-
PDF
- Split View
-
Views
-
Cite
Cite
Anna F Feller, Grace Burgin, Nia F Lewis, Rohan Prabhu, Robin Hopkins, Mismatch between pollen and pistil size causes asymmetric mechanical reproductive isolation across Phlox species, Evolution, Volume 78, Issue 12, 1 December 2024, Pages 1936–1948, https://doi.org/10.1093/evolut/qpae128
- Share Icon Share
Abstract
Characterizing the mechanisms of reproductive isolation between lineages is key to determining how new species are formed and maintained. In flowering plants, interactions between the reproductive organs of the flower—the pollen and the pistil—serve as the last barrier to reproduction before fertilization. As such, these pollen–pistil interactions are both complex and important for determining a suitable mate. Here, we test whether differences in style length (a part of the pistil) generate a postmating prezygotic mechanical barrier between five species of perennial Phlox wildflowers with geographically overlapping distributions. We perform controlled pairwise reciprocal crosses between three species with long styles and two species with short styles to assess crossing success (seed set). We find that the heterospecific seed set is broadly reduced compared to conspecific cross success and reveal a striking asymmetry in heterospecific crosses between species with different style lengths. To determine the mechanism underlying this asymmetric reproductive isolating barrier, we assess pollen tube growth in vivo and in vitro. We demonstrate that pollen tubes of short-styled species do not grow long enough to reach the ovaries of long-styled species. We find that short-styled species also have smaller pollen and that both within- and between-species pollen diameter is highly correlated with pollen tube length. Our results support the hypothesis that the small pollen of short-styled species lacks resources to grow pollen tubes long enough to access the ovaries of the long-styled species, resulting in an asymmetrical, mechanical barrier to reproduction. Such reproductive isolating mechanisms, combined with additional pollen–pistil incompatibilities, may be particularly important for closely related species in geographic proximity that share pollinators.
Introduction
In both plants and animals, the process by which new species are formed (speciation) can be characterized as the evolution of barriers to reproduction that reduce and ultimately prevent gene flow between diverging lineages (Coyne & Orr, 2004; Mayr 1942). Identifying the causes of reproductive isolation (RI) is thus key to understanding speciation. Such isolating barriers can evolve and act at various stages in the reproductive process: They can prevent mating/pollination from occurring at all (premating/prepollination isolation), prevent a zygote from forming (postmating/postpollination prezygotic isolation), or cause reduced fitness of hybrid offspring (postzygotic isolation) (Coyne & Orr, 2004; Dobzhansky 1937).
In flowering plants (angiosperms), variation in floral traits—size, shape, and color—can alter and optimize the reproductive process in several ways and is therefore thought to be integral to angiosperm diversification (Faegri & Van Der Pijl, 1979; Soltis & Soltis, 2014; Stebbins, 1970). The role of floral trait variation in the speciation process is particularly well-appreciated for its impact on pollination; variation in floral morphology is linked to variation in pollination vector or mode, which can decrease pollen flow between diverging lineages (Fenster et al., 2004; Grant, 1949, 1994; Hernández-Hernández & Wiens, 2020; Kay et al., 2006; Landis et al., 2018; Stewart et al., 2022; Van der Niet et al., 2014; Waser & Ollerton, 2006). Less widely explored is how variation in reproductive organs within the flower might generate mechanical barriers to gene flow during speciation. As overall flower size and shape diversify, so does the size, shape, and placement of the male and female reproductive organs, which can lead to mechanical mismatch during reproduction between species.
A mechanical mismatch in male and female reproductive organs is a phenomenon that has been documented across a variety of organisms but is generally understudied due to the often cryptic nature of these interactions (Garlovsky et al., 2023). Mechanical mismatches in reproductive organs in flowering plants can occur both during pollination as a mismatch in the position of the stigma (the pollen-receiving part of the female reproductive organ) and pollen deposition (Grant, 1994; Kay & Surget-Groba, 2022; Minnaar et al., 2019), as well as post-pollination through mismatches between the pollen (containing the sperm) and the pistil (the female reproductive organ consisting of stigma, style, and ovaries) (Brothers & Delph, 2017; Garlovsky et al., 2023; Nista et al., 2015).
After arriving at the flower, pollen must germinate on the receptive stigma, grow a pollen tube through the style, locate ovules housed at the base of the pistil, and deliver sperm for fertilization. The length of the style varies to a great extent across flowering plants, sometimes significantly even between closely related species (e.g., Aguilar et al., 2002; Buchholz et al., 1935; Levin & Kerster, 1967; Nista et al., 2015; Torres, 2000; Williams & Rouse, 1988). By determining how far a pollen tube must grow to reach the ovules, differences in style length may serve as an important post-pollination mechanical isolating barrier (Tiffin et al., 2001). It has been observed that plants with long styles do not set successful seeds when pollinated by plants with short styles while short-styled plants can successfully reproduce with pollen from long-styled plants. This asymmetry has been documented in a variety of closely related plant species (e.g., Lee et al., 2008; Nista et al., 2015; Perez & Moore, 1985; Tiffin et al., 2001; Williams & Rouse, 1988; Yost & Kay, 2009), demonstrating that variation in style length can be associated with a strong post-pollination mechanical isolating barrier across flowering plant diversity. However, we know little about how this barrier functions. Why are pollen grains from short-styled species unsuccessful at fertilizing the ovules of long-styled species?
One hypothesis is that pollen from short-styled species may lack the resources to grow pollen tubes long enough to reach the ovules of long-styled species (Brothers & Delph, 2017; Delpino, 1867; Nista et al., 2015). Support for this hypothesis is largely correlative; longer-styled species tend to have larger pollen grains than shorter-styled species (e.g., Aguilar et al., 2002; Plitmann & Levin, 1983; Roulston et al., 2000; Torres, 2000), and larger pollen grains also tend to contain higher energy stores (Baker & Baker, 1979; Cruden & Miller-Ward, 1981; Manicacci & Barrett, 1995). If smaller pollen is less-resourced, it could be unable to grow tubes long enough to reach ovules at the base of long styles. While there is broad acceptance for this hypothesis, it has rarely been demonstrated (but see Brothers & Delph, 2017; Nista et al., 2015). An alternative hypothesis is that the pistil environment of short- versus long-styled species differs, generating a mismatch in biochemical signaling between pollen from short-styled species and long-styled pistils (Amici, 1830; Broz & Bedinger, 2021; Cruden, 2009; Cruden & Lyon, 1985; Kato et al., 2022; Swanson et al., 2004). Following pollination, sexual reproduction in flowering plants requires complex and coordinated communication between pollen and pistil (reviewed in Bedinger et al., 2017; Cheung et al., 2022; Johnson et al., 2019; Robichaux & Wallace, 2021; Zheng et al., 2018). Throughout this postpollination process, pollen receives molecular signals from receptive pistils to facilitate germination, initiate pollen tube growth, and guide pollen to the ovule (Cheung et al., 2022; Johnson et al., 2019; Robichaux & Wallace, 2021; Zakharova et al., 2022; Zheng et al., 2018). Species-specific biochemical signaling, which can include active heterospecific rejection, by the pistil tissue at any one of these postpollination steps can cause reproductive isolation (Broz & Bedinger, 2021). Distinguishing between these two scenarios thus requires disentangling the effect of pollen size/resources from variation in the pistil environment across species.
