-
PDF
- Split View
-
Views
-
Cite
Cite
Feng Sun, Ya Fang, Man-Man Zhang, Rui-Jia Zhang, Feng-Yao Wu, Rui-Meng Yang, Ping-Hui Tu, Mei Dong, Shuang-Xia Zhao, Huai-Dong Song, Genetic Manipulation on Zebrafish duox Recapitulate the Clinical Manifestations of Congenital Hypothyroidism, Endocrinology, Volume 162, Issue 8, August 2021, bqab101, https://doi.org/10.1210/endocr/bqab101
- Share Icon Share
Abstract
Congenital hypothyroidism (CH) is a highly prevalent but treatable neonatal endocrine disorder. Thyroid dyshormonogenesis is the main cause of congenital hypothyroidism in Chinese CH patients, and DUOX2 is the most frequent mutated gene involved in H2O2 production. In humans, the primary sources for H2O2 production are DUOX1 and DUOX2, while in zebrafish there is only a single orthologue for DUOX1 and DUOX2. In this study, duox mutant zebrafish were generated through knockdown duox by morpholino or knockout duox by CRISPR Cas9. The associated phenotypes were investigated and rescued by thyroxine (T4) treatment. Mutant zebrafish displayed hypothyroid phenotypes including growth retardation, goiter and, infertility. Homozygous mutants in adults also displayed extrathyroidal abnormal phenotypes, including lacking barbels, pigmentation defects, erythema in the opercular region, ragged fins, and delayed scales. All these abnormal phenotypes can be rescued by 10 nM T4 treatment. Strikingly, the fertility of zebrafish was dependent on thyroid hormone; T4 treatment should be continued and cannot be stopped over 2 weeks in hypothyroid zebrafish in order to achieve fertility. Thyroid hormones played a role in the developing and maturing of reproductive cells. Our work indicated that duox mutant zebrafish may provide a model for human congenital hypothyroidism.
Congenital hypothyroidism (CH), caused by thyroid hormone deficiency present at birth, is the most common neonatal endocrine disorder and occurs in 1:3000 to 4000 live births (1-3). If untreated, CH can cause several growth retardation and intellectual disabilities. The etiology of this disease can be divided into 2 groups: thyroid dysgenesis (abnormal differentiation, migration, or growth of the thyroid gland) and thyroid dyshormonogenesis (disruption of thyroid hormone biosynthesis) (4). Interestingly, different from the universally held opinion that thyroid dysgenesis accounts for 85% of CH cases and dyshormonogenesis accounts for the remaining 15% of CH cases (1, 5), our previous molecular studies have found that dyshormogenesis was the main cause of CH in Chinese patients and DUOX2 was the most common mutated gene in dyshormogenesis (6).
Dual oxidase (DUOX) belongs to the NOX family, and was originally identified as thyroid NADPH oxidase (NOX), which is involved in the production of reactive oxygen species (ROS) in response to various extracellular signals and was first cloned by Dupuy et al. (7). In humans, there are 2 DUOX genes, DUOX1 and DUOX2, which are located in opposite transcriptional orientations on chromosome 15 (15q15.3) and share 83% sequence similarity (7-9). The DUOX2 gene consists of 34 exons and encodes a 1548 amino acid polypeptide located at the apical membrane of the thyrocytes, which mediate hydrogen peroxide (H2O2) generation with the help of its maturation factors to form functional heterodimeric complexes (10). The generation of H2O2 is a critical step in the synthesis of thyroid hormones. The complex DUOX2/DUOXA is devoted to producing H2O2, which is used as a substrate by thyroid peroxidase in the incorporation of iodine into thyroglobulin, an essential step of thyroid hormonogenesis known as organification (11, 12).
To date, various approaches have been adopted to induce hypothyroidism in animal models including surgical removal of the thyroid gland, thyroid gland removal via radioactive iodine isotope (131I), dietetic reduction of iodine, and administration of substances (propylthiouracil or methimazole) (13). For example, methimazole/sodium perchlorate/low-iodine diet treatment can lead to chronic hypothyroidism in mice (14). Another important approach to induce hypothyroidism is through genetic manipulation. At present, missense mutation in genes related CH can induce hypothyroidism in animal models. Mice with a spontaneous missense Duox2 mutation have been described and suffer from congenital hypothyroidism (15), similar to combined DuoxA1/DuoxA2 KO mice (16, 17).
Zebrafish models are widely used for a variety of human diseases because zebrafish possess unique features that make them particularly well suited for experimental and genetic analysis of vertebrate development, such as a short reproductive cycle, a large numbers of embryos, and the availability of several genetic manipulation strategies (18). In addition, the zebrafish thyroid is composed of single follicles localized along the ventral aorta instead of organized as a compact gland, as found in mammals (19). As a consequence, this model has been extensively used to study the molecular mechanism underlying alteration in thyroid development and function (20). Moreover, a molecular evolutionary study has identified only a single zebrafish duox gene, in contrast to 2 loci, Duox1 and Duox2, in mammals (21). In consideration of these advantages, zebrafish would be a preferred model that could recapitulate the clinical manifestations of CH.
Based on the above reasons, in this study, we examined the in vivo roles of duox using morpholino and CRISPR Cas9 in a vertebrate animal model of zebrafish (Danio rerio). Our results showed that both duox knockdown and duox KO zebrafish exhibited several phenotypes associated with CH in humans, and CH phenotypes could be rescued by T4 treatment.
Materials and Methods
Zebrafish Care
Zebrafish breeding and maintenance were carried out under standard conditions at 28.5°C. We used Tübingen background wildtype (WT) zebrafish. Animal stages were designated as hours post fertilization (hpf) and days post fertilization (dpf). In the rescue experiment for duox knockdown embryos, a 5-day treatment with different concentrations of T4 (1 nM, 5 nM, 10 nM, 50 nM, 100 nM) was added. In the rescue experiment for duox KO embryos, a 3-month treatment with 10 nM T4 prepared in dimethyl sulfoxide (Sigma-Aldrich) was sustained. Briefly, tanks with zebrafish that needed T4 treatment were removed from the system at 22:00 and 10 nM T4 was added for protection from degradation due to light exposure. Then the treated zebrafish were transferred into new tanks with fresh water at 8:00 the next morning and tanks were placed back into the system. All experiments were approved by our institution’s animal care and use committee.
Injection of Morpholinos
duox ATG morpholino was designed and purchased from Gene Tools LLC: duox (ATG): 5′-TGAACCCATTCTCGCAGACCAATGA-3′, the standard control SCMO: 5′- CCTCTTACCTCAGTTACAATTTATA-3′ was purchased from Gene Tools LLC. Morpholinos were diluted in H2O containing 0.05% phenol red; the duox morpholino and standard control morpholino were injected at the 1-cell stage with 5.6 ng per embryo.
Generation of duox Mutant Zebrafish by CRISPR/Cas9
The detailed procedure for CRISPR/Cas9 editing in zebrafish was described previously (22, 23). The duox target in this