; Wielinga et al., 2006). PCR-RLB is most suitable for the screening of different bacterial groups expected to be present in ticks. DNA sequencing of random clone libraries made from PCR amplicons of tick DNA extracts is an option to screen for dominant bacterial groups present in Ixodes species (Benson et al., 2004). The advantage of DNA sequencing of clone libraries over PCR-RLB is that it allows detection of the most dominant species present, including those that had not been associated with I. ricinus previously. Molecular fingerprinting techniques in combination with 16S rRNA gene sequencing were applied to determine species diversity in ticks and to elucidate the most dominant groups present in ticks (Schabereiter-Gurtner et al., 2003; Halos et al., 2006; Moreno et al., 2006). Molecular fingerprinting techniques can be used to investigate shifts in microbial communities in I. ricinus, e.g. from individuals collected at different locations.
The prevalence of many other pathogenic and nonpathogenic bacteria present in I. ricinus varies depending on geographic location, as for Borrelia species. Local environmental factors affecting the survival and establishment of different groups of animals that can act as hosts for I. ricinus will influence the microbial community composition in I. ricinus. The aim of this study was to investigate to what extent geographically separated and structurally different habitats can influence bacterial composition in I. ricinus. From other studies, it is already known that different habitats can influence the distribution of different pathogens in I. ricinus (Kirstein et al., 1997; Rauter & Hartung, 2005; Wielinga et al., 2006). It is, however, unknown whether this is the case for other bacterial groups present in I. ricinus. Information about environmental factors affecting bacterial communities in I. ricinus is important to elucidate factors that contribute to the transmission of pathogens from vertebrate animals to I. ricinus. The establishment and survival of pathogens in I. ricinus is expected to be mediated by other microorganisms residing in ticks. The present study focuses on potential differences in bacterial communities in ticks collected from different habitats in the Netherlands using PCR-denaturing gradient gel-electrophoresis (DGGE). Concomitantly, these ticks were also screened for the presence of Borrelia species by PCR-RLB. From a selection of these ticks, the most dominant bacterial groups were identified by 16S rRNA gene PCR amplification, sequencing and blast-assisted database searches.
Materials and methods
Location and characterization of tick sampling areas
Three forest habitats from different places in the Netherlands were selected on the basis of frequent reports on the presence of I. ricinus ticks. These areas were Amsterdam water supply dunes (A), Ede, deSysselt (E) and Veldhoven, Hoogeind (V). Within each area, two locations covering an area of 20 m × 70 m were chosen for tick collections and the GPS coordinates were W 9° 98.63′ and N 48° 39.64′ for A1; W 10° 01.18′ and N 48° 46.67′ for A2; W 17° 56.71′ and N 44° 88.93′ for E1; W 17° 58.45′ and N 44° 88.02′ for E2; W 15° 13.45′ and N 38° 16.36′ for V1; and W 15° 02.19′ and N 38° 16.48′ for V2. For each location, local vegetation was described by estimating the coverage of different layers of plant species. Three vegetation layers were considered as most relevant: a soil covering layer containing mosses, grasses and herbs, an intermediate layer containing all shrubs and a tree layer. Vegetation type was classified in accordance with a modified system of Braun-Blanquet (1928), described in Stortelder et al. (1999), which is a common system to classify different vegetation types into associations.
Forest floor composition at all six locations was determined by measuring leaf litter and humus layers at four different places per location. Composite samples of 100 g per location were drawn, both from the leaf litter and from the humus layers. From all samples pH was measured in suspensions made of 20 g subsamples in 20-mL demineralized water. Dry weights and organic matter contents were determined from 10 g subsamples that were heated overnight at 110 and 550 °C, respectively. Differences in weight before and after heat treatments were used for calculations of percentages of dry weight and organic matter content.
Collection of ticks
Ticks were collected on three successive days during daytime (between 8 am and 1 pm) in October 2006. The period before sampling was relatively warm and dry for over one month with daily oscillating temperatures between 18 and 22 °C during the day, and 8 and 15 °C at night. In each location four tracts of 50 m were chosen, with a maximum distance of 2 m between each tract. Ticks were collected by dragging a 1 m2 white cotton blanket over the vegetation layer, and in each location, a total of 200 m2 was sampled. Every 25 m, the blanket was visually inspected for the presence of ticks. The ticks were removed from the blanket and transferred into 70% ethanol. The developmental stage of each tick was determined and all ticks were stored in vials containing 70% ethanol at 4 °C for no longer than 1 month before DNA extraction and molecular analyses.
DNA extraction from ticks
Total DNA was extracted from 30 individual nymphal ticks per location, resulting in a total of 180 ticks from all six locations. Total DNA was extracted according to the procedure described in Schouls et al. (1999). Briefly, ticks were removed from the vials and dried for 20 min on tissue paper. Individual ticks were then transferred to Eppendorf tubes containing 100 μL of 0.7 M NH4OH, boiled for 20 min and immediately chilled on ice. Tubes were centrifuged at 10 000 g for 20 s and heated at 90 °C with open caps for 20 min to allow ammonia to evaporate. Supernatants containing tick DNA were immediately stored at −80 °C until further analyses.
DNA amplification and molecular analysis
Total tick DNA was amplified with Phi 29 (Amersham Biosciences, NJ) before further analyses. Therefore, 0.5 μL (c. 5 ng of DNA) of tick DNA extract was included in a volume of 4.5 μL reaction mixture with Phi 29 and amplified, according to the protocol provided by the manufacturer.
Construction of tick clone libraries and database comparisons
Phi 29-amplified tick DNA samples from nine ticks (three from each area) were PCR amplified using the primers 27F and 1492R (Rochelle et al., 1992), both directing the 16S rRNA gene in 50 μL PCR mixtures containing 200 nM of each primer, 100 μM of each dNTP, 1x Super Taq PCR buffer (HT Biotechnology, Cambridge, UK) and 2 U of Super Taq DNA polymerase (HT Biotechnology). PCR conditions were: one cycle at 94 °C for 4 min (initial denaturation); 35 cycles at 94 °C for 1 min (denaturation); 55 °C for 1 min (annealing); 72 °C for 2 min (primer extension) and one cycle at 72 °C for 5 min (final extension). PCR products were purified and checked for their expected length of about 1500 bp in agarose gels stained with ethidium bromide. Purified PCR products were then cloned into the pGEM-T vector (Promega, Leiden, NL) and introduced into Escherichia coli JM109 by transformation, according to the protocol provided by the manufacturer. Clones containing the expected 1500-bp inserts were subjected to sequencing with primer 1492R using the service of Greenomics, Plant Research International B.V., the Netherlands. The resulting sequences (c. 450 bp) were checked for chimeras using the Check Chimera tool and nonchimeric sequences were assessed for similarities with the database sequences available at the NCBI web site (http://www.ncbi.nlm.nih.gov) by blast searching.