Here, we investigate the mechanisms driving mechanical isolation between long- and short-styled species of Phlox wildflowers. Phlox is a genus of flowering plants with more than 60 species that are predominantly found in Northern America (Ferguson & Jansen, 2002; Wherry, 1955). The species used in this study, P. glaberrima ssp. interior (GLA), P. maculata (MAC), P. paniculata (PAN), P. pilosa ssp. pilosa North (PIL), and P. divaricata (DIV; including the two subspecies P. divaricata ssp. divaricata and P. divaricata ssp. laphamii) are all perennial outcrossing species that evolved in the prairies of the Midwestern United States and still occur with widely overlapping geographic distributions in the states of Illinois and Indiana (Wherry, 1955; Figure 1; Supplementary Table S1). These species have unique flowering time peaks but largely overlapping flowering periods, occupy distinct but not mutually exclusive habitats, and share similar pollinator assemblages, which predominantly include butterflies and long-tongued bees (Robertson, 1891, 1895, 1928; Wherry, 1932, 1933, 1955; Grant & Grant, 1965; Hadley & Levin, 1969). Pollen exchange has been documented in detail between GLA and PIL (Levin & Kerster, 1967; Levin & Smith, 1965) and likely occurs between the other species as well. Hence, reproductive isolating postpollination barriers are likely important in maintaining species boundaries within this group. Three of the species (GLA, MAC, and PAN) have long styles ranging from 10.5 to 28.9 mm that protrude significantly from the calyx and two (DIV and PIL) have short styles ranging from 2.7 to 6.3 mm that are hidden deep within the calyx. In previous experiments involving these Phlox species, crosses between species with different style lengths appeared to result in asymmetric success such that crosses only yielded seeds when the pollen parent was long-styled (Levin, 1963, 1966; Levin & Smith, 1965; Zale, 2014). However, the exact cause of this asymmetry has yet to be identified. Phlox is a particularly powerful system to investigate the mechanism underlying style-length reproductive isolation because flower morphology across this genus (and particularly in the five species investigated here) is broadly conserved (Figures 1 and 2). Unlike in other systems (e.g., Brothers & Delph, 2017; Lanuza et al., 2023), style length across the focal Phlox species is not associated with an overall difference in flower size or morphology. As a result, our study isolates the specific effect of style length variation as a reproductive isolating barrier from other floral trait changes.
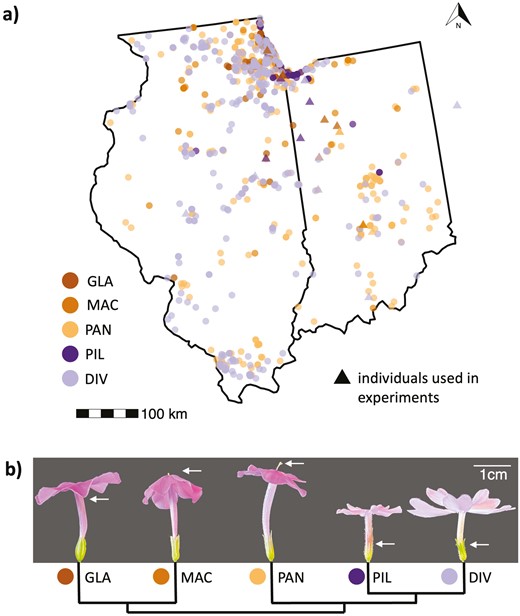
Distributions and representative images of the five focal Phlox species with geographically overlapping ranges in the states of Indiana and Illinois (USA). (a) Map of Illinois and Indiana with occurrence data obtained from the Global Biodiversity Information Facility (GBIF.org, 2023; see Supplementary Table S1 for the full reference list and download links). Triangles indicate the locations of individuals used in this study (including one P. divaricata in Ohio; see Supplementary Table S2). The map was plotted with the R packages usmap v.0.6.1 (Di Lorenzo, 2023), ggplot2 v.3.4.2 (Wickham, 2016), and ggspatial v.1.1.9 (Dunnington, 2023). (b) Lateral representative images and phylogeny (derived from Garner et al., 2024) of the five species. P. glaberrima ssp. interior (GLA), P. maculata (MAC), and P. paniculata (PAN) are long-styled. P. pilosa ssp. pilosa (PIL) and P. divaricata (DIV) are short-styled. The white arrows indicate the approximate height of styles for each species.
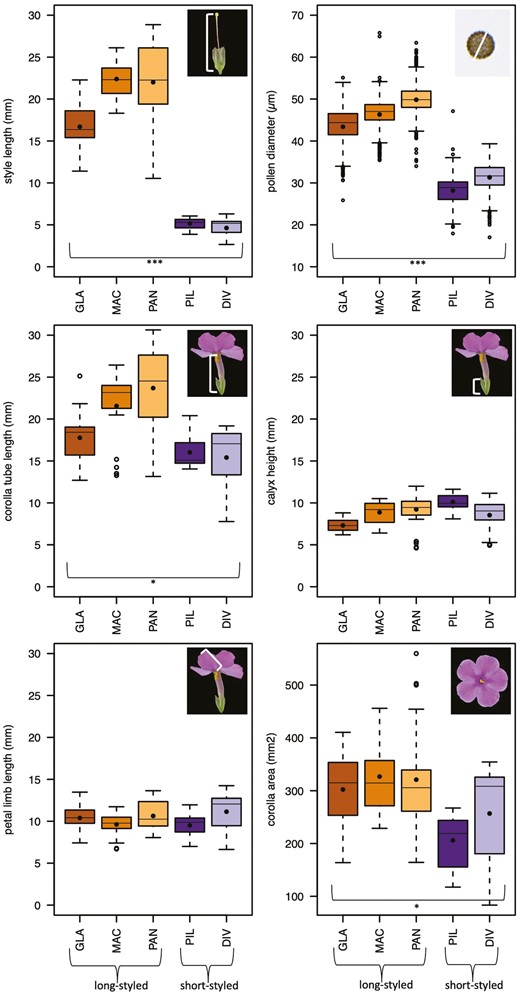
Long-styled species have larger pollen than short-styled species while other floral traits are similar. Five to nine flowers on three to five individuals per species were measured for the flower morphological traits, and pollen grains of three flowers of three individuals per species. The black dots indicate the means. The first three species in each plot (shades of orange) are long-styled, the following two (shades of purple) are short-styled. Statistically significant differences between the two style types are highlighted with stars, where ***<0.001, **<0.01, *<0.05.
