-
PDF
- Split View
-
Views
-
Cite
Cite
Jasen W Liu, Paulo Milet-Pinheiro, Günter Gerlach, Manfred Ayasse, Carlos Eduardo Pereira Nunes, Isabel Alves-dos-Santos, Santiago R Ramírez, Macroevolution of floral scent chemistry across radiations of male euglossine bee-pollinated plants, Evolution, Volume 78, Issue 1, January 2024, Pages 98–110, https://doi.org/10.1093/evolut/qpad194
- Share Icon Share
Abstract
Floral volatiles play key roles as signaling agents that mediate interactions between plants and animals. Despite their importance, few studies have investigated broad patterns of volatile variation across groups of plants that share pollinators, particularly in a phylogenetic context. The “perfume flowers,” Neotropical plant species exhibiting exclusive pollination by male euglossine bees in search of chemical rewards, present an intriguing system to investigate these patterns due to the unique function of their chemical phenotypes as both signaling agents and rewards. We leverage recently developed phylogenies and knowledge of biosynthesis, along with decades of chemical ecology research, to characterize axes of variation in the chemistry of perfume flowers, as well as understand their evolution at finer taxonomic scales. We detect pervasive chemical convergence, with many species across families exhibiting similar volatile phenotypes. Scent profiles of most species are dominated by compounds of either the phenylpropanoid or terpenoid biosynthesis pathways, while terpenoid compounds drive more subtle axes of variation. We find recapitulation of these patterns within two independent radiations of perfume flower orchids, in which we further detect evidence for the rapid evolution of divergent floral chemistries, consistent with the putative importance of scent in the process of adaptation and speciation.
Resumen
Los volátiles florales cumplen una función fundamental en las interacciones entre plantas y animales. A pesar de su importancia, pocos estudios se han enfocado en establecer patrones generales de evolución de volátiles en grupos de plantas que comparten polinizadores, especialmente en un contexto filogenético. Las “flores perfumadas” de los trópicos americanos dependen exclusivamente de abejas euglosinas machos que buscan y colectan compuestos químicos para usar en el cortejo y a cambio prestan servicios de polinización. Este grupo de flores ofrecen oportunidades para investigar patrones evolutivos y función de los fenotipos químicos como agentes de señalización y recompensas. Utilizando filogenias recientes e información de biosíntesis, caracterizamos la variación química y su evolución a escalas taxonómicas más finas. Detectamos convergencia química generalizada entre especies de diferentes familias que muestran fenotipos volátiles similares. La mayoría de las especies tienen perfiles de fragancia dominados por compuestos de las vías de biosíntesis de fenilpropanoides o terpenoides, con estos últimos mostrando patrones de variación más sutil. También observamos patrones similares en dos radiaciones independientes de orquídeas de flores perfumadas, con evidencia de divergencia rápidas en los compuestos volátiles florales. Estos hallazgos respaldan la importancia del olor en adaptación y especiación en plantas con asociaciones especializadas.
Resumo
Os voláteis florais desempenham papéis essenciais como sinais na mediação das interações entre plantas e animais. Apesar de sua importância, poucos estudos investigaram padrões abrangentes de variação desses atributos entre grupos de plantas que compartilham polinizadores, especialmente em um contexto filogenético. As “flores de perfume,” flores de espécies neotropicais que apresentam polinização exclusiva por machos de abelhas Euglossini em busca de recursos químicos, constituem um sistema intrigante para investigar esses padrões graças à função singular de seus fenótipos químicos como atrativos e recompensas florais. Utilizando filogenias recentemente desenvolvidas, conhecimento sobre as vias biossintéticas e décadas de pesquisa em ecologia química, caracterizamos as variações químicas das flores de perfume para compreender sua evolução em uma fina escala taxonômica. Observamos convergências químicas amplamente difundidas, com muitas espécies de diferentes famílias exibindo fenótipos voláteis semelhantes. Os perfis de voláteis florais da maioria das espécies são dominados por compostos das vias dos fenilpropanóides ou dos terpenóides, sendo que estes últimos impulsionam variações mais sutis. Identificamos a repetição desses padrões em duas radiações adaptivas independentes de orquídeas, nas quais também encontramos evidências de uma rápida evolução de voláteis florais divergentes, condizente com a suposta importância destes atributos no processo de adaptação e especiação.
Introduction
A key objective of evolutionary biology is to understand patterns of complex trait variation and the mechanisms that generate this diversity. Of particular interest are traits that evolve rapidly during the process of speciation, such as those that facilitate reproductive isolation. In angiosperms (flowering plants), floral traits such as scent and morphology that mediate interactions with animal pollinators are critical for efficient pollen removal, transport, and receipt by conspecifics (Waser & Ollerton, 2006). With an estimated 87.5% of all flowering plants requiring pollinators to ensure reproductive success, these traits have evolved to fill a diverse phenotypic space spanning a wide range of animal sensory modalities (Ollerton et al., 2011). Divergence in these floral traits can result in differential patterns of visitation, potentially driving prezygotic isolation and incipient speciation if the barriers are sufficiently strong.
Pollinator shifts are often accompanied by changes in floral traits, including color (Dyer et al., 2012; Trunschke et al., 2021), morphology (Sayers et al., 2021), and scent (Amrad et al., 2016; Ayasse et al., 2011; Mant et al., 2005). Floral scent traits in particular offer nuanced insights into the evolution of prezygotic reproductive barriers in angiosperms (Grant, 1949). These chemical signals play key roles in mediating pollinator attraction, and specificity and divergence in volatile expression have been demonstrated to generate patterns of differential pollinator visitation, a process that may cause incipient speciation (Byers et al., 2014; Dressler, 1968; Xu et al., 2012). Floral volatiles are heritable and have the capacity to evolve rapidly in response to pollinators at the intraspecific level, with both selection experiments detecting substantial changes within just a few generations (Gervasi & Schiestl, 2017; Opedal et al., 2022; Ramos & Schiestl, 2020; Zu et al., 2016) and the observation of strong contemporary selection relative to other floral traits (Parachnowitsch et al., 2012). At broader scales, the observations of high olfactory signal disparity among sympatric species, both at macroevolutionary and community levels, further demonstrate the capacity of natural selection to act upon these traits to maintain species boundaries (Eisen et al., 2022; Friberg et al., 2019; Weber et al., 2018).
Floral volatiles usually contain mixtures of compounds belonging to different compound classes, many associated with well-characterized biosynthetic pathways that are conserved across taxa, such as the terpenoid and phenylpropanoid pathways (Junker, 2018; Opedal et al., 2022). As certain compounds present within a blend may be derived from the same biosynthetic pathway, this creates issues with nonindependence, making standard analyses of chemical variation using methods that treat each compound as an independent variable less interpretable (Gfrerer et al., 2021). Another challenging aspect of understanding patterns of scent variation across different taxa is the uncertainty of compound functionality. In most floral systems utilizing honest signals, scent acts as a proxy for other rewards, such as nectar and pollen. However, one system stands out as a unique case where scent acts as both a signaling agent and as a reward: the so-called “perfume flowers” (sensu Vogel, 1966) of the Neotropics.