PCR-DGGE analysis
PCR amplifications were performed on 100 ng of Phi 29-amplified tick DNA samples in standard 50-μL reaction mixtures according to Van Elsas & Wolters (1995), using primers 968F, with GC clamp (Muyzer et al., 1993), and 1401R, both directed towards the 16S rRNA gene region V6 (Heuer et al., 1997; Heuer & Smalla, 1999). PCR amplification was performed in a PTC-100 (MJ Research Inc., MA) thermocycler. PCR products (expected length 450 bp) were run in 0.8% agarose gels, and bands were visualized under UV after staining with ethidium bromide. For DGGE, polyacrylamide gels (6%) were prepared with denaturing gradients of 45–65% (100% denaturant consists of 7 M urea and 40% formamide). Gels were loaded with 15-μL volumes prepared by mixing 10 μL PCR product (c. 200 ng) with 5 μL loading buffer (0.25% bromophenol blue, 0.25% xylene cyanol FF and 30% glycerol). All gels were run in a PhorU2 apparatus (Ingeny, Goes, NL) at 60 °C, 100 V for 16 h. After running, gels were stained with SYBR Gold (Molecular Probes, Leiden, NL) and fingerprints from individual lanes were analysed using the molecular analyst software (version 1.61, BioRad, Veenendaal, NL). Normalization of the gels was achieved using a marker, consisting of 16S rRNA gene amplicons from Enterobacter cloaceae BE1, Listeria innocua ALM 105, Arthrobacter sp. AR1 and Burkholderia cepacia P2, which was loaded at three different positions in the gel. Migration distance and intensity of individual bands in the fingerprints were chosen as the parameters used for the calculation of Shannon diversity index (H′). The Shannon diversity index combines diversity and evenness of bacterial species and increases with the number of species (individual bands in fingerprints) and the more even distribution of biomass (total band size) over the different bacterial species in each sample.
PCR-DGGE fingerprints from all 180 ticks were inspected for the presence of specific bands comigrating with PCR-amplified products of clone inserts that gave nearest matches with Candidatus Neoehrlichia mikurensis and R. australis (see later) and with PCR products of 16S rRNA genes of B. burgdorferi ss, B. afzelii, B. garinii and B. valaisiana. Ticks containing one or more of these bands in their fingerprints were scored positive for the presence of these species.
Reverse line blot
For the detection of different Borrelia species in ticks, the PCR-RLB procedure described in Rijpkema et al. (1995) was used. This protocol was adapted according to Wielinga et al. (2006) and included six Borrelia species-specific probes (Alekseev et al., 2001; Wielinga et al., 2006). Two modifications were applied: (1) the use of primers B-5Sbor modified (biotin-5′-ATGGTACTGCGAGTTCGCGG-3′) and 23Sbor modified (5′-TTTCGCTCGCCACTACTAAGG-3′) to generate Borrelia genus-specific PCR products from Phi 29-amplified tick DNA samples, and (2) chemiluminescence detection and signal quantification from hybridized filters with a chemidoc XRS camera and accompanying software (Bio-Rad Laboratories, Veenendaal, the Netherlands). These modifications were applied to reduce nonspecific annealing of primers and to improve signal sensitivity from hybridized filters.
Statistical analyses
Statistical analyses were performed on (1) thickness, water content, organic matter content and pH of humus and leaf litter layers, with two replicates per area; (2) Borrelia species-, Candidatus Neoehrlichia mikurensis- and R. australis-infected ticks, with two replicates per area; and (3) bacterial diversity and evenness (Shannon index, H′) in individual ticks determined by PCR-DGGE, containing 30 replicates per location. Comparisons in thickness, water content, organic matter content, pH, infected tick numbers and diversity (H′) values between different locations and areas were made by anova (GenStat 9.1, Rothamsted Experimental Station, UK), and least significant difference (LSD) values were calculated.
Results
Forest floor and vegetation characteristics of the study areas
Amsterdam water supply dunes
The soil in Amsterdam water supply dunes was characterized as sand (dune sand). The thicknesses of humus and leaf litter layers were the same at both locations (Table 1). In the humus layer, the water content was higher in A1 than in A2, and also organic matter content, whereas pH was the same at both locations. In the leaf litter, the layer water content in A1 was lower than in A2, also was organic matter content, whereas pH was the same at both locations. The vegetation layer covering the forest floors at both locations in A consisted of Schreber's moss and common bracken (Table 2). Rowan only occurred in the shrub layer of A2 and was absent in that of A1, whereas black cherry was present at both locations. Sycamore was only present in the tree layer in A2 and absent in A1, whereas Pedunculate Oak was present at both locations. Vegetation association at both locations was Fago-Quercetum roboris pteridietosum (Beech–Oak association, subassociated with Common Bracken).
Forest floor characteristics of three different habitats in the Netherlands used for Ixodes ricinus tick collections
A, Amsterdam water supply dunes; E, Ede deSysselt; V, Veldhoven Hoogeind; 1, location 1; 2, location 2.
Statistically different; a<b<c; P<0.01.
Forest floor characteristics of three different habitats in the Netherlands used for Ixodes ricinus tick collections
A, Amsterdam water supply dunes; E, Ede deSysselt; V, Veldhoven Hoogeind; 1, location 1; 2, location 2.
Statistically different; a<b<c; P<0.01.
Vegetation in three different habitats in the Netherlands used for Ixodes ricinus tick collections
A, Amsterdam water supply dunes; E, Ede deSysselt; V, Veldhoven Hoogeind; 1, location 1; 2, location 2.
