-
PDF
- Split View
-
Views
-
Cite
Cite
Andreas Goette, Domenico Corradi, Dobromir Dobrev, Luis Aguinaga, Jose-Angel Cabrera, Sumeet S Chugh, Joris R de Groot, Laurie Soulat-Dufour, Guilherme Fenelon, Stephane N Hatem, Jose Jalife, Yenn-Jiang Lin, Gregory Y H Lip, Gregory M Marcus, Katherine T Murray, Hui-Nam Pak, Ulrich Schotten, Naohiko Takahashi, Takanori Yamaguchi, William A Zoghbi, Stanley Nattel, Lluis Mont, Joseph G Akar, Nazem Akoum, Till Althoff, Juan Carlos Diaz, Jean-Baptiste Guichard, Amir Jadidi, Jonathan Kalman, Han Lim, Ricardo Alkmim Teixeira, Atrial cardiomyopathy revisited—evolution of a concept: a clinical consensus statement of the European Heart Rhythm Association (EHRA) of the ESC, the Heart Rhythm Society (HRS), the Asian Pacific Heart Rhythm Society (APHRS), and the Latin American Heart Rhythm Society (LAHRS), EP Europace, Volume 26, Issue 9, September 2024, euae204, https://doi.org/10.1093/europace/euae204
- Share Icon Share
Abstract
The concept of “atrial cardiomyopathy” (AtCM) had been percolating through the literature since its first mention in 1972. Since then, publications using the term were sporadic until the decision was made to convene an expert working group with representation from four multinational arrhythmia organizations to prepare a consensus document on atrial cardiomyopathy in 2016 (EHRA/HRS/APHRS/SOLAECE expert consensus on atrial cardiomyopathies: definition, characterization, and clinical implication). Subsequently, publications on AtCM have increased progressively.
The present consensus document elaborates the 2016 AtCM document further to implement a simple AtCM staging system (AtCM stages 1–3) by integrating biomarkers, atrial geometry, and electrophysiological changes. However, the proposed AtCM staging needs clinical validation. Importantly, it is clearly stated that the presence of AtCM might serve as a substrate for the development of atrial fibrillation (AF) and AF may accelerates AtCM substantially, but AtCM per se needs to be viewed as a separate entity.
Thus, the present document serves as a clinical consensus statement of the European Heart Rhythm Association (EHRA) of the ESC, the Heart Rhythm Society (HRS), the Asian Pacific Heart Rhythm Society (APHRS), and the Latin American Heart Rhythm Society (LAHRS) to contribute to the evolution of the AtCM concept.
Table of contents
1. Background, definition and classification
2. Important recent basic mechanistic insights
2.1. Early-onset atrial fibrillation due to underlying genetic cardiomyopathy and arrhythmia syndromes
2.2. Atrial amyloidosis
2.3. Inflammatory signalling
2.4. Gut microbiome
2.5. Reactive lipid mediators of oxidative stress
2.6. Atrial adipose tissue and fibro-fatty infiltration
3. New findings in the pathophysiology of atrial cardiomyopathy
3.1. Ageing and atrial cardiomyopathy
3.2. Gender and atrial cardiomyopathy
3.3. Atrial failure: definitions, causes, and consequences
3.4. Atrial functional valvular regurgitation
3.5. Metabolic disorders and atrial cardiomyopathy
3.6. Atrial cardiomyopathy and atrial ablation
3.7. The development of atrial fibrosis
4. Imaging advances
4.1. Atrial imaging tools in the evaluation of atrial cardiomyopathy
4.2. Atrial size and function
4.3. LA late gadolinium-enhanced cardiac magnetic resonance imaging
4.4. Electroanatomic mapping
4.5. Prediction of incident atrial fibrillation by cardiac imaging
4.6. Recurrent atrial fibrillation
4.7. Reverse atrial remodelling and atrial remodelling
4.8. Risk stratification
5. Biomarkers, omics, ECG parameters
5.1. Biomarkers
5.2. Blood-based biomarkers
5.3. Limitations of biomarkers
5.4. Multi-omics and atrial cardiomyopathy
5.5. ECG parameters
5.6. Artificial intelligence
5.7. Future directions
6. Stroke and cognitive decline
6.1. Empiric evidence that atrial cardiomyopathy leads to stroke
6.2. Mechanisms underlying the relationship between atrial cardiomyopathy and stroke
6.3. Does atrial cardiomyopathy lead to cognitive decline?
7. Therapeutic implications
7.1. Primary prevention of atrial cardiomyopathy
7.2. Lifestyle interventions to reverse atrial cardiomyopathy
7.3. Pharmacological therapies to reduce progression of atrial cardiomyopathy
7.4. Impact of rhythm control strategies on atrial cardiomyopathy
7.5. Rhythm control and atrial cardiomyopathy
7.6. Reversal of atrial cardiomyopathy by rhythm control?
7.7. Device therapy to reverse atrial cardiomyopathy
8. Conclusions and future directions
Supplementary material
Acknowledgements
Data availability
1. Background, definition and classification
The concept of ‘atrial cardiomyopathy’ (AtCM) had been percolating through the literature since its first mention in 1972.1 Since then, publications using the term were sporadic until the decision was made to convene an expert working group with representation from four multinational arrhythmia organizations to prepare a consensus document on atrial cardiomyopathy (EHRA/HRS/APHRS/SOLAECE expert consensus on atrial cardiomyopathies: definition, characterization, and clinical implication).2 Subsequent to the publication of the working group report,2–4 the number of publications on AtCM has increased progressively (Figure 1). In 2022, progress in the area motivated the decision to reconstitute the working group in order to take stock of a range of important developments. The present manuscript presents the results of that reflection.

The colour shading is pretty but could be confusing, if readers think the red colour designates something different from the blue. Consider keeping simple monochrome (which avoids this potential confusion) or else adding a note to the figure legend indicating that each vertical bar represents the number of publications each year, with the colours simply being decorative.2–4
In the first consensus document on AtCM, the working group recommended the following working definition of atrial cardiomyopathy: ‘Any complex of structural, architectural, contractile, or electrophysiological changes affecting the atria with the potential to produce clinically relevant manifestations’ (Table 1). This definition is of necessity broad, as AtCM is the basis for clinical manifestations of atrial arrhythmia (e.g. atrial fibrillation (AF) and atrial flutter), atrial thrombogenesis and clinically important functional–structural manifestations like atrial failure and atrio-ventricular valve dysfunction secondary to atrial dilation.
‘Any complex of structural, architectural, contractile or electrophysiological changes affecting the atria with the potential to produce clinically relevant manifestations.’ |
‘Any complex of structural, architectural, contractile or electrophysiological changes affecting the atria with the potential to produce clinically relevant manifestations.’ |
‘Any complex of structural, architectural, contractile or electrophysiological changes affecting the atria with the potential to produce clinically relevant manifestations.’ |
‘Any complex of structural, architectural, contractile or electrophysiological changes affecting the atria with the potential to produce clinically relevant manifestations.’ |
Accordingly, AtCM encompasses all functionally relevant complexes of atrial tissue changes, including those in contractility, electrophysiology and chamber architecture. Several systemic diseases or disease states can influence overall atrial function, causing pathophysiological changes and overt pathology. We also proposed a histological classification scheme for atrial cardiomyopathies in the consensus document, with the acronym EHRAS (for EHRA/HRS/APHRS/SOLAECE), defining four classes: I (principally cardiomyocyte changes); II (principally fibrotic changes); III (combined cardiomyocyte-pathology/fibrosis); IV primarily non-collagen infiltration (with or without cardiomyocyte changes). This simple classification might help to convey the primary underlying atrial pathology in various clinical conditions; however, it has not, to date, been widely accepted.5–7 A reason for this current situation might be that systematic analyses of atrial biopsies is not feasible in vivo, and therefore, histological validation of clinical findings/atrial imaging is rarely done.
Members of the working group considered that Table 2 might present a simple working system for staging AtCM by integrating biomarkers, atrial geometry, and electrophysiological changes to define different stages of AtCM. Importantly, AtCM has to be differentiated from AF, which might be a consequence of AtCM: AtCM commonly causes AF, however, AF may also accelerate AtCM. To concretize the suggested AtCM staging system, prospective trials are needed to validate the stages and cut-off values.
1.Mild (subclinical) atrial cardiomyopathy | Detected by electrophysiological measures or imaging technologies without overt arrythmia or significant mechanical atrial dysfunction |
2. Moderate (clinically manifest) atrial cardiomyopathy | Significant structural abnormalities or atrial mechanical dysfunction and elevated biomarkers (BNP, ANP, etc.) and /or manifestation of atrial fibrillation |
3. Severe atrial cardiomyopathy: | Atrial systolic failure (severely reduced left atrial ejection fraction ≤35%) associated with substantially impaired atrial contractility (flow velocities: ≤20 cm/sec within LAA/tissue strain), and/or major degrees (≥35% of LA wall volume) of interstitial alterations (atrial fibrosis, fatty infiltrates, amyloid infiltration, inflammation), and/or severe atrial enlargement (LA diameter ≥5.0 cm, LA volume index ≥50 mL/m2), and/or long-standing persistent/permanent atrial fibrillation |
1.Mild (subclinical) atrial cardiomyopathy | Detected by electrophysiological measures or imaging technologies without overt arrythmia or significant mechanical atrial dysfunction |
2. Moderate (clinically manifest) atrial cardiomyopathy | Significant structural abnormalities or atrial mechanical dysfunction and elevated biomarkers (BNP, ANP, etc.) and /or manifestation of atrial fibrillation |
3. Severe atrial cardiomyopathy: | Atrial systolic failure (severely reduced left atrial ejection fraction ≤35%) associated with substantially impaired atrial contractility (flow velocities: ≤20 cm/sec within LAA/tissue strain), and/or major degrees (≥35% of LA wall volume) of interstitial alterations (atrial fibrosis, fatty infiltrates, amyloid infiltration, inflammation), and/or severe atrial enlargement (LA diameter ≥5.0 cm, LA volume index ≥50 mL/m2), and/or long-standing persistent/permanent atrial fibrillation |
Functional measurements (LAEF etc.) in sinus rhythm; validation of suggested AtCM stages and cut-off values need to be done in prospective trials.
LA, left atrium; LAA, left atrial appendage.
1.Mild (subclinical) atrial cardiomyopathy | Detected by electrophysiological measures or imaging technologies without overt arrythmia or significant mechanical atrial dysfunction |
2. Moderate (clinically manifest) atrial cardiomyopathy | Significant structural abnormalities or atrial mechanical dysfunction and elevated biomarkers (BNP, ANP, etc.) and /or manifestation of atrial fibrillation |
3. Severe atrial cardiomyopathy: | Atrial systolic failure (severely reduced left atrial ejection fraction ≤35%) associated with substantially impaired atrial contractility (flow velocities: ≤20 cm/sec within LAA/tissue strain), and/or major degrees (≥35% of LA wall volume) of interstitial alterations (atrial fibrosis, fatty infiltrates, amyloid infiltration, inflammation), and/or severe atrial enlargement (LA diameter ≥5.0 cm, LA volume index ≥50 mL/m2), and/or long-standing persistent/permanent atrial fibrillation |
1.Mild (subclinical) atrial cardiomyopathy | Detected by electrophysiological measures or imaging technologies without overt arrythmia or significant mechanical atrial dysfunction |
2. Moderate (clinically manifest) atrial cardiomyopathy | Significant structural abnormalities or atrial mechanical dysfunction and elevated biomarkers (BNP, ANP, etc.) and /or manifestation of atrial fibrillation |
3. Severe atrial cardiomyopathy: | Atrial systolic failure (severely reduced left atrial ejection fraction ≤35%) associated with substantially impaired atrial contractility (flow velocities: ≤20 cm/sec within LAA/tissue strain), and/or major degrees (≥35% of LA wall volume) of interstitial alterations (atrial fibrosis, fatty infiltrates, amyloid infiltration, inflammation), and/or severe atrial enlargement (LA diameter ≥5.0 cm, LA volume index ≥50 mL/m2), and/or long-standing persistent/permanent atrial fibrillation |
Functional measurements (LAEF etc.) in sinus rhythm; validation of suggested AtCM stages and cut-off values need to be done in prospective trials.
LA, left atrium; LAA, left atrial appendage.
2. Important recent basic mechanistic insights
2.1. Early-onset atrial fibrillation due to underlying genetic cardiomyopathy and arrhythmia syndromes
Recent evidence indicates that AF developing in younger patients is often the initial manifestation of an atrial cardiomyopathy caused by rare variants in cardiomyopathy (CM) or arrhythmia syndrome genes.8 In one study, patients with AF-onset at ages ≤65 years underwent whole-exome sequencing for 145 genes commonly included on commercial cardiomyopathy and arrhythmia syndrome panels including dilated cardiomyopathy, hypertrophic cardiomyopathy, Brugada syndromes and other inherited arrhythmia syndromes.9 In a cohort of 1293 patients with a median age of 50 years, 10.1% had a disease-causing variant, with 62.8% carrying a variant of unknown significance (VUS), and 7.1% heterozygous for an autosomal recessive disease. Patients with AF-onset at <30 years of age are most likely to have a disease-associated variant (16.8%), with the most common genes being TTN, followed by MYH7, MYH6, LMNA, and KCNQ1. During a median follow-up of 9.9 years in the same cohort, the presence of a disease-causing variant was associated with a 50% increase in mortality that included 73 deaths related to cardiomyopathy, 40 sudden deaths, and 10 stroke-related deaths.10 Thus, patients with early-onset AF have a significant risk of a disease-associated variant, primarily in cardiomyopathy genes, with increased mortality, supporting a potential role for genetic testing in these patients. However, while there are data pointing towards the potential value of genetic testing for AtCM, no major society guidelines presently recommend such testing, and it is not covered by most health insurers.11 Further work in this promising area is certainly needed. A systematic multicenter registry of atrial-specific cardiomyopathies is a very-much needed first step.12
2.2. Atrial amyloidosis
Increasing evidence indicates that the prevalence of atrial amyloidosis is greater than previously suspected, particularly in the elderly.13–16 The prevalence of heart failure with a preserved ejection fraction (HFpEF) increases with ageing, and senile amyloidosis due to wild-type transthyretin (ATTRwt) accounts for up to 25% of HFpEF in patients >80 years of age, commonly with atrial involvement and AF.13,16 In a recent study of HFpEF patients aged >60 years with LV hypertrophy,99m Tc-DPD scintigraphy was highly suggestive of ATTRwt in 13% of patients.14 Isolated atrial amyloidosis (IAA) is a different entity mediated by atrial natriuretic peptide (ANP), and deposits containing both TTR and ANP have been detected in the same atria, indicating that both forms of senile atrial amyloidosis can co-exist.15 Although some forms of amyloid were previously considered organ specific [e.g. amyloid β (Aβ) in Alzheimer's disease], cardiac involvement with Aβ deposition and diastolic dysfunction has been identified in patients with Alzheimer's disease.17 Mechanistically, there is increasing evidence for the cardiotoxicity of soluble pre-amyloid oligomers. Light-chain variable domain proteins from cardiac light-chain amyloidosis (AL) patients are highly toxic to atrial HL-1 cardiomyocytes,18 while natriuretic peptide oligomers cause mitochondrial dysfunction, superoxide generation, and electrophysiological alterations in atrial cells.19
2.3. Inflammatory signalling
Recent findings showed that atrial NLRP3-inflammasome signalling creates AF-promoting AtCM in mouse models, and is increased in patients with common forms of AF including paroxysmal, persistent, and post-operative AF20,21 as well as in conditions that promote AF (obesity,22diabetes,22 gut dysbiosis,23 sepsis,24 and HFpEF25–27). Expression (‘priming’) and assembly (‘triggering’) of the NLRP3-inflammasome complex consisting of NLRP3, apoptosis-associated speck-like protein containing a C-terminal caspase activation and recruitment domain (ASC) and pro-caspase-1 accelerates auto-cleavage of pro-caspase-1 in atrial and immune cells. The resulting increase in active caspase-1 cleaves the inactive precursors of IL-1β and IL-18 to their active forms. In addition, caspase-1 degrades gasdermin-D, releasing the N-terminal fragment that forms plasma membrane pores, which allow IL-1β and IL-18 to leave the cells to exert autocrine and paracrine effects.25,26 Mice with cardiomyocyte-restricted constitutive activation of the NLRP3 inflammasome show increased atrial ectopy associated with abnormal Ca2+-handling due to increased RyR2 expression, as well as reentry-promoting APD shortening (electrical remodelling), likely due to upregulation of atrial-selective ultra-rapid delayed-rectifier (IKur) and acetylcholine-activated inward-rectifier (IK,ACh) K+-current, as well as structural remodelling involving hypertrophy and fibrosis.20 The activation of the NLRP3 inflammasome is likely mediated by increased activity of CaMKII-related pathways21 and impaired AMPK signalling.27 Gut microbiota-derived lipopolysaccharide may constitute a biologically relevant upstream activator of the atrial NLRP3 inflammasome in AtCM, particularly during ageing.23 Recent data have linked another inflammasome, the Absent-in-Melanoma 2 (AIM2) inflammasome, to AtCM, and AF susceptibility.28 This study showed that high-protein diets create AF-promoting AtCM by activating the AIM2-inflammasome, which is associated with mitochondrial oxidative stress along with proarrhythmic sarcoplasmic reticulum Ca2+-release events.28 These findings position the NLRP3 and AIM2 inflammasomes as potential novel drug targets for AF treatment.