Perfume flowers are pollinated exclusively by male euglossine bees. Males visit flowers in addition to other sources such as rotting wood and fungi to collect volatile and semivolatile compounds that are stored as blends in hindleg pockets for future courtship displays (Dressler, 1968; Henske et al., 2023; Whitten et al., 1993). These chemical blends act as species-specific signals and exhibit far less variation within species compared to among species (Darragh et al., 2023; Weber et al., 2016). An analysis of two closely related sister species revealed that odorant receptors (ORs) exhibit signatures of rapid evolution between a sister pair of cryptic Euglossa species, further demonstrating the importance of olfaction in this group of bees during the process of speciation (Brand et al., 2020). As such, divergence in plant volatiles can result in changes in the composition of bee visitors, driving reproductive isolation.
Approximately 1,000 species of plants have evolved specialized pollination by male euglossine bees, spanning phylogenetically disparate families, including the Annonaceae, Araceae, Arecaceae, Bromeliaceae, Clusiaceae, Euphorbiaceae, Gesneriaceae, Orchidaceae, Solanaceae, and Theaceae, among others (Armbruster, 2011; Armbruster et al., 1992; Gerlach & Schill, 1991; Knudsen, 2002; Milet-Pinheiro et al., 2021; Nogueira et al., 1998; Sazima et al., 1993; Teichert et al., 2009). Perfume flowers evolved well after the origin of perfume collection behavior (~15 MYA vs. ~34–38 MYA), likely exploiting pre-existing sensory biases exhibited by bees (Ramírez et al., 2011). Around 80% of these plants lie within the hyperdiverse family Orchidaceae, and male euglossine pollination has resulted in faster diversification rates within this clade (Givnish et al., 2015).
Due to the unique role that scent plays as both a signaling agent and a reward in this system, and the known function of many compounds present in these scent profiles as attractants to male euglossine bees, perfume flowers offer an unparalleled opportunity to investigate scent variation across phylogeny in the context of complex functional trait evolution. Numerous chemical ecology studies have characterized the scent profiles of these male euglossine-pollinated plants, with a rich literature spanning over 50 years of research. However, no recent syntheses of the data have been conducted, and to date, no phylogenetically informed analyses of scent have been performed within this pollination system to allow for inference of evolutionary rates. We sought to address these gaps in knowledge by curating chemical data from 1972 to 2016, integrating them with biosynthetic information, and performing analyses utilizing a phylogenetic framework to answer the following questions:
1) What are the major axes of biochemical variation in floral volatile composition across perfume flowers, and do these patterns align with patterns of pollinator composition?
2) What is the tempo and mode of scent evolution within two independent radiations of perfume flowers?
Methods
Database curation
We built a database of floral perfume chemical composition, as well as pollinator identity, for any angiosperm pollinated by perfume-collecting male euglossine bees. Both published and unpublished data from the literature and collaborators were used to build this database. For the published data, a literature search in ISI Web of Science and Scopus using the following search terms was conducted: (“scent plant” OR “perfume plant” OR “VOC” OR “volatile” OR “scent reward* plant” OR “perfume reward* flower” OR “scent reward* flower” OR “perfume reward* flower”) AND (eugloss* OR “orchid bee”). In addition to this search, we screened the reference list of all obtained articles to check for works not obtained from the literature search. Only studies using headspace analyses were used. We note that in our literature search, floral perfume compounds and pollinators from the same species were sometimes obtained from different sources. However, knowing that floral scent chemistry in perfume flowers is often species specific, we assumed that this would not have biased our results in a substantial manner (Hetherington-Rauth & Ramírez, 2016; Milet-Pinheiro & Gerlach, 2017). Following the compilation of perfume data in a single database, we searched for the CAS number of individual floral perfume compounds in the online database (https://webbook.nist.gov/chemistry/cas-ser.html). Based on the number, we checked for possible synonyms. Compounds included more than once were then merged.
For pollinator taxonomy, we primarily utilized the classification presented by Ramírez et al. (2010). However, we considered all the interactions with Eulaema cingulata as being with Eulaema marcii, as most records under the name of the former come from misuse of this name (Nemésio, 2009). We considered the name Eulaema meriana as inclusive of the recently described Eulaema atleticana for simplicity (Nemésio, 2009). The subspecies luteola and mimetica of the Eulaema seabrai complex were analyzed as separate species, as the subspecific distributions are geographically isolated. Eulaema tropica was considered as a synonym of Eulaema polychroma (Dressler, 1982). The list of literature references for the interactions between plants and pollinators can be found in Supplementary Material.
Data processing
As the studies in the data set spanned a wide range of years (1972–2016), we excluded compounds present in relative proportions below 1% of each species’ perfume to avoid biased sampling of rare compounds with more sensitive technology in recent years. This also allowed us to compare studies that elected to characterize compounds below 1% as “trace” without providing further quantitative information. Species with less than 70% of their total perfume blends resolved were then excluded from the data set. The resulting chemical matrix was then re-standardized such that the sum of relative proportions within each species was 1. We note that our filtering scheme may have affected our ability to understand patterns of variation in minor compounds that act as “private channels” to pollinator communication (Svensson et al., 2011). Additionally, compounds at such low concentrations were found to elicit antennal responses with euglossine pollinators in Catasetum uncatum, a perfume flower in our data set (Milet-Pinheiro et al., 2015). However, our primary objective in this study was to compare across studies to understand broad patterns of chemical variation. As we found no significant relationship between study year and number of samples (range: 1–17, mean = 2.443) on compound richness, proportion of phenylpropanoids, and proportion of terpenoids (Supplementary Figure S1), we consider our filtered data set to be fit for such analysis. Our final data set for all downstream analyses consisted of 167 compounds and 175 plant species.