Vegetation in three different habitats in the Netherlands used for Ixodes ricinus tick collections
A, Amsterdam water supply dunes; E, Ede deSysselt; V, Veldhoven Hoogeind; 1, location 1; 2, location 2.
Ede, deSysselt
The soil in Ede, deSysselt was characterized as loamy sand. The thicknesses of the humus layers, and also of the leaf litter layers, were different at both locations. (Table 1). In the humus layers at both locations, water content was the same, organic matter content was higher in E1 than in E2, and pH was the same at both locations. In the leaf litter layer, water content in E1 was lower than in E2, organic matter content in E1 was lower than in E2, and pH was the same at both locations. The vegetation layer covering forest floors at both locations consisted of Schrebers's moss, blueberry, sheep fescue and lady fern (Table 2). Black Cherry, rowan, European larch (only in E2) and Norway spruce (only in E1) were the plants present in the shrub layers. Pedunculate oak, common beech, down birch and scots pine (only in E1) were present in the tree layers. Vegetation associations at both locations were Betulo-Quercetum roboris vaccinietosum (birch–oak association, subassociated with blueberry; E1) and Betulo-Quercetum roboris cladonietosum (birch–oak association, subassociated with mosses; E2) (Table 2).
Veldhoven, Hoogeind
The soil in Veldhoven, Hoogeind was characterized as sand (‘Enkheerd’ soil). The thicknesses of the humus layers were the same at both locations whereas those of the leaf litter layers differed (Table 1). In the humus layer water content in V1 was lower than in V2, organic matter content was lower in V1 than in V2 and pH was also lower in V1 than in V2. In the leaf litter layer water content in V1 was higher than in V2, organic matter content in V1 was higher than in V2 and pH was also higher in V1 than in V2. The vegetation layers covering the forest floors at both locations consisted of Schreber's moss, hair cap moss (only in V2), purple moore grass and sheep fescue (only in V2) (Table 2). Black cherry, blackberry (only in V1), honeysuckle (only in V1) and rowan (only in V1) were the shrubs, and pendunculate oak and scots pine (only in V2) were the trees present in V. Vegetation associations at both locations were Betulo-Quercetum roboris molinietosum (birch–oak association, subassociated with purple moore grass; V1) and Betulo-Quercetum roboris cladonietosum (birch–oak association, subassociated with mosses; V2) (Table 2).
The most striking differences between the three areas were thickness of the leaf litter layer in E that was less than half of the size present in A and V and the organic matter content in the humus layer in E that was about double the amount present in A and V, whereas pH was lower in E than in A and V (Table 1). Diversity in vegetation was higher in E (13 plant species) than in V (10 plant species) and A (six plant species), and vegetation association in A was different from those in E and V (Table 2).
Collection of I. ricinus ticks
In total, 391 I. ricinus ticks were collected from the three areas and represented three developmental stages: adult (40; 21 male and 19 female), nymph (292) and larvae (59). Highest tick numbers were collected from V (162), then from A (116) and finally from E (113). Large differences were found in different locations: V1 (106), E1 (71), A1 (60), A2 (56), V2 (56) and E2 (42). The number of nymphs collected from the different locations were V1 (82), E1 (48), V2 (49), A2 (45), A1 (38) and E2 (30). Nymphs represented the most abundant developmental stage found, and these were used for further analysis. From each location 30 nymphs were selected and subjected to PCR-DGGE and PCR-RLB, and from each area 3 nymphs were selected for cloning of 16S rRNA gene amplicons.
Molecular identification of bacteria present in ticks
Clone libraries made from PCR-amplified 16S rRNA genes of nine Phi 29-amplified tick DNA extracts (see later) were used for identification of the most dominant species. Sequences of 77 clone inserts were compared with sequences present in databases for nearest matches with strains and type strains (Table 3). Among inserts from all nine clone libraries, nearest matches with nine different strains and eight different type strains were found. In 29 inserts from six clone libraries (Ei, Eii, Eiii, Vi, Vii and Viii) nearest matches (100% similarity) with Candidatus Neoehrlichia mikurensis were found, and in 35 inserts from five libraries (Ei, Eiii, Vi, Vii, Viii), nearest matches (99% similarity) with Ehrlichia sp. Belluno were found. Inserts that gave nearest matches with Ehrlichia sp. Belluno and Candidatus Neoehrlichia mikurensis all gave nearest matches with Candidatus Neoehrlichia mikurensis type strain, indicating that both strains are closely related. The nearest match with Rickettsia species IP1 (99% similarity with type strain R. australis) was found in six inserts from clone libraries Ai and Aii. In clone library Aiii, two inserts gave nearest matches with Wolbachia species Avit (94% similarity with type strain Wolbachia pipiensis) and one with Wolbachia species (87% similarity with type strain Anaplasma phagocytophilum). In clone library Eiii, one insert gave a nearest match with Rickettsiales bacterium lt86 (88% similarity with type strain Ensifer meliloti), one with Candidatus Midichloria mitochondrii (89% similarity with type strain Rhizobium vitis), one with Pelomonas soli (100% similarity with type strain Pelomonas saccharophila) and one with Acinetobacter calcoaceticus (95% similarity with type strain A. calcoaceticus).