Recent data point to an important role of recruited macrophages in the development of inflammatory signalling leading to AtCM and AF,29 with macrophage targets being of potential interest as novel AF therapeutic compounds.30 There is also evidence that genetic and epigenetic regulation of innate immunity may play a major role in conditioning AF susceptibility by controlling inflammatory responses.31 Active resolution of inflammation is emerging as a theme in combatting inflammation and preventing its chronicization.26 Pro-resolution mediators like resolvin D1 have shown effectiveness in prevention of AF substrate development in animal models of both right32 and left heart disease.32,33 Nevertheless, specific differences between various types of inflammation, like sterile inflammatory processes or viral/bacterial infections, cannot be made at present.
2.4. Gut microbiome
The gut microbiota is a dynamic system containing microorganisms that produce bioactive metabolites, the type and quality of which depend on lifestyle-related factors (e.g. diet, exercise), among others. This microbiota contributes to the pathophysiology of many cardiovascular conditions like heart failure, hypertension, obesity, and coronary artery disease, and there is accumulating evidence for a role of gut microbiota in AtCM and related arrhythmogenesis.34 Although many gut microbiota-derived metabolites could play a role in atrial arrhythmogenesis,34 recent evidence suggests that gut dysbiosis may promote AtCM and AF partly through increased levels of circulating lipopolysaccharide and glucose, along with enhanced activity of the atrial NLRP3 inflammasome, which causes atrial fibrosis.23 As a proof of causality, faecal microbiota transplantation (FMT) from young (2–3 months) to old (22–24 months) rats reduced atrial fibrosis and the age-related increase in AF susceptibility by attenuating atrial NLRP3-inflammasome activity. Selective inhibition of both lipopolysaccharide and the NLRP3 inflammasome also reduced AF susceptibility and atrial fibrosis, suggesting lipopolysaccharide as a key upstream driver of the atrial NLRP3 inflammasome and the associated arrhythmogenesis.25,26 Although gut microbiota could create inflammatory AtCM, the precise cellular and molecular mechanisms, as well as the clinical contexts in which this system is active in AtCM, require further investigation.
2.5. Reactive lipid mediators of oxidative stress
During inflammation and oxidative stress, lipid peroxidation generates dicarbonyl compounds that adduct to proteins, DNA, and other lipids to promote molecular dysfunction and amyloid formation.35 The most reactive dicarbonyls are isolevuglandins (IsoLGs),36 and small molecule scavengers that selectively inactivate these and other lipid dicarbonyls are beneficial in multiple preclinical studies, indicating that IsoLGs are a major component of oxidative stress-related injury.37 In a murine model of hypertension, IsoLG protein adducts and preamyloid oligomers (PAOs) developed in the atria prior to structural or histologic abnormalities coincident with AF susceptibility. These abnormalities and arrhythmia vulnerability were prevented by administration of a dicarbonyl scavenger 2-hydroxybenzylamine (2-HOBA), but not by an inactive analog 4-hydroxybenzylamine (4-HOBA). These findings support the concept of pre-emptively scavenging reactive downstream mediators, rather than targeting generation of reactive oxidative species per se, as a potential therapeutic approach to prevent AtCM. Preclinical and Phase I studies of 2-HOBA have demonstrated its safety and tolerability, and clinical trials in humans are in progress.38,39
2.6. Atrial adipose tissue and fibro-fatty infiltration
Altered metabolism and increased abundance of epicardial adipose tissue (EAT) in the atria is associated with a higher risk of AF.40–42 EAT43–47 could contribute to the formation of the AF substrate by several mechanisms. First, EAT continuously secrete fatty acids that fuel the myocardium, together with a variety of cytokines and peptides, as well as extracellular vesicles.48,49 Some of these secreted molecules, like TGFα, KIL-1B, and FAPB4, can regulate myocardial electrophysiology, others like adiponectin regulate the oxidative stress of the atrial myocardium.50–52 Furthermore, during AF, heart failure or in diabetic patients, EAT secretes profibrotic cytokines such as activin-A and metalloproteases that contribute to the spreading of fibrosis from the epicardial layer to the neighbouring subepicardial myocardium.53–55 Clusters of mononuclear inflammatory cells including cytotoxic CD8+ T-lymphocytes are often observed at the junction between subepicardial fat and fibrotic tissue, resembling lymphocytic myocarditis of patients with AtCM.56,57 Furthermore, the secretome of abnormal EAT contains several inflammatory chemokines. Therefore, EAT is an important potential contributor to atrial inflammation.58,59
The replacement of the EAT beneath the epicardial layer by fibrosis causes fibro-fatty infiltration of the subepicardial atrial layers in AF patients and in aged patients with heart failure or mitral regurgitation.55,58,60 It is established that subepicardial fibro-fatty infiltration is a major arrhythmogenic substrate in both the ventricles and the atria, favouring epicardial–endocardial asynchrony with local conduction block, breakthroughs, and rotor formation.61–63 Moreover, 3D computational modelling of human atria integrating MR images and histo-anatomical data suggests that there is a relationship between the slowing of electrical conduction and the degree of fibro-fatty infiltration.64
The precise mechanisms regulating the accumulation and shaping of the biological properties of EAT are poorly understood. EAT originates mainly from epicardial progenitor cells (EPC). EPC are normally quiescent in the adult heart, but can be reactivated to differentiate into various lineages, including adipocytes.65,66 In human atrial myocardium, subsets of cells derived from EPC (EPDC) are confined to the sub-epicardium where they can differentiate into adipocytes or fibroblasts in response to local stimuli. For instance, the ANP secreted by stretched atrial cardiomyocytes induces, at low concentrations, the adipogenic differentiation of EPDC by activating cGMP-dependent signalling pathways.67 Angiotensin-II produced by the atria can also induce the differentiation of EPDC into fibroblasts, primarily via activation of canonical SMAD2/3, and p38-MAPK signalling pathways.68 Adipogenic differentiation of EPDC is related to lipid metabolism and the capacity of EAT to store fat, for example when submitted to an excess dietary fatty acid load.69 The multiple mechanisms causing the accumulation of EAT likely contribute to the formation of an arrhythmogenic AF substrate over time.
In conclusion, the formation of EAT is a complex interplay between metabolism, inflammation, and oxidative stress, promoting AtCM and AF by local and systemic mechanisms, and may offer new opportunities for management of AtCM/AF.
3. New findings in the pathophysiology of atrial cardiomyopathy
Many animal model and human studies have revealed a close interplay among AtCM, AF, and stroke, via various mechanisms such as ageing, inflammation, oxidative stress and stretch, which, in turn, lead to fibrosis, electrical, and autonomic remodelling and a pro-thrombotic state.70,71 The complex interplay between the mechanisms creates a feedback loop of ever-worsening AtCM and higher risk of more sustained AF and strokes.70 However, there is often a lack of a clear temporal relationship between the onset of AF and stroke. In fact, the current paradigm of selecting individuals at elevated risk of stroke and therefore warranting oral anticoagulation (OAC) therapy, endorsed by major international societies,71–73 by CHA2DS2-VASc (including congestive heart failure, hypertension, age, diabetes mellitus, prior stroke/transient ischaemic attack/thromboembolism, vascular disease) or related scores, are all variables related to the risk of AtCM but not the properties of AF per se (frequency, duration, ventricular rates, etc.). This observation suggests that additional factors inherent in AtCM may be important contributors to stroke occurrence and that the presence of AF per se may not be necessary.74,75 Of note, the recently published Apixaban to Prevent Recurrence After Cryptogenic Stroke in Patients With Atrial Cardiopathy (ARCADIA) trial failed to observe benefit from the oral anticoagulant apixaban in patients with cryptogenic stroke and biomarker evidence of AtCM.76
3.1. Ageing and atrial cardiomyopathy
One major factor leading to AtCM may be ageing, which leads to progressive decline in the structure and function of the heart and is a leading risk factor for cardiovascular diseases. There is strong epidemiological evidence for a close link between ageing, AF, and stroke. The prevalence of many AF and stroke risk factors, including diabetes, hypertension, and coronary/peripheral atherosclerotic vascular disease, steadily increases with age. All these risk factors contribute to the development of atrial cardiomyopathy, and there is mounting evidence supporting the concept that AtCM not only leads to stasis, but endothelial/endocardial dysfunction and a hypercoagulable state, three key factors in thrombogenesis, as originally described by Virchow.77
Tissue remodelling, characterized by myocyte loss, reactive cellular hypertrophy, fibrosis and autonomic nervous system dysregulation, is part of the pathophysiology of AtCM, and a possible common denominator of ageing, stroke, and other AF risk factors (Figures 2 and 3). On the other hand, recent evidence indicates that AF is a major accelerator of ageing of the atrial myocardium (Table 3). At the molecular level, the association between AtCM and ageing (Figure 4) appears to be mediated by oxidative stress,79 calcium handling dysregulation,80 electrophysiologic changes, and structural remodelling with apoptosis and fibrosis,70 all of which contribute to the initiation and/or maintenance of AF, but the mechanisms have been inadequately explored.81 In fact, it is unknown why some ageing individuals with AtCM develop AF while others do not. Chronic inflammation is associated with several age-related diseases such as atherosclerosis, Alzheimer's disease, sarcopenia, and arthritis.82 The genesis of chronic inflammation with ageing (so-called ‘inflammaging’) is unclear but inflammation could be an underlying mechanism connecting ageing to AtCM and AF.83 Furthermore, several markers of cellular ageing, such as telomere length, SA-β-gal, and p16a are associated with AF.81 DNA damage, a mechanism underlying cell senescence, has been shown in tachypaced atrial myocytes and patients in persistent AF.84,85 Clearly, a comprehensive understanding of the molecular mechanisms of intrinsic cardiac ageing, including atrial ageing, will be required to improve understanding of the relation between ageing, inflammation, AtCM, and AF. Such new understanding should guide the development and future translation of novel therapies to clinical application.

Age-related changes in atrial tissue structure. Commonly found age-related changes in atrial tissue structure (Masson's trichrome staining) which represent the structural bases of atrial ageing. yo, years old.
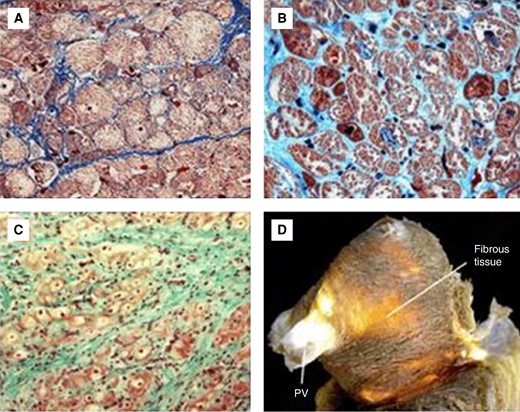
(A) Light microscopy image (Masson's trichrome stain) of atrial tissue with normal intercellular space (41 yo) and (B) cardiomyocyte loss with expansion of interstitial fibrous tissue (82 yo). (C) and (D): myofibrillar loss and fibrotic replacement in the aged left atrium. yo, years old.
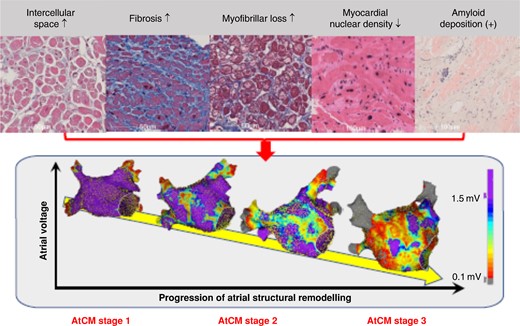
Histological factor associated with atrial structural remodelling characterized by electroanatomic mapping. The upper panel shows light microscopy images of atrial biopsy samples obtained from patients with non-valvular atrial fibrillation. The lower panel shows examples of high-density bipolar voltage map of the left atrium created during high right atrial pacing at 100 beats per minute. Not only fibrosis, but also increased intercellular space preceding fibrosis, myofibrillar loss, decrease in myocardial nuclear density, and amyloid deposition are associated with the progression of atrial structural remodelling.78 The rightmost image in the upper panel shows Congo Red staining, the second from the right shows haematoxylin and eosin staining, and the others show Masson's trichrome staining. The colour gradation in the lower panel voltage maps indicates differences in voltage amplitude from purple at 1.5 mV to grey at 0.1 mV, as shown in the colour bar. AtCM, atrial cardiomyopathy (see Table 2 for explanation of AtCM stages).
1. Age-dependent cardiomyocyte loss and myocyte hypertrophy |
2. Interstitial changes and increase in intercellular space |
3. Enhancement of the interstitial adipose and fibrosis tissue |
3. Endocardial fibrosis |
4. Inflammatory infiltrates |
6. Vacuolar degeneration and Aschoff bodies |
8. Iron depositions |
9. Amyloidosis |
1. Age-dependent cardiomyocyte loss and myocyte hypertrophy |
2. Interstitial changes and increase in intercellular space |
3. Enhancement of the interstitial adipose and fibrosis tissue |
3. Endocardial fibrosis |
4. Inflammatory infiltrates |
6. Vacuolar degeneration and Aschoff bodies |
8. Iron depositions |
9. Amyloidosis |
1. Age-dependent cardiomyocyte loss and myocyte hypertrophy |
2. Interstitial changes and increase in intercellular space |
3. Enhancement of the interstitial adipose and fibrosis tissue |
3. Endocardial fibrosis |
4. Inflammatory infiltrates |
6. Vacuolar degeneration and Aschoff bodies |
8. Iron depositions |
9. Amyloidosis |
1. Age-dependent cardiomyocyte loss and myocyte hypertrophy |
2. Interstitial changes and increase in intercellular space |
3. Enhancement of the interstitial adipose and fibrosis tissue |
3. Endocardial fibrosis |
4. Inflammatory infiltrates |
6. Vacuolar degeneration and Aschoff bodies |
8. Iron depositions |
9. Amyloidosis |
3.2. Gender and atrial cardiomyopathy
Based on epidemiologic investigations, women seem less vulnerable to AF development than men,86 with the prevalence-discrepancy between genders becoming less clear with advancing age, in part perhaps due to the higher life expectancy in women or increased amount of epicardial fat.86–88 A substantial reason for the lower prevalence of AF in women vs. men appears to be their smaller size, since body size (and particularly lean body mass) is a major determinant of AF risk.89 Furthermore, compared to men, women suffering from AF appear to be exposed to significantly greater risks of all-cause and cardiovascular death,90 as well as reporting graver symptoms and a worse quality of life, all of which suggest more serious AF-related structural/functional impairment.91
Recent studies on AF ablation have also shown significant gender-based differences between males and females, with the latter showing a higher risk of arrhythmia recurrence92,93 and increased periprocedural complications and hospitalization rates.94 In addition, a recent large systematic review and meta-analysis of post-ablation outcomes found that, independently of age, comorbidities, and hospital factors, women display higher rates of complications and readmissions following AF ablation.95 In 2022, Wong et al. explored whether gender-based electrophysiological substrate differences might explain the aforementioned worse AF ablation outcomes in women. Intriguingly, they found that females are characterized by significantly more conduction velocity slowing, a greater proportion of complex fractionated signals, and at 22-month follow-up, notably poorer single-procedure and multi-procedure arrhythmia-free survival. These results strongly suggest more advanced atrial remodelling in women.96 Clinical studies have suggested that oestrogen deficiency or oestrogen–progestin imbalance (as that in the luteal phase of the menstrual cycle, during oral contraceptive treatment, during pregnancy, and menopause) may be associated with an increased occurrence of cardiac arrhythmias. The RETAC-group, in a recent study involving 220 AF patients that underwent high-density voltage mapping in SR prior to their first PVI procedure, found that women had greater low-voltage LA voltage-mapping scores than men, with this propensity driven by older women (>60 years) with persistent AF while absent in younger women.97 Moreover, it has already been demonstrated that administration of oestrogen, whose receptors are significantly expressed in cardiomyocytes, is linked with prolonged action potential duration, atrial and atrio-ventricular nodal conduction time, as well as atrial refractoriness, with these functional abnormalities likely due to increased calcium influx leading to weaker repolarizing currents or potassium channel downregulation.98,99 Moreover, prior investigations had shown that, compared to men, and in individuals with or without AF, women possess greater amounts of atrial fibrosis both in histopathological and cardiac magnetic resonance (CMR) studies.100,101
3.3. Atrial failure: definitions, causes, and consequences
Over the past few years, various definitions of atrial failure have been proposed, which, in turn, have been based on functional, morphological, or morpho/functional approaches.102–104 Generally, all of these are based on the concept of ‘atrial cardiomyopathy’ proposed by the original EHRA/HRS/APHRS/SOLAECE expert consensus document.2 Based on the AtCM staging proposed in Table 2, atrial failure would primarily feature in stage 3 AtCM (Figure 3).