Due to shared biosynthetic pathways, individual compounds are not independent variables (Junker, 2018). As such, we used the methods of Junker (2018) to account for this nonindependence, scoring each compound for primary metabolic pathway (e.g., terpenoid, shikimate, etc.), subcategorization within each pathway (e.g., monoterpenoid within the terpenoid pathway, phenylpropanoid within the shikimate pathway, etc.), and presence of functional groups as a proxy for shared biosynthesis, creating a “compound x property” matrix. The full scheme of classification is available in Supplementary Table S1. From this compound × property matrix, we then calculated a dissimilarity matrix of all compounds present in the filtered data set. This matrix was then weighted by the species × compound matrix, generating a dissimilarity matrix among all species present in the data set accounting for both compound identity and relative proportions. To evaluate the effect of our scoring scheme, we also used the chemodiv package in R to perform similar analyses of the data, using the “PubChemfingerprint” and “fMCS” methods to generate similar dissimilarity matrices (Petrén et al., 2023). Briefly, the “PubChemfingerprint” method utilizes a similar approach as described above, utilizing 881 binary variables pertaining to compound structure to infer dissimilarities among compound pairs. The “fMCS” (flexible maximum common substructure) method uses an algorithm to identify shared substructures among all pairs of compounds. Principal coordinates analysis (PCoA) was performed on these dissimilarity matrices and correlations of principal coordinates were performed between the different schemes. For the rest of the article, the three schemes will be referred to as the “simple scheme,” “fingerprint scheme,” and “fMCS scheme,” reflecting our use of Junker’s biosynthetically informed distances, and the two chemodiv methods, respectively. These schemes resulted in qualitatively similar results in the first 3 PCos, hence we elected to only investigate these axes for downstream analyses and visualizations (see discussion in Appendix).
As a metric of functional dispersion, we calculated mean pairwise distances (MPDs) of compounds within each species utilizing the distance matrix generated from the “compound x property” matrix as a proxy for relatedness among the different compounds (MPDobserved). We further calculated standard effect sizes (SESs) of these MPDs, using a null distribution of MPDs generated from 999 permutations of the “compound x property” matrix (MPDexpected). SES was calculated using MPDobserved − MPDexpected. Negative values correspond to underdispersion (i.e., overrepresentation of a biosynthetic pathway or branches within that pathway), while positive values correspond to overdispersion (i.e., utilization of multiple or disparate pathways). As we could not calculate functional dispersion for species with only one compound present in their perfumes, we excluded them from analyses utilizing this metric (total of 11 species). These calculations were performed using the picante package in R (Kembel et al., 2010).
We further investigated two groups of monoterpenoid compounds for correlative and phylogenetic comparative analyses. The cineole cassette compounds are a group of 6 monoterpenes (1,8-cineole, sabinene, limonene, α- and β-pinene, and α-terpineol) that have previously been demonstrated to be produced by the multiproduct cineole synthase (CIN) class of monoterpene synthases in several model systems (Fähnrich et al., 2014). Notably, 1,8-cineole is a well-characterized general attractant of male euglossine bees in search of perfumes. We elected to not include myrcene, another frequent product of CINs, in this categorization, as this linear compound is also a frequent product of other terpene synthases (TPSs), including several that produce it as their dominant products (Fähnrich et al., 2014). Additionally, synthesis of myrcene in CINs occurs via an acyclic intermediate cation, while the other ringed products are catalyzed by a cyclic α-terpinyl intermediate. The carvones are another group of ringed monoterpenes (in our data set: carvone, (E)- and (Z)-carvone epoxide, and (E)- and (Z)-dihydrocarvone) that have been characterized as attractants that act primarily to attract bees from the genus Eulaema, although some Euglossa species are also known to be attracted to these compounds. Both 1,8-cineole and the carvones are relatively rare in high abundances within floral volatiles outside of perfume flowers (Raguso et al., 2003; Whitten et al., 1986).
Pollinator analyses
To evaluate the effects of pollinators on scent phenotypes, we performed a regression of the first three axes of variation on bee species richness in addition to bee genera, treating the presence of each genus as a binary factor. Additionally, we performed logistic regression of each PCo axis on pollination by each bee genus to understand the effect of changes in this multivariate space on the probability of pollination. In total, 100 plant species were present in the subset of our filtered data set with both scent and pollinator data available, while 91 bee species were present as pollinators.
We also performed Mantel tests to understand the relationship between pollinator distances and chemical distances. We utilized two metrics of pollinator distances: binary distances based on species and on genera, to test if there were signatures of pollinator-mediated selection at different levels of taxonomic organization. Mantel tests were performed using the vegan package in R, using Spearman correlations and 9,999 permutations (Jari Oksanen et al., 2022).
Phylogenetic comparative methods
Phylogenies
For visualization and analyses of chemical patterns across the broadest scale of taxonomic resolution, we constructed a phylogeny to include as many plant species present in our data set as possible using the V.Phylo2 package in R (Jin & Qian, 2022). Where species in our data set were not present in the megaphylogeny but congeneric/consubgeneric members were, we randomly substituted them for one of these closely related members present in the tree. This was performed for four species (Kegeliella houtteana, Paphinia grandiflora, Solanum circinatum, and Solanum melissarum). Furthermore, as this tree lacked many members of Catasetum, we grafted a time-calibrated phylogeny of this group (Pérez-Escobar et al., 2017a) onto the larger phylogeny, using the gromogram.phylo function in phytools (Revell, 2012). This final tree contained 78 species (Figure 1).
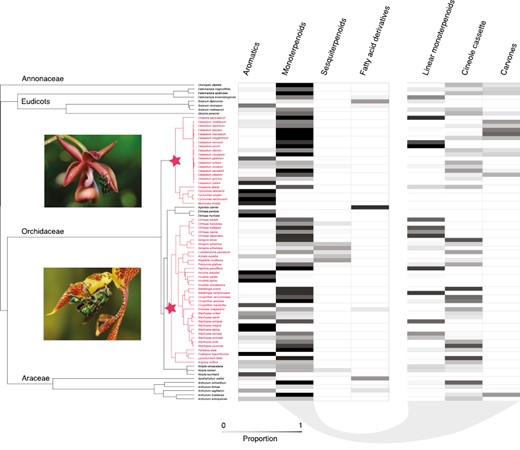
Phylogeny of perfume flowers with heatmap of chemical composition. The block on the right corresponds to three categories of monoterpenoids. Stars on the phylogeny represent the base of our two orchid clades of interest, highlighted in pink: the Catasetinae (above) and the Stanhopeinae (below). Photographs of representative taxa (Mormodes sp. and Gongora sp., respectively) were taken by Thomas Eltz.
As this first tree spanned the breadth of angiosperm evolution, we performed finer scale analyses of two independent radiations of exclusively male euglossine-pollinated orchids: (a) the diverse Stanhopeinae subtribe along with its species-poor sister group the Coeliopsidinae (henceforth referred to as the Stanhopeinae) and (b) the Catasetinae subtribe excluding the early-diverging, species-poor lineage containing Grobya and Cyanaeorchis that include oil bee-pollinated species (henceforth referred to as the Catasetinae). Both groups are of similar age (root age ~20 million years), exhibit similar widespread geographic ranges throughout low- to mid-elevation Neotropical forests, and include similar species richness (~300 for the Stanhopeinae, ~220 for the Catasetinae). Phylogenies for these two clades were made by pruning the megaphylogeny referenced above into two phylogenies of 35 and 22 species, respectively. We further standardized branch lengths to a large, time-calibrated neotropical orchid phylogeny (Pérez-Escobar et al., 2017b), using the congruify.phylo function in the phytools package (Revell, 2012).