Taxonomic identity of cloned 16S rRNA gene amplicons from Ixodes ricinus tick DNA extracts
Area1 | Library | Number of clones | Nearest match | Accession no. | Identity (%) | Nearest match type strain | Accession | Identity (%) |
A | Ai | 3 | Rickettsia sp. IP1 | AF394904 | 98 | Rickettsia australis (T) | L36101 | 99 |
Aii | 3 | Rickettsia sp. IP1 | AF394904 | 99 | Rickettsia australis (T) | L36101 | 99 | |
Aiii | 2 | Wolbachia sp. Avit16SWol | AY007549 | 98 | Wolbachia pipientis (T) | X61768 | 94 | |
1 | Wolbachia sp. | U83098 | 98 | Anaplasma phagocytophilum (T) | AF172167 | 87 | ||
E | Ei | 2 | Candidatus Neoehrlichia mikurensis | AB213021 | 100 | Candidatus Neoehrlichia mikurensis (T) | AB084582 | 100 |
5 | Ehrlichia sp. Belluno | AY098730 | 99 | Candidatus Neoehrlichia mikurensis (T) | AB084582 | 99 | ||
Eii | 8 | Candidatus Neoehrlichia mikurensis | AB213021 | 100 | Candidatus Neoehrlichia mikurensis (T) | AB084582 | 100 | |
Eiii | 1 | Candidatus Neoehrlichia mikurensis | AB213021 | 100 | Candidatus Neoehrlichia mikurensis (T) | AB084582 | 100 | |
1 | Ehrlichia sp. Belluno | AY098730 | 100 | Candidatus Neoehrlichia mikurensis (T) | AB084582 | 99 | ||
1 | Candidatus Midichloria mitochondrii | AJ566640 | 99 | Rhizobium vitis (T) | X67225 | 89 | ||
1 | Pelomonas soli | EF660749 | 100 | Pelomonas saccharophila (T) | AB021407 | 100 | ||
1 | Rickettsiales bacterium It86 | AF525482 | 99 | Ensifer meliloti (T) | X67222 | 88 | ||
V | Vi | 4 | Candidatus Neoehrlichia mikurensis | AB213021 | 100 | Candidatus Neoehrlichia mikurensis (T) | AB084582 | 100 |
5 | Ehrlichia sp. Belluno | AY098730 | 99 | Candidatus Neoehrlichia mikurensis (T) | AB084582 | 99 | ||
1 | Acinetobacter calcoaceticus | AY211134 | 99 | Acinetobacter calcoaceticus (T) | ATCC23055T | 95 | ||
Vii | 7 | Candidatus Neoehrlichia mikurensis | AB213021 | 100 | Candidatus Neoehrlichia mikurensis (T) | AB084582 | 100 | |
11 | Ehrlichia sp. Belluno | AY098730 | 99 | Candidatus Neoehrlichia mikurensis (T) | AB084582 | 99 | ||
Viii | 7 | Candidatus Neoehrlichia mikurensis | AB213021 | 100 | Candidatus Neoehrlichia mikurensis (T) | AB084582 | 100 | |
13 | Ehrlichia sp. Belluno | AY098730 | 99 | Candidatus Neoehrlichia mikurensis (T) | AB084582 | 99 |
Area1 | Library | Number of clones | Nearest match | Accession no. | Identity (%) | Nearest match type strain | Accession | Identity (%) |
A | Ai | 3 | Rickettsia sp. IP1 | AF394904 | 98 | Rickettsia australis (T) | L36101 | 99 |
Aii | 3 | Rickettsia sp. IP1 | AF394904 | 99 | Rickettsia australis (T) | L36101 | 99 | |
Aiii | 2 | Wolbachia sp. Avit16SWol | AY007549 | 98 | Wolbachia pipientis (T) | X61768 | 94 | |
1 | Wolbachia sp. | U83098 | 98 | Anaplasma phagocytophilum (T) | AF172167 | 87 | ||
E | Ei | 2 | Candidatus Neoehrlichia mikurensis | AB213021 | 100 | Candidatus Neoehrlichia mikurensis (T) | AB084582 | 100 |
5 | Ehrlichia sp. Belluno | AY098730 | 99 | Candidatus Neoehrlichia mikurensis (T) | AB084582 | 99 | ||
Eii | 8 | Candidatus Neoehrlichia mikurensis | AB213021 | 100 | Candidatus Neoehrlichia mikurensis (T) | AB084582 | 100 | |
Eiii | 1 | Candidatus Neoehrlichia mikurensis | AB213021 | 100 | Candidatus Neoehrlichia mikurensis (T) | AB084582 | 100 | |
1 | Ehrlichia sp. Belluno | AY098730 | 100 | Candidatus Neoehrlichia mikurensis (T) | AB084582 | 99 | ||
1 | Candidatus Midichloria mitochondrii | AJ566640 | 99 | Rhizobium vitis (T) | X67225 | 89 | ||
1 | Pelomonas soli | EF660749 | 100 | Pelomonas saccharophila (T) | AB021407 | 100 | ||
1 | Rickettsiales bacterium It86 | AF525482 | 99 | Ensifer meliloti (T) | X67222 | 88 | ||
V | Vi | 4 | Candidatus Neoehrlichia mikurensis | AB213021 | 100 | Candidatus Neoehrlichia mikurensis (T) | AB084582 | 100 |
5 | Ehrlichia sp. Belluno | AY098730 | 99 | Candidatus Neoehrlichia mikurensis (T) | AB084582 | 99 | ||
1 | Acinetobacter calcoaceticus | AY211134 | 99 | Acinetobacter calcoaceticus (T) | ATCC23055T | 95 | ||
Vii | 7 | Candidatus Neoehrlichia mikurensis | AB213021 | 100 | Candidatus Neoehrlichia mikurensis (T) | AB084582 | 100 | |
11 | Ehrlichia sp. Belluno | AY098730 | 99 | Candidatus Neoehrlichia mikurensis (T) | AB084582 | 99 | ||
Viii | 7 | Candidatus Neoehrlichia mikurensis | AB213021 | 100 | Candidatus Neoehrlichia mikurensis (T) | AB084582 | 100 | |
13 | Ehrlichia sp. Belluno | AY098730 | 99 | Candidatus Neoehrlichia mikurensis (T) | AB084582 | 99 |
A, Amsterdam water supply dunes; E, Ede deSysselt; V, Veldhoven Hoogeind; i–iii, libraries made from individual ticks.
Nearest matches with sequences present in public databases (http://www.ncbi.nlm.nih.gov), accessed by blast searches.
