-
PDF
- Split View
-
Views
-
Cite
Cite
Hilda Rodriguez, Alberto Mendoza, M. Antonia Cruz, Gina Holguin, Bernard R. Glick, Yoav Bashan, Pleiotropic physiological effects in the plant growth-promoting bacterium Azospirillum brasilense following chromosomal labeling in the clpX gene, FEMS Microbiology Ecology, Volume 57, Issue 2, August 2006, Pages 217–225, https://doi.org/10.1111/j.1574-6941.2006.00111.x
- Share Icon Share
Abstract
Azospirillum brasilense 8-I was chromosomally labeled with green fluorescent protein (gfp) genes, using either the native promoterless gfp gene or the mutant gfpmut2 gene under the transcriptional control of the neomycin phosphate transferase (npt2) promoter inserted into Tn5 suicide plasmid vectors. One A. brasilense exconjugant, showing a steady and strong fluorescence following irradiation with 365-nm UV light was characterized in detail. This strain, A. brasilense 8-I-gfp showed increased N2-fixation of approximately threefold, up to a twofold increase in exopolysaccharide production, and a significant decrease in indole-3-acetic acid and poly-β-hydroxybutyrate production over the parental strain. Sequence analysis showed that the Tn5 carrying the gfp gene was inserted in the clpX gene encoding a heat-shock protein. This data is consistent with a model in which the observed physiological changes are a consequence of pleiotropic changes that occur as a consequence of impaired heat shock (stress) protein synthesis. In summary, (i) chromosomally labelled Azospirillum brasilense was obtained carrying either native or mutant gfp genes, (ii) Pleiotropic physiological effects were caused by disruption of the clpX gene as the consequence of the insertion, (iii) a new indole-3-acetic acid-attenuated mutant of A. brasilense producing only 0.25% of the indole-3-acetic acid produced by the wild-type is presented.
Introduction
Azospirillum is one of the most studied plant growth-promoting bacteria (PGPB), known for enhancing yield of numerous crop plants. It significantly increases growth and affects the metabolism of higher plants and unicellular plants (Gonzalez & Bashan, 2000; Bashan et al.,2004) via an assortment of mechanisms; the most prominent are hormone production and N2-fixation (Bashan & Holguin, 1997; Steenhoudt & Vanderleyden, 2000).
As the association of the bacteria with roots is of crucial importance, various techniques have been developed for the specific visualization of the bacteria colonizing the roots (Levanony et al.,1989; Assmus et al.,1995; Bloemberg et al.,2000). Labeling microorganisms with the green fluorescent protein (gfp) gene is advantageous for environmental studies in plant-microbes interactions (Errampli et al.,1999) and is generally considered harmless to the cells. This tagging system has been successfully used for monitoring different soil species, such as Pseudomonas spp., Kluyvera ascorbata, and Agrobacterium tumefaciens, in the rhizosphere, either using plasmids (Bloemberg et al.,1997; Prayitno et al.,1999) or chromosomal insertions (Normander et al.,1999; Koch et al.,2001; Ma et al.,2001; Boldt et al.,2004). The chromosomal insertion of the labeling gene is a more recent development and an advantageous approach because it increases the stability of the inserted trait and greatly reduces the possibility of the horizontal transfer of labeling genes to other bacteria in the soil.
Previously, Azospirillum strains have been labeled with plasmid-born gfp genes (Ramos et al.,2002; Zhu et al.,2002; Rothballer et al.,2003). To date, successful chromosomal tagging of A. brasilense with gfp genes has not been reported. Previously, only weak fluorescent exconjugants were obtained (Xi et al.,1999).
The pUT vector systems (de Lorenzo et al.,1998) are suicide delivery plasmids suitable for chromosomal insertion of heterologous DNA fragments. The pUT minTn5kmgfp plasmid carries a promoterless wild type gfp gene (Eberl et al.,1997), while pUTminTn5ngfp harbors a mutant2gfp gene under the ntp2 promoter (Dandie et al.,2001). The pUT plasmids carrying gfp genes were used to report the chromosomal labeling of A. brasilense with gfp genes and the physiological consequences of that labeling.
Materials and methods
Bacterial strains, plasmids, and growth conditions
The bacterial strains and plasmids used in this study are listed in Table 1. Azospirillum strains were usually grown in nutrient broth (NB, Sigma, St. Louis, MO) (Bashan et al.,2002), but when needed, they were grown in rich liquid peptone-yeast (PY) medium containing 5 g L−1 peptone and 5 g L−1 yeast extract (Noel et al.,1984). Azospirillum spp. cultures were incubated on a rotary shaker (200 r.p.m.) at 37°C. Escherichia coli was grown in Luria Broth (LB) (Sambrook & Russell, 2001) at 37±1°C at 160 r.p.m. Growth on solid media in Petri dishes included adding 2% agar to the above media. For indole-3-acetic acid (IAA) analysis, bacteria were cultivated in conical flasks containing 50 mL synthetic malate medium (Zakharova et al.,1999) supplemented with 0.5 g L−1 NH4Cl and 100 mg mL−1 tryptophan. To induce poly-β-hydroxybutyrate (PHB) accumulation, bacteria were grown in a medium with a high carbon-to-nitrogen ratio (C : N). (Azotobacter medium described by Atkinson & Mavituna, 1983). For exopolysaccharide (EPS) production, the same high C : N medium was used, but the carbon source was substituted by glucose (5 g L−1). For assay of nitrogenase activity, the strains were grown in the Okon, Burris, Albrecht (OAB), N-free medium (as modified and described by Bashan et al.,1993). M9 salt solution, described by Sambrook & Russell (2001) was supplemented with malic acid (5 g L−1, Sigma) as the carbon source, and an appropriate antibiotic (listed below) was used as a selection medium for conjugation. Antibiotics were added at the following concentrations (μg mL−1): tetracycline (Tc), 10; kanamycin (Km), 50; ampicillin (Ap), 50, streptomycin (Sm), 100 (Sigma). Cells for cloning DNA of the A. brasilense Tn5 mutant were grown in tryptone, yeast, glucose (TYG) medium containing 0.5% tryptone, 0.5% yeast extract, 0.5% glucose and salts of OAB medium (Bashan et al.,2002) supplemented with kanamycin (50 μg mL−1).
Strain/plasmid | Description | Reference or source |
Azospirillum brasilense 8-I | Wild type strain isolated from sugarcane rhizosphere | Bacilio (2004) |
A. brasilense 8-I-gfp | A. brasilense 8I with a chromosomal insertion of MiniTn5-kmgfp | This study |
pUTminiTn5-Kmgfp | pUT-based Mini-Tn5 vector carrying the wild type, promoterless gfp gene, Apr,Kmr, | Eberl (1997) |
pUTminiTn5ngfp | pUT-based Mini-Tn5 vector carrying, mut2gfp gene under the control of the npt2 promoter Apr,Tcr | Dandie (2001) |
pRK2013 | Helper PLasmid, Kmr, tra+ | Figurski & Helinski (1979) |
Strain/plasmid | Description | Reference or source |
Azospirillum brasilense 8-I | Wild type strain isolated from sugarcane rhizosphere | Bacilio (2004) |
A. brasilense 8-I-gfp | A. brasilense 8I with a chromosomal insertion of MiniTn5-kmgfp | This study |
pUTminiTn5-Kmgfp | pUT-based Mini-Tn5 vector carrying the wild type, promoterless gfp gene, Apr,Kmr, | Eberl (1997) |
pUTminiTn5ngfp | pUT-based Mini-Tn5 vector carrying, mut2gfp gene under the control of the npt2 promoter Apr,Tcr | Dandie (2001) |
pRK2013 | Helper PLasmid, Kmr, tra+ | Figurski & Helinski (1979) |
Strain/plasmid | Description | Reference or source |
Azospirillum brasilense 8-I | Wild type strain isolated from sugarcane rhizosphere | Bacilio (2004) |
A. brasilense 8-I-gfp | A. brasilense 8I with a chromosomal insertion of MiniTn5-kmgfp | This study |
pUTminiTn5-Kmgfp | pUT-based Mini-Tn5 vector carrying the wild type, promoterless gfp gene, Apr,Kmr, | Eberl (1997) |
pUTminiTn5ngfp | pUT-based Mini-Tn5 vector carrying, mut2gfp gene under the control of the npt2 promoter Apr,Tcr | Dandie (2001) |
pRK2013 | Helper PLasmid, Kmr, tra+ | Figurski & Helinski (1979) |
Strain/plasmid | Description | Reference or source |
Azospirillum brasilense 8-I | Wild type strain isolated from sugarcane rhizosphere | Bacilio (2004) |
A. brasilense 8-I-gfp | A. brasilense 8I with a chromosomal insertion of MiniTn5-kmgfp | This study |
pUTminiTn5-Kmgfp | pUT-based Mini-Tn5 vector carrying the wild type, promoterless gfp gene, Apr,Kmr, | Eberl (1997) |
pUTminiTn5ngfp | pUT-based Mini-Tn5 vector carrying, mut2gfp gene under the control of the npt2 promoter Apr,Tcr | Dandie (2001) |
pRK2013 | Helper PLasmid, Kmr, tra+ | Figurski & Helinski (1979) |
Construction and characterization of the gfp-labeled Azospirillum strain
The plasmid pUTminiTn5 kmgfp/E. coli CC118 (Eberl et al.,1997) was introduced into the recipient cells by a triparental conjugation using the helper plasmid pRK2013 (Figurski & Helinski, 1979). A 10 : 1 : 1 proportion (v/v) of recipient: donor : helper strains, each from late logarithmic phase cultures (6 h growth), was used. Plasmid pUTminiTn5ngfp, harboring the mutant gfp gene (Dandie et al.,2001), was transferred from E. coli S17.1 λpir by biparental conjugation, employing a 4 : 1 proportion of recipient : donor strains. In both cases, the mixture was diluted with 5 mL saline solution (0.9% NaCl) and passed through a 0.22 μm millipore filter and incubated overnight on a nutrient agar (NA) plate, at 37±1°C. After this, cells were collected, washed twice with saline solution and re-suspended in the same solution, before plating on the selection medium. The plates were incubated at 37±1°C for over 48 h for detection of fluorescence.
The stability of the fluorescence trait was determined after growth in NB medium without kanamycin for 50 generations, and subsequent plating of the culture in NA medium with and without the antibiotic. After 48 h on the solid medium, all colonies were evaluated for fluorescence, as described below.
Detection of fluorescence
Colonies growing on the selected medium were screened for fluorescence after exposure with a hand-held UV lamp at 365 nm. For a more accurate screening for fluorescence, we used a black background in a dark room with the plate positioned with the cap upward, which reveals a much brighter fluorescence. An epifluorescence microscope (Nikon Optiphot-2, Tokyo, Japan) was used for observation of cells at excitation at 470–490 nm using a V1A filter, DM510 dichroid mirror, and a BA520 barrier filter.
Cloning of the transposon insertion
Genomic DNA from A. brasilense Tn5 mutant 8-I-gfp was isolated from a 5 mL culture grown overnight using the Wizard Genomic DNA Purification Kit (Promega, Madison, WI). Isolated DNA (20 μg) was digested for 240 min at 37±1°C in a volume of 100 μL with different restriction endonucleases (NE Biolabs, Beverly, MA). Digestion was monitored by electrophoresis on a 0.8% agarose, ethidium bromide-stained gel (2 g mL−1). Southern blot analysis, using the gfp gene as a probe, was used to determine suitable restriction fragments to recover fragments harboring the transposon-inactivated loci (enzymes cutting outside the insert). Digested DNA fragments (10 μg) were ligated into the pCR2.1 vector (Invitrogen, Carlsbad, CA) (25 ng mL−1) with T4 DNA ligase (NE Biolabs, Beverly, MA) in a final volume of 5 μL. 2 μL aliquots of ligated DNA were used to transform competent E. coli DH101. Kanamycin-resistant transformants were restreaked on LB supplemented with kanamycin, and plasmid DNA was purified from transformant colonies by alkaline lysis procedure (Sambrook & Russel, 2001).
DNA sequence analysis and physical mapping of the insert
DNA sequencing was performed with an ABI Prism 377 DNA sequencer (PerkinElmer, Inc., Foster City, CA) by Agencourt Bioscience Co., MA. For sequence analysis of the 16S rDNA, the following primers were used: 27f (5′GAGTTTGATCCTGGCTCAG) and 1495r (5′ CTACGGCTACCTTGTTACGA), which allow amplification of nearly the entire 16S rDNA gene (Grifoni et al.,1995). To determine the precise site of transposition of the cloned restriction fragment described above, the fragment was subcloned (using unique restriction sites in the transposons) as two smaller fragments, each of which included one end of the transposon along with the adjacent A. brasilense chromosomal DNA. Each of these subclones was then sequenced with the oligonucleotide Tn5-OUT (5′ CGGGAAAGGTTCCGTTCAGG 3′), which directs DNA synthesis from both ends of Tn5 outward, i.e. hybridizes to either end of the Tn5 derivatives. The analysis and assembly of the final sequence shown in Fig. 2 were performed with AutoAssembler software (Perkin Elmer, Inc.). Homology searches were performed with the software Gapped BLAST (Altschul et al.,1997), available online at (http://www.ncbi.nlm.nih.gov/Education/BLASTinfo/information3.html).
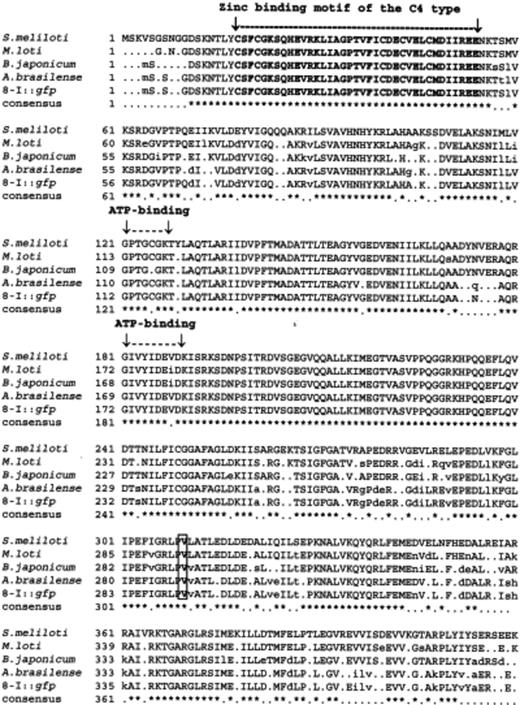
Multiple alignments of the deduced amino acid sequence of the heat-shock protein CplX-like gene of Azospirillum brasilense 8-I-gfp (Acc. no. AY918963) with the corresponding sequences of cplX genes from Sinorhizobium meliloti (Acc. no. NP385362), Mesorhizobium loti (Acc. no. NP108565), Bradyrhizobium japonicum (Acc. no. NP771583), and Azospirillum brasilense Sp7 (Acc. no. AAD37436). The Zn-binding motif, and the ATP-binding domains are indicated by lines and inverted arrows. Residue 292 (V), in a box, indicates the insertion point of the transposon.
N2-fixation and analytical analyses
N2-fixation was performed as an acetylene reduction assay using gas chromatography as described by Holguin (1992). Briefly, acetylene was injected into cultures grown for 48 h in the semi-solid N-free, OAB (Bashan et al.,1993) medium, and ethylene production was quantified after 24 to 48 h incubation at 30±1°C. Standard ethylene (Sigma-Aldrich) was used to quantify conversion of acetylene to ethylene. Indole-3-acetic acid (IAA) was analyzed by HPLC (Zakharova et al.,1999). Briefly, the culture fluid was centrifuged at 13 000 g for 5 min, the supernatant was filtered through a 0.22 μm membrane filter (Millipore Corp. Bedford, MA), and injected into an HPLC HP-1100 chromatograph (Hewlett Packard, Waldbroom, Germany). A 125 × 4.0 mm Ultrasphere® ODS RP-18 reversed-phase column (Beckman-Coulter, Inc., Fullerton, CA., 5 mm particle size) was used to analyze indole derivatives. Separation was performed at 220 nm, using acetonitrile/phosphate (30 : 70, v/v) as the solvent system at pH 3.0. Flow rate of the elluent was 1.0 mL min−1. Indole substances were quantified by integrating areas under peaks and comparing with standard indole compounds (Sigma, St. Louis, MO). Poly-β-hydroxybutyrate was measured according to the procedure described by Law & Slepecky (1961) and exopolysaccharide by reduced sugar analysis (Dische, 1962).
Statistical analysis
Experiments were performed in triplicate where one culture medium and one analytical analysis or injection into the GC or HPLC served as a replicate. Each experiment was repeated three times. Data from all experiments of the same treatment were polled and analyzed by anova and were followed by Tukey's post hoc analysis at P≤0.05 and Student's t-test at P≤0.05. All analyses used Statistica™ software (StatSoft Inc., Tulsa).
Results
Detection of chromosomally-labeled gfp clones
M9 minimum medium, supplemented with malic acid as the carbon source (used by A. brasilense, but not by E. coli) and kanamycin was used for selection of exconjugants derived from mating with pUTMiniTn5kmgfp. Kanamycin-resistant exconjugants were obtained at rates of 1 × 10−4 exconjugants per recipient cell. Approximately 3000 colonies were obtained and examined from the mating experiment. No fluorescent colonies were detected after 7 days of growth at 37±1°C in the selection medium. However, after an additional 7 days of incubation at 4±1°C, a single bright green fluorescent colony was detected by epifluorescence microscopy.
Tetracycline-resistant exconjugants were obtained after the insertion of plasmid pUTminiTn5ngfp carrying the mut2gfp gene, at a frequency of 1.8 × 10−4 exconjugants per recipient cell. From these, c. 40% of the colonies showed different fluorescence intensities under 365 nm UV rays.
Characterization of gfp-labeled Azospirillum brasilense
The selected labeled strain (A. brasilense 8-I-gfp carrying the wild type, promoterless gfp gene) was characterized and compared with the wild type by determining the growth rates in a rich medium. In fact, the growth rates in NB of the labeled strain and the wild type were similar (specific growth rates of 0.67 vs. 0.66 h−1, respectively). The labeled strain was further tested for stability of the fluorescent trait. After 50 generations under nonselective conditions (absence of antibiotic), 100% of about 4000 screened colonies kept the fluorescence character when examined under a 365 nm UV lamp. Additionally, A. brasilense strain verification was carried out by the restriction and sequence analysis of the amplified 16S rDNA (Grifoni et al.,1995).
To evaluate whether the tagging genes interfered with the ability of Azospirillum to fix atmospheric nitrogen, A. brasilense 8-I-gfp was evaluated for its growth on N-free medium and its capacity for acetylene reduction (nitrogenase) activity, in comparison with its parental strain A. brasilense 8-I. Interestingly, besides conserving its ability to grow on N-free medium, the labeled strain exhibited enhanced nitrogenase activity (Fig. 1a) (303.7% increase).
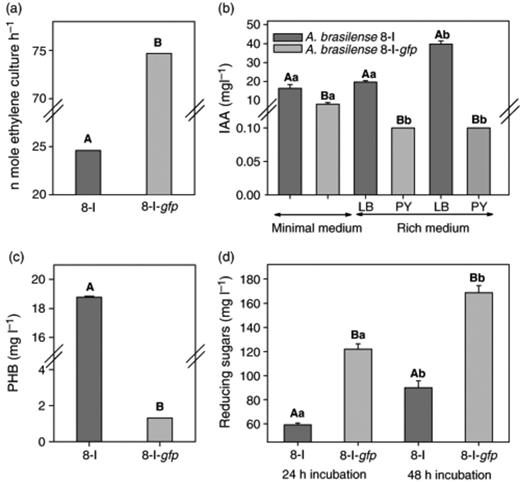
Changes in N2-fixation (a); indole-3-acetic acid (b); poly-β-hydroxybutyrate (c); and exopolysaccharide (d) production of A. brasilense 8-I and its mutant A. brasilense 8-I-gfp. In each subfigure separately, columns denoted by a different lower case letter differ significantly by anova at P≤0.05. Pairs of columns (parent and the mutant strains) denoted by a different capital letter differ significantly: Student's t-test at P≤0.05 (C, D). Bars represent the SE; the absence of SE indicates a negligible SE.
Three other major traits of Azospirillum were evaluated in A. brasilense 8-I-gfp. In LB and PY-rich media, there was a dramatic decline in the level of indole-3-acetic acid produced by the labeled strain (only 0.25% of the native strain) and it was still lower in minimal medium (Fig. 1b). Similarly, the level of poly-β-hydroxybutyrate production by the labeled strain significantly decreased (only 8% compared with the native strain) (Fig. 1c). However, the quantity of exopolysaccharides was significantly greater in the labeled strain (Fig. 1d).
DNA sequence analysis has been utilized to localize the exact point of insertion of the transposon into the A. brasilense genome (Fig. 2). The assembled DNA sequence corresponded to a heat shock protein encoded by a clpX-like gene. The nucleotide sequence shown in Fig. 2 has been deposited in the GenBank nucleotide sequence database (accession no. AY918963).
The sequence of the disrupted gene of A. brasilense 8-I-gfp, cloned in pCR2.1, belongs to an ATP-binding subunit of the heat shock protein clpX-like gene. The transposon insertion point is marked at residue 292 (V) (Fig. 2). The DNA sequence revealed an open reading frame (ORF) of 1269 nucleotides that encodes a predicted polypeptide of 423 residues with a predicted molecular mass of 46368 Da. The major motifs are highlighted: the zinc-binding motif from residues 12 (C) to 50 (K) and the two ATP-binding domains (Gottesman et al.,1993); GPTGCGKT from residues 112–119, and GIVYIDEVDK from residues 172 to 181, respectively (Fig. 2). These locations are similar to those found in four rhizobia and Azospirillum strains (Fig. 2). The multiple alignment of the deduced amino acid sequence revealed a high degree of homology with other ClpX proteins.
Discussion
Insertion of various reporter genes (gus, gfp, rfp, yfp, lux, and lacZ) alone or in combination to detect and quantify colonization of roots by plant growth-promoting bacteria is a common procedure (Arsène et al.,1994; Bloemberg et al.,2000; Unge & Jansson, 2001; Bacilio et al.,2004), currently being tested under field conditions (Basaglia et al., 2003). The fundamental assumption of these insertions is that they serve only as a detection tool and that the basic metabolic characteristics of the strains are not changed by the insertion.
Xi (1999) attempted to tag A. brasilense strains with gfp genes inserted into the chromosome. Although they successfully tagged other rhizobacterial species, they obtained only weak fluorescence in A. brasilense with a mutant gene under the constitutive npt2 promoter and no exconjugants when transferring the promoterless gfp gene. They attributed this to differences in codon usage among different species. Here, the labeling of A. brasilense using a promoterless wild-type gfp gene is reported. In the conjugation experiments, a technical step of washing the cells collected from the conjugation plates twice, aiming to avoid the background growth that sometimes appears in the presence of traces of nutrients from the culture medium, was added. As a result, besides eliminating the background traces, a higher frequency of recombinants in comparison with unwashed cells was observed. This is probably a consequence of eliminating compound(s) from the conjugation mixture that negatively affect the survival of the recombinants, thereby allowing a higher frequency than that reported for other promoterless gfp genes inserted into Gram-negative bacteria (Suarez et al.,1997).
The successful detection of gfp clones after incubation at 4°C suggests that incubation at low temperatures could have favored the expression of the fluorescence trait. Similarly, the fluorescence of whole cells and sonicated fractions of a gfp-tagged P. fluorescens increased with incubation at 4°C, while a decrease was observed at 23 and 30°C (Cassidy et al.,2000). The proportion of gfp-expressing colonies, in comparison with the total exconjugants obtained with the promoterless gfp gene (0.03%), was lower than that reported by Suarez (1997), but similar to the results of Eberl (1997). In the present study, different fluorescence intensities were observed among the obtained exconjugants. This phenomenon is similar to what has been determine for gfp-tagged P. aeruginosa (Dandie et al.,2001).
The A. brasilense 8-I-gfp was further characterized to determine whether the inserted transposon had an effect on any phenotypic changes in the fundamental traits of this species. The growth rate of the selected recombinant strain was not affected. Surprisingly, an increase in nitrogenase activity and modifications in three other metabolic activities of the labeled strain were detected. Increases in the N2-fixation rate, as the result of transposon insertions, have previously been reported in Bradyrhizobium japonicum (Maier & Graham, 1990) and Rhizobium tropici (Marroqui et al.,2001). As well, the improvement of N2-fixation capacity by attenuation of apparently unrelated traits has also been reported. Thus, azide-resistant mutants of Azorhizobium caulinodns exhibited improved N2-fixation (Sini et al.,2001), while in A. lipoferum, binding of the lectin wheat-germ-agglutinin to a receptor protein produced a pleiotropic effect that included increased transcription of the nifH and nifA genes (Karpati et al.,1999).
Cloning and sequencing of the gene interrupted by the insertion indicated that a heat shock protein gene (clpX) in A. brasilense 8-I was knocked out, in this case giving rise to increased nitrogen fixation and exopolysaccharide production and decreased indole-3-acetic acid and poly-β-hydroxybutyrate synthesis. We propose the following model to explain the observed pleiotropic effects of an insertion in the clpX gene in our strain. The process of nitrogen fixation that occurs under N-limiting conditions may be viewed as stressful to the bacterium, in that it is likely to deplete much of the available energy stores, thereby inducing a stress response. This stress response would include the synthesis of heat shock proteins which, by stabilizing existing cellular proteins, limits synthesis of new proteins, including nitrogenase. When the heat shock protein gene is disrupted, to compensate for the increased rate of protein turnover, cells must synthesize additional copies of essential proteins, including nitrogenase, to maintain cell viability. As a consequence, the energy in the cell is depleted more rapidly. Cells that are energy deficient enter a quiescent phase in which excess carbon is directed toward synthesizing extracellular polysaccharides (Glick, 1995). In the labeled strain, as a result of an energy deficit, the cell synthesizes greater than normal amounts of extracellular polysaccharides. Consequently, carbon that is not metabolized and would have been stored as PHB (in the native strain) is now converted to exopolysaccharides. Concomitantly, decreasing available energy leads to decreasing synthesis of indole-3-acetic acid.
Pleiotropic effects of Tn5 mutagenesis, including increased nitrogen-fixing capacity of A. brasilense, were reported by Revers (2000). In that case, the disrupted open reading frame (ORF280) is similar to the universal stress protein family (Galindo & Silveira, 2003). The fact that the disruption of another gene involved in a stress response increases the nitrogenase synthesis and produces other pleiotropic effects is consistent with our findings. However, no significant similarity was found for the alignment of the interrupted gene, the ATP-binding subunit heat-shock protein ClpX or ORF280, indicating that a new kind of gene was targeted in our A. brasilense strain.
A study of the mode of action in Azospirillum spp. producing IAA requires a source of IAA-deficient mutants of the parental strains (Patten & Glick, 1996, 2002; Gonzalez & Bashan, 2000; Bashan et al.,2004). Such mutants have not been developed, and only two IAA-attenuated mutants of Azospirillum spp. (2–5% of the IAA produced by the wild type) are known (Costacurta et al.,1992; Vande Broek et al.,1999). Similarly, Patten & Glick (2002) reported constructing a Pseudomonas putida strain where the ipdc gene is disrupted and IAA levels are 5% of the wild type. In that case, it was definitively shown that IAA played an essential role in plant root development. Our mutant, A. brasilense 8-I-gfp, adds a new strain to this limited inventory, producing only 0.25% of the IAA synthesized by the wild-type and could be considered an IAA-deficient mutant for study purposes.
In summary, the insertion of seemingly unrelated gfp marker genes in Azospirillum brasilense induced major changes in the physiology of the cell. These changes are of utmost importance when considering modifying this species for traits, such as reporter genes, that are not related to the basic function of the bacterium. Additionally, pleiotropic effects from inactivating a heat shock protein suggests an important role of this gene in the major metabolic activities in bacteria, as well as offering a new IAA-attenuated A. brasilense strain for research purposes.
Acknowledgements
We thank Leo Eberl at the Technical University of Munich, Germany and Catherine Dandie at The Flinders University of South Australia for providing plasmid pUTminiTn5Kmgfp, and plasmid pUTminTn5ngfp, respectively. At CBG, Patricia Larralde provided technical support and PHB and EPS analysis. At CIBNOR, Ariel Cruz performed gas chromatography, Juan-Pablo Hernandez did statistical analysis and graph production, and the editor improved the English text. H.R. was a recipient of a fellowship from Consejo Nacional de Ciencia y Tecnología, Mexico (CONACYT grant EX-000580).The study was supported by CONACYT contract #U39520-Z, and partially by The Bashan Foundation, USA.
References