Phylogenetic comparative analyses of floral scent
We visualized the phylogenetic structuring of traits using 2D “phylochemospaces,” where we projected phylogenies onto the previously described chemical space using selected axes of the PCoA. These visualizations were made using the phytools package.
To rigorously quantify patterns of chemical evolution, we performed disparity-through-time (dtt) analysis and calculated morphological disparity indices (MDI), which describe within-clade and among-clade variation in traits (Harmon et al., 2003). Positive values of MDI suggest that variation is partitioned within clades, suggesting that closely related species exhibit divergent trait values, while negative values of MDI indicate that variation is partitioned among clades, suggesting that closely related species exhibit similar trait values and that different clades occupy distinct regions of trait space. We calculated MDI for the log-transformed full chemical data set after replacing all 0’s with 0.005, and subsets of this data set that included only aromatic compounds, monoterpenoids, cineole cassette compounds, or carvone compounds—for this last group, we were only able to perform analyses within the Catasetinae as these compounds were not present in the Stanhopeinae. MDI values were compared against a null distribution of 1,000 simulations of each data set using Brownian motion to obtain average values. The significance of this analysis was assessed using the 95% confidence interval generated by the simulations in this null distribution. These analyses were performed using the dtt function in the geiger package (Harmon et al., 2023).
We further calculated the phylogenetic signal using the multivariate generalization of Blomberg’s K (Adams, 2014). Values of “Kmult” range from 0 to infinity, with Kmult = 1 representing the expectation of trait evolution under Brownian motion, and 0 < Kmult < 1 and Kmult > 1 representing data with a lower and higher phylogenetic signal than this null expectation, respectively. We calculated Kmult within the Stanhopeinae and the Catasetinae using the same traits described above for which we performed the dtt analyses. Ten thousand iterations of the data sets were used for the calculations of significance in each test.
Due to the modest sample size and considerable difference in sample sizes between the two groups in the analysis described above, we further randomly rarefied the Stanhopeinae data to 22 species and reran the analyses described above to understand the potential effects of subsampling on our observed results. We randomly subsetted the data 1,000 times, performed dtt analyses and calculated phylogenetic signal in these data subsets, and computed the corresponding test statistics and P-values. All analyses discussed were performed in R version 4.2.1 (R Core Team, 2022).
Results
Chemical composition
Our filtered data set consisted of 167 compounds, of which 92 were terpenoids (49 monoterpenoids, 34 sesquiterpenoids, 5 carotenoids, and 4 irregular terpenoids), 49 were aromatics, and 26 were fatty acid derivatives. The compounds with the highest prevalence across the data set were 1,8-cineole (present in 106 spp.), α-pinene (present in 82 spp.), myrcene (present in 66 spp.), limonene (present in 56 spp.), (E)-β-ocimene (present in 40 spp.), β-pinene (present in 36 spp.), and benzyl acetate (present in 36 spp.; Table 1), all generally ubiquitous floral volatile compounds (Farré-Armengol et al., 2020). Species ranged from having 1–6 compounds (mean = 5.83, SD = 3.18) in their scent profiles. Eleven species were found to exhibit only one compound in their volatile blends, all members of the Orchidaceae, and all exhibiting aromatic products of the shikimate pathway. Of note, two of these species also exhibited only one known compound in their profiles prior to filtering (Cycnoches ventricosum with indole and Gongora claviodora with eugenol).
Most prevalent compounds in the data set (present in above 13% of all species).
Compound name (number of spp present in) . | Chemical class . | Function (inferred from Ramírez & Dressler, 2002) . | Antennal responses (inferred from Brandt et al., 2021) . |
---|---|---|---|
1,8-Cineole (106) | Monoterpene | Attractant | Moderate |
α-pinene (82) | Monoterpene | Modifier | Weak |
Myrcene (66) | Monoterpene | Modifier | Weak |
Limonene (56) | Monoterpene | Modifier | Weak |
(E)-β-ocimene (40) | Monoterpene | Modifier | N/A |
β-pinene (36) | Monoterpene | Modifier | N/A |
Benzyl acetate (36) | Aromatic | Attractant | Strong |
Sabinene (27) | Monoterpene | N/A | N/A |
Methyl salicylate (23) | Aromatic | Attractant | Moderate |
Compound name (number of spp present in) . | Chemical class . | Function (inferred from Ramírez & Dressler, 2002) . | Antennal responses (inferred from Brandt et al., 2021) . |
---|---|---|---|
1,8-Cineole (106) | Monoterpene | Attractant | Moderate |
α-pinene (82) | Monoterpene | Modifier | Weak |
Myrcene (66) | Monoterpene | Modifier | Weak |
Limonene (56) | Monoterpene | Modifier | Weak |
(E)-β-ocimene (40) | Monoterpene | Modifier | N/A |
β-pinene (36) | Monoterpene | Modifier | N/A |
Benzyl acetate (36) | Aromatic | Attractant | Strong |
Sabinene (27) | Monoterpene | N/A | N/A |
Methyl salicylate (23) | Aromatic | Attractant | Moderate |
Most prevalent compounds in the data set (present in above 13% of all species).
Compound name (number of spp present in) . | Chemical class . | Function (inferred from Ramírez & Dressler, 2002) . | Antennal responses (inferred from Brandt et al., 2021) . |
---|---|---|---|
1,8-Cineole (106) | Monoterpene | Attractant | Moderate |
α-pinene (82) | Monoterpene | Modifier | Weak |
Myrcene (66) | Monoterpene | Modifier | Weak |
Limonene (56) | Monoterpene | Modifier | Weak |
(E)-β-ocimene (40) | Monoterpene | Modifier | N/A |
β-pinene (36) | Monoterpene | Modifier | N/A |
Benzyl acetate (36) | Aromatic | Attractant | Strong |
Sabinene (27) | Monoterpene | N/A | N/A |
Methyl salicylate (23) | Aromatic | Attractant | Moderate |
Compound name (number of spp present in) . | Chemical class . | Function (inferred from Ramírez & Dressler, 2002) . | Antennal responses (inferred from Brandt et al., 2021) . |
---|---|---|---|
1,8-Cineole (106) | Monoterpene | Attractant | Moderate |
α-pinene (82) | Monoterpene | Modifier | Weak |
Myrcene (66) | Monoterpene | Modifier | Weak |
Limonene (56) | Monoterpene | Modifier | Weak |
(E)-β-ocimene (40) | Monoterpene | Modifier | N/A |
β-pinene (36) | Monoterpene | Modifier | N/A |
Benzyl acetate (36) | Aromatic | Attractant | Strong |
Sabinene (27) | Monoterpene | N/A | N/A |
Methyl salicylate (23) | Aromatic | Attractant | Moderate |
In addition to being the most abundant compound in our data set with respect to presence, 1,8-cineole was also the dominant compound in 44 species. Other compounds with high dominance included (E)-β-ocimene, 1,4-dimethoxybenzene, benzyl acetate, and (E)-carvone epoxide, present in at least 11 species (Supplementary Figure S2). These compounds have all been demonstrated to be biologically active as they attract male euglossine bees in their pure form, although to date (E)-β ocimene has only been shown to attract Euglossa augaspis and Eg. stilbonata in its pure form (Ramírez & Dressler, 2002; Williams & Dodson, 1972).