Taxonomic identity of cloned 16S rRNA gene amplicons from Ixodes ricinus tick DNA extracts
Area1 | Library | Number of clones | Nearest match | Accession no. | Identity (%) | Nearest match type strain | Accession | Identity (%) |
A | Ai | 3 | Rickettsia sp. IP1 | AF394904 | 98 | Rickettsia australis (T) | L36101 | 99 |
Aii | 3 | Rickettsia sp. IP1 | AF394904 | 99 | Rickettsia australis (T) | L36101 | 99 | |
Aiii | 2 | Wolbachia sp. Avit16SWol | AY007549 | 98 | Wolbachia pipientis (T) | X61768 | 94 | |
1 | Wolbachia sp. | U83098 | 98 | Anaplasma phagocytophilum (T) | AF172167 | 87 | ||
E | Ei | 2 | Candidatus Neoehrlichia mikurensis | AB213021 | 100 | Candidatus Neoehrlichia mikurensis (T) | AB084582 | 100 |
5 | Ehrlichia sp. Belluno | AY098730 | 99 | Candidatus Neoehrlichia mikurensis (T) | AB084582 | 99 | ||
Eii | 8 | Candidatus Neoehrlichia mikurensis | AB213021 | 100 | Candidatus Neoehrlichia mikurensis (T) | AB084582 | 100 | |
Eiii | 1 | Candidatus Neoehrlichia mikurensis | AB213021 | 100 | Candidatus Neoehrlichia mikurensis (T) | AB084582 | 100 | |
1 | Ehrlichia sp. Belluno | AY098730 | 100 | Candidatus Neoehrlichia mikurensis (T) | AB084582 | 99 | ||
1 | Candidatus Midichloria mitochondrii | AJ566640 | 99 | Rhizobium vitis (T) | X67225 | 89 | ||
1 | Pelomonas soli | EF660749 | 100 | Pelomonas saccharophila (T) | AB021407 | 100 | ||
1 | Rickettsiales bacterium It86 | AF525482 | 99 | Ensifer meliloti (T) | X67222 | 88 | ||
V | Vi | 4 | Candidatus Neoehrlichia mikurensis | AB213021 | 100 | Candidatus Neoehrlichia mikurensis (T) | AB084582 | 100 |
5 | Ehrlichia sp. Belluno | AY098730 | 99 | Candidatus Neoehrlichia mikurensis (T) | AB084582 | 99 | ||
1 | Acinetobacter calcoaceticus | AY211134 | 99 | Acinetobacter calcoaceticus (T) | ATCC23055T | 95 | ||
Vii | 7 | Candidatus Neoehrlichia mikurensis | AB213021 | 100 | Candidatus Neoehrlichia mikurensis (T) | AB084582 | 100 | |
11 | Ehrlichia sp. Belluno | AY098730 | 99 | Candidatus Neoehrlichia mikurensis (T) | AB084582 | 99 | ||
Viii | 7 | Candidatus Neoehrlichia mikurensis | AB213021 | 100 | Candidatus Neoehrlichia mikurensis (T) | AB084582 | 100 | |
13 | Ehrlichia sp. Belluno | AY098730 | 99 | Candidatus Neoehrlichia mikurensis (T) | AB084582 | 99 |
Area1 | Library | Number of clones | Nearest match | Accession no. | Identity (%) | Nearest match type strain | Accession | Identity (%) |
A | Ai | 3 | Rickettsia sp. IP1 | AF394904 | 98 | Rickettsia australis (T) | L36101 | 99 |
Aii | 3 | Rickettsia sp. IP1 | AF394904 | 99 | Rickettsia australis (T) | L36101 | 99 | |
Aiii | 2 | Wolbachia sp. Avit16SWol | AY007549 | 98 | Wolbachia pipientis (T) | X61768 | 94 | |
1 | Wolbachia sp. | U83098 | 98 | Anaplasma phagocytophilum (T) | AF172167 | 87 | ||
E | Ei | 2 | Candidatus Neoehrlichia mikurensis | AB213021 | 100 | Candidatus Neoehrlichia mikurensis (T) | AB084582 | 100 |
5 | Ehrlichia sp. Belluno | AY098730 | 99 | Candidatus Neoehrlichia mikurensis (T) | AB084582 | 99 | ||
Eii | 8 | Candidatus Neoehrlichia mikurensis | AB213021 | 100 | Candidatus Neoehrlichia mikurensis (T) | AB084582 | 100 | |
Eiii | 1 | Candidatus Neoehrlichia mikurensis | AB213021 | 100 | Candidatus Neoehrlichia mikurensis (T) | AB084582 | 100 | |
1 | Ehrlichia sp. Belluno | AY098730 | 100 | Candidatus Neoehrlichia mikurensis (T) | AB084582 | 99 | ||
1 | Candidatus Midichloria mitochondrii | AJ566640 | 99 | Rhizobium vitis (T) | X67225 | 89 | ||
1 | Pelomonas soli | EF660749 | 100 | Pelomonas saccharophila (T) | AB021407 | 100 | ||
1 | Rickettsiales bacterium It86 | AF525482 | 99 | Ensifer meliloti (T) | X67222 | 88 | ||
V | Vi | 4 | Candidatus Neoehrlichia mikurensis | AB213021 | 100 | Candidatus Neoehrlichia mikurensis (T) | AB084582 | 100 |
5 | Ehrlichia sp. Belluno | AY098730 | 99 | Candidatus Neoehrlichia mikurensis (T) | AB084582 | 99 | ||
1 | Acinetobacter calcoaceticus | AY211134 | 99 | Acinetobacter calcoaceticus (T) | ATCC23055T | 95 | ||
Vii | 7 | Candidatus Neoehrlichia mikurensis | AB213021 | 100 | Candidatus Neoehrlichia mikurensis (T) | AB084582 | 100 | |
11 | Ehrlichia sp. Belluno | AY098730 | 99 | Candidatus Neoehrlichia mikurensis (T) | AB084582 | 99 | ||
Viii | 7 | Candidatus Neoehrlichia mikurensis | AB213021 | 100 | Candidatus Neoehrlichia mikurensis (T) | AB084582 | 100 | |
13 | Ehrlichia sp. Belluno | AY098730 | 99 | Candidatus Neoehrlichia mikurensis (T) | AB084582 | 99 |
A, Amsterdam water supply dunes; E, Ede deSysselt; V, Veldhoven Hoogeind; i–iii, libraries made from individual ticks.
Nearest matches with sequences present in public databases (http://www.ncbi.nlm.nih.gov), accessed by blast searches.