We first confirm that style length variation is an asymmetric postmating prezygotic barrier between short- and long-styled species by performing pairwise reciprocal crosses to assess crossing success (seed set). Second, we measure a suite of floral morphological traits to determine the extent of floral divergence associated with style length variation. Finally, we investigate the mechanism of fertilization failure by documenting pollen tube growth in vivo on cross-pollinated pistils as well as quantifying pollen tube growth in vitro on a standardized medium (i.e., in a neutral environment not influenced by biochemical signals and resources in the pistil).
Methods
Study plants and greenhouse conditions
Plant cuttings were collected from natural populations occurring in rural as well as urban areas in the states of Illinois, Indiana, and Ohio during the summers of 2017–2019 and 2022 by Garner et al. (2024) and AFF (Supplementary Table S2). We propagated the field-collected cuttings under controlled conditions in greenhouses of the Arnold Arboretum of Harvard University with 16-h days at 23 °C during the day and 20 °C at night, and at 50% relative humidity. In this study, we used four to six individuals per species (in most cases from different populations, see Supplementary Table S2), and multiple clones per individual were generated throughout the years 2022 and 2023 by vegetative propagation. After a minimum growing time of 6 weeks, plants were vernalized in Conviron E8 growth chambers (Controlled Environments Inc., ND, USA) for 6 weeks at 7.2 °C and a 16:8 night:day cycle to induce flowering. All experimental plants were housed together in the greenhouse and randomized haphazardly, and all crossing experiments were performed on the same bench within the greenhouse. Crosses occurred over the course of 18 months as plants were induced to flower.
Crossing experiment for seed set
To assess the degree of successful sexual reproduction between and within species, we quantified seed set from controlled cross-pollinations. We performed all pairwise reciprocal crosses between the five focal species, including conspecific crosses, with three replicate individuals of each pair in each direction for a total of 75 cross combinations. For each cross combination, we pollinated 10 replicate flowers (except for nine cross combinations where only four to nine flowers were available to cross; see Supplementary Table S3). Flowers in all five species produce one fruit with a maximum of three seeds. Conspecific crosses were performed between individuals from different populations. To prevent potential self-pollination in all species and individuals, we removed petals and the attached anthers from buds that were predicted to open within 24–48 hr. Three days after anther removal, it was confirmed that stigmas were receptive (all three lobes clearly visible and separated) before transferring pollen onto them using forceps. We used light mesh fabric to bag pollinated flowers so we could collect and count seeds that would otherwise be explosively dehisced. We counted seeds produced from fully ripened fruits.
Floral trait measurements
We used five to nine mature flowers of three to five individuals per species to measure style length, calyx height, tube length, petal limb length, and corolla area (see Figure 2). These traits capture the main components of the overall variation in the size and shape of these flowers and relevant reproductive organs. The flowers were adhered to white paper with book tape and 2D-scanned using an EPSON Perfection V600 Photo scanner. A scale and color bar were included in each scan. For the style length and calyx height measurements, the anthers and petals were removed from the flowers. After setting the scale, we measured all linear traits in ImageJ 1.53k (Rasband, 1997–2018) using the “straight line tool.” For the corolla area, we color-thresholded the image such that only the corolla was highlighted and then used the “analyze particles” functions to quantify the highlighted area.
Pollen size quantification
We quantified the pollen size of three individuals per species using three flowers per individual. We transferred the mature anthers of a single flower into a 1.5 ml Eppendorf tube containing 50 µl of 1× phosphate-buffered saline (PBS). We vortexed the tube for 10 s to liberate the pollen from the anthers and transferred 10 µl of the PBS with pollen into one chamber of a 2-Chip Disposable Hemocytometer (Bulldog Bio Inc., NH, USA). We used an Axio Imager.A2 (Zeiss, Jena, Germany) microscope with ZEN 2.3 pro software to take standardized photos with a 10× objective. We batch-processed the resulting TIFF images in ImageJ 1.53k (Rasband, 1997-2018) using a customized macro based on (Visikol, 2019). In brief, the images were converted to grayscale, thresholded using default parameters, and then the “analyze particles” function was used to quantify the pollen area, and from this, we calculated pollen diameter in R (v.4.2.3; R Core Team, 2023). The scale was set globally on one image before running the macro.
In vivo pollen tube imaging
We used the same crossing procedure as described above in the seed set experiment to obtain images of hetero- and conspecific pollen tubes growing in pistils. We first experimentally verified that pollen tubes were able to reach conspecific ovules within 48 hr by performing a time series of conspecific (GLA, MAC, PAN, and PIL) crosses in which we inspected the pistils 10, 16, 18, 24, 45, 48, 72, and 96 hr after pollen transfer. The crossed flowers (style and calyx) were removed from the plants with forceps after 48 hr, transferred into glass scintillation vials, and covered with a formalin-aceto-alcohol (FAA). After 24 hr, FAA was exchanged with 70% ethanol and vials were stored at 4 °C until further processing. After a minimum of 24 hr, the ethanol was removed and 8M sodium hydroxide was added. After 24 hr in sodium hydroxide, we dissected out the pistils from the calyces and stained them in 0.1% aniline blue for 3 hr. The pistils were then mounted on microscope slides in 50% glycerol. Pistils were visualized in the DAPI channel (358 nm excitation) using an Axio Imager.A2 (Zeiss, Jena, Germany) microscope with ZEN 2.3 pro software. Pistils were imaged in either 5× or 10× magnification, depending on style length. For long-styled species, multiple fields of view were stitched together using the ZEN 2.3 pro software. We examined a minimum of three to six flowers per conspecific cross and 6–14 flowers per heterospecific cross until we obtained at least one representative image per cross-type. Due to overall low heterospecific crossing success (see Results section), a much larger number of crosses would have been required to obtain a quantitative measure of in vivo pollen tube growth success, which was not feasible due to the limited availability of flowers in these perennial species.