The causes of atrial failure are multiple: (i) Atrial rhythm disorders. Rapid atrial arrhythmias—such as AF—induce ineffective active muscle contraction, with both systolic and diastolic LV dysfunction. A similar LV impairment may also be a consequence of suboptimal electrical AV coupling [e.g. due to long PR interval, left bundle branch block, advanced interatrial block (IAB)]102; (ii) Atrial myocardial structural remodelling. Morphological changes at the histological and ultrastructural levels (cardiomyocyte and/or interstitial modifications), often as a consequence of associated cardiac or non-cardiac diseases, along with AF in a vicious cycle (‘AF begets AF’); (iii) Atrial ischaemia, myocarditis, and induced post-ablation stiff LA syndrome. These can lead to increased atrial stiffness, reduced contractility, and electromechanical dissociation.2,105–108 As for the consequences of atrial failure, heart failure, increased thrombogenicity, and pulmonary hypertension may be caused by altered flow dynamics and suboptimal LV filling; AF may also result from atrial failure and contribute to the consequences.102,109 Recent investigations have shown that both LA dysfunction and remodelling are independently associated with, or may even herald, the onset of HF in an asymptomatic healthy population, in which reduced atrial reserve during exercise can indicate the first evidence of a failing LA.110,111 In addition to these pathogenetic mechanisms, atrial electrical dysfunction—and impaired LA–LV coupling—could play a key part in patients suffering from HFpEF.110,112 By the same token, atrial failure may reveal previously latent LV dysfunction and precipitate a HF syndrome. According to the Frank–Starling law, in the initial stages of LV dysfunction, adaptive atrial chamber dilation develops to counteract higher preload, while an increase in active pump function contributes to preserving adequate LV filling. Further impairment in LA function does nothing to mitigate the above-mentioned disproportionate atrial volume/pressure, leading to higher LA and pulmonary-wedge pressures and manifest atrial failure syndrome, as well as perpetuating a harmful vicious cycle.102
Recent evidence suggests a relationship between atrial disease and the risk of stroke independent of AF.74,75 The Asymptomatic Atrial Fibrillation and Stroke Evaluation in Pacemaker Patients and the Atrial Fibrillation Reduction Atrial Pacing Trial (ASSERT) followed 2580 patients aged ≥65 years with a pacemaker and defibrillator and a history of hypertension but without a history of AF. The authors found that only 15% of patients with subclinical atrial fibrillation (SCAF)-associated embolic events had evidence of SCAF >6 min in duration during the month before their stroke or systemic embolism. In addition, most SCAF that had occurred before embolic events was far shorter than 48 h (the minimum duration which, in the past, was believed to be required for thrombus formation in the LAA before cardioversion).113,114 Based on the results of this trial, it appears that some thromboembolic events may be secondary to stasis from an actual AF episode, some to persistent endothelial changes (e.g. due to previous AF episodes), and, lastly, some to mechanisms not directly related to AF, in which the supraventricular arrhythmia may simply represent a risk marker or, if causal, related via complex indirect pathogenetic mechanisms within the context of AF-related AtCM.115 Two additional clinical studies (TRENDS and IMPACT), have found similar results to those of the ASSERT study on the lack of temporal correlation between AF episodes and cerebrovascular events.74,75 Interestingly, analysis of data from the Multi-Ethnic Study of Atherosclerosis (MESA) displayed an inverse association between LA reservoir function (as total LA emptying fractions), and the risk of cerebrovascular events, with this association being independent of acknowledged cerebrovascular risk factors and AF itself.102 These findings suggest value of LA functional parameters in the stratification of individuals at risk for ischaemic cerebrovascular events, particularly in those with no clear evidence of AF.111 In addition to the risk of thrombosis in AF, the amount of atrial fibrosis in patients with stroke of unknown aetiology seems to be greater than in patients with an identified cause and comparable to those suffering from AF.102,116–118 Endothelial changes and regional/global wall motion abnormalities associated with increased fibrosis have been proposed as potential risk factors for atrial thrombosis and cerebrovascular events.102,116,119 Müller-Edenborn et al., in a large cohort of AF patients undergoing TEE prior to electrical cardioversion for AF, found that in individuals with LAA thrombi, 97% of them had a p-wave-based diagnosis of AtCM, which was associated with reduced LAA-flow velocity at TEE. This study adds further evidence that AtCM is associated with both prolonged atrial conduction time and reduced contractile function with reduction in LAA-flow velocity, especially in those who develop LAA thrombi.120 The observation of the ARCADIA trial of no benefit from apixaban in patients with cryptogenic stroke and biomarker evidence of AtCM argues against the idea that AtCM without clinical AF increases the risk of stroke appreciably, but further consideration of the results and design of the trial is needed before any definite conclusions can be made.76
The rhythm and contraction of the atria contribute to efficient coronary blood flow. Controlling for other known risk factors, patients with AF have a three-fold increased risk of myocardial infarction,121 possibly because irregular, shortened diastole with altered flow reserve is a common cause of ventricular ischaemia and a contributor to type 2 myocardial infarction.122,123 Additional mechanisms involved in the pathogenesis of atrial ischaemia/infarction in individuals with atrial myopathy/AF include increased inflammation/oxidative stress, altered platelet activation, and particularly atrial thromboembolism, which can occur even in the absence of AF.111,124
Atrial dysfunction secondary to supraventricular arrhythmias involves activation of neurohormonal pathways, (e.g. RAA system and vasoactive peptides) and can induce ventricular chamber dilation with altered systolic and diastolic cardiac function. In this scenario, the LV myocardium shows inflammation, cardiomyocyte histopathological changes, and loss of normal myocardial extracellular matrix (ECM) structure, composition, and function.125
3.4. Atrial functional valvular regurgitation
Atrial functional mitral and tricuspid regurgitation are clinicopathological conditions, which are closely related to atrial failure and atrial dilatation.126–129 Secondary or functional mitral and tricuspid regurgitation (FMR; FTR) is defined as valvular regurgitation caused by abnormal left/right heart function as opposed to primary structural valve changes.130 While FMR/FTR is most often the result of abnormalities in left/right ventricular remodelling, more recently recognized is the entity of atrial FMR (AFMR/AFTR). In this scenario, ventricular geometry and function are relatively preserved, the culprit being mitral/tricuspid annular enlargement associated with left/right atrial dilatation, which results in poor coaptation of the valve leaflets causing AFMR and AFTR. Compared to ventricular FMR, the entity of AFMR/AFTR, including its prevalence, pathophysiology, prognostic implication, and potential therapy, is less clear.126,127,129 Variability in the literature as to the definition of AFMR is a contributing factor. Recently, an expert opinion paper has been communicated that presents a unifying definition of AFMR.131 The major criteria for AFMR include normal LV cavity size and systolic function (global and regional), and the presence of both mitral annular dilation and LA enlargement. The LV may dilate secondarily in later stages of significant AFMR. AFMR is primarily rooted in excessive LA and mitral annular dilation which pulls both leaflets apart and thereby causes leaflet tethering and malcoaptation.132,133 Generally, two common clinical scenarios may lead to excessive LA and subsequent mitral annular dilation in the context of preserved LV function: AF and/or HFpEF.132 Both syndromes are linked by LA remodelling and dysfunction. AF is known to be both cause and consequence of atrial remodelling.102 In addition, chronically elevated filling pressures in HFpEF may induce adverse anatomical and functional LA remodelling. Furthermore, AF and HFpEF are strongly inter-related as they share common cardiovascular risk factors and potentiate each other. In patients with AF, restoration of sinus rhythm is associated with reduction in LA volumes, mitral annular size, and the degree of MR.134,135 Although this observation suggests that rhythm rather than rate control in AF might mitigate AFMR, this idea has not been formally tested.
Recent data point to a high prevalence of occult HFpEF among patients with AF and dyspnoea, especially in those who remain symptomatic after sinus rhythm restoration.136,137 This observation has led several groups to discern an AF-predominant HFpEF subtype with more advanced LA myopathy, disproportionate to the degree of LV dysfunction.137–139 The occurrence of AFMR in HFpEF is another marker of this subtype of advanced AtCM, leading to worse haemodynamics and poorer exercise reserve.134 A recent community study of moderate-to-severe isolated MR found that only 50% of patients with AFMR had a history of AF.135 Even when mild-to-moderate, the presence of AFMR tended to be associated with worse outcomes, though this association faded after correcting for LA cardiomyopathy. While this latter finding may suggest that the degree of AtCM is of greater importance in prognostic significance compared to AFMR per se, it does not refute the concept that treating AFMR in HFpEF could be beneficial by improving AtCM and thereby outcomes. In addition, recent cross-sectional data cannot disentangle the sequence of causality between AFMR, LA cardiomyopathy, and HFpEF. Presumably, a bidirectional relationship exists between AFMR and AtCM.134 Future prospective studies are needed to answer these questions.
3.5. Metabolic disorders and atrial cardiomyopathy
Metabolic disorders are the most common modifiable risk factors for AtCM and AF.43–47 This relationship is well established for obesity, diabetes, and metabolic syndrome, whereas it is still debated for liver steatosis-associated diseases.140,141 In the Framingham heart study, diabetes is associated with 40% increased risk of AF, and the risk increases with the duration of AF.142,143 High BMI is also associated with a higher incidence of AF.144
Metabolic diseases can directly impact the progression of AF substrate and AtCM through several mechanisms and signalling pathways.43,45 For instance, diabetes and glucose intolerance are characterized by inefficient energy production and metabolic inflexibility of the atrial myocardium with predominance of fatty acid beta-oxidation, mitochondrial dysfunction, and ROS production.145 This metabolic remodelling has a marked impact on atrial excitability and contractile properties, for example, explaining the decreased peak longitudinal strain function and left atrial deformation reported in diabetes mellitus patients.111 The excitation–contraction coupling process of cardiomyocytes is tightly controlled by cellular metabolism. Abnormal activity of several calcium and potassium channels of atrial cardiomyocytes have been reported in experimental models of diabetes.146 K-ATP channels that are normally closed and preferentially coupled to glycolysis over beta-oxidation lipolysis, are open in atrial myocytes of dysmetabolic mice, contributing to action potential shortening.69
An excess of dietary fatty acids can induce an adaptive metabolic signature of the atrial myocardium characterized by impaired glycolysis and predominance of fatty acid oxidation.67 Furthermore, when the uptake of lipid by atrial cardiomyocytes overwhelms their beta-oxidation capacity, a process of lipid storage ensues, activated through elongation of fatty acids, recruitment of adipogenic transcription factors, and adipogenic differentiation of progenitor cells.69 Altogether, these phenomena result in accumulation of intramyocardial lipid and expansion of EAT,147 whose role in the progression of AtCM and risk of AF is detailed elsewhere in this article. However, it must be noted here that metabolic disorders have specific impacts on EAT properties. For instance, in obese or diabetic patients, EAT secretes inflammatory cytokines which favour myocardial fibrosis and contribute to altered excitability.148 By analogy with what is known for ventricle remodelling or atherosclerotic plaque, another potential interface between metabolic disorders and myocardial inflammation are macrophages.149,150 Clinical studies are warranted to investigate the impact of improving metabolic status of patients on the risk of AF and AtCM.
3.6. Atrial cardiomyopathy and atrial ablation
Structural remodelling, including histological and anatomical-structural changes, contributes to the trigger and maintenance mechanisms of AF151 (Figure 4). Conversely, long-lasting AF itself also contributes to the progression of AF, leading to AtCM.152 The most effective way to interrupt this vicious cycle between AF maintenance and AtCM is to restore sinus rhythm. AF ablation is the most effective rhythm control strategy; it significantly reduces AF burden and prevents the progression of AtCM.153,154 Using bipolar voltage mapping and MRI, Sugumar et al.155 reported that rhythm control by AF ablation induces recovery of atrial electrical and structural changes, suggesting that AF ablation may result in some reversal of AF-induced AtCM (see Section 7).
3.7. The development of atrial fibrosis
Fibrosis is a central, causally related pathophysiological component of AtCM.156,157 It is now well established that the extent of LA interstitial fibrosis roughly parallels the biological/clinical history of AF; i.e. from early structural remodelling in most sinus rhythm patients, to paroxysmal, persistent, and, ultimately, long-standing lasting persistent AF.157,158 Additionally, interstitial fibrosis is often a progressive multi-step event in anatomical/architectural terms, with, in most cases, the pulmonary vein outlet area being the predominant initial location, with subsequent progression extending the pathology to the rest of the LA.105,159,160
The above-mentioned pathogenic peculiarities have raised various interesting questions, such as (i) Why is the pulmonary vein area—and, in a broader sense, the left atrial posterior wall—the area most prone to fibrosis (and structural remodelling in general)? (ii) Assuming that interstitial collagen deposition occurs through autocrine/paracrine mechanisms, what are the key cellular sources and cell-networks involved? (iii) Is collagen deposition in the atria a ‘local affair’, i.e. are atrial mesenchymal cell-components the only players in this process? Do any extra-cardiac mesenchymal components cooperate in the development of interstitial structural remodelling?
There are several possible explanations for why fibrosis begins in the LA posterior wall: (i) According to Laplace's law, the left atrial posterior wall is prone to greater stress than the left atrial appendage or other LA free walls. This is because the LA posterior wall is the thinnest part of the LA, blood pressure is similar throughout the LA and LA appendage, and the LA chamber volume is greater than that of the LA appendage; (ii) Anatomically, the LA posterior wall is close to the pulmonary vein orifices, a region subject to intense and continuous mechanical stretching; (iii) Embryologically, the LA appendage develops from the embryonic LA, whereas the LA proper originates from an outgrowth of the pulmonary vein.105,159 It has also recently been suggested that, in AF patients, atrial fibrosis (assessed by magnetic resonance imaging (MRI) with late gadolinium enhancement) might be more extensive in the area adjacent to the descending thoracic aorta, with its extent inversely correlated with the distance between LA and the aorta.161,162 A recent study explored fibrotic remodelling in LA biopsies from a series of patients with and without AF.158 In the vast majority of cases, collagen fibre deposition begins unevenly throughout the atrial myocardium, with separate fronts of fibrosis progressing from both the adventitial/periadventitial tissues and the thickened sub endocardium into the inter-cardiomyocyte interstitium. This tendency might be explained by the fact that both the adventitia and sub endocardium are already richer in collagen-producing cells under normal conditions. Subsequently, fibrosis becomes increasingly widespread and more homogeneous by gradually filling in the preserved myocardial areas. On these grounds, fibrosis stages might be defined as follows: early, presence of sparse single myocardiosclerotic foci originating from the sub endocardium or the periadventitial tissues; intermediate, formation of multiple single myocardiosclerotic foci originating from the subendocardium or the periadventitial tissues; advanced, generation of confluent myocardiosclerotic foci; and very advanced, development of areas of diffuse fibrosis (Figure 5).163 In agreement with this concept, elevated circulating fibrocytes have been proposed as a marker of left atrial fibrosis (in low-voltage regions) and AF recurrence.164,165
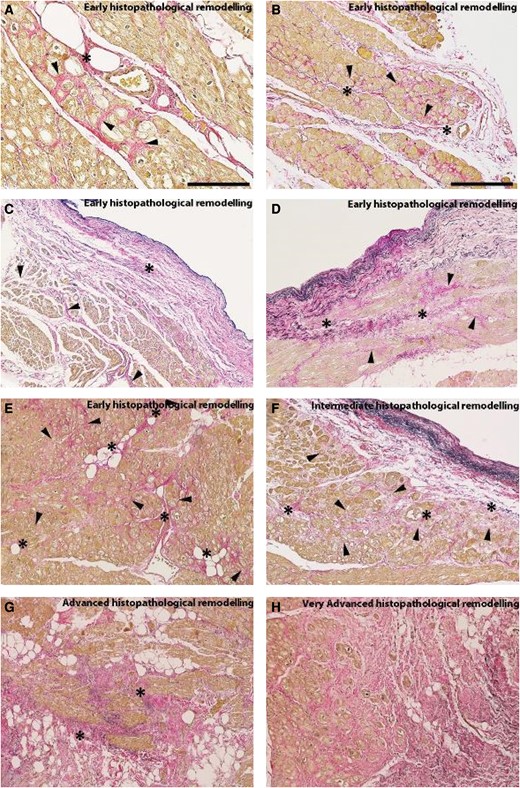
Microphotographs captured from left atrial biopsies belonging to patients suffering from long-lasting atrial fibrillation associated with severe mitral-valve regurgitation. These histopathological sections have been stained with a combined Weigert/Van Gieson dye where cardiomyocytes are highlighted in yellow-brown, collagen fibres in red, and elastic fibres in black. (A) Medium-power view of a developing Early-Stage histopathological remodelling (ATCM stage 1) where multiple foci of interstitial fibrosis (arrowheads) stem from the adventitia of a penetrating coronary branch (asterisk). (B) Low-power view of an incipient Early-Stage histopathological remodelling with multiple interstitial fibrosis foci (arrowheads) originating from a longitudinally oriented blood vessel (asterisks). (C) An initial Early-Stage AtCM histopathological remodelling with a fibroelastotic endocardial thickening (asterisk) from which newly deposed collagen fibres enlarge the adventitia of subendocardial blood vessels (arrowheads). (D) Incipient Early-Stage histopathological remodelling interstitial fibrosis (arrowheads) stemming from an endocardium thickened by fibroelastosis which had also enlarged local blood vessel adventitia (asterisk). (E) Early-Stage histopathological remodelling characterized by multiple foci of interstitial fibrosis (arrowheads) that had originated from sparse penetrating blood vessel adventitias (asterisks). (F) Another type of Intermediate-Stage histopathological remodelling (AtCM stage 2) compound interstitial fibrosis (arrowheads) which takes origin from two blood vessels and a thickened endocardium (asterisks). (G) Advanced-Stage histopathological remodelling characterized by confluent myocardiosclerotic foci (asterisks). H. Compact and diffuse areas of interstitial fibrosis (Very Advanced-Stage histopathological remodelling; AtCM stage 3). Staining. (A-G) Weigert/Van Gieson combined dye. Original magnifications. (A): ×20 (scale bar is 150μm); (B-G): ×10 (scale bar in (B) is 300μm).