Broad patterns of chemical variation
The primary axis of variation in the data set was strongly associated with the primary biosynthetic pathway utilized, where species with high values of PCo1 were terpenoid dominated and species with low values were aromatic dominated (Figure 2). Only around 10% of the data set consisted of species with fatty acid-dominated scent profiles or species with mixed aromatic-terpenoid blends (40%–60% of either biochemical class; Supplementary Table S2). Functional dispersion values of species with more than one compound were largely negative (mean = −2.057, SD = 1.809; only positive in 12 species), suggesting general patterns of chemical underdispersion. Functional dispersion was also negatively associated with PCo1 (F1,162 = 14.17, p < .001), suggesting that plants with aromatic-dominated perfumes produced blends of compounds that were more divergent from each other or utilized different biosynthetic pathways more often than species with terpenoid-dominated perfumes.
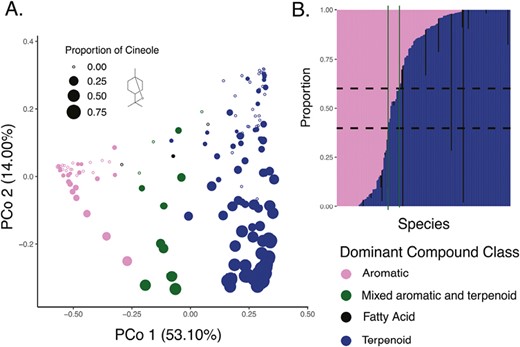
Biosynthetic pathway variation across the full data set. (A) Ordination of the full chemospace of all perfume flowers represented in the data set. Points represent species, sizes correspond to relative concentration of cineole (skeletal structure shown by the legend) within that species, while colors correspond to the dominant biosynthetic pathway represented. (B) Composition of all species with respect to aromatics, fatty acids, and terpenoids. Columns between the green vertical lines represent the species categorized as “mixed aromatic and terpenoid.”
The second axis of variation was found to be strongly driven by the abundances of certain compounds. PCo2 is strongly negatively associated (R = −0.532, p < .001) with 1,8-cineole, a ringed monoterpene that is a well-known generalized attractant of male euglossine bees. This axis was also significantly positively associated with linear monoterpenes (R = 0.357, p < .001), of which myrcene and (E)-β-ocimene were most abundant.
The third axis of variation was found to be driven by concentrations of the ringed carvone monoterpenoid and its derivatives, with a strong negative relationship between PCo3 and relative proportion of these compounds (Supplementary Figure S4; R = −0.571, F1,173 = 163.485, p < .001). This axis was strongly positively associated with linear monoterpenes (R = 0.179, F1,173 = 44.442, p < .001).
Pollinator analyses
The first two PCos were not significantly associated with any group of pollinators. The third axis of variation was significantly negatively associated with Eulaema pollination, but not with other genera (F3,98 = 26.36, p < .001). Using logistic regression, we also found that each increase in 1 unit of PCo3 was associated with a 0.00018-fold reduction in the odds of being Eulaema-pollinated (p < .001). No PCos were significantly associated with the diversity of bee visitors. All schemes of generating compound dissimilarities and chemical distances resulted in weak but marginally nonsignificant to significantly positive relationships with pollinator differences at the species level (Mantel r = .0440, .0372, and .0773; p = .0352, .0896, and .0255 for simple, fingerprint schemes, and fMCS schemes, respectively). Pollinator distances as measured by bee genera resulted in weak but significant positive relationships with the simple and fingerprint schemes (Mantel r = .0447 and .0424, p = .0249 and .0424, respectively), but no significant relationship with the fMCS scheme.
Patterns of scent variation within the Catasetinae and the Stanhopeinae
Broad patterns of scent variation were not strongly structured by phylogeny. In the first data set, we observed that plant families tend to be scattered across chemospace, with several examples of species exhibiting close chemical affinities despite sharing a common ancestor more than a hundred million years ago (Figure 1). As the majority of the plants sampled were in the Orchidaceae, we further investigated patterns within the Stanhopeinae and the Catasetinae. We found that the general broad pattern of aromatic-dominated versus terpenoid-dominated perfume profiles was recapitulated within these independent radiations of male euglossine pollination (Figure 3C and D). Additionally, the second axis of variation was strongly driven by variation between scent profiles dominated by linear versus ringed monoterpenes, with concentrations of 1,8-cineole strongly driving this pattern in both clades.
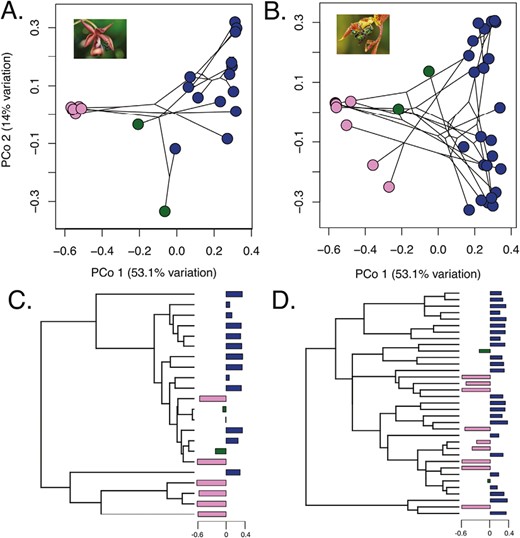
Ordinations of chemical space in two independent radiation of perfume flowers. (A) and (B) show phylochemospaces of the Catasetinae and the Stanhopeinae, respectively, using the same color scheme as in Figure 2. (C) and (D) Phylogenetic relationships within the two groups, while barplots correspond to values of PCo1. Bars are colored according to which chemical class is more highly represented within that species, using the same scheme in Figure 2.
We detected a weak and nonsignificant phylogenetic signal (Kmult = 0.139, p = .180) using the full chemical data set in the Catasetinae and weak and marginally nonsignificant phylogenetic signal in the Stanhopeinae (Kmult = 0.335, p = .0733), suggesting chemical variation was evolutionarily labile in both groups. Even weaker phylogenetic signal was detected in the aromatic compounds within both groups, suggesting that this subset of the entire chemical phenotype was particularly evolutionarily labile (Kmult = 0.0900 and 0.288, p = .851 and .787 for the Catasetinae and Stanhopeinae, respectively). However, the two groups differed with respect to their monoterpene compounds, with the Catasetinae exhibiting a significant phylogenetic signal (Kmult = 0.217, p = .011) and the Stanhopeinae exhibiting no signal (Kmult = 0.341, p = .153). This pattern was likely not driven by cineole cassette compounds, as both groups exhibited no phylogenetic signal in that phenotype (Kmult = 0.119 and 0.333, p = .566 and .212 for the Catasetinae and the Stanhopeinae, respectively). However, the carvones exhibited a significant phylogenetic signal in the Catasetinae (Kmult = 0.331, p = .011), potentially acting as a driver of the differences in phylogenetic signal observed at the broader compound class (monoterpene) level. These results are summarized in Table 2.