In vitro pollen tube quantification
To compare the growth of pollen from short- and long-styled species in a standardized environment, we germinated pollen grains of two to three individuals per species in an artificial growth medium (Rodriguez-Enriquez et al., 2013). We used the following modified concentrations: 10% sucrose, 0.1 mM spermidine, 10 mM GABA, with an adjusted pH of 7.5. We divided microscope slides (Fisher Scientific) into three compartments using an ImmEdge Hydrophobic Barrier PAP Pen (H-4000) (Vector Labs, Inc., CA, USA) and filled each with 125 µl of medium (we did not use cellulose membranes). Prepared slides were used on the same or next day, following the storage and acclimation procedures in Rodriguez-Enriquez et al. (2013). We used fine-tip (size #00) paint brushes to apply pollen directly taken from freshly collected flowers to the medium. The slides were then incubated in Petri dishes with a wet tissue for 24 hr at 24 °C in the dark. After incubation, we transferred them to 4 °C until imaging (which we performed immediately). We used an Axio Imager.A2 (Zeiss, Jena, Germany) microscope with ZEN 2.3 pro software for imaging. To be quantified, the pollen tube had to fulfill three criteria: (1) the length had to be at least three times the diameter of the pollen grain; (2) the beginning and end had to be clearly visible; and (3) the whole tube length had to be mostly in the same z-plane. From each pollen grain that passed our criteria, we took paired measurements of pollen diameter and pollen tube length. We used the “straight-line tool” in ImageJ to measure pollen diameter (the automated pollen size quantification described above could not be applied to the images of pollen tubes on media) and the “segmented line tool” to measure the pollen tubes.
Statistical analyses
We used R v.4.2.3 (R Core Team, 2023) to visualize and analyze the results. Functions in the R packages dplyr v.1.1.2 (Wickham et al., 2023) and here v.1.0.1 (Müller, 2020) were used for data processing.
To test if the number of seeds obtained per cross was significantly different between con- and heterospecific crosses, we constructed a generalized linear mixed-effect model with a negative binomial link function that included “cross-type” (conspecific vs. heterospecific) as a fixed effect, “pollen receiving species” and “pollen donor species” as random effects, and the number of crossed flowers as offset using the R package lme4 v. 1.1-34 (Bates et al., 2015). We used the simulation-based tests implemented in the R package DHARMa 0.4.6 (Hartig, 2022) to check the model fit (testing for dispersion and inspecting residuals). Marginal means were estimated with the emmeans function in the R Package emmeans 1.0.20 (Lenth, 2024).
To statistically assess the asymmetry in heterospecific crosses between species with different style types, we performed a paired t-test of the average number of seeds per flower across the three replicates per reciprocal cross direction.
We calculated RI based on seed set (the average number of seeds per flower obtained across three replicates of each species combination and direction of cross) following (Sobel & Chen, 2014) using their Equation 4A: RI = 1 − 2 (H/(H + C)), where H is heterospecific and C conspecific seed set. This metric ranges from −1 to 1, where a value of 1 indicates complete RI, a value of 0 indicates the absence of RI, and a value below 0 indicates that heterospecific matings are more successful than conspecific matings.
To statistically assess the morphological differences between long- and short-styled species, we built mixed effect models with “type” (style length long vs. short) as fixed effect and “individual” nested within “species” as random effect. In vitro pollen tube length data were log10 transformed. We used the R package lme4 v. 1.1-34 (Bates et al., 2015) to fit the models. To test the significance of the fixed effect, we compared the model with and without the fixed effect using the anova function (with REML = F). We checked whether model assumptions (linearity, homoscedasticity, and normality) were met by inspecting plots of residuals vs. fitted values, and Q-Q plots, respectively. In addition, we performed Pearson’s correlation tests between pollen diameter and pollen tube length by species and across all species.
Results
Seed set is asymmetric
Our results confirm that long-styled plants will not set seed with pollen from short-styled species (Figure 3). We found that the seed set was significantly lower in heterospecific crosses (marginal mean = 1.21) compared to conspecific crosses (marginal mean = 10.45) (z = -15.51, P < 0.001) (Figure 3; Table 1; Supplementary Table S4). In all five species, a single flower can set a maximum of three seeds. The average number of seeds per flower in conspecific crosses ranged from 1 to 1.5 and was < 1 in all heterospecific crosses (Figure 3a). In heterospecific crosses between different long-styled species (long × long) or between the two short-styled species (short × short), an average of 0.31–0.33 seeds per flower were obtained per crossing direction (with the exception of MACxPAN, which did not produce any seeds across the three replicates; Figure 3b). In heterospecific crosses between species with different style lengths, seeds (on average 0.14 seeds per flower) developed only in crosses when short-styled species received pollen from long-styled species (short × long). When long-styled species received pollen from short-styled species (long × short), no seeds were produced (Figure 3c; Supplementary Table S4). This pattern of asymmetry is significant (t(5) = 2.36, p = 0.032) and is also reflected in the RI values (Table 2). RI equals 1 (fully reproductively isolated) in all long × short crosses, and values below 0.5 are exclusively found in either long × long heterospecific crosses or short × long crosses.
Results from a negative binomial generalized mixed effects model to assess differences in the number of seeds obtained in conspecific vs. heterospecific crosses with pollen-receiving species and pollen-donor species as random effects, and the number of crossed flowers as offset.
Value . | Fixed effect . | Estimate . | ± . | SE . | z-value . | p-value . | . |
---|---|---|---|---|---|---|---|
Number of seeds | Intercept (conspecific cross-type) | −7.20 | ± | 0.46 | −15.51 | <0.001 | *** |
Heterospecific cross-type | −2.16 | ± | 0.43 | −5.06 | <0.001 | *** |
Value . | Fixed effect . | Estimate . | ± . | SE . | z-value . | p-value . | . |
---|---|---|---|---|---|---|---|
Number of seeds | Intercept (conspecific cross-type) | −7.20 | ± | 0.46 | −15.51 | <0.001 | *** |
Heterospecific cross-type | −2.16 | ± | 0.43 | −5.06 | <0.001 | *** |
Significance codes: ***<0.001, **<0.01, *<0.05, <0.1. SE, standard error.
Results from a negative binomial generalized mixed effects model to assess differences in the number of seeds obtained in conspecific vs. heterospecific crosses with pollen-receiving species and pollen-donor species as random effects, and the number of crossed flowers as offset.
Value . | Fixed effect . | Estimate . | ± . | SE . | z-value . | p-value . | . |
---|---|---|---|---|---|---|---|
Number of seeds | Intercept (conspecific cross-type) | −7.20 | ± | 0.46 | −15.51 | <0.001 | *** |
Heterospecific cross-type | −2.16 | ± | 0.43 | −5.06 | <0.001 | *** |
Value . | Fixed effect . | Estimate . | ± . | SE . | z-value . | p-value . | . |
---|---|---|---|---|---|---|---|
Number of seeds | Intercept (conspecific cross-type) | −7.20 | ± | 0.46 | −15.51 | <0.001 | *** |
Heterospecific cross-type | −2.16 | ± | 0.43 | −5.06 | <0.001 | *** |
Significance codes: ***<0.001, **<0.01, *<0.05, <0.1. SE, standard error.