Bacterial diversity in ticks
DNA extracts from ticks were first amplified by Phi 29, before PCR-DGGE and PCR-RLB analyses and construction of 16S rRNA gene clone libraries. Amplification with Phi 29 appeared to be an essential step before PCR-DGGE analysis. PCR-DGGE tests on nonamplified tick DNA resulted in fingerprints with too few bands (data not shown). Inclusion of a Phi 29 amplification step before PCR-DGGE resulted in a higher number of bands, up to 13 bands (Fig. 1). Thus, inclusion of the Phi 29 amplification step improved the resolution of the PCR-DGGE. As a consequence, however, even slight contaminations might be coamplified. Therefore, the same extracts were repeatedly subjected to Phi 29 amplification and no differences in PCR-DGGE fingerprints were observed. In addition, a blast search of sequences from clone libraries did not detect contaminating DNA sequences from species common in laboratories such as E. coli.
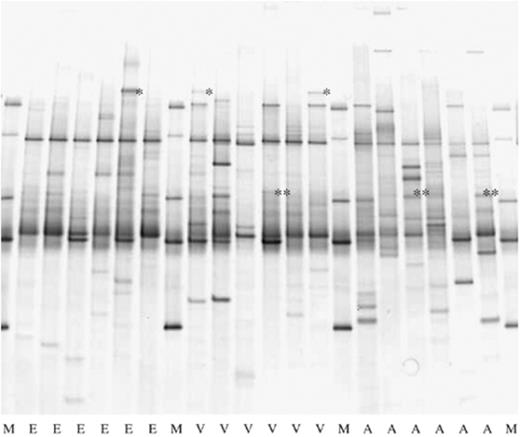
Bacterial PCR-DGGE on 18 individual ticks from three different habitats in the Netherlands. M, marker; E, Ede deSysselt; V, Veldhoven Hoogeind; A, Amsterdam water supply dunes. A specific band co-migrating with Candidatus Neoehrlichia mikurensis is marked with (*) and a band co-migrating with Rickettsia australis with (**).
PCR-DGGE fingerprints from 18 different ticks, six from each area, differed from each other, although three bands were common in the majority of the fingerprints (Fig. 1). PCR-DGGE fingerprints of all 180 ticks differed per location and in total 55 bands were present at different positions in the gels. Intensities of the bands were different and also for bands at the same position in different lanes, indicating that bacterial population densities differed per tick. The number of bands in each lane, representative of bacterial communities in one tick, varied between 1 and 13 bands. Band numbers differed between locations: between 2 and 10 for A1, E2, V1 and V2; between 1 and 13 for A2; and between 2 and 7 for E1. The Shannon diversity index (H′), calculated from the number of bands (species) and intensity (biomass) of all 180 ticks, was between 0 and 2.15 (Fig. 2). Significantly lower diversity per area was observed in E (0.743), followed by V (1.114) and finally by A (1.251). Bacterial diversity in ticks also differed per location and significantly lower diversity in ticks was found in E1 (0.556), followed by E2 (0.930), V1 (0.892), A1 (1.061) and finally by V2 (1.335) and A2 (1.441). This indicates that microbial diversity differed per area and even within.
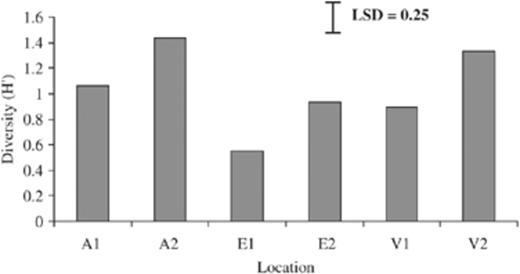
Diversity (H′) of bacterial communities present in ticks from three different habitats in the Netherlands. A, Amsterdam water supply dunes; E, Ede deSysselt; V, Veldhoven Hoogeind; 1, 2 different locations within each area. LSD=0.25; P<0.001.
PCR amplified products of inserts from four different clones, two showing 99% match with Ehrlichia sp. Belluno and two showing 100% match with Candidatus Neoehrlichia mikurensis, all gave a band with identical mobility in DGGE gels. In 21 tick PCR-DGGE fingerprints, a band comigrating with the Candidatus Neoehrlichia mikurensis-specific band was found, indicating that these ticks were positive for Candidatus Neoehrlichia mikurensis. The highest number of ticks positive for Candidatus Neoehrlichia mikurensis was found in V2, followed by, respectively, E1, E2, V1, A2 and A1 (Table 4). Between locations, no significant differences in the number of Candidatus Neoehrlichia mikurensis-infected ticks were found. PCR-amplified products of four clone inserts from two different ticks showing nearest matches with R. australis gave bands with the same mobility in DGGE gel. In a total of 28 tick fingerprints, a band was found that comigrated with this R. australis-specific band, indicating that these ticks were all positive for R. australis. The highest number of R. australis-positive ticks was found in A1, followed by A2, V2, E2 and finally the same in E1 and V1 (Table 4). Between locations, no significant differences were found between numbers of R. australis-infected ticks. In one fingerprint of a tick from E1, Candidatus Neoehrlichia mikurensis- and R. australis-specific bands were found, indicating that a double infection was present in this tick. PCR-amplified 16S rRNA genes of B. burgdorferi, B. afzelii, B. garinii and B. valaisiana gave bands with identical mobility in DGGE gel. In none of the 180 tick fingerprints a band comigrating with this Borrelia species-specific band was found, indicating that Borrelia species were absent or cell numbers were below the threshold level for detection by PCR-DGGE (see later). Candidatus Neoehrlichia mikurensis and R. australis were the most prevalent species found in ticks.
Presence of Candidatus Neoehrlichia mikurensis and Rickettsia australis in Ixodes ricinus ticks collected from three different habitats in the Netherlands
A, Amsterdam water supply dunes; E, Ede deSysselt; V, Veldhoven Hoogeind; 1,2 different locations within each area.
Total number of ticks analyzed: 180, 30 per location.
Presence of Candidatus Neoehrlichia mikurensis and Rickettsia australis in Ixodes ricinus ticks collected from three different habitats in the Netherlands
A, Amsterdam water supply dunes; E, Ede deSysselt; V, Veldhoven Hoogeind; 1,2 different locations within each area.
Total number of ticks analyzed: 180, 30 per location.