Phylogenetic signal of several multidimensional chemical traits. Traits with significant phylogenetic signal at the α = .05 level are bolded, while marginally non-significant traits are italicized.
Trait . | Kmult . | p-value . |
---|---|---|
Full data set Catasetinae | 0.139 | .180 |
Full data set Stanhopeinae | 0.335 | .0733 |
Aromatics Catasetinae | 0.0900 | .851 |
Aromatics Stanhopeinae | 0.288 | .787 |
Monoterpenes Catasetinae | 0.217 | .0106 |
Monoterpenes Stanhopeinae | 0.341 | .153 |
Cineole cassette Catasetinae | 0.119 | .566 |
Cineole cassette Stanhopeinae | 0.333 | .212 |
Linear monoterpenes Catasetinae | 0.229 | .149 |
Linear monoterpenes Stanhopeinae | 0.319 | .428 |
Carvones Catasetinae | 0.331 | .0114 |
Carvones Stanhopeinae | N/A | N/A |
Trait . | Kmult . | p-value . |
---|---|---|
Full data set Catasetinae | 0.139 | .180 |
Full data set Stanhopeinae | 0.335 | .0733 |
Aromatics Catasetinae | 0.0900 | .851 |
Aromatics Stanhopeinae | 0.288 | .787 |
Monoterpenes Catasetinae | 0.217 | .0106 |
Monoterpenes Stanhopeinae | 0.341 | .153 |
Cineole cassette Catasetinae | 0.119 | .566 |
Cineole cassette Stanhopeinae | 0.333 | .212 |
Linear monoterpenes Catasetinae | 0.229 | .149 |
Linear monoterpenes Stanhopeinae | 0.319 | .428 |
Carvones Catasetinae | 0.331 | .0114 |
Carvones Stanhopeinae | N/A | N/A |
Phylogenetic signal of several multidimensional chemical traits. Traits with significant phylogenetic signal at the α = .05 level are bolded, while marginally non-significant traits are italicized.
Trait . | Kmult . | p-value . |
---|---|---|
Full data set Catasetinae | 0.139 | .180 |
Full data set Stanhopeinae | 0.335 | .0733 |
Aromatics Catasetinae | 0.0900 | .851 |
Aromatics Stanhopeinae | 0.288 | .787 |
Monoterpenes Catasetinae | 0.217 | .0106 |
Monoterpenes Stanhopeinae | 0.341 | .153 |
Cineole cassette Catasetinae | 0.119 | .566 |
Cineole cassette Stanhopeinae | 0.333 | .212 |
Linear monoterpenes Catasetinae | 0.229 | .149 |
Linear monoterpenes Stanhopeinae | 0.319 | .428 |
Carvones Catasetinae | 0.331 | .0114 |
Carvones Stanhopeinae | N/A | N/A |
Trait . | Kmult . | p-value . |
---|---|---|
Full data set Catasetinae | 0.139 | .180 |
Full data set Stanhopeinae | 0.335 | .0733 |
Aromatics Catasetinae | 0.0900 | .851 |
Aromatics Stanhopeinae | 0.288 | .787 |
Monoterpenes Catasetinae | 0.217 | .0106 |
Monoterpenes Stanhopeinae | 0.341 | .153 |
Cineole cassette Catasetinae | 0.119 | .566 |
Cineole cassette Stanhopeinae | 0.333 | .212 |
Linear monoterpenes Catasetinae | 0.229 | .149 |
Linear monoterpenes Stanhopeinae | 0.319 | .428 |
Carvones Catasetinae | 0.331 | .0114 |
Carvones Stanhopeinae | N/A | N/A |
We detected significantly positive morphological disparity index (MDI) values using the full data set of compounds within both the Catasetinae and Stanhopeinae (MDI = 0.455 and 0.428, respectively; p = .002 and p < .001, respectively; Figure 4A and F), in addition to in the aromatic compounds (MDI = 0.934 and 0.431, respectively; p < .001 for both; Figure 4B and G), demonstrating that variation is partitioned within clades for these traits and that closely related species exhibit divergent trait values. In the Stanhopeinae, both monoterpenes and cineole cassette compounds exhibited significantly positive MDI values (MDI = 0.425 and 0.480, respectively; p < .001 and p = .001, respectively; Figure 4C and H). Both of these traits exhibited far lower levels of MDI in the Catasetinae (MDI = 0.176 and 0.241, p = .086 and .12, for monoterpenes and cineole cassette compounds, respectively; Figure 4D and I). Furthermore, the MDI of carvone compounds in the Catasetinae was low, and the disparity was not significantly different from the null distribution generated by Brownian motion (MDI = 0.0614, p = .422; Figure 4E). We note that the two groups did not differ significantly with respect to richness, proportion of monoterpenes, proportion of aromatics, or proportion of cineole cassette compounds, so different results in our multivariate analyses are not likely due to strong differences in trait dimensionality between clades.
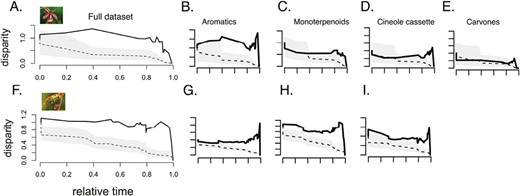
Disparity-through-time (dtt) plots of floral volatile traits in the Catasetinae (top row) and the Stanhopeinae (bottom row). (A) and (F) show dtt of the log-transformed full chemical data set, (B) and (G) show dtt of aromatic compounds, (C) and (H) show dtt of monoterpenoids, (D) and (I) show dtt plots of cineole cassette compounds, and (E) shows dtt of carvone compounds (present only in the Catasetinae). In all figures, solid black lines correspond to observed disparity, while the dotted lines represent median values from 1,000 simulations of the data using Brownian motion. The gray area corresponds to the 95% confidence intervals from the simulated null distribution.
We find that the differences in patterns observed between the Stanhopeinae and the Catasetinae were independent of the larger sample size in the former clade. In our rarefactions of the Stanhopeinae data set to 22 species, we detected no significant differences in patterns compared to analyses performed using the full data set of 35 species in both Kmult and dtt (Supplementary Figures S7 and S8). While the outcomes of the simulations in the Kmult analysis tended to have higher Kmult values than what we observed in our data set, suggesting a stronger phylogenetic signal, p-values on average were all well above 0.1 (0.207–0.686) and the observed Kmult values were within the central 95% of simulation values (Supplementary Figure S8).