As mentioned above, the deposition of collagen in the extracellular space is an important hallmark of atrial fibrosis, and AtCM develops as a consequence of many pathophysiological processes, including inflammation, oxidative stress, ischaemia, and AF itself (Figure 5).2,43,45 However, the process of fibrosis is broader than just the deposition of collagens and encompasses the proliferation of fibroblasts and other mesenchymal cells and their differentiation into α-smooth muscle actin (α-SMA) expressing myofibroblasts, and the deposition of significant amounts of a range of ECM proteins, including various molecular species of collagen as well as other matricellular proteins (Figure 5).166–168 In a proteomic analysis of ECM proteins in left and right atria of patients with and without AF, the glycoprotein decorin was found to be most altered.169 Decorin is implicated in collagen fibrillogenesis and the production of a variety of other ECM molecules involved in cellular signalling; cleaved decorin suppress myostatin activity and increases myocyte size. These effects are similar to observations in a goat model of pacing-induced AF.170 Another analysis of the full proteome of atrial tissue of patients with and without AF identified neutrophil degranulation as the most upregulated process in AF, and the reactive oxygen donor myeloperoxidase as the most abundantly upregulated protein. Myeloperoxidae, in turn activates atrial fibroblasts and promotes ECM expansion.171,172 A whole-genome RNA sequencing analysis of LA tissue of patients with no AF, paroxysmal or persistent AF suggested epithelial-to-mesenchymal transition as an important driver of fibrosis formation and AF progression. Interestingly, increased (or decreased) proteins in paroxysmal vs. non-AF tended to increase (or decrease) further in persistent vs. paroxysmal AF.173 It has been shown in experimental models that early molecular and electrophysiological abnormalities precede LA structural remodelling and the formation of fibrosis. Several kinases, including liver kinase B1 (LKB1) and atrial AMP-activated protein kinase (AMPK) regulate transcription factors involved in the expression of ion channels and fibrosis and contribute to AtCM.43,45,174 These studies provide a link between inflammation and metabolic stress and the development of AtCM. The PREDICT AF trial demonstrated upregulation of various ECM proteins, including collagen1A1, collagen 3A1, collagen8A2, tenascin-C, thrombospondin-2, and biglycan in patients without a history of AF or AtCM who develop AF during the subsequent months or years.175
4. Imaging advances
The multimodality evaluation of atrial size and function using cardiac imaging is key to the diagnosis of AtCM and the characterization of the degree of atrial damage.176
4.1. Atrial imaging tools in the evaluation of atrial cardiomyopathy
AtCM can be evaluated with echocardiography, CMR imaging, and cardiac computed tomography (CCT). Echocardiography and CMR allow continuous recording of atrial volumes through the cardiac cycle. CCT evaluates accurately atrial volume at a single point in the cardiac cycle. Three-dimensional transthoracic echocardiography (TTE) avoids the problem of foreshortening of the atria that can occur with two-dimensional TTE.177 Left and right atrial appendages are more specifically characterized by transoesophageal echocardiography (TEE) and CCT. CCT is also validated in the evaluation of EAT and has been recently implemented with deep learning algorithms.178 The progression of LA roof shape, as determined by CT, is associated with the development of non-PV arrhythmic substrates in patients undergoing AF ablation.179,180 Pre-AF ablation CCT-based regional wall deformations were better predictors of low-voltage area than other remodelling surrogates, suggesting potential clinical utility.181
CMR has been applied to evaluate atrial fibrosis with late gadolinium enhancement analysis and atrial velocity based on 4D flow imaging (see detailed discussion below).182,183 The specific characteristics of each technique in atrial evaluation are summarized in Table 4.
Atrial parameters . | TTE . | TEE . | Cardiac MRI . | Cardiac CT . | Electroanatomic mappinga . |
---|---|---|---|---|---|
Atrial Size | ++ (more accurate in 3D TTE) | — | ++ | +++ | + |
Atrial Function | +++ (more accurate in 3D TTE) | — | +++ | + | — |
Atrial appendage size | — | +++ | + | +++ | — |
Thrombus | + | +++ | ++ | +++ (delayed acquisition)b | — |
Epicardial adipose tissue | + | — | + | +++ | — |
Atrial fibrosis | — | — | + (LGE)b | — | + |
Atrial velocity | — | ++ (exclusively in atrial appendage) | +++ (4D flow imaging) | — | — |
Atrial activation/conduction time | — | — | — | — | + p-wave duration |
Atrial parameters . | TTE . | TEE . | Cardiac MRI . | Cardiac CT . | Electroanatomic mappinga . |
---|---|---|---|---|---|
Atrial Size | ++ (more accurate in 3D TTE) | — | ++ | +++ | + |
Atrial Function | +++ (more accurate in 3D TTE) | — | +++ | + | — |
Atrial appendage size | — | +++ | + | +++ | — |
Thrombus | + | +++ | ++ | +++ (delayed acquisition)b | — |
Epicardial adipose tissue | + | — | + | +++ | — |
Atrial fibrosis | — | — | + (LGE)b | — | + |
Atrial velocity | — | ++ (exclusively in atrial appendage) | +++ (4D flow imaging) | — | — |
Atrial activation/conduction time | — | — | — | — | + p-wave duration |
LGE, late gadolinium enhancement; 3D, Three-dimensional; TTE, transthoracic echocardiography; TEE, transoesophageal echocardiography; MRI, magnetic resonance imaging; CT, computed tomography.
aElectroanatomic mapping includes voltage mapping, activation sequence mapping, and evaluation of conduction velocity and fractionated electrograms.
bContrast agents are necessary.
Atrial parameters . | TTE . | TEE . | Cardiac MRI . | Cardiac CT . | Electroanatomic mappinga . |
---|---|---|---|---|---|
Atrial Size | ++ (more accurate in 3D TTE) | — | ++ | +++ | + |
Atrial Function | +++ (more accurate in 3D TTE) | — | +++ | + | — |
Atrial appendage size | — | +++ | + | +++ | — |
Thrombus | + | +++ | ++ | +++ (delayed acquisition)b | — |
Epicardial adipose tissue | + | — | + | +++ | — |
Atrial fibrosis | — | — | + (LGE)b | — | + |
Atrial velocity | — | ++ (exclusively in atrial appendage) | +++ (4D flow imaging) | — | — |
Atrial activation/conduction time | — | — | — | — | + p-wave duration |
Atrial parameters . | TTE . | TEE . | Cardiac MRI . | Cardiac CT . | Electroanatomic mappinga . |
---|---|---|---|---|---|
Atrial Size | ++ (more accurate in 3D TTE) | — | ++ | +++ | + |
Atrial Function | +++ (more accurate in 3D TTE) | — | +++ | + | — |
Atrial appendage size | — | +++ | + | +++ | — |
Thrombus | + | +++ | ++ | +++ (delayed acquisition)b | — |
Epicardial adipose tissue | + | — | + | +++ | — |
Atrial fibrosis | — | — | + (LGE)b | — | + |
Atrial velocity | — | ++ (exclusively in atrial appendage) | +++ (4D flow imaging) | — | — |
Atrial activation/conduction time | — | — | — | — | + p-wave duration |
LGE, late gadolinium enhancement; 3D, Three-dimensional; TTE, transthoracic echocardiography; TEE, transoesophageal echocardiography; MRI, magnetic resonance imaging; CT, computed tomography.
aElectroanatomic mapping includes voltage mapping, activation sequence mapping, and evaluation of conduction velocity and fractionated electrograms.
bContrast agents are necessary.
4.2. Atrial size and function
The atria serve a variety of important functions, e.g. (i) as a reservoir for blood prior to ventricular filling, (ii) as a transport chamber for the conduit of blood during diastole, and (iii) as an active booster pump during atrial systole. Three main atrial volumes reflect these three main functional phases of the atria during the cardiac cycle: (i) maximum atrial volume, evaluated in end-systole during the reservoir phase, (ii) pre-atrial contraction volume evaluated in mid-diastole during the conduit phase and (iii) minimum atrial volume evaluated in late diastole during the booster-pump phase (Figure 6). Atrial function indices are calculated from the different atrial volumes. Atrial total emptying fraction and expansion index consider minimal and maximal atrial volumes, atrial passive emptying fraction considers maximal and pre-atrial contraction volumes, atrial active emptying fraction considers pre-atrial contraction and minimal volumes. Atrial strain is evaluated using TTE and cardiac CMR during the three phases of the atria: reservoir strain, conduit strain and contractile strain (Figure 6; supplementary material online, Figure S1 and S1.1). Notably, CCT emerges as a valuable tool for comprehensively evaluating atrial function in AtCM. CCT provides unparalleled spatial resolution when compared to other imaging methods, allowing detailed assessment of atrial function and morphology.184 Different studies define normal values of size and function for the left and right atria in the different phases of the cardiac cycle in TTE, CMR, and CCT (see Supplementary material online, Table S1).185–192 Recently, data in the literature suggest that LA reservoir strain <18% could be useful in heart failure to define high left ventricular filling pressures. However, to the best of our knowledge, there is no cut-off value defining irreversible atrial dysfunction in AtCM (Table 2; Table 5).
![Atrial size and function during the cardiac cycle: volume (A) and strain (B) curve analysis. Precise calculations: Total EF = [(maxAV − minAV)/ maxAV]; Expansion Volume = [(maxAV − minAV)/minAV]; Passive EF = [(maxAV − preAV)/maxAV]; Active EF = [(preAV − minAV)/preAV]. Abbreviations: maxAV, Maximal Atrial Volume; minAV, Minimal Atrial Volume; preAV, Pre–atrial contraction Volume; EF, Emptying Fraction.](https://oup.silverchair-cdn.com/oup/backfile/Content_public/Journal/europace/26/9/10.1093_europace_euae204/5/m_euae204f6.jpeg?Expires=1749338162&Signature=kXJzpJvWXvFT05t3vZzSsYR~GFdUCFahjuJdtjXf-grVgW4nJQh-vRLTwnf7I4Iim7JANnMIvfCpnwlCGFUj9Gb-GZhzxl8I5n6b9xlNJJdmCf19Fycejj10B7BnpUk8nTfPvB5ySvjCJFjwnov4sK3gBh04TS-uIzIkPJjOCtRBqUJjeTMj4YrZTsFnAdkF2s0viZ6np1KYmatLdGuECyJUtw4mQW6bq00oSkgCsD-d7vKOcdcoE0dOfmL8oNyqokEyw3HMQL9upowuahe2dlH5w8sLFS5zmx-wXK~b8t6LYbxSYbUEuK7vBx1uoii12ZqA6dMScI~biKPVJKZbhA__&Key-Pair-Id=APKAIE5G5CRDK6RD3PGA)
Atrial size and function during the cardiac cycle: volume (A) and strain (B) curve analysis. Precise calculations: Total EF = [(maxAV − minAV)/ maxAV]; Expansion Volume = [(maxAV − minAV)/minAV]; Passive EF = [(maxAV − preAV)/maxAV]; Active EF = [(preAV − minAV)/preAV]. Abbreviations: maxAV, Maximal Atrial Volume; minAV, Minimal Atrial Volume; preAV, Pre–atrial contraction Volume; EF, Emptying Fraction.
Multimodal assessment of cardiac chamber anatomy and function in AF: assessment of stroke risk prediction using LA strain study
Author, year, Reference . | Population . | Technique, methods of evaluating parameters . | Results . |
---|---|---|---|
Stroke risk prediction with LA deformation studies . | |||
Shih, 2011178 |
|
|
|
Azemi, 2012182 |
|
| Compared to controls, patients with AF, stroke or TIA and CHADS2 scores ≤1 before their event had:
|
Obokata, 2014183 |
|
|
|
Leung, 2018184 |
|
| 100 patients (7%) developed an ischemic stroke Despite the similarity of LV dimensions, LVEF, longitudinal global strain, LA volumes patients with AF and stroke have in comparison with patients without stroke have:
|
Author, year, Reference . | Population . | Technique, methods of evaluating parameters . | Results . |
---|---|---|---|
Stroke risk prediction with LA deformation studies . | |||
Shih, 2011178 |
|
|
|
Azemi, 2012182 |
|
| Compared to controls, patients with AF, stroke or TIA and CHADS2 scores ≤1 before their event had:
|
Obokata, 2014183 |
|
|
|
Leung, 2018184 |
|
| 100 patients (7%) developed an ischemic stroke Despite the similarity of LV dimensions, LVEF, longitudinal global strain, LA volumes patients with AF and stroke have in comparison with patients without stroke have:
|
2D, two-dimensional; 3D, three-dimensional; AF, atrial fibrillation, TTE, transthoracic echocardiography; LAV, LA volume; LA, left atrium; LVEF, left ventricular ejection fraction; CI, confidence interval; GLS, global longitudinal strain; HR, hazard ratio; LV, left ventricle; OR, odds ratio; PA-TDI, P-wave to A' duration on tissue Doppler imaging.
Multimodal assessment of cardiac chamber anatomy and function in AF: assessment of stroke risk prediction using LA strain study
Author, year, Reference . | Population . | Technique, methods of evaluating parameters . | Results . |
---|---|---|---|
Stroke risk prediction with LA deformation studies . | |||
Shih, 2011178 |
|
|
|
Azemi, 2012182 |
|
| Compared to controls, patients with AF, stroke or TIA and CHADS2 scores ≤1 before their event had:
|
Obokata, 2014183 |
|
|
|
Leung, 2018184 |
|
| 100 patients (7%) developed an ischemic stroke Despite the similarity of LV dimensions, LVEF, longitudinal global strain, LA volumes patients with AF and stroke have in comparison with patients without stroke have:
|
Author, year, Reference . | Population . | Technique, methods of evaluating parameters . | Results . |
---|---|---|---|
Stroke risk prediction with LA deformation studies . | |||
Shih, 2011178 |
|
|
|
Azemi, 2012182 |
|
| Compared to controls, patients with AF, stroke or TIA and CHADS2 scores ≤1 before their event had:
|
Obokata, 2014183 |
|
|
|
Leung, 2018184 |
|
| 100 patients (7%) developed an ischemic stroke Despite the similarity of LV dimensions, LVEF, longitudinal global strain, LA volumes patients with AF and stroke have in comparison with patients without stroke have:
|
2D, two-dimensional; 3D, three-dimensional; AF, atrial fibrillation, TTE, transthoracic echocardiography; LAV, LA volume; LA, left atrium; LVEF, left ventricular ejection fraction; CI, confidence interval; GLS, global longitudinal strain; HR, hazard ratio; LV, left ventricle; OR, odds ratio; PA-TDI, P-wave to A' duration on tissue Doppler imaging.