Postpollination reproductive isolation (RI) values for each heterospecific cross with the first four crosses between species with similar style lengths, and the remaining between species with different style lengths (see also Supplementary Table S4).
Species A . | Species B . | RI when pollen recipient is species A . | RI when pollen recipient is species B . |
---|---|---|---|
DIV (short) | PIL (short) | 0.670 | 0.909 |
GLA (long) | PAN (long) | 0.686 | 0.415 |
GLA (long) | MAC (long) | 0.365 | 0.262 |
MAC (long) | PAN (long) | 1.000 | 0.871 |
DIV (short) | GLA (long) | 0.368 | 1.000 |
DIV (short) | MAC (long) | 0.916 | 1.000 |
DIV (short) | PAN (long) | 0.876 | 1.000 |
PIL (short) | GLA (long) | 0.377 | 1.000 |
PIL (short) | PAN (long) | 0.750 | 1.000 |
PIL (short) | MAC (long) | 1.000 | 1.000 |
Species A . | Species B . | RI when pollen recipient is species A . | RI when pollen recipient is species B . |
---|---|---|---|
DIV (short) | PIL (short) | 0.670 | 0.909 |
GLA (long) | PAN (long) | 0.686 | 0.415 |
GLA (long) | MAC (long) | 0.365 | 0.262 |
MAC (long) | PAN (long) | 1.000 | 0.871 |
DIV (short) | GLA (long) | 0.368 | 1.000 |
DIV (short) | MAC (long) | 0.916 | 1.000 |
DIV (short) | PAN (long) | 0.876 | 1.000 |
PIL (short) | GLA (long) | 0.377 | 1.000 |
PIL (short) | PAN (long) | 0.750 | 1.000 |
PIL (short) | MAC (long) | 1.000 | 1.000 |
DIV, P. divaricata (including subspecies divaricata and laphamii); GLA, P. glaberrima ssp. interior; MAC, P. maculata; PAN, P. paniculata; PIL, P. pilosa ssp. pilosa.
Postpollination reproductive isolation (RI) values for each heterospecific cross with the first four crosses between species with similar style lengths, and the remaining between species with different style lengths (see also Supplementary Table S4).
Species A . | Species B . | RI when pollen recipient is species A . | RI when pollen recipient is species B . |
---|---|---|---|
DIV (short) | PIL (short) | 0.670 | 0.909 |
GLA (long) | PAN (long) | 0.686 | 0.415 |
GLA (long) | MAC (long) | 0.365 | 0.262 |
MAC (long) | PAN (long) | 1.000 | 0.871 |
DIV (short) | GLA (long) | 0.368 | 1.000 |
DIV (short) | MAC (long) | 0.916 | 1.000 |
DIV (short) | PAN (long) | 0.876 | 1.000 |
PIL (short) | GLA (long) | 0.377 | 1.000 |
PIL (short) | PAN (long) | 0.750 | 1.000 |
PIL (short) | MAC (long) | 1.000 | 1.000 |
Species A . | Species B . | RI when pollen recipient is species A . | RI when pollen recipient is species B . |
---|---|---|---|
DIV (short) | PIL (short) | 0.670 | 0.909 |
GLA (long) | PAN (long) | 0.686 | 0.415 |
GLA (long) | MAC (long) | 0.365 | 0.262 |
MAC (long) | PAN (long) | 1.000 | 0.871 |
DIV (short) | GLA (long) | 0.368 | 1.000 |
DIV (short) | MAC (long) | 0.916 | 1.000 |
DIV (short) | PAN (long) | 0.876 | 1.000 |
PIL (short) | GLA (long) | 0.377 | 1.000 |
PIL (short) | PAN (long) | 0.750 | 1.000 |
PIL (short) | MAC (long) | 1.000 | 1.000 |
DIV, P. divaricata (including subspecies divaricata and laphamii); GLA, P. glaberrima ssp. interior; MAC, P. maculata; PAN, P. paniculata; PIL, P. pilosa ssp. pilosa.
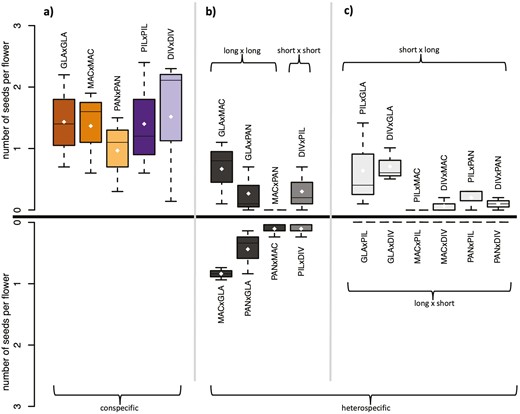
Seed set is reduced in heterospecific vs conspecific crosses and asymmetric in crosses between long- and short-styled species. (a) Average numbers of seeds obtained per flower in conspecific crosses. (b) Average number of seeds obtained per flower in heterospecific crosses between species with the same style type (long × long or short × short). Reciprocal crosses are shown above and below the zero line (thick horizontal line in the middle of the plot), with the y-axis flipped in the below part. (c) Average number of seeds obtained per flower in heterospecific crosses between species with different style types. Crosses with a short-styled pollen-receiving species and a long-styled pollen donor (short × long) are shown above the zero line, crosses with a long-styled pollen-receiving species and a long-styled pollen donor (long × short) are shown below the zero line (y-axis flipped). Each cross consists of three replicates with up to ten crossed flowers per replicate (see Supplementary Table S3). Shown are the average numbers of seeds obtained per flower in each replicate. The maximum number of seeds per flower is three. The white dots indicate the means. Species abbreviations are given as pollen-receiving species × pollen-donor species.
Pollen size and some floral traits vary with style length
As expected, style lengths were on average four times greater in long-styled species than in short-styled species (χ2(1) = 13.09, p = 0.0003) (Table 3; Figure 2). Also significantly different between the two style types were pollen diameters, which were on average 1.6 times greater in long-styled species than in short-styled species (χ2(1) = 12.23, p = 0.0005), corolla tube lengths, which were on average 1.3 times greater in long-styled species than in short-styled species (χ2(1) = 4.21, p = 0.04), and corolla area, which was on average 1.4 times greater in long-styled species than in short-styled species (χ2(1) = 6.4346, p = 0.011) (Table 3; Figure 2). The latter difference seems to be mainly driven by one of the short-styled species (PIL), which has slender petal limbs compared to the long-styled species. Calyx height (χ2(1) = 0.54, p = 0.463) and petal limb length (χ2(1) = 0.04, p = 0.840) did not significantly differ between short- and long-styled species (Table 3; Figure 2).
Results from mixed effect models of the continuous floral trait measurements with individual nested within species as random effect.
Trait . | Fixed effect . | Estimate . | ± . | SE . | df . | χ2-value . | p-value . | . |
---|---|---|---|---|---|---|---|---|
Style length | Intercept (long style) | 20.31 | ± | 1.57 | ||||
Short style | −15.51 | ± | 2.55 | 1 | 13.09 | 0.0003 | *** | |
Calyx height | Intercept (long style) | 8.47 | ± | 0.67 | ||||
Short style | 0.65 | ± | 1.11 | 1 | 0.54 | 0.4630 | ||
Corolla tube length | Intercept (long style) | 20.74 | ± | 1.59 | ||||
Short style | −5.38 | ± | 2.66 | 1 | 4.21 | 0.0401 | * | |
Petal limb length | Intercept (long style) | 10.25 | ± | 0.39 | ||||
Short style | −0.13 | ± | 0.69 | 1 | 0.04 | 0.8419 | ||
Corolla area | Intercept (long style) | 314.23 | ± | 19.95 | ||||
Short style | −91.36 | ± | 34.60 | 1 | 6.43 | 0.0112 | * | |
Pollen diameter | Intercept (long style) | 46.29 | ± | 1.84 | ||||
Short style | −16.39 | ± | 2.91 | 1 | 12.23 | 0.0005 | *** | |
Pollen tube length | Intercept (long style) | 667.04 | ± | 54.94 | ||||
Short style | −281.60 | ± | 87.39 | 1 | 7.38 | 0.0066 | ** |
Trait . | Fixed effect . | Estimate . | ± . | SE . | df . | χ2-value . | p-value . | . |
---|---|---|---|---|---|---|---|---|
Style length | Intercept (long style) | 20.31 | ± | 1.57 | ||||
Short style | −15.51 | ± | 2.55 | 1 | 13.09 | 0.0003 | *** | |
Calyx height | Intercept (long style) | 8.47 | ± | 0.67 | ||||
Short style | 0.65 | ± | 1.11 | 1 | 0.54 | 0.4630 | ||
Corolla tube length | Intercept (long style) | 20.74 | ± | 1.59 | ||||
Short style | −5.38 | ± | 2.66 | 1 | 4.21 | 0.0401 | * | |
Petal limb length | Intercept (long style) | 10.25 | ± | 0.39 | ||||
Short style | −0.13 | ± | 0.69 | 1 | 0.04 | 0.8419 | ||
Corolla area | Intercept (long style) | 314.23 | ± | 19.95 | ||||
Short style | −91.36 | ± | 34.60 | 1 | 6.43 | 0.0112 | * | |
Pollen diameter | Intercept (long style) | 46.29 | ± | 1.84 | ||||
Short style | −16.39 | ± | 2.91 | 1 | 12.23 | 0.0005 | *** | |
Pollen tube length | Intercept (long style) | 667.04 | ± | 54.94 | ||||
Short style | −281.60 | ± | 87.39 | 1 | 7.38 | 0.0066 | ** |
Significance codes: ***<0.001, **<0.01, *<0.05, <0.1. SE, standard error; df, degrees of freedom.
Results from mixed effect models of the continuous floral trait measurements with individual nested within species as random effect.
Trait . | Fixed effect . | Estimate . | ± . | SE . | df . | χ2-value . | p-value . | . |
---|---|---|---|---|---|---|---|---|
Style length | Intercept (long style) | 20.31 | ± | 1.57 | ||||
Short style | −15.51 | ± | 2.55 | 1 | 13.09 | 0.0003 | *** | |
Calyx height | Intercept (long style) | 8.47 | ± | 0.67 | ||||
Short style | 0.65 | ± | 1.11 | 1 | 0.54 | 0.4630 | ||
Corolla tube length | Intercept (long style) | 20.74 | ± | 1.59 | ||||
Short style | −5.38 | ± | 2.66 | 1 | 4.21 | 0.0401 | * | |
Petal limb length | Intercept (long style) | 10.25 | ± | 0.39 | ||||
Short style | −0.13 | ± | 0.69 | 1 | 0.04 | 0.8419 | ||
Corolla area | Intercept (long style) | 314.23 | ± | 19.95 | ||||
Short style | −91.36 | ± | 34.60 | 1 | 6.43 | 0.0112 | * | |
Pollen diameter | Intercept (long style) | 46.29 | ± | 1.84 | ||||
Short style | −16.39 | ± | 2.91 | 1 | 12.23 | 0.0005 | *** | |
Pollen tube length | Intercept (long style) | 667.04 | ± | 54.94 | ||||
Short style | −281.60 | ± | 87.39 | 1 | 7.38 | 0.0066 | ** |
Trait . | Fixed effect . | Estimate . | ± . | SE . | df . | χ2-value . | p-value . | . |
---|---|---|---|---|---|---|---|---|
Style length | Intercept (long style) | 20.31 | ± | 1.57 | ||||
Short style | −15.51 | ± | 2.55 | 1 | 13.09 | 0.0003 | *** | |
Calyx height | Intercept (long style) | 8.47 | ± | 0.67 | ||||
Short style | 0.65 | ± | 1.11 | 1 | 0.54 | 0.4630 | ||
Corolla tube length | Intercept (long style) | 20.74 | ± | 1.59 | ||||
Short style | −5.38 | ± | 2.66 | 1 | 4.21 | 0.0401 | * | |
Petal limb length | Intercept (long style) | 10.25 | ± | 0.39 | ||||
Short style | −0.13 | ± | 0.69 | 1 | 0.04 | 0.8419 | ||
Corolla area | Intercept (long style) | 314.23 | ± | 19.95 | ||||
Short style | −91.36 | ± | 34.60 | 1 | 6.43 | 0.0112 | * | |
Pollen diameter | Intercept (long style) | 46.29 | ± | 1.84 | ||||
Short style | −16.39 | ± | 2.91 | 1 | 12.23 | 0.0005 | *** | |
Pollen tube length | Intercept (long style) | 667.04 | ± | 54.94 | ||||
Short style | −281.60 | ± | 87.39 | 1 | 7.38 | 0.0066 | ** |
Significance codes: ***<0.001, **<0.01, *<0.05, <0.1. SE, standard error; df, degrees of freedom.
In vivo pollen tube growth images confirm asymmetry
In styles on which we performed controlled crosses, we observed some pollen tubes from long-styled species reaching and entering the ovaries of short-styled species, even overshooting (Figure 4b). Pollen tubes from short-styled species were never observed to reach the ovaries of long-styled species (Figure 4c).
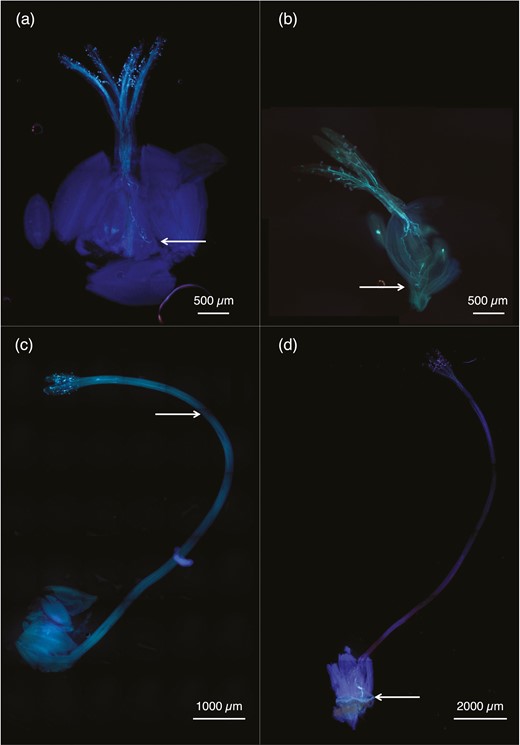
Representative images of pollen tubes grown in vivo. (a) A representative image of a short-style conspecific cross, (b) a cross wherein a short-styled species received pollen from a long-styled species, (c) a cross wherein a long-styled species received pollen from a short-styled species, and (d) a long-style conspecific cross. The arrows indicate how far the pollen tubes grew within 48 hr.
In vitro pollen tube length correlates with style size and pollen size
Pollen tube length grown in vitro for 24 hr on a standardized medium differed significantly by style type, with long-styled species growing pollen tubes that were on average 1.7 times longer than those of short-styled species (χ2(1) = 7.38, p = 0.007; Table 3; Figure 5). The length of the pollen tubes was highly correlated with pollen diameter across all species (r(643) = 0.429, p < 0.001) as well as within species (GLA: r(129) = 0.235, p = 0.007; MAC: r(116) = 0.207, p = 0.024; PAN: r(97) = 0.286, p = 0.004; PIL: r(186) = 0.269, p = 0.0002; and DIV: r(107) = 0.279, p = 0.003) (Figure 5).
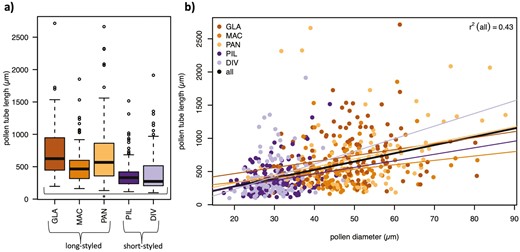
Pollen from long-styled species grow longer tubes in vitro and pollen tube length is significantly correlated with pollen grain size within and across species. Pollen grains were germinated and allowed to grow pollen tubes for 24 hr in a standardized medium, at which point 13–98 pollen grains and tubes were measured of two to three individuals per species. (a) Pollen tubes are significantly longer in long-styled species than in short-styled species. (b) Pollen tube length is highly correlated within species and across all species (thick line).
Discussion
We used a combination of crossing experiments, pollen tube growth assays, and morphological measurements to investigate the causes of asymmetric reproductive success between five species of Phlox wildflowers with varying style lengths. Our results show that short-styled species have smaller pollen, which grew shorter pollen tubes in a neutral environment (in vitro) than the larger pollen from long-styled species. Tubes from these small pollen grains were not observed to reach the ovaries of long-styled species in hand-pollinated pistils (in vivo). These findings suggest that the underlying mechanism driving asymmetric crossing success between species of different style lengths is, at least partially, due to differences in resource properties of the pollen, generating a mechanical postmating prezygotic barrier.
The crossing results presented here corroborate previous experiments involving these Phlox species (Levin, 1963, 1966; Levin & Smith, 1965; Zale, 2014): heterospecific crosses yielded fewer seeds than conspecific crosses, and crosses between species with different style lengths only yielded seeds when the pollen parent was long-styled. As in these previous experiments, we found that controlled crosses within a species often did not result in the maximum possible seed set (three seeds per fruit). This phenomenon could be because greenhouse conditions are not optimal for fertilization or seed maturation, or due to a normal developmental constraint that flowers tend to only mature seeds in a subset of available ovules. No data exist on average seed set per flower in natural conditions, although because all crosses were conducted under a common-garden environment, we expect no systematic bias between conspecific versus heterospecific cross types.
Asymmetric crossing success between species with divergent style lengths is well documented (Lee et al., 2008; Nista et al., 2015; Perez & Moore, 1985; Williams & Rouse, 1988; Yost & Kay, 2009), but the underlying mechanism driving this reproductive isolating barrier is often uncertain. To disentangle the biochemical effect of the pistil environment from the resource properties of the pollen grains, we grew pollen tubes in a standardized agar medium, thereby allowing pollen tubes to grow without the influence of a particular pistil environment. While neither pollen type grew pollen tubes as long as the length of any style in the experimental time, indicating that successful pollen tube growth also relies on signals and nutrients from the stylar tissue (Stephenson et al., 2003), larger pollen may have a head start due to higher initial energy content (Baker & Baker, 1979; Cruden & Miller-Ward, 1981; Manicacci & Barrett, 1995). We found that consistent with our previous measure of pollen diameter, germinated pollen from short-styled plants is significantly smaller than pollen from long-styled plants. We then found that when allowed to grow for the same amount of time, pollen from short-styled species grows significantly shorter pollen tubes than pollen from long-styled species. Finally, we found that pollen size and pollen tube length are correlated not only across species but also within each of the species we examined; smaller pollen makes smaller tubes both within and between species. This final result is particularly interesting because it indicates the relationship between pollen size and tube length is robust to any species-specific differences in pollen content or chemistry. Together, our data support the conclusion that pollen tubes from short-styled plants are limited by available resources in the small pollen grains and thus cannot successfully reproduce as pollen donors to long-styled plants.
Style length variation across species is often associated with shifts in floral size following mating system transitions as a component of the evolution of selfing syndrome: Selfing species typically have an overall reduction in floral size (and thus style length) relative to closely related outcrossing species, which may reflect the absence of the need to attract pollinators and facilitate self-pollination (Cutter, 2019; Lanuza et al., 2023; Sicard & Lenhard, 2011; Tsuchimatsu & Fujii, 2022). Importantly, these evolutionary correlations complicate interpretations of the underlying mechanism driving reproductive isolation. Transitions to self-fertilization are also associated with patterns of asymmetric postmating prezygotic reproductive isolation due to biochemical mechanisms. A classic asymmetric crossing barrier in flowering plants is caused by a correlation in self- and heterospecific pollen rejection mediated by shared molecular pathways (Hancock et al., 2003; Li & Chetelat, 2015; Roda & Hopkins, 2019). This overlap in pollen rejection has manifested a pattern of self-incompatible species (often with longer styles) being able to recognize and reject pollen from self-compatible species but not vice versa. In this context, Phlox is an interesting system to study for two reasons. First, in Phlox, both the long- and the short-styled species are self-incompatible and are predominantly outcrossing (Levin, 1963, 1966). The conservation of mating system across these five species can isolate the role of the mechanical mismatch between pollen and style length variation in reproductive isolation. Second, the overall floral size and shape is largely consistent across species. Although we do see minimal shifts in corolla tube length, the drastic difference between the long- and short-styled Phlox species in our study is predominantly associated with a change in the position of the stigma relative to the corolla tube opening and not with variation in the length of petals or calyx (Figures 1 and 2). Therefore, our study demonstrates that style length alone can cause reproductive isolation irrespective of biochemical changes associated with pollen recognition or morphological changes associated with overall floral size differences.
Nevertheless, the mechanical barrier driven by style length differences in Phlox likely acts in concert with these other postmating prezygotic isolating barriers. We find support for this expectation from our controlled crossing data. First, crossing success was reduced in all heterospecific crosses, even those between species of similar style lengths. Second, pollen from long-styled species only sometimes resulted in seed set when used to pollinate a short-styled species, even though they generated sufficiently long pollen tubes to reach the ovaries of these species. Third, we observed generally low germination rates of pollen on heterospecific stigmas, and germinated pollen on heterospecific stigmas often did not result in pollen tube growth. This observation indicates the presence of additional postmating prezygotic reproductive isolating mechanisms, which is not surprising considering that successful fertilization requires a suite of sequential interactions between pollen and pistil, each of which can act as a barrier if the previous fails (reviewed in Broz & Bedinger, 2021). These pollen–pistil barriers may be important in preventing gene flow across Phlox species broadly, while style length variation prevents interspecific gene flow in just one crossing direction.
Because variation in style length across species does not appear to be associated with other major transitions in reproductive strategy (e.g., transition to selfing), it is challenging to infer what drove the evolution of style length variation in this system. Across the phylogeny of Phlox, most species have long styles, and the monophyletic clade of short-styled species is nested within the broader group of long-styled species (Garner et al., 2024). This phylogenetic pattern suggests that the ancestor of the short-styled Phlox had a long style, and that short style/small pollen is a derived state having evolved just once. Therefore, as style length was evolving from long to short, individuals with short styles would have been unable to pollinate long-styled plants but would still be accessible to (and potentially out-competed by) pollen from long-styled individuals (McCallum & Chang, 2016). This scenario would present a likely reproductive disadvantage to short-styled plants relative to long-styled individuals. We, therefore, hypothesize that style length evolved due to selection for some other selective purpose and not due to selection for its role in decreasing hybridization. As selection drove the evolution of short styles/small pollen, reproductive isolation may have emerged as a byproduct. Future studies are necessary to better understand what might have driven these evolutionary changes in reproductive organs. For example, our findings motivate future studies to determine if shorter styles allow the stigma to be better protected from environmental damage inside the walls of the corolla tube. Furthermore, it will be important to determine if pollen size and pistil length are evolving genetically independently or if genetic pleiotropy or linkage causes selection on one trait to result in a correlated response of the other. Our study demonstrates the evolutionary implications of this morphological change, but future work will need to untangle its evolutionary cause.
Asymmetries in reproductive isolation in plants are most commonly observed at the postzygotic stage or at the postpollination prezygotic stage (reviewed in Christie et al., 2022 and Tiffin et al., 2001). At the postpollination prezygotic stage, reproductive isolation is usually due to differences in mating systems or style lengths (Christie et al., 2022). We have shown that the pattern of asymmetry in the Phlox system is caused by a mechanical mismatch between pollen size and style length driven by the inability of less-resourced pollen to grow tubes long enough to traverse long-styled pistils, likely enhanced by additional incompatibilities between pollen and pistils. Such asymmetries affect the direction of gene flow and can impact introgression dynamics (Christie et al., 2022; Tiffin et al., 2001). The next steps should thus determine if these asymmetries translate into asymmetric patterns of gene flow between species. In general, prezygotic reproductive isolation tends to be stronger than postzygotic reproductive isolation in plants (Christie et al., 2022; Lowry et al., 2008), suggesting that the impact of pollen–pistil incompatibilities is likely important for determining patterns of gene flow across species boundaries.
Supplementary material
Supplementary material is available online at Evolution.
Data availability
Data and R-scripts are available from the Dryad digital repository under https://doi.org/10.5061/dryad.fttdz091s.
Author contributions
Conceptualization: A.F.F., R.H.; Methodology: A.F.F., G.B., N.F.L., R.H.; Formal analysis: A.F.F.; Investigation: A.F.F., G.B., N.F.L., R.P.; Resources: R.H.; Data curation: A.F.F.; Writing—Original draft: A.F.F., G.B., R.H.; Writing—Review & editing: A.F.F., G.B., N.F.L., R.P., R.H.; Visualization: A.F.F., G.B., N.F.L.; Supervision: A.F.F., R.H.; Project administration: A.F.F., R.H.; Funding acquisition: A.F.F., R.H.
Funding
Swiss National Science Foundation (SNSF) Postdoc Mobility Grant No. 203023 to A.F.F.; National Science Foundation (NSF) CAREER Award No. 1844906 to R.H.; NSF IOS—19061133 to R.H.; and National Institutes of Health NIGMS—1R35GM142742-01 to R.H.
Conflict of interest:
The authors declare no conflict of interest. The editorial process of the manuscript was conducted independently of R.H., who is an Associate Editor of Evolution.
Acknowledgments
Many thanks to Austin Garner for providing his insights on the Midwestern perennial Phlox; to Izzy Acevedo for initiating the propagation of the experimental plants; to Megan Ardolino, Lee Toomey, Scott Pedemonte, and Mike Barrett for their work in the greenhouses; and to the Hopkins lab group for many helpful discussions and comments. We thank the members of the following departments and parks for issuing permits for the 2022 sample collection to A.F.F. and for facilitating access: Indiana Department of Natural Resources, Eagle Creek Park (IN), Illinois Department of Natural Resources, Illinois Nature Preserves Commission, Champaign County Forest Preserve District (IL), Grand Prairie Friends (IL), Forest Preserves of Cook County (IL), and Johnny Appleseed Metropolitan Park District (OH).
References
Author notes
Present address: Anna F. Feller Department of Environmental Systems Science, ETH Zürich, Zürich 8092, Switzerland