Discussion
Olfactory preferences evolve rapidly in euglossine bees, and divergence in the choice of exogenous perfumes collected from the environment is a key component in the process of speciation (Weber et al., 2016). We predicted that, as a result, plants that are obligately pollinated by these bees using scent compounds as rewards would also exhibit patterns of rapid chemical evolution. We find signatures of this rapid evolution across two independent radiations of perfume flower orchids, with radically different chemical phenotypes present in floral volatiles, even among closely related taxa. We also find pervasive chemical convergence, with many species across our data set exhibiting perfumes that contain high proportions of 1,8-cineole, a compound that is highly attractive to many species of male euglossine bees.
The primary axis of this variation across male euglossine-pollinated floral volatiles is driven by differences between terpenoid and aromatic-dominated perfumes (Figure 2). This general pattern was consistent in independent radiations of male euglossine pollination within the Catasetinae and the Stanhopeinae, and both clades additionally exhibited relatively few intermediate phenotypes or those that utilize other pathways (Figure 3). Due to the unique role of these floral signals as constituents of courtship displays for the pollinators themselves, the utilization of multiple biosynthetic pathways may have been generated due to the rapid evolution of olfactory biases present in euglossine bees. Both the terpene and aromatic biosynthesis pathways are present across flowering plants, and in addition to their importance in generating floral volatiles, they are also necessary for the production of primary metabolites (Vogt, 2010). Thus, divergence in biochemical pathways underlying scent production that cause reproductive isolation is more likely to be driven by changes in gene regulation, rather than through deeper modifications of biosynthetic architecture that may result in widespread negative pleiotropy (Boersma et al., 2022; Li et al., 2021; Verdonk et al., 2005). Major variation in perfumes driven primarily by transcriptomic differences may facilitate rapid divergences in phenotype among closely related taxa that we observed in our data set, spurring the process of reproductive isolation and speciation.
Despite an abundance of aromatic- and terpenoid-producing flowers, we observed a striking lack of species where these two compound classes were produced in similar proportions. This presence of only a few mixed profiles is difficult to explain, as the perfumes present in male bees tend to be diverse, generated from multiple chemical classes (Weber et al., 2016). Additionally, many euglossine bee species exhibit strong antennal responses to compounds in both chemical groups (Brandt et al., 2021; Milet-Pinheiro et al., 2015). While we lack sufficient data to fully understand the adaptive role of mixed perfumes, we note that Catasetum luridum emits primarily 1,8-cineole and 1,4-dimethoxybenzene, two compounds that are both attractive in their pure form and elicit moderate to strong responses in the antennae of Euglossa carolina (syn. Eg. cordata), one of its pollinators, suggesting multifunctional capacities of these perfume blends (Brandt et al., 2021). While physiological constraints due to tradeoffs in chemical production could also contribute to the lack of intermediate phenotypes, flowering plants often express volatiles consisting of compounds from multiple biosynthetic pathways (Junker et al., 2018). Manipulative studies on the combinatorial effects of different compounds on male euglossine attraction and perfume-collecting behavior are needed to better understand and attempt to explain these patterns.
Odorant receptors (ORs) in insects exhibit a broad range of specificity, but many are narrowly tuned to certain compounds or compound classes (Conchou et al., 2019). For example, in lepidopterans, the OR42 receptor is narrowly tuned to phenylacetaldehyde, a common aromatic compound found in floral volatiles (Guo et al., 2021). This receptor is highly conserved across the order, and all functionally tested orthologs showed the same narrow tuning to that compound (Guo et al., 2021). Producing compounds that target a single OR may allow for a more predictable set of behavioral responses, facilitating pollination efficiency. In euglossine bees, OR genes exhibit signatures of rapid molecular evolution, consistent with their role in sexual selection (Brand et al., 2015). However, thus far, the function of only one gene has been rigorously tested, with narrow tuning to a compound not present in our data set, the aromatic 2-hydroxy-6-nona-1,3-dienyl-benzaldehyde (Brand et al., 2020). Knowledge of how other euglossine bee ORs function and their specificity to floral scent compounds would provide valuable insight into the functional consequences of biochemical divergence in perfume flowers.
While the first axis of chemical variation was driven by differences in biosynthetic pathways, we observed substantial differences among terpenoid-dominated species that drove variation along the second and third axes (Figure 2A). Terpenoids are a highly diverse class of natural compounds, with over 20,000 structures identified in plants. Key to their diversification is the terpene synthase (TPS) family of enzymes that catalyze the final step in an otherwise deeply conserved pathway. TPSs are known to be chemically promiscuous, often producing multiple products per enzyme, while further functional modifications can be mediated by other enzymes, particularly those of the Cytochrome p450 family. Additionally, small changes in TPS sequence have been demonstrated to result in divergent products in mutagenesis experiments (Srividya et al., 2015). Together, these properties may explain the greater amount of chemospace occupied by terpenoid-dominated species than aromatic-dominated species, and the general pattern of terpenes driving overall patterns of diversity within the data set (Karunanithi & Zerbe, 2019; Pichersky & Raguso, 2018).
We find that monoterpene variation drove observed patterns in the second and third major axes of variation. Both axes were characterized by opposing gradients of linear compounds versus ringed compounds (1,8-cineole and carvones for the second and third axes, respectively). 1,8-Cineole is a well-known generalized attractant, and in its pure form, attracts diverse assemblages of male bees. Carvones are less generalized in their biological function, being associated with the attraction of bees from the genus Eulaema, in addition to a few members of Euglossa. Linear monoterpenoids tend to be further specialized in nature, attracting few to no bee species on their own and generally eliciting weak antennal responses (Brandt et al., 2021). While these axes of variation appear to represent gradients in pollinator specificity, spanning the range of high abundance in generally weakly-attractive compounds to the high abundance of more attractive compounds, the pollinator data do not support this interpretation, with a lack of significant correlations with bee species richness or visitation by bee genera in the first two axes of variation. However, PCo3 exhibits a strong negative correlation with the presence of Eulaema pollination, suggesting a signature of pollinator-mediated selection on this major axis of variation driven by carvones.
The general patterns of gradients between linear and ringed monoterpenes observed in the second and third axes of variation could be caused by biosynthetic tradeoffs. Monoterpene synthases catalyze a precursor compound into a series of cationic intermediates, the first of which is linear and can be catalyzed into linear products in our data set such as myrcene and ipsdienol. When this cation is closed, it becomes a precursor intermediate to ringed products, such as members of the cineole cassette or carvones (Huang et al., 2021). Thus, a potential tradeoff could be created by this divergence within a biosynthetic pathway, where movement through chemospace is constrained by which path in synthesis that precursors are shunted towards, as determined via TPS function (Supplementary Figure S9). As TPS evolution is rapid and many terpenes are the product of multiple TPSs, functional validation of these enzymes across male euglossine-pollinated plants is required to rigorously test this hypothesis.