Borrelia species present in ticks
PCR-RLB analyses on all 180 Phi 29-amplified tick DNA extracts yielded 41 positive signals with three Borrelia genus-specific probes (Table 5). The number of Borrelia genus-positive ticks differed per area; the highest were found in E (18, 30%), then in A (13, 22%) and finally in V (10, 16%). Also, this number differed per location and was highest in E2, followed by A1, E1, A2, V1 and V2, and these differences were not significant (Table 5). In total, 36 of these positive ticks were also positive for one, and at four occasions for two probes specific for B. burgdorferi ss, B. garinii, B. afzelii and/or B. valaisiana. No positive reactions were observed with the probes specific for B. ruski (B. afzelii-like; Alekseev et al., 2001) and B. lusitaniae. Ticks positive for B. afzelii (34) were highest in number, followed by those positive for B. valaisiana (1) and B. garinii (1). Of the four tick DNA extracts that gave positive reactions with two probes, two were positive for B. afzelii and B. valaisiana, one for B. garinii and B. valaisiana and one for B. garinii and B. burgdorferi ss. At one occasion, positive signals were detected with genus-specific probes and not with the six species-specific probes, indicating that this tick harboured a different species of Borrelia.
Borrelia species-infected Ixodes ricinus ticks collected from three different habitats in the Netherlands
Location | Number positive for Borrelia genus | Number of Borrelia species present in ticks | ||||||
Ba | Bg | Bv | Ba+Bv | Bg+Bv | Bg+Bb | Unknown | ||
A1 | 7 | 5 | 1 | 1 | ||||
A2 | 6 | 3 | 1 | 2 | ||||
E1 | 6 | 5 | 1 | |||||
E2 | 12 | 12 | ||||||
V1 | 6 | 5 | 1 | |||||
V2 | 4 | 4 |
Location | Number positive for Borrelia genus | Number of Borrelia species present in ticks | ||||||
Ba | Bg | Bv | Ba+Bv | Bg+Bv | Bg+Bb | Unknown | ||
A1 | 7 | 5 | 1 | 1 | ||||
A2 | 6 | 3 | 1 | 2 | ||||
E1 | 6 | 5 | 1 | |||||
E2 | 12 | 12 | ||||||
V1 | 6 | 5 | 1 | |||||
V2 | 4 | 4 |
A, Amsterdam water supply dunes; E, Ede deSysselt; V, Veldhoven Hoogeind; 1 and 2, different locations within each area.
Number of ticks that were positive with Borrelia genus-specific primers; total number of ticks analyzed: 180, 30 per location.
Number of ticks that were positive in PCR-RLB for Borrelia species-specific probes; Ba, Borrelia afzelii; Bg, Borrelia garinii; Bv, Borrelia valaisiana; Bb, Borrelia burgdorferi sensu stricto; Unknown, no response with Borrelia species-specific probes including Borrelia lusitaniae and Borrelia ruski (Borrelia afzelii-like).
Borrelia species-infected Ixodes ricinus ticks collected from three different habitats in the Netherlands
Location | Number positive for Borrelia genus | Number of Borrelia species present in ticks | ||||||
Ba | Bg | Bv | Ba+Bv | Bg+Bv | Bg+Bb | Unknown | ||
A1 | 7 | 5 | 1 | 1 | ||||
A2 | 6 | 3 | 1 | 2 | ||||
E1 | 6 | 5 | 1 | |||||
E2 | 12 | 12 | ||||||
V1 | 6 | 5 | 1 | |||||
V2 | 4 | 4 |
Location | Number positive for Borrelia genus | Number of Borrelia species present in ticks | ||||||
Ba | Bg | Bv | Ba+Bv | Bg+Bv | Bg+Bb | Unknown | ||
A1 | 7 | 5 | 1 | 1 | ||||
A2 | 6 | 3 | 1 | 2 | ||||
E1 | 6 | 5 | 1 | |||||
E2 | 12 | 12 | ||||||
V1 | 6 | 5 | 1 | |||||
V2 | 4 | 4 |
A, Amsterdam water supply dunes; E, Ede deSysselt; V, Veldhoven Hoogeind; 1 and 2, different locations within each area.
Number of ticks that were positive with Borrelia genus-specific primers; total number of ticks analyzed: 180, 30 per location.
Number of ticks that were positive in PCR-RLB for Borrelia species-specific probes; Ba, Borrelia afzelii; Bg, Borrelia garinii; Bv, Borrelia valaisiana; Bb, Borrelia burgdorferi sensu stricto; Unknown, no response with Borrelia species-specific probes including Borrelia lusitaniae and Borrelia ruski (Borrelia afzelii-like).
Discussion
Ixodes ricinus ticks collected from three different natural habitats in the Netherlands differed in bacterial diversity. Ticks collected from location E1 showed lowest bacterial diversity, indicating that these ticks harboured a few dominating species. Highest numbers of Borrelia species-, Candidatus Neoehrlichia mikurensis- and R. australis-infected ticks also differed per habitat; Borrelia species-infected ticks were most abundant in E, Candidatus Neoehrlichia mikurensis-infected ticks in E and V and R. australis-infected ticks in A. That these species did not prevail all together in the same habitat would indicate that different factors are responsible for the transmission of these (presumed) pathogens to ticks. Most likely, reservoir hosts for ticks present in these habitats carry different bacterial communities, and this may explain why there is so much variation in PCR-DGGE fingerprints between habitats and even within. It is an intriguing question as to whether certain environmental factors in these habitats could prevent successful colonization and establishment of particular bacterial species in ticks. From this study there is no unequivocal evidence for the existence of these factors, although it is remarkable that the area lowest in the number of Candidatus Neoehrlichia mikurensis-infected ticks (A) was highest in R. australis-infected tick numbers.