4.3. LA late gadolinium-enhanced cardiac magnetic resonance imaging
Late gadolinium-enhanced CMR (LGE-CMR) methods play a pivotal role for diagnostic and prognostic assessment of ventricular cardiomyopathies. LGE may be caused by conditions that significantly increase the interstitial space or that slow down gadolinium exit.193 LGE is a marker of myocardial fibrosis in chronic myocardial infarction and various non-ischaemic cardiomyopathies. In addition, LGE may represent an increase in the interstitial space caused by interstitial oedema or by tissue infiltration of inflammatory cells, like acute myocarditis or cardiac sarcoidosis.193,194 Interstitial overload of amyloid proteins is also a potential cause of LGE.193 In recent years, LGE-CMR methods have been developed in an attempt to systematically image and quantify LA fibrosis.181 However, LGE-CMR presents challenges for the detection of LA fibrosis, including lack of standardized acquisition protocols and processing techniques, and low image resolution related to the thin LA wall,2,195 and is still available only in a limited number of research centers. Recent studies comparing the most common LGE-CMR methods revealed considerable discrepancies in global and regional LGE extent.196,197 LGE-CMR cannot be used to visualize diffuse fibrosis because ‘normal’ myocardium with diffuse fibrosis is nulled to highlight focal scar, resulting in loss of information on any background interstitial expansion.198 This limitation is potentially quite problematic in light of an autopsy study showed that atrial fibrosis is often a diffuse process.199 LGE regions detected in the atria may represent relatively advanced fibrotic or non-fibrotic lesions. Studies using LGE-CMR to quantify LA fibrosis have provided interesting observations such as anchoring of LA rotors at the border between fibrotic and non-fibrotic zones,200 a correlation between greater fibrosis and more AF recurrence post-AF ablation201 and a relationship between the extent of LA fibrosis and stroke risk.202 Two recent randomized controlled studies reported that targeting LA fibrosis detected on LGE-MRI in addition to PV isolation, compared to PV isolation alone, resulted in no significant improvement in atrial arrhythmia recurrence rates.203,204
4.4. Electroanatomic mapping
Electroanatomic mapping has been widely used during catheter ablation (CA) procedures to define AtCM. Electroanatomical mapping provides voltage mapping, activation sequence mapping, and evaluation of conduction velocity and fractionated electrograms.78,196,205,206 A reduction in endocardial bipolar voltage amplitudes, represented as low-voltage areas on electroanatomic maps (LVA, commonly defined as an area with bipolar voltage <0.5 mV), has been widely used as a marker for AtCM. Diffuse voltage reductions are associated with increase in left atrial volume and other cardiovascular risk factors (Figure 4).205 LVAs have been considered a surrogate of local fibrosis. An electroanatomical mapping study showed that atrial bipolar voltage reduction is a diffuse process; with LVAs being local reflections of diffuse voltage reduction associated with histological fibrosis.205 However, a recent atrial biopsy study showed that not only fibrosis, but also other histological factors including increased extracellular space, myofibrillar loss, decreased myocardial nuclear density, and amyloid deposition are associated with atrial voltage reduction, reflecting associated complex pathological changes (Figure 4).78
4.5. Prediction of incident atrial fibrillation by cardiac imaging
At present, no specific AF treatment decision is based solely on cardiac imaging. In large cohort studies using TTE and CMR, increases in LA volumes and decreases in LA function were associated with incident AF.207–211 In a large multi-ethnic population, larger RA volume indices were also independently associated with incident AF after adjustment for cardiovascular risk factors and LA parameters.212 CCT-determined EAT mass appears correlated with the risk of incident AF.213,214 In addition, EAT volume is highly associated with paroxysmal and persistent AF independent of traditional cardiovascular risk factors.41 Impaired LA function and LA enlargement were associated with the number of premature atrial contractions per hour on extended ambulatory electrocardiographic monitoring.215,216
4.6. Recurrent atrial fibrillation
The progression of LA roof shape, as determined by CT, is associated with the development of non-PV arrhythmic substrates in patients undergoing AF ablation.179,180 Pre-AF ablation CCT-based regional wall deformations were better predictors of LVA presence than other remodelling surrogates, suggesting potential clinical utility.181 LA fibrosis extent estimated by LGE-CMR is significantly and independently associated with recurrent arrhythmia post-AF ablation.201 However, two recent randomized controlled studies reported that targeting LA fibrosis detected on LGE-MRI in addition to PV isolation, compared to PV isolation alone, resulted in no significant improvement in atrial arrhythmia recurrence rates.203,204 The authors suggested that the lack of benefit from fibrosis-guided ablation is explained by limited overall fibrosis burden in the cohort204 and technical challenges, including lack of standardized and established end-points for fibrosis ablation.203 They also hypothesized that different types of fibrosis can occur in atrial tissue, including interstitial and reparative fibrosis, unequally contributing to AF development.203
4.7. Reverse atrial remodelling and atrial remodelling
Cardiac imaging could be useful to predict reverse cardiac remodelling following treatment of AF217 and to evaluate anatomical and functional components of reverse remodelling, as well as improvement in functional atrio-ventricular valve regurgitation after restoration of sinus rhythm.218 Using CCT, Huang et al.219 observed that atrial remodelling begins with increased atrial (LA and RA) volumes after 6 min of atrial high-rate episodes (AHRE), and atrial contractile dysfunction (both LA and RA) manifests when the duration of AHRE exceeded 6 h.
4.8. Risk stratification
Using CCT, Tsao et al. 220, in their investigation of atrial remodelling in relation to AF-related stroke, found that increased age and reduced booster-pump function of the atria are independently associated with stroke occurrence, suggesting that substrate-based assessments might enhance stroke risk stratification in AF patients. LA strain analysis appeared to improve the risk stratification of thromboembolism in patients with AF183 and to discriminate the presence of LA or LA appendage (LAA) thrombus on TEE.221,222 LAA morphologies and volume are correlated with the history of ischaemic stroke and transient ischaemic attack.223,224 AF resulted in overall impaired flow dynamics in both LA and LAA.225 Regardless of history of AF, patients at moderate-to-high risk of stroke exhibit modified flow characteristics in the LA on CMR.225,226 A greater atrial FDG uptake on PET-scanning was associated with a greater prevalence of stroke in AF patients.227 The presence of LVA has been reported to be associated with history of stroke and subclinical silent cerebral ischaemia (SCI)228 and concurrent heart failure with systolic dysfunction in patients with non-valvular AF.229 In addition, a recent observational study enrolling 1488 patients undergoing AF ablation showed that LVA presence and its extent were both associated with poor long-term composite end-points of death, heart failure, and stroke, irrespective of AF recurrence or other confounders.230 Cardiac imaging allows identification of early atrial dysfunction and its systematic integration in the characterization of AtCM, and as a diagnostic and prognostic imaging tool merits active investigation.227
5. Biomarkers, omics, ECG parameters
5.1. Biomarkers
A whole range of biomarkers, whether urine, blood, imaging, or ECG-based, have been proposed as surrogates of an ‘AtCM state’ that may predict the occurrence and progression of AF. Blood-based biomarkers can be used in combination with ECG/electrophysiological or echocardiographic data, to define such a state. These biomarkers can be electrophysiological, structural, haemodynamic, serological, and genetic (Table 6). Importantly, these biomarkers of atrial cardiomyopathy have all been associated with the risk of stroke, even in the absence of overt AF. Hence, they have been used as inclusion criteria in some studies investigating AtCM in relation to stroke or cerebrovascular risk, as defining criteria for the condition. For example, in the ARCADIA trial,232 which investigated patients with cryptogenic ischaemic stroke and atrial cardiomyopathy, the inclusion criteria included patients ≥45 years of age with embolic stroke of undetermined source (ESUS) and evidence of AtCM defined as ≥1 of the following markers: P-wave terminal force >5000 µV × ms in ECG lead V1, serum NT-proBNP > 250 pg/mL, and left atrial diameter index ≥3 cm/m2 on the echocardiogram. Of note, the ARCADIA trial did not confirm effectiveness of these biomarkers in selecting patients with cryptogenic stroke for OAC therapy.76 The ARIC (Atherosclerosis Risk in Communities) study evaluated the association of echocardiographic left atrial function (reservoir, conduit, and contractile strain) and left atrial size (left atrial volume index) with ischaemic stroke.233 A further analysis from ARIC found that the presence of AtCM (based on P-wave terminal, NT-proBNP, and LA volume index criteria) was significantly associated with an increased risk of dementia, with limited impact of the effect by AF or stroke.234
Biomarkers classified into categories . | Specific examples . |
---|---|
Electrophysiological | |
Atrial fibrillation | Subclinical atrial fibrillation |
P-wave features | P-wave terminal force in lead V1 |
Duration of amplified p-wave | |
P-wave morphology | |
Paroxysmal supraventricular tachycardia | |
Atrial ectopy | Excessive supraventricular ectopic activity |
Structural | |
Left atrial size | Left atrial enlargement, left atrial volume index |
LAA morphology | Non-chicken wing type |
Myocardial fibrosis | Regions of delayed gadolinium enhancement on cardiac MRI |
Haemodynamic | |
LAA-flow velocity | Low flow velocity |
Serological | |
NT-proBNP, BNP | |
Hs-cTnT | |
Genetic | |
Polymorphisms | rs2200733, rs10033464 in chromosome 4q25 |
Biomarkers classified into categories . | Specific examples . |
---|---|
Electrophysiological | |
Atrial fibrillation | Subclinical atrial fibrillation |
P-wave features | P-wave terminal force in lead V1 |
Duration of amplified p-wave | |
P-wave morphology | |
Paroxysmal supraventricular tachycardia | |
Atrial ectopy | Excessive supraventricular ectopic activity |
Structural | |
Left atrial size | Left atrial enlargement, left atrial volume index |
LAA morphology | Non-chicken wing type |
Myocardial fibrosis | Regions of delayed gadolinium enhancement on cardiac MRI |
Haemodynamic | |
LAA-flow velocity | Low flow velocity |
Serological | |
NT-proBNP, BNP | |
Hs-cTnT | |
Genetic | |
Polymorphisms | rs2200733, rs10033464 in chromosome 4q25 |
LAA, left atrial appendage; MRI, magnetic resonance imaging; NT-proBNP, N-terminal pro-brain natriuretic peptide; BNP, brain natriuretic peptide; Hs-cTnT, high-sensitivity cardiac troponin T.231
Biomarkers classified into categories . | Specific examples . |
---|---|
Electrophysiological | |
Atrial fibrillation | Subclinical atrial fibrillation |
P-wave features | P-wave terminal force in lead V1 |
Duration of amplified p-wave | |
P-wave morphology | |
Paroxysmal supraventricular tachycardia | |
Atrial ectopy | Excessive supraventricular ectopic activity |
Structural | |
Left atrial size | Left atrial enlargement, left atrial volume index |
LAA morphology | Non-chicken wing type |
Myocardial fibrosis | Regions of delayed gadolinium enhancement on cardiac MRI |
Haemodynamic | |
LAA-flow velocity | Low flow velocity |
Serological | |
NT-proBNP, BNP | |
Hs-cTnT | |
Genetic | |
Polymorphisms | rs2200733, rs10033464 in chromosome 4q25 |
Biomarkers classified into categories . | Specific examples . |
---|---|
Electrophysiological | |
Atrial fibrillation | Subclinical atrial fibrillation |
P-wave features | P-wave terminal force in lead V1 |
Duration of amplified p-wave | |
P-wave morphology | |
Paroxysmal supraventricular tachycardia | |
Atrial ectopy | Excessive supraventricular ectopic activity |
Structural | |
Left atrial size | Left atrial enlargement, left atrial volume index |
LAA morphology | Non-chicken wing type |
Myocardial fibrosis | Regions of delayed gadolinium enhancement on cardiac MRI |
Haemodynamic | |
LAA-flow velocity | Low flow velocity |
Serological | |
NT-proBNP, BNP | |
Hs-cTnT | |
Genetic | |
Polymorphisms | rs2200733, rs10033464 in chromosome 4q25 |
LAA, left atrial appendage; MRI, magnetic resonance imaging; NT-proBNP, N-terminal pro-brain natriuretic peptide; BNP, brain natriuretic peptide; Hs-cTnT, high-sensitivity cardiac troponin T.231
5.2. Blood-based biomarkers
While AF-related biomarkers have been studied extensively,235,236 relatively few biomarkers reflecting AtCM per se (without AF) have been assessed.237,238 Nonetheless, many biomarkers have been assessed in both scenarios, reflecting the non-specificity of many biomarkers (see later). Progression of AF from paroxysmal to persistent is sometimes taken to indicate underlying atrial substrate abnormalities, and some biomarkers have been tested in this scenario.
The natriuretic peptides closely associated with HF239 are also associated with risk of incident AF and its complications.240,241 Cardiac troponin (cTnT), a biomarker of myocardial damage used to diagnose myocardial ischaemic events, was associated cardioembolic stroke In the ARIC study.242 Soluble suppressor of tumorigenicity-2 protein (sST2) and tissue inhibitor of matrix metalloproteinase (TIMP)-1 are associated with AF progression, independent of clinical characteristics and biomarkers.243 Fibrosis biomarkers may predict AF recurrence after cardioversion.238 Recently, elevated serum levels of bone morphogenetic protein 10 (BMP10), primarily expressed by atrial myocytes, was shown to be associated with recurrences of AF following CA.244 Cohort studies in patients with AF demonstrated an association with ischaemic stroke,245 all-cause mortality and major adverse cerebrovascular and cardiovascular events.246
C-reactive protein (CRP) is associated with AF and AF burden in nonsurgical patients, suggesting that inflammatory processes contribute to atrial remodelling.247 The association of van Willebrand factor and soluble P-selectin with stroke supports the role of hypercoagulability and endothelial damage to the risk for stroke in AF patients.248
5.3. Limitations of biomarkers
Some biomarkers are non-specific, perhaps reflecting a sick patient or a sick heart.249,250 For example, Growth Differentiation Factor 15 (GDF-15) is a stress-responsive biomarker associated with several types of cardiovascular diseases, and has been proposed as a specific biomarker of bleeding risk in AF.251 Nonetheless, GDF-15 is also associated with mortality and MACE in non-AF subjects.252 Assay variability, diurnal variation of biomarker levels and costs may limit practical usability of both urine and blood biomarkers. A balance is needed between potentially useful information they can provide and simplicity/practical application in busy clinical settings.253 Thus far, there are no biomarkers that are validated for clinical management guidance in AtCM.
5.4. Multi-omics and atrial cardiomyopathy
Primary atrial cardiomyopathy arises from atrial-specific gene variants, as well as genes that associate with extra-atrial features that are functional in the atrial myocardium.12,254 One atrial-specific gene variant that gives rise to atrial electromechanical defects is the MYL4 gene, which encodes the highly atrial-selective essential myosin light chain.255,256 Another example is the NPPA gene, which encodes ANP A: NPPA variants have been associated with extreme bi-atrial dilation, thromboembolic risk and atrial standstill.254,257 Examples of gene variants that associate with ventricular and/or systemic disorders, but can also produce atrial dysfunction include HCN4, encoding the cardiac pacemaker channel;258 SCN5A, encoding the main cardiac voltage-gated sodium channel;259 SCN1B, which encodes the voltage-gated sodium channel β1/β1B subunits;260 and LMNA, which encodes Lamin A/C.261
Most of our knowledge related to AtCM genomics, transcriptomics and epigenomics derives from studies on AF, which also leads to atrial dysfunction. Large-scale, genome-wide association studies (GWAS) have identified chromosomal loci harboring common variants that influence atrial ECG parameters, including heart rate, P-wave duration, and PR interval,262 as well as variants associated with cardiac size, structure and contractile function.263 Altogether, GWAS studies have revealed over 100 genetic loci associated with AF susceptibility (Figure 7).264,265 It is unknown whether the expression of any of these variants also results in primary AtCM, but their association with AF may lead to secondary AtCM.
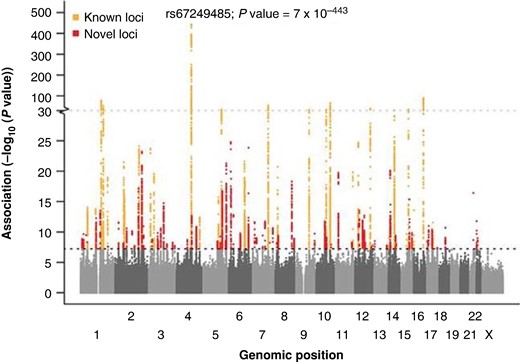
Manhattan plot showing known loci and novel loci associated with atrial fibrillation. A total of 34 740 186 genetic variants (each represented by a dot) were tested, comparing 60 620 atrial fibrillation cases and 970 216 controls free of atrial fibrillation. The x axis represents the genome in physical order, and the y axis represents P values (−log10(P value)) of association. The black horizontal dotted line represents a Bonferroni-corrected threshold of statistical significance corresponding to 1 000 000 independent tests (P < 5 × 10−8). Reprinted by permission from Nielsen et al.264
Transcriptional studies in human atrial samples using both microarrays and RNA-seq have identified genes responsible for atrial remodelling,266 among them NPPA and NPPB,267 GPR22 and RGS6, encoding G-protein signalling components; NTM, that encodes neurotrimin, a promising novel marker of HF,268 or COLQ, which is found in both left and right atria, and encodes a variant collagen that anchors acetylcholinesterase to the basal lamina.269 A recent mRNA sequencing study in atrial specimens from patients undergoing cardiac surgery identified 35 genes robustly associated with AF independently of the clinical diagnose of heart failure.267 Genes were involved in regulation of cardiomyocyte structure, conduction properties, fibrosis, inflammation, and endothelial dysfunction.270 A large-animal model study on AF progression showed that significant differential transcriptomic and proteomic expression changes are established early during the transition from paroxysmal to persistent AF.271
It is now clear that AF is a condition in which both environmental and genetic factors contribute to disease pathogenesis.265,272 Most AF-associated variants identified by GWAS lie within non-coding regions of the genome,273 where they affect gene-expression by altering transcription factor activity and the epigenetic state of chromatin. Epigenetics refers to heritable alterations that are not due to changes in DNA. Methylation of cytosine-phosphate-guanine (CpG) islands by DNA methyltransferases (DNMTs) occurs in paroxysmal and permanent AF.274,275 A recent article used 4 DNA methylation-based ageing biomarkers to provide an epigenetic basis for the known association between chronological age and incident AF (Figure 8).276,277 Other studies demonstrated histone modifications associated with AF.278,279 Specifically, histone deacetylases (HDAC) and histone transferases (HAT) appear to play an important role in AF.278 Patients suffering from AF have increased expression of EZH2, encoding the histone methyltransferase responsible for the H3K27me3 signature, in both atrial cardiomyocytes and fibroblasts.279 It is clear that progress in the understanding of the mechanisms of AtCM will require a new generation of multiomic studies in patients with AtCM independent of AF.