Across the full chemical data set, we observe low phylogenetic signal and high MDI in both the Stanhopeinae and the Catasetinae, suggesting that this multidimensional trait exhibits patterns of rapid divergence among closely related species in addition to the sharing of phenotypic space across their evolutionary histories (Figure 4). This is consistent with the putative role of scent in the process of speciation across these plants, as shifts in floral chemistry can result in the attraction of different pollinator species, resulting in reproductive isolation. High MDI and low phylogenetic signal are mirrored in a similar analysis characterizing perfume variation across the genus Euglossa, attributed by the authors to rapid evolution due to sexual selection (Weber et al., 2016). The macroevolutionary patterns of chemical disparity observed here in the plants pollinated by these bees and their close relatives may reflect this rapid diversification in olfactory preferences.
At a finer level of chemical variation, aromatic compounds exhibited a similar pattern to that of the full chemical data set, with high MDI and low phylogenetic signal in both clades. We found that aromatic-dominated species in our phylochemospaces tended to be most closely related to species that were not aromatic-dominated (Figures 1 and 2B and C). In an electroantennographic study characterizing responses across 26 euglossine bee species, six of nine of the aromatic compounds tested generated generally strong responses, suggesting a strong sensory bias for this chemical class and potentially major effects on pollinator visitation through shifts to utilization of this biosynthetic pathway (Brandt et al., 2021). This pattern of rapid evolution in specific compounds mirrors a study of the Pseudophrys section of sexually deceptive Ophrys (Orchidaceae), where alkenes and alkadienes that are key in mediating pollinator shifts exhibited similarly high evolutionary lability (Ayasse et al., 2011; Joffard et al., 2020). The contrasting patterns between the two clades in monoterpenoid evolution, with low MDI and significant phylogenetic signal detected only in the Catasetinae, suggest that stronger constraints to chemical evolution may exist within this clade (Figure 4C and H). This pattern may be driven in part by the carvones that, among our sampled orchid species, are exclusively present within this clade (Figure 4E). As the carvones exhibit more specific functions than generalized attractants such as 1,8-cineole, being associated with visitation by Eulaema bees, stabilizing selection may be occurring due to specialization for a chemical niche within the Catasetinae (Milet-Pinheiro & Gerlach, 2017).
While ecologically similar with respect to pollination, the Stanhopeinae and Catasetinae differ with respect to the scale at which mechanical isolation operates, potentially driving differences in scent evolution. Within the Stanhopeinae, mechanical isolation generally operates at the genus level, with congeners typically depositing their pollen structures on the same body parts of visiting bees. Thus, closely related species within this clade may be expected to exhibit highly divergent scent profiles, as has been confirmed in the genera Gongora, Stanhopea, and Coryanthes, to avoid potential costly hybridization (Gerlach & Schill, 1991; Hetherington-Rauth & Ramírez, 2016). Members of the Catasetinae, however, exhibit more rapid evolution of mechanical isolation, with congeners often exhibiting different placement positions of their pollen structures on bees, perhaps allowing for more pollinator sharing and thus more similar patterns of chemical variation among related taxa (Hills et al., 1972).
Conclusions
Our results suggest two primary modes of scent diversification within perfume flowers—either along the axis of aromatic to terpenoid variation or via modulating relative proportions of terpenoids, in particular the cineole cassette and carvone compounds. We find evidence for pervasive convergence in several regions of this chemical space across distant clades. However, we also observe the rapid evolution of divergent chemistries among closely related taxa. While broad patterns of chemical evolution are shared between two independent radiations of plants utilizing this pollination system, we find differences in more subtle patterns among the clades, indicating lineage-specific effects of pollinator-mediated selection at the macroevolutionary level, potentially due to differences in the relative roles of mechanical isolation. Our study provides the first phylogenetically informed and biosynthetically explicit view of scent evolution across the radiations of a unique pollination system that has captured the attention of both horticulturists and evolutionary biologists alike for over a century.
Data availability
Data and R scripts used for analysis have been archived at Dryad under https://doi.org/10.25338/B85938.
Author contributions
J.W.L., P.M.-.P, C.E.P., and S.R.R. conceived of the project. P.M.-P. and C.E.P. built the database, with input from G.G., M.A., and I.A.S. J.W.L. analyzed the data and wrote the first draft of the manuscript. All authors commented on the draft.
Funding
Grants were provided to JWL (National Science Foundation Graduate Research Fellowship under Grant No. 1650042), CEP (São Paulo Research Foundation; FAPESP Postdoc Proc. N. 2017/22642-5), and PM-P (Conselho Nacional de Desenvolvimento Científico e Tecnológico: CNPq/PQ Proc. N. 313948/2021-6). SRR received support from the David and Lucile Packard Foundation, MA from the Deutsche Forschungsgemeinschaft (AY 12/12-1) and PM-P from Conselho Nacional de Desernvolvimento Científico e Tecnológico (CNPq/Universal Proc. N. 422647/2021-7).
Conflict of interest: The authors have no conflicts of interest to declare. Editorial processing of the manuscript was done independently of SRR, who is an associate editor of Evolution.
Acknowledgments
We are grateful to Kathleen M. Kay, Peter C. Wainwright, Salvatore Cozzolino, and members of the Ramírez Lab (Kathy Darragh, Marissa C. Sandoval, Denise Y. Ramirez, Seira A. Adams) for providing helpful feedback on this manuscript. We are also grateful to Nelly Bautista, who helped curate the database for chemodiv analyses. We thank Thomas Eltz for the use of his photographs in our figures.
Appendix
Consistency among schemes of generating chemical dissimilarities
The three schemes of generating dissimilarity matrices produced qualitatively similar results in the first few axes of variation apart from some reflections about the origin of PCo scores (Supplementary Figures S3–S6). All pairs of PCo’s were highly correlated on the first axis (all R2 > 0.75, p < .001; Supplementary Figure S3A), and PCo’s 2 and 3 were still strongly correlated with each other between the simple scheme and the fingerprint scheme (R2 all > 0.75, p < .001; Supplementary Figure S3A). Despite a low explanatory relationship between fMCS and the other schemes in the second and third axes (0.11 < R2 < 0.2), the relationships were still highly significant (p < .001; Supplementary Figure S3B and C). In the fourth axis and beyond, relationships among schemes began to drop off substantially (Supplementary Figure S3D). We thus elected to primarily investigate the first three PCo axes for downstream correlative analyses and visualization of broad patterns of biochemical variation. The significance of patterns shown at the α = 0.05 level was generally concordant among schemes (Supplementary Figures S3A–C, S4, and S5; Directionality of relationships may be different due to reflections about the origin exhibited by the different schemes). We hence reported only the results of the simple scheme in figures and test statistics unless deviations at the significance threshold occurred.