In order to assess whether environmental factors drive bacterial diversity in ticks, differences in forest floor composition and vegetation between habitats were compared. Major differences were found between E and the two other study areas with respect to thickness of the leaf litter layer and organic matter content in the humus layer. The forest floor structure is important for tick survival. Female adults lay eggs therein and ticks will retreat in the leaf litter layer for transstadial development after each blood meal and when conditions are unfavourable for questing (Randolph & Storey, 1999). Vegetation associations also differed per study area and differences were largest between A and both the other areas. Differences in vegetation type determine the occurrence of tick populations because some are more favourable for tick survival and activity than others (Randolph & Storey, 1999). Vegetation is also important as a food and protection source of local tick hosts. Ticks mostly acquire bacteria from vertebrate animals because blood is their only food source (Kurtenbach et al., 2006; Gassner & Van Overbeek, 2007). Variation in animal host species composition is an important factor in determining tick-associated bacterial communities. Borrelia afzelii is a rodent-associated species (Gray, 1998; Kurtenbach et al., 2002, 2006) and was most prevalent in ticks in this study, which would indicate that rodents played an important role in transmission of Borrelia and other species to and from ticks. If and how different Borrelia species would interact with each other in ticks and reservoir hosts remains so far unresolved. From observations made with coinfected ticks, it seems likely that interactions occur. Namely, it was reported that incidences of coinfections were slightly higher than calculated from single occurrences (Rar et al., 2005; Wielinga et al., 2006). Apparently, there is an interaction between different Borrelia species present in the same tick. This, in combination with the fact that Borrelia species have different transmission rates in different reservoir hosts (Kurtenbach et al., 1998a), indicates that transmission of Borrelia species between ticks and reservoir hosts in nature is highly complex.
Geographic differences in tick bacterial composition have been observed before (Kirstein et al., 1997; Wielinga et al., 2006). In the study by Wielinga et al., ticks were collected from four different habitats in the Netherlands during two to four successive years. Because ticks were collected over a longer period in time, it must be assumed that differences found in Borrelia-, Anaplasma- and Ehrlichia-species infection rates included both spatial and temporal variations. In a study on Ixodes scapularis populations in different natural habitats in New York State, bacterial diversity in individual ticks at different developmental stages and nutritional status (unfed, partially or fully engorged) were investigated by temporal temperature-gradient gel electrophoresis (Moreno et al., 2006). It was found that both developmental stage and nutritional status influenced the bacterial composition of the ticks. In our study, we examined I. ricinus ticks that were in the same developmental stage and nutritional status and that had been collected within a short time frame, thus excluding factors other then geography and vegetation type. Surprisingly, differences in tick bacterial diversity were also found between separate locations within the three habitats. Distances between locations in different habitats never exceeded 300 m, which indicates that differences in tick bacterial composition occurred at a smaller scale. Wielinga et al. (2006) also reported on small-scale level variations in tick infection rates of different pathogens. Small-scale level variations in bacterial diversity in ticks is an important finding as it indicates that Borrelia species and possibly also other pathogens are heterogeneously distributed over habitats.
A majority of the clones from tick clone libraries gave nearest matches in the database with Candidatus Neoehrlichia mikurensis. This species was proposed to be a new member in the family of Anaplasmataceae (Kawahara et al., 2004) and has been found before in ticks from the Netherlands (Schouls et al., 1999), in rats and shrews in China (Pan et al., 2003), in rats and different mice species in Japan (Kawahara et al., 2004; Naitou et al., 2006), and in rodents and roe deer in Italy (Beninati et al., 2006). Members of this species are likely to occur in ticks and possibly rodents act as reservoir hosts. In two clone libraries, nearest matches were found with R. australis in the database. Recently, this bacterium was isolated from a patient from north-eastern Australia suffering from Queensland tick typhus (Unsworth et al., 2007), and to our knowledge this species has not been detected in European ticks before. Candidatus Neoehrlichia mikurensis and R. australis are species dominating in cell number in ticks. Analysis of the clone libraries reveals that the majority of ticks in our study carries pathogens other than Lyme borreliosis agents and highlights the ability of these ticks to transmit various pathogens to humans. Although likely, it is still not proven that all observed species represent actual pathogens. So far, detection of these pathogens is based on a relatively small part of the genome and additional tests for the presence of virulence genes on isolates or tick DNA extracts should provide more information on the true nature of these species.
Nearest matches with typical endosymbionts were also found in the clone libraries. In one clone library, hits were found with W. pipientis and in another one with Candidatus Midichloria mitochondrii. Wolbachia pipientis is a commonly found member of bacterial communities residing in arthropods (O'Neill et al., 1992; Lo et al., 2007). Candidatus Midichloria mitochondrii was recently proposed as a new member in the group of Rickettsiales (Sassera et al., 2006). This species has been found in I. ricinus ticks before (Beninati et al., 2004; Lo et al., 2006) and it must be regarded as a common member of tick-associated bacterial communities. It is the first described species that invades and persists in mitochondria present in eukaryotic cells (Sassera et al., 2006). It is interesting that no hits related to Borrelia species were found in the clone library. Possibly, Borrelia species are not dominating members of the bacterial communities present in unfed and questing ticks. However, cell numbers of Borrelia species may be higher in I. ricinus when ticks are fully engorged, which was the case in I. scapularis (Moreno et al., 2006). Hits with two other bacterial species, P. soli and A. calcoaceticus belonging to, respectively, the groups of Beta- and Gammaproteobacteria, were also found in the clone libraries. All other species found in the clone libraries from ticks belonged to the Alphaproteobacteria and this group must be regarded as the most dominant bacterial group associated with I. ricinus in this study. Dominance of the group of Alphaproteobacteria in Ixodes species is possibly not a general phenomenon as was shown in I. scapularis from Massachusetts, where a much higher diversity of associated bacterial species was found (Benson et al., 2004).
In conclusion, geographic differences were found in tick-associated bacterial communities. It is likely that Borrelia species and also other pathogens like Candidatus Neoehrlichia mikurensis and R. australis in ticks will differ per habitat. The observation of the endosymbiotic species W. pipiensis and Candidatus Midichloria mitochondrii in ticks makes the group of Alphaproteobacteria living in association with I. ricinus important. Differences in habitat structure, in particular the leaf litter composition and vegetation, may affect the presence and survival of ticks, and hence affect the risk of disease transmission. The observed differences in bacterial species composition and infection rates in the ticks are likely to be influenced by habitat structure as well as by the resident reservoir vertebrate hosts of Borrelia species.
Acknowledgements
This research was supported by a grant (IMPULS) from the Plant Sciences Group of Wageningen University and Research Centre. We are grateful to Dr Emil Hovius for his advice and background information on the tick populations of Veldhoven. We thank Frans Jacobs, Fernando Dini Andreote and Manuella Nobrega Dourado for helpful discussion and excellent technical support.
References
Author notes
Editor: Christoph Tebbe