Although chronological age progresses at an immutable rate, biological ageing, measured by epigenetic age in this article, is modifiable by various exposures and life experiences. Thus, establishing a relationship between epigenetic age and atrial fibrillation (AF) risk gives a unique insight into modifiable ageing associated atrial fibrillation risks. Reprinted from Ward-Caviness et al.277
5.5. ECG parameters
In AtCM, structural, architectural, contractile, and electrophysiologic changes in the atria manifest as ECG abnormalities. Table 7 provides examples of studies supporting these associations, which have been reviewed in detail elsewhere.301–303
. | Associations . | References . |
---|---|---|
Amplified digital p-wave duration (APWD) | Atrial fibrosis/low voltage Atrial contractile dysfunction, LAA thrombogenesis Reduced LAA-velocity AF AF recurrence after catheter ablation | 280,281 120,221 120 282 280,281 |
PTFV1 | Low LA appendage ejection velocity | 283 |
Functional atrial remodelling | 284 | |
AF | 285 | |
AF recurrence after catheter ablation | 286 | |
P-wave axis | AF | 287 |
P-wave voltage | AF | 288 |
AF recurrence after catheter ablation | 289 | |
P-wave area | LA enlargement | 290 |
AF in mitral stenosis | 291 | |
P-wave dispersion | Idiopathic paroxysmal AF | 292,293 |
AF recurrence after cardioversion | 294 | |
Fibrillatory wave (F-wave) | LA enlargement | 295–297 |
Low LA appendage flow | 296 | |
Long-standing persistent AF | 297,298 | |
Heart failure | 297 | |
AF recurrence after catheter ablation | 299,300 |
. | Associations . | References . |
---|---|---|
Amplified digital p-wave duration (APWD) | Atrial fibrosis/low voltage Atrial contractile dysfunction, LAA thrombogenesis Reduced LAA-velocity AF AF recurrence after catheter ablation | 280,281 120,221 120 282 280,281 |
PTFV1 | Low LA appendage ejection velocity | 283 |
Functional atrial remodelling | 284 | |
AF | 285 | |
AF recurrence after catheter ablation | 286 | |
P-wave axis | AF | 287 |
P-wave voltage | AF | 288 |
AF recurrence after catheter ablation | 289 | |
P-wave area | LA enlargement | 290 |
AF in mitral stenosis | 291 | |
P-wave dispersion | Idiopathic paroxysmal AF | 292,293 |
AF recurrence after cardioversion | 294 | |
Fibrillatory wave (F-wave) | LA enlargement | 295–297 |
Low LA appendage flow | 296 | |
Long-standing persistent AF | 297,298 | |
Heart failure | 297 | |
AF recurrence after catheter ablation | 299,300 |
LA, left atrial; AF, atrial fibrillation.
. | Associations . | References . |
---|---|---|
Amplified digital p-wave duration (APWD) | Atrial fibrosis/low voltage Atrial contractile dysfunction, LAA thrombogenesis Reduced LAA-velocity AF AF recurrence after catheter ablation | 280,281 120,221 120 282 280,281 |
PTFV1 | Low LA appendage ejection velocity | 283 |
Functional atrial remodelling | 284 | |
AF | 285 | |
AF recurrence after catheter ablation | 286 | |
P-wave axis | AF | 287 |
P-wave voltage | AF | 288 |
AF recurrence after catheter ablation | 289 | |
P-wave area | LA enlargement | 290 |
AF in mitral stenosis | 291 | |
P-wave dispersion | Idiopathic paroxysmal AF | 292,293 |
AF recurrence after cardioversion | 294 | |
Fibrillatory wave (F-wave) | LA enlargement | 295–297 |
Low LA appendage flow | 296 | |
Long-standing persistent AF | 297,298 | |
Heart failure | 297 | |
AF recurrence after catheter ablation | 299,300 |
. | Associations . | References . |
---|---|---|
Amplified digital p-wave duration (APWD) | Atrial fibrosis/low voltage Atrial contractile dysfunction, LAA thrombogenesis Reduced LAA-velocity AF AF recurrence after catheter ablation | 280,281 120,221 120 282 280,281 |
PTFV1 | Low LA appendage ejection velocity | 283 |
Functional atrial remodelling | 284 | |
AF | 285 | |
AF recurrence after catheter ablation | 286 | |
P-wave axis | AF | 287 |
P-wave voltage | AF | 288 |
AF recurrence after catheter ablation | 289 | |
P-wave area | LA enlargement | 290 |
AF in mitral stenosis | 291 | |
P-wave dispersion | Idiopathic paroxysmal AF | 292,293 |
AF recurrence after cardioversion | 294 | |
Fibrillatory wave (F-wave) | LA enlargement | 295–297 |
Low LA appendage flow | 296 | |
Long-standing persistent AF | 297,298 | |
Heart failure | 297 | |
AF recurrence after catheter ablation | 299,300 |
LA, left atrial; AF, atrial fibrillation.
P-wave duration (IAB): a p-wave duration ≥120 ms indicates partial IAB.304 Biphasic P-wave morphology in inferior leads (II, III, aVF) indicates complete block of Bachmann's bundle (advanced IAB).302,303 IAB is strongly associated with LA enlargement.305 Advanced IAB is associated with fibrotic atrial cardiomyopathy, an environment for re-entry, and premature atrial beats, which promote the development and progression of AF.306,307 Patients with prolonged P-wave duration301 or advanced IAB have a higher risk of recurrent AF after CA.308 Paradoxically, abnormally short P-wave duration is also a risk factor for incident309 or recurrent AF.310 Amplified (150–200 mm/sec, 80–100 mm/mV) digital P-wave duration can predict arrhythmia recurrences after PVI, detection of HF patients at risk for AF, and determine different AtCM-severity stages (discrete stage of AtCM: APWD 140–150 ms; moderate AtCM-stage: APWD 150–180 ms and advanced AtCM-stage: APWD > 180 ms or presence of pos-negative p-waves in two of three inferior leads) implicating LAA slow flow velocity and LAA thrombogenesis and major adverse event rates.120,280–282
Besides P-wave duration, P-wave terminal force in V1 (PTFV1) is a commonly used marker for AtCM. PTFV1 is calculated as the product of its duration component (PTDV1) and its absolute amplitude component (PTAV1). An absolute PTFV1 value >4 mV·ms is considered pathological.287 Abnormal PTFV1 is associated with low LA appendage ejection velocity in TEE.283 Originally, a pathological PTFV1 was suggested as a marker of LA enlargement and posterior displacement, but more recent studies suggest that it is caused by conduction abnormalities resulting in predominantly posterior terminal LA activation.284,311 LA strain measured by speckle tracking echocardiography, an indicator of functional atrial remodelling, is reduced in the presence of PTFV1 abnormalities.284 A recent meta-analysis showed that PTFV1 is associated with risk of AF and is a predictor of AF in patients with and without cardiovascular disease285 and of AF recurrence after CA.286 Recently, it was shown that amplified P-wave duration of digital 12-lead ECG is associated with left atrial low-voltage substrate and can identify patients with persistent AF who are at high risk for arrhythmia recurrence after CA312 along with new-onset AF in patients with HFpEF.3,313
P-wave axis: a normal frontal plane p-wave axis is between 0 and +75.302 A recent meta-analysis indicates that an abnormal P-wave axis is associated with future detection of AF.287
P-wave voltage: P-wave voltage ≤0.1 mV in lead I is considered abnormal.302 A score incorporating abnormal p-wave voltage predicts new-onset AF.288 Lead I P-wave amplitude ≤0.1 mV is associated with recurrence of AF after radiofrequency ablation.289
P-wave area: P-wave area is calculated in lead II using the following formula: P-wave area = ½P-wave duration × P-wave voltage.302 Abnormal P-wave area is defined as ≥4 ms × mV, and has been found to be associated with LA enlargement.290 In patients with mitral stenosis, P-wave area in V3 predicts new-onset AF.291 P-wave dispersion: P-wave dispersion is defined as the difference between P-wave maximum and P-wave minimum duration on the 12-lead ECG.302 Greater P-wave dispersion was associated with incident AF and AF recurrence after cardioversion.292,294 In patients with cryptogenic stroke and an implantable loop recorder, P-wave dispersion >40 ms is a predictor of subsequent AF detection.293
Fibrillatory wave (f-wave): lower-amplitude f-waves are associated with larger LA size and lower LA appendage flow.295,296 A low f-wave amplitude in lead II and high dominant rate in lead V1 independently discriminates long-standing persistent AF from other AF-types (paroxysmal/persistent).298 In a community-based prospective survey, fine f-waves were more prevalent than coarse in patients with a larger LA diameter or with persistent AF, and was independently associated with a higher risk of HF.297 Lower preprocedural f-wave amplitudes are associated with AF recurrence in patients undergoing AF ablation.299,300
Signal averaged P-wave analysis: technological advancement of ECG analysis is currently evolving rapidly and has the potential to further improve the prognostic value of ECG recordings in patients with atrial cardiomyopathy. Signal averaged P-wave analysis of ECG recording for several minutes gives more precise and reproducible information about atrial spread of activation. Prolonged signal averaged P-wave durations were found in patients with paroxysmal AF vs. controls without history of AF314 and in patients with recurrences of AF after CA.315 P-wave parameters from prolonged recordings better distinguish between patients with paroxysmal vs. persistent AF compared to standard 10-s ECGs.316
Premature atrial contractions: several studies have identified premature atrial contractions as marker for AtCM, AF and increased stroke risk.317,318
5.6. Artificial intelligence
Artificial intelligence (AI)-based P-wave wavelet analysis promises to provide significant advancements in the field.302 Deep learning approaches have shown that patients at risk for AF can be identified by their ECG in sinus rhythm with very high accuracy,319 suggesting that the commonly used arsenal of P-wave metrics for prediction of incident AF is most likely incomplete. In a pragmatic study to test the efficacy of an AI-ECG guided screening for AF,320 the AF detection rate was five-fold higher in the high-risk AI-ECG group compared to the low-risk group, indicating that AI might increase the effectiveness of ECG-based AF screening. Other studies have successfully applied AI to quantify the substrate for AF and detect targets for AF ablation.321,322
5.7. Future directions
ECG analysis has the potential to identify patients at risk for AtCM, AF, AF recurrences and strokes, particularly if used in conjunction with imaging or circulating biomarkers. The potential clinical impact is significant. Patients at risk for AF or stroke might undergo intensified rhythm monitoring. Patients with high risk for AF recurrences might undergo more aggressive ablation strategies or the treating electrophysiologist might refrain from recommending AF ablation. However, successful implementation of these strategies will depend on finding solutions for structured collection of digital ECGs, standardization of analysis, identification of robust thresholds for risk assessment and ultimately the integration of ECG-derived parameters in prospective trials for clinical decision making.302 Prospective randomized clinical trials will be needed to evaluate the prognostic value of pre-specified ECG parameters as inclusion criteria.
6. Stroke and cognitive decline
6.1. Empiric evidence that atrial cardiomyopathy leads to stroke
There is substantial and growing evidence that AtCM, even in the absence of AF, predicts (and presumably causes) stroke.117,118 Multiple P-wave indices, as discussed above, have been associated with an increased stroke risk.323 Frequent atrial ectopy predicts stroke even without interim evidence of AF.324 While inter-observer variability and changes over time may affect reliability,325 P-wave terminal force in V1 appears to be especially predictive of embolic stroke, even in the absence of evident AF.326,327 Echocardiographic measurements demonstrating a larger LA similarly predict increased risk of stroke, again even when no AF is present.328,329 When P-wave terminal force in V1, left atrial diameter, and incident AF were included in the same multivariable model in a population-based longitudinal cohort, LA diameter did not emerge as an independent risk factor for stroke.330 More recent investigations have focused on dynamic assessments of LA function, with evidence that, among community-dwelling individuals without AF, echocardiographically-ascertained reduced LA strain is associated with incident stroke and adds predictive value to the CHA2DS2-VASc score.233
6.2. Mechanisms underlying the relationship between atrial cardiomyopathy and stroke
Even in AtCM without AF, atrial-wall substrate changes may be evident in association with risk factors such as hypertension, coronary disease, diabetes mellitus, and HF.331–334 Flow abnormalities associated with AtCM117,118 may relate to the presence of diastolic dysfunction and (relative) atrial stasis, most evident as TEE-detected LAA spontaneous echo contrast, even in sinus rhythm,335 or when a paroxysm of AF occurs. Global LA longitudinal strain assessed by speckle tracking TTE discriminates the presence of LA or LA appendage thrombus on TEE in patients with acute ischaemic stroke. Abnormal blood constituents include abnormalities of coagulation and platelets, which characterize the risk factors mentioned above.336,337 Indeed, the concept of ‘thrombogenic endocardial remodelling’ suggests that a diseased atrial endocardium may facilitate the production of thrombi independent of the presence of AF.2 In this circumstance, AF may be an epiphenomenon, with the true causal pathway between AtCM and stroke attributed more directly to pro-thrombotic properties of the atria itself.338–342
6.3. Does atrial cardiomyopathy lead to cognitive decline?
AF might induce cognitive decline through multiple mechanisms: hypoperfusion due to reduced cardiac output, silent brain infarction, inflammation, or platelet dysfunction.343–346 Cerebral microbleeds associated with anticoagulation can also cause cognitive decline.347 Finally, beat-to-beat variability seen in patients with AtCM and AF reduces cardiac output,343,348 and brain hypoperfusion is associated with cognitive dysfunction, brain ageing, and low brain volume.343,348,349 There are selected reports that have evaluated the potential association between abnormal atrial function and cognitive dysfunction, independent of AF or stroke occurrence. In an early consecutive series of 180 older individuals without AF, stroke, or a diagnosis of dementia, LA enlargement on echocardiography was significantly associated with cognitive impairment.350 Greater LA volumes and reduced LA reservoir function are associated with subclinical cerebrovascular disease on brain MRI. The ARIC Study found that the association of LA enlargement with cognitive function was borderline,351 and identified in cross-sectional analyses but not longitudinally.352 Adverse LA electrical remodelling as measured by abnormal P-wave indices was associated with an increased risk of dementia after adjusting for incident AF and stroke.351 In the most recent ARIC report focused on this question, LA enlargement was not associated with dementia risk,353 but abnormal LA strain and emptying fraction were significantly associated with an increased risk of dementia independent of AF or stroke. It is also possible that AtCM and dementia commonly co-exist without a direct causal connection and without relationship to thromboembolism per se. For example, a propensity to amyloid deposits in both the atria and the brain may lead to a concomitant AtCM and Alzheimer's disease.15,354 Left atrial dysfunction as a predictor of cognitive decline may similarly suggest a general predilection to profibrotic remodelling that adversely effects both the heart and the brain.353 In summary, based on existing data there is some evidence linking AtCM to risk of incident dementia, but no clear evidence of a strong independent association between AtCM and cognitive decline.
7. Therapeutic implications
7.1. Primary prevention of atrial cardiomyopathy
Pathological atrial remodelling may be prevented, or possibly even reversed, by addressing the initiating stimulus/disease (e.g. elevated pressure, volume, AF) or targeting the key underlying mechanisms.
Primary prevention refers to preventive therapies before the disease phenotype has emerged, for example preventing AF by preventing or reversing an AtCM.355 Lifestyle modification including achieving/maintaining a healthy bodyweight, has been shown to reduce AF burden and prevent AtCM in patients with AF,356,357 but there is limited evidence on primary prevention of AtCM (i.e. before AF presentation). Similarly, there are few data on the treatment of obstructive sleep apnoea for primary prevention of AtCM. Interventions in the renin-angiotensin-aldosterone system (RAAS) have yielded mixed results,358 with particularly convincing data supporting mineralocorticoid receptor antagonists (MRA)359,360 and RAAS inhibitors in general in primary prevention.352 A meta-analysis of 14 studies (5 RCTs) showed an odds ratio of 0.52 for new-onset AF in patients treated with MRA.359 An updated meta-analysis pooled data from 20 trials (21 791 participants, mean age: 65.2 years, 31.3% women) showed that MRA reduce AF events (risk ratio = 0.76; 95% CI: 0.67–0.87) in both patients with and without prior AF.361 Our current inability to detect the early development of AtCM limits the options to provide effective preventive medicine.
7.2. Lifestyle interventions to reverse atrial cardiomyopathy
Lifestyle-related factors (obesity, smoking, alcohol excess, hypertension and obstructive sleep apnoea) are important contributors to adverse LA remodelling and AF risk.140,141,362–364 The 2020 ESC guidelines list several risk factors and comorbidities, including cardiovascular and non-cardiovascular comorbidities and lifestyle factors, that increase the risk of AF and of AtCM.140 Randomized controlled trials examining weight loss, cardiometabolic risk factor management,141 and alcohol reduction365,366 have demonstrated reduction in AF burden and recurrence rate. In the Framingham cohort, each 10-g/day increase in alcohol intake was associated with a 0.16-mm (95%CI: 0.10–0.21-mm) larger LA dimension367 and among those with established AF, habitual drinkers had larger atria than lifelong non-drinkers (LAVi: 50 ± 13 mL/m2 vs. 43 ± 12 mL/m2). A randomized trial on alcohol abstinence vs. continuation in alcohol users with AF demonstrated later and less frequent recurrence of AF in abstainers.366 A meta-analysis of 10 prospective studies demonstrated a reduction in LA size upon obstructive sleep apnoea treatment with continuous positive airway pressure (CPAP).368 A direct effect of CPAP on electrophysiological remodelling (LA voltage, conduction velocity and LVAs) was also demonstrated in a small randomized study.369
7.3. Pharmacological therapies to reduce progression of atrial cardiomyopathy
Inhibition of the renin-angiotensin aldosterone system has shown some efficacy in preventing new-onset AF, but mostly in those with a history of HF. In a meta-analysis of 14 randomized controlled trials (92 817 patients), angiotensin receptor blockers (RR: 0.78; 95% CI: 0.66–0.92), and angiotensin-converting enzyme inhibitors (RR: 0.79; 95% CI: 0.65–1.00) both reduced the risk of incident AF.370 Another meta-analysis showed that recurrent AF was less frequent (OR 0.37; 95%CI: 0.24–0.57 P < 0.001) in MRA treated patients.359 MRA most prominently suppressed recurrent AF or AF in patients with reduced left ventricular function.371 In a meta-analysis of 5 randomized trials involving HF patients, MRA were associated with modest reductions in LAVI (−1.12 mL/m2; 95%CI: −1.91–0.33 mL/m2).372 Animal studies suggest that angiotensin converting enzyme inhibitors, angiotensin receptor blockers, and aldosterone antagonists can prevent LA enlargement, atrial fibrosis and markers (TGFβ), and atrial dysfunction.372–376 There was no difference in AF burden, nor on AF burden in 425 patients with paroxysmal AF treated with the angiotensin receptor antagonist olmesartan, but this is perhaps not surprising given the lack of angiotensin-renin system activation and generally limited structural remodelling in paroxysmal AF patients.358 SGLT-2 inhibitors are now often used as part of comprehensive therapy in systolic HF, with beneficial effects including improved cardiac energy metabolism, prevention of inflammation, oxidative stress, and adverse cardiac remodelling.377 Treatment of diabetic rats for 8 weeks with empagliflozin prevented LA enlargement, fibrosis, atrial mitochondrial dysfunction, inflammation, and AF inducibility.378 In type 2 diabetes, treatment with dapagliflozin was associated with a 19% reduction in AF events.379
7.4. Impact of rhythm control strategies on atrial cardiomyopathy
Although there is a large body of evidence linking atrial remodelling to the risk of AF and the lower success of medical rhythm control therapy or LA ablation, the opposite, i.e. studies linking reversal of atrial remodelling to improvement in AF-indices, is less well documented (Figure 9).
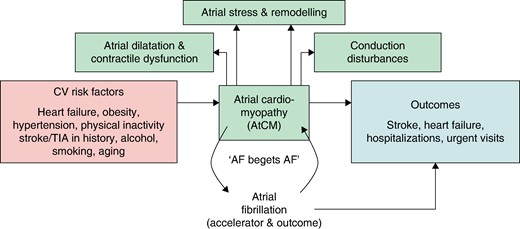
Interaction of risk factors, atrial cardiomyopathy, occurrence of atrial fibrillation and outcome. AtCM, atrial cardiomyopathy; AF, atrial fibrillation; CV, cardiovascular; TIA, transient ischaemic attack.
7.5. Rhythm control and atrial cardiomyopathy
The ATHENA trial demonstrated that dronedarone was associated with a reduction in the combined endpoint of first hospitalization or death.380 The use of dronedarone was also associated with fewer cases of progression to permanent AF HR: 0.65; 95% CI: 0.56–0.75 (P < 0.001).381 The EAST-AFNET 4 trial demonstrated a reduction in adverse cardiovascular outcomes in patients with recently diagnosed AF treated with early (mostly medical) rhythm control, compared to standard of care (mostly rate control).382 The effects were most pronounced in patients with cardiovascular comorbidities (CHA2DS2-VASc score ≥4).383 Restoration of sinus rhythm in a selected population appeared to reduce cardiovascular complications, potentially through reduction or reversal of atrial remodelling/AtCM.
7.6. Reversal of atrial cardiomyopathy by rhythm control?
Catheter ablation by means of pulmonary vein isolation (PVI) is the most effective rhythm control strategy for AF and can partially reverse atrial structural (LA volume increases and mitral regurgitation).133,384 and electrical remodelling. Patients with sinus rhythm after ablation showed a decrease in circulating C-reactive protein, whereas there was no change in patients with AF recurrence in the first 3 months after ablation.385 Recent work suggests that effective rhythm control can prevent progression of AF to more persistent forms.153,386 Potential mechanisms for post-ablation reduction in LA volume include the shrinkage of the left atrium through contraction of the ablation scar,387 but also reduction of mitral regurgitation and reversal of AF-induced atrial remodelling/cardiomyopathy. In the absence of AF recurrence, there was a reduction in LAVI from 31 ± 4 to 28 ± 4 mL/m2 and in atrial electromechanical conduction delay (19 ± 9–13 ± 5 msec), while no reversal of remodelling occurred in patients with AF recurrence after cryoballoon ablation.388 In 106 patients with AF (43 paroxysmal and 63 persistent AF) undergoing PVI, a significant reduction in LAVi from 43 ± 13 to 33 ± 12 mL/m2 was observed.389 Although thoracoscopic AF ablation can be effective in patients with severe LA enlargement, it is uncertain whether reduced LA volume is due to restoration of sinus rhythm per se.133,387,390 In a meta-analysis of 24 studies (2040 patients), maximum LA volume decreased by 6.5 mL (95%CI: 4.4–8.6 mL) after ablation, regardless of whether AF was paroxysmal or persistent.391 Even in the presence of severe LA dilatation (LA volume >200 mL), a 10% reduction in LA size was observed in 52% and was a strong predictor of freedom from AF post-ablation.392 In a substudy of 15 patients with systolic dysfunction who underwent AF ablation (the CAMERA-MRI [Catheter Ablation Versus Medical Rate Control in Atrial Fibrillation and Systolic Dysfunction-Magnetic Resonance Imaging] trial), RA and LA area decreased by 27% and 19%, respectively, after 23 ± 12 months of follow-up, associated with improvements in LV systolic function and atrial electrical reverse remodelling.155 In 86 patients undergoing ablation for persistent AF, those who maintained sinus rhythm at 12 months had a greater reduction in RA area, which correlated with reduced FTR.393 CMR studies have shown that both LA and RA emptying fraction increased significantly by 30–40% after 6 months of follow-up after ablation for persistent AF in 20 consecutive patients.394 LA functional reserve during exercise was lower in 61 patients with AF recurrence after ablation.395 LA strain improved after CA (21.3% ± 1.7% to 28.6% ± 1.7%), whereas it declined with medical management (26.3% ± 1.2% to 21.7% ± 1.2%), driven in part by AF burden.154 Restoration of sinus rhythm was associated with greater reductions in LA volume, presence and extent of MR and annular size.133 Electrical cardioversion of AF did result in return of atrial systolic function, but did not alter the elevated levels of plasma Von Willebrandfactor, fibrinogen and soluble P-selectin concentrations.396 Overall, the evidence strongly suggests that SR restoration and maintenance is effective in AtCM reversal.
7.7. Device therapy to reverse atrial cardiomyopathy
Cardiac resynchronization therapy (CRT) in patients with HFrEF can partially reverse atrial remodelling. In 107 patients (3 months post-CRT), LA volume was reduced, with improvements in emptying fraction; benefits were largely due to pressure and volume unloading effects from LV reverse remodelling.397 Conduction system pacing is emerging as a more physiological alternative to RV apex pacing, but did not reduce left atrial size in AF patients with HFrEF or HFpEF.398,399 Mitral-valve disease-induced LA enlargement and electrical remodelling can be partially reversed with valve surgery. In patients with severe mitral regurgitation, valve repair resulted in reductions in maximum LAVi (43 ± 14–25 ± 8 mL/m2).400 In patients undergoing mitral commissurotomy, immediate reductions in LA pressure (23 ± 7 to 10 ± 4 mm Hg) were accompanied by reductions in LA volume (75 ± 20 mL to 52 ± 18 mL) with associated improvements in conduction.401
8. Conclusions and future directions
In this document, we have summarized the extensive progress in the area of AtCM since the first consensus document.2 This substantial progress has provided new insights in many important areas, but important knowledge gaps remain, as summarized in Table 8. Additional experimental and clinical studies, registries and big data analyses are needed to fill these knowledge gaps in order to harness the full potential of the AtCM concept for improving the prevention and management of AF.402 The proposed AtCM classification system (AtCM stages; Table 2) and the synopsis about the development/progression of AtCM (Figure 9) warrant further study (for stylistic symmetry), reflection and testing.
Knowledge Gaps in Atrial Cardiomyopathy (AtCM) . | |
---|---|
1. | Optimal monitoring frequency and duration to measure development of AtCM |
2 | Threshold of AtCM that results in an increased risk of stroke, heart failure, dementia, and other AF-related outcomes |
3. | Prevalence of subclinical AtCM in broad community-based cohorts |
4. | Risk factors and determinants of AtCM in broad community-based cohorts |
5. | Temporal relationship between different types of AtCM |
6. | Precise correlation of various biomarkers (ECG, blood parameters etc.) with AtCM |
7. | Threshold of AtCM that indicates need for anticoagulation in patients without the presence of AF (e.g. CHA2DS2-VASc score ≥4?) |
8. | Reversibility of AtCM in response to different therapies, and time course |
9. | Diversity of structural alterations in atrial tissue in patients with AtCM |
10. | Association of AtCM (and its subtypes) with CV comorbidities. |
11. | Molecular mechanisms underlying the development and progression of AtCM |
12. | Histological findings associated with AtCM in patients without AF |
13. | Relationships between atrial function and histological findings in AtCM |
14. | Relationship between LGE-MRI and histology in the diagnosis of AtCM |
15. | Multiomic analysis to elucidate the mechanisms of development and progression of AtCM |
16. | Impact of incretins (GLP-1 analogues, GIP etc.) on AtCM development and regression |
Knowledge Gaps in Atrial Cardiomyopathy (AtCM) . | |
---|---|
1. | Optimal monitoring frequency and duration to measure development of AtCM |
2 | Threshold of AtCM that results in an increased risk of stroke, heart failure, dementia, and other AF-related outcomes |
3. | Prevalence of subclinical AtCM in broad community-based cohorts |
4. | Risk factors and determinants of AtCM in broad community-based cohorts |
5. | Temporal relationship between different types of AtCM |
6. | Precise correlation of various biomarkers (ECG, blood parameters etc.) with AtCM |
7. | Threshold of AtCM that indicates need for anticoagulation in patients without the presence of AF (e.g. CHA2DS2-VASc score ≥4?) |
8. | Reversibility of AtCM in response to different therapies, and time course |
9. | Diversity of structural alterations in atrial tissue in patients with AtCM |
10. | Association of AtCM (and its subtypes) with CV comorbidities. |
11. | Molecular mechanisms underlying the development and progression of AtCM |
12. | Histological findings associated with AtCM in patients without AF |
13. | Relationships between atrial function and histological findings in AtCM |
14. | Relationship between LGE-MRI and histology in the diagnosis of AtCM |
15. | Multiomic analysis to elucidate the mechanisms of development and progression of AtCM |
16. | Impact of incretins (GLP-1 analogues, GIP etc.) on AtCM development and regression |
AtCM, atrial cardiomyopathy; AF, atrial fibrillation; CHA2DS2-VASc score, congestive heart failure, hypertension, age ≥75 (doubled), diabetes, stroke (doubled), vascular disease, age 65–74 and sex category (female). CV, cardiovascular; ECG, electrocardiogram.
Knowledge Gaps in Atrial Cardiomyopathy (AtCM) . | |
---|---|
1. | Optimal monitoring frequency and duration to measure development of AtCM |
2 | Threshold of AtCM that results in an increased risk of stroke, heart failure, dementia, and other AF-related outcomes |
3. | Prevalence of subclinical AtCM in broad community-based cohorts |
4. | Risk factors and determinants of AtCM in broad community-based cohorts |
5. | Temporal relationship between different types of AtCM |
6. | Precise correlation of various biomarkers (ECG, blood parameters etc.) with AtCM |
7. | Threshold of AtCM that indicates need for anticoagulation in patients without the presence of AF (e.g. CHA2DS2-VASc score ≥4?) |
8. | Reversibility of AtCM in response to different therapies, and time course |
9. | Diversity of structural alterations in atrial tissue in patients with AtCM |
10. | Association of AtCM (and its subtypes) with CV comorbidities. |
11. | Molecular mechanisms underlying the development and progression of AtCM |
12. | Histological findings associated with AtCM in patients without AF |
13. | Relationships between atrial function and histological findings in AtCM |
14. | Relationship between LGE-MRI and histology in the diagnosis of AtCM |
15. | Multiomic analysis to elucidate the mechanisms of development and progression of AtCM |
16. | Impact of incretins (GLP-1 analogues, GIP etc.) on AtCM development and regression |
Knowledge Gaps in Atrial Cardiomyopathy (AtCM) . | |
---|---|
1. | Optimal monitoring frequency and duration to measure development of AtCM |
2 | Threshold of AtCM that results in an increased risk of stroke, heart failure, dementia, and other AF-related outcomes |
3. | Prevalence of subclinical AtCM in broad community-based cohorts |
4. | Risk factors and determinants of AtCM in broad community-based cohorts |
5. | Temporal relationship between different types of AtCM |
6. | Precise correlation of various biomarkers (ECG, blood parameters etc.) with AtCM |
7. | Threshold of AtCM that indicates need for anticoagulation in patients without the presence of AF (e.g. CHA2DS2-VASc score ≥4?) |
8. | Reversibility of AtCM in response to different therapies, and time course |
9. | Diversity of structural alterations in atrial tissue in patients with AtCM |
10. | Association of AtCM (and its subtypes) with CV comorbidities. |
11. | Molecular mechanisms underlying the development and progression of AtCM |
12. | Histological findings associated with AtCM in patients without AF |
13. | Relationships between atrial function and histological findings in AtCM |
14. | Relationship between LGE-MRI and histology in the diagnosis of AtCM |
15. | Multiomic analysis to elucidate the mechanisms of development and progression of AtCM |
16. | Impact of incretins (GLP-1 analogues, GIP etc.) on AtCM development and regression |
AtCM, atrial cardiomyopathy; AF, atrial fibrillation; CHA2DS2-VASc score, congestive heart failure, hypertension, age ≥75 (doubled), diabetes, stroke (doubled), vascular disease, age 65–74 and sex category (female). CV, cardiovascular; ECG, electrocardiogram.
Supplementary material
Supplementary material is available at Europace online.
Acknowledgements
We thank Dr. Anjaly Vijayan and IPPMed for their excellent technical assistance and support.
The authors thank the EHRA Scientific Documents Committee:
Prof. Katja Zeppenfeld, Prof. Jens Cosedis Nielsen, Prof. Isabel Deisenhofer, Dr. Luigi di Biase, Prof. Kristina Hermann Haugaa, Dr. Daniel Keene, Prof. Christian Meyer, Prof. Petr Peichl, Prof. Silvia Priori, Dr. Alireza Sepehri Shamloo, Prof. Markus Stühlinger, Prof. Jacob Tfelt Hansen, Prof. Arthur Wilde
(2023) Payment from healthcare industry: speaker fees, honoraria, consultancy, advisory board fees, investigator, committee member, etc., including travel funding related to these activities. Biosense Webster: Atrial Fibrillation (AF); Boston Scientific, Medtronic, Abbott Medical: Atrial Fibrillation (AF), Device Therapy. Research funding from healthcare industry received by your department/institution or an entity controlled by your department/institution, under your direct/personal responsibility. Johnson & Johnson: Research projects, PI; Boston Scientific, Medtronic, Biotronik, Abbott Medical: Research projects, PI.
J.-A.C.: (2021) Direct personal payment from healthcare industry: speaker fees, honoraria, consultancy, advisory board fees, investigator, committee member, etc. Biotronik, Abbott, Medtronic, Johnson & Johnson: Speaker fees.
(2023) Payment from healthcare industry: speaker fees, honoraria, consultancy, advisory board fees, investigator, committee member, etc., including travel funding related to these activities. Abbott: Arrhythmias, General; Johnson & Johnson: Arrhythmias, General.
J.R.d.G.: (2021) Direct personal payment from healthcare industry: speaker fees, honoraria, consultancy, advisory board fees, investigator, committee member, etc. Johnson & Johnson, AtrianMedical: Ablation of atrial fibrillation; Bayer, Daiichi Sankyo: Stroke prevention in atrial fibrillation. Research funding from healthcare industry under your direct/personal responsibility (to department or institution). Bayer, Daiichi Sankyo: Atrial fibrillation; Boston Scientific, Johnson & Johnson: Electrophysiology/atrial fibrillation. Any other interest (financial or otherwise) that should be declared in view of holding an ESC position. Owner of RhythmCARE, personal consultancy firm specialising in consulting, advice, research, and education on the broad field of atrial fibrillation and cardiac electrophysiology. The parties for which RhythmCARE provided services are listed above.
(2023) Ongoing research funding from public and/or not-for-profit organisations (which started in 2023 or continues in 2023). This information is not mandatory and does not impact one's ability to join an ESC activity. ERA4Health. Payment from healthcare industry: speaker fees, honoraria, consultancy, advisory board fees, investigator, committee member, etc., including travel funding related to these activities. Daiichi Sankyo: Atrial Fibrillation (AF), Stroke. AtrianMedical: Other. Payment from healthcare industry to your department or institution or any other body for your personal services: speaker fees, honoraria, consultancy, advisory board fees, investigator, committee member, etc., including travel funding related to these activities. Bayer, Daiichi Sankyo, Atricure: Atrial Fibrillation (AF). Research funding from healthcare industry received by your department/institution or an entity controlled by your department/institution, under your direct/personal responsibility. Johnson & Johnson: AF ablation, PI, Boston Scientific, Medtronic: ICD, PI; Bayer, Daiichi Sankyo: Stroke prevention in AF, PI.
D.D.: (2021) Any other interest (financial or otherwise) that should be declared in view of holding an ESC position. I am Editor-in-Chief of Int J Cardiol Heart Vasc and Deputy-Editor of Int J Cardiol. For my services on both journals, I get compensation from Elsevier.
(2023) Payment from healthcare industry: speaker fees, honoraria, consultancy, advisory board fees, investigator, committee member, etc., including travel funding related to these activities. TMA Foundation: Atrial Fibrillation (AF); Daiichi Sankyo: Pharmacology and Pharmacotherapy, Other.
G.F.: (2021) Direct personal payment from healthcare industry: speaker fees, honoraria, consultancy, advisory board fees, investigator, committee member, etc. Libbs: Pharma—antiarrhythmic drugs; Daiichi Sankyo: Pharma—DOACs.
(2023) Payment from healthcare industry: speaker fees, honoraria, consultancy, advisory board fees, investigator, committee member, etc., including travel funding related to these activities. Libbs Pharma: Arrhythmias, General.
J.J.: (2021) Payment from healthcare industry to your department or institution or any other body for your personal services: speaker fees, honoraria, consultancy, advisory board fees, investigator, committee member, etc. Stembiosys: Scientific Advisory Board. Direct ownership of shares or direct financial interest in healthcare, media, education companies or in companies related to (suppliers), or in competition with the ESC and its mission: to reduce the burden of cardiovascular disease. Healthcare—ARRHYTHMIAS—Direct ownership of shares in Corify and Stembiosys.
G.Y.L.: (2021) Payment from healthcare industry to your department or institution or any other body for your personal services: speaker fees, honoraria, consultancy, advisory board fees, investigator, committee member, etc. Daiichi-Sankyo: Anticoagulation; Boehringer Ingelheim: Anticoagulation; Registries; Steering Committees; Pfizer: Anticoagulation; Registries; BMS: Antithrombotic therapy. Research funding from healthcare industry under your direct/personal responsibility (to department or institution). Boehringer-Ingelheim: AF registries [unrestricted educational grant]. Any other interest (financial or otherwise) that should be declared in view of holding an ESC position. Shares in private limited company (a legal separate entity in UK), but no salary/dividends/income/personal renumeration received.
(2023) Research funding from healthcare industry received by your department/institution or an entity controlled by your department/institution, under your direct/personal responsibility. Boehringer-Ingelheim: AF registries [unrestricted educational grant]. Academic, non-promotional research work on registries by fellow, Principal Investigator. Anthos: AF registries [unrestricted educational grant]. Academic, non-promotional research work on registries by fellow, Principal Investigator. Any other interest (financial or otherwise) that should be declared in view of holding an ESC position (e.g. editorial role in non-ESC publications—editor in chief or other editorial position—and/or officer position in other organisations). Shares in private limited company (a legal separate entity in UK), but no salary/income/personal renumeration received.
G.M.: (2021) Direct personal payment from healthcare industry: speaker fees, honoraria, consultancy, advisory board fees, investigator, committee member, etc. Johnson and Johnson: Anticoagulation for atrial fibrillation; InCarda: Novel antiarrhythmic drug delivery system. Research funding from healthcare industry under your direct/personal responsibility (to department or institution). Baylis Medical: Transseptal puncture procedures.
(2023) Payment from healthcare industry: speaker fees, honoraria, consultancy, advisory board fees, investigator, committee member, etc., including travel funding related to these activities. InCarda: Atrial Fibrillation (AF).
K.M.: (2021) Receipt of royalties for intellectual property. Metabolic Technologies Inc: Pharmaceutical company. Research funding from healthcare industry under your direct/personal responsibility (to department or institution). NIH: American Heart Association: Atrial fibrillation.
(2023) Receipt of royalties for intellectual property. Metabolic Technologies Inc: Atrial Fibrillation (AF), Basic Science.
S.N.: (2021) Direct personal payment from healthcare industry: speaker fees, honoraria, consultancy, advisory board fees, investigator, committee member, etc. Johnson & Johnson: Inflammation in Heart Cellws; GlaxoSmithKline: Novel AF Therapies; LQT Therapeutics: Novel Long QT therapies.
(2023) Any other interest (financial or otherwise) that should be declared in view of holding an ESC position (e.g. editorial role in non-ESC publications—editor in chief or other editorial position—and/or officer position in other organisations). Editor-in-Chief, Canadian Journal of Cardiology Section Editor, Rhythm Disorders, JACC.
U.S.: (2023) Ongoing research funding from public and/or not-for-profit organisations (which started in 2023 or continues in 2023). This information is not mandatory and does not impact one's ability to join an ESC activity. Dutch Heart Foundation, EP Solutions, European Union, Roche.
Payment from healthcare industry: speaker fees, honoraria, consultancy, advisory board fees, investigator, committee member, etc., including travel funding related to these activities. YourRhythmics: Atrial Fibrillation (AF). Direct financial interest in healthcare, media, education companies, or in companies related to or in competition with the ESC and its mission: to reduce the burden of cardiovascular disease. Healthcare—Atrial Fibrillation (AF)—Shareholder of YourRhythmics BV.
L.S.-D.: (2021) Declaration not submitted.
(2023) Payment from healthcare industry: speaker fees, honoraria, consultancy, advisory board fees, investigator, committee member, etc., including travel funding related to these activities. Pfizer: Atrial Fibrillation (AF), Acute Heart Failure; Bristol Myers Squibb: Atrial Fibrillation (AF), Acute Heart Failure.
T.Y.: (2021) Direct personal payment from healthcare industry: speaker fees, honoraria, consultancy, advisory board fees, investigator, committee member, etc. Bristol Myers Squibb, Bayer, Boehringer-Ingelheim, Daiichi Sankyo, Takeda Pharmaceuticals, Otuska Pharmaceutical: atrial fibrillation; Abbott, Medtronic, Japan lifeline: catheter ablation. Payment from healthcare industry to your department or institution or any other body for your personal services: speaker fees, honoraria, consultancy, advisory board fees, investigator, committee member, etc. Abbott: catheter ablation. Research funding from healthcare industry under your direct/personal responsibility (to department or institution). Boston Scientific Japan, Japan lifeline, Abbott Medical Japan, Nihon Kohden Corpatation, Japan Medtronic, Fides-ONE Corporation: arrhythmia management.
(2023) Payment from healthcare industry: speaker fees, honoraria, consultancy, advisory board fees, investigator, committee member, etc., including travel funding related to these activities. Abbott, Novartis, NIHON KOHDEN: Arrhythmias, General; Bayer, Daiichi Sankyo, Medtronic, Bristol Myers Squibb: Atrial Fibrillation (AF). Research funding from healthcare industry received by your department/institution or an entity controlled by your department/institution, under your direct/personal responsibility. Abbott, Boston Scientific, Medtronic, NIHON KOHDEN, Japan lifeline, Fides-One corporation: research funding for atrial cardiomyopathy, atrial fibrillation, and heart failure, principal investigator.
A.G.: (2021) Direct personal payment from healthcare industry: speaker fees, honoraria, consultancy, advisory board fees, investigator, committee member, etc. Abbott, Boston Scientific, Medtronic: Ablation; Boehringer-Ingelheim, Daiichi Sankyo, Pfizer, Bayer Healthcare, Bristol Myers Squibb: anticoagulation; Berlin Chemie AG: antiischemic drugs; OMEICOS: Atrial fibrillation; Viofor: Heart Failure; Astra Zeneca: PCI, antiplatelets.
(2023) Payment from healthcare industry: speaker fees, honoraria, consultancy, advisory board fees, investigator, committee member, etc., including travel funding related to these activities. Daiichi Sankyo, Bristol Myers Squibb: Atrial Fibrillation (AF); Medtronic: Device Therapy; Boston Scientific, Bayer Healthcare: History of Cardiology, Chronic Heart Failure.
K.Z.: (2021) Research funding from healthcare industry under your direct/personal responsibility (to department or institution). Biosense Webster: Research electrophysiology.
(2022) Payment from healthcare industry to your department or institution or any other body for your personal services: speaker fees, honoraria, consultancy, advisory board fees, investigator, committee member, etc. Biosense Webster: Ventricular Arrhythmias and Sudden Cardiac Death (SCD). Research funding from healthcare industry under your direct/personal responsibility (to department or institution). Biosense Webster: investigator initiated research, PI.
(2023) Payment from healthcare industry to your department or institution or any other body for your personal services: speaker fees, honoraria, consultancy, advisory board fees, investigator, committee member, etc., including travel funding related to these activities. Biosense Webster: Ventricular Arrhythmias and Sudden Cardiac Death (SCD). Research funding from healthcare industry received by your department/institution or an entity controlled by your department/institution, under your direct/personal responsibility. Biosense Webster: Investigator initiated research, ventricular arrhythmias, PI.
N.A.: (2022) Nothing to be declared.
(2023) Ongoing research funding from public and/or not-for-profit organisations (which started in 2023 or continues in 2023). This information is not mandatory and does not impact one's ability to join an ESC activity. National Institutes of Health.
R.T.A.: (2022) Travel and meeting support from healthcare industry, independent of the above activities. Spectranetics: Device Therapy.
(2023) Payment from healthcare industry: speaker fees, honoraria, consultancy, advisory board fees, investigator, committee member, etc., including travel funding related to these activities. Daiichi Sankyo: Clinical Skills; Boston Scientific, Biotronik, Spectranetics: Device Therapy.
T.F.A.: (2022) Research funding from healthcare industry under your direct/personal responsibility (to department or institution). Biosense Webster: Investigator-initiated trial on High power-short duration RF ablatoin (QDOT), Principal Investigator.
(2023) Ongoing research funding from public and/or not-for-profit organisations (which started in 2023 or continues in 2023). This information is not mandatory and does not impact one's ability to join an ESC activity. German Research Foundation. Payment from healthcare industry: speaker fees, honoraria, consultancy, advisory board fees, investigator, committee member, etc., including travel funding related to these activities. CorifyCare: Atrial Fibrillation (AF). Funding from healthcare industry for travel to, and attendance of events and meetings, unrelated to the activities listed in 2.A and 3.A. Biosense Webster: Arrhythmias, General.
J.D.: (2022) Direct personal payment from healthcare industry: speaker fees, honoraria, consultancy, advisory board fees, investigator, committee member, etc. Astra Zeneca, Medtronic: Device Therapy, Chronic Heart Failure; Boston Scientific: Other. Travel and meeting support from healthcare industry, independent of the above activities. Boston Scientific: Other.
(2023) Payment from healthcare industry: speaker fees, honoraria, consultancy, advisory board fees, investigator, committee member, etc., including travel funding related to these activities. Astra Zeneca: Chronic Heart Failure; Medtronic: Device Therapy, Chronic Heart Failure; Boston Scientific: Other. Funding from healthcare industry for travel to, and attendance of events and meetings, unrelated to the activities listed in 2.A and 3.A. Boston Scientific: Other.
J.-B.G.: (2022) Direct personal payment from healthcare industry: speaker fees, honoraria, consultancy, advisory board fees, investigator, committee member, etc. Abbott Laboratories: Arrhythmias, General, Atrial Fibrillation (AF), Device Therapy; Microport: Arrhythmias, General, Atrial Fibrillation (AF), Supraventricular Tachycardia (Non-Atrial Fibrillation), Device Therapy. Travel and meeting support from healthcare industry, independent of the above activities. Abbott Laboratories: six items checked.
(2023) Payment from healthcare industry: speaker fees, honoraria, consultancy, advisory board fees, investigator, committee member, etc., including travel funding related to these activities. Abbott: Arrhythmias, General, Atrial Fibrillation (AF), Syncope and Bradycardia, Ventricular Arrhythmias and Sudden Cardiac Death (SCD).
J.K.: (2022) Research funding from healthcare industry under your direct/personal responsibility (to department or institution). Biosense Webster, Medtronic: Fellowship and AF research support, Principle investigator; Zoll Medical: Randomised trial of CPAP treatment for sleep apnoea patients with AF, Principal investigator.
(2023) Payment from healthcare industry to your department or institution or any other body for your personal services: speaker fees, honoraria, consultancy, advisory board fees, investigator, committee member, etc., including travel funding related to these activities. Biosense Webster: Atrial Fibrillation (AF). Research funding from healthcare industry received by your department/institution or an entity controlled by your department/institution, under your direct/personal responsibility. Zoll Medical: Atrial fibrillation and sleep apnoea, Chief investigator; Biosense Webster: Atrial fibrillation mapping and ablation, Principal investigator; Medtronic: Atrial fibrillation mapping, Chief investigator; Abbott: Atrial fibrillation PFA ablation, Principal investigator. Any other interest (financial or otherwise) that should be declared in view of holding an ESC position (e.g. editorial role in non-ESC publications—editor in chief or other editorial position—and/or officer position in other organisations). Associate editor Journal of the American College of Cardiology-Cardiac Electrophysiology Chair of the Heart Rhythm Council, Cardiac Society of Australia and New Zealand.
H.L.: (2022) Direct personal payment from healthcare industry: speaker fees, honoraria, consultancy, advisory board fees, investigator, committee member, etc. Abbott: Arrhythmias, General. Research funding from healthcare industry under your direct/personal responsibility (to department or institution). Abbott, Medtronic: Research Funding for Electrophysiology Fellow, Supervisor.
(2023) Payment from healthcare industry: speaker fees, honoraria, consultancy, advisory board fees, investigator, committee member, etc., including travel funding related to these activities. Abbott: Arrhythmias, General. Research funding from healthcare industry received by your department/institution or an entity controlled by your department/institution, under your direct/personal responsibility. Abbott, Medtronic: Research Funding for Electrophysiology Fellow, Supervisor.
E.L.A.A., S.C., S.H., Y.-J.L., H.-N.P., N.T., S.A.J.: (2021, 2023) Nothing to be declared.
J.J., D.C.: (2023) Nothing to be declared.
Data availability
No new data were generated or analysed in support of this research.
References
Author notes
Developed in partnership with the European Heart Rhythm Association (EHRA), (a registered branch of the European Society of Cardiology (ESC)) the Heart Rhythm Society (HRS), the Asia Pacific Heart Rhythm Society (APHRS), the Latin America Heart Rhythm Society (LAHRS).
Conflict of interest: L.M.: (2022) Direct personal payment from healthcare industry: speaker fees, honoraria, consultancy, advisory board fees, investigator, committee member, etc. Biosense Webster: Atrial Fibrillation (AF); Boston Scientific, Medtronic, Abbott Medical: Atrial Fibrillation (AF), Device Therapy. Research funding from healthcare industry under your direct/personal responsibility (to department or institution). Johnson & Johnson: Research projects, PI; Boston Scientific, Medtronic, Biotronik, Abbott Medical: Research projects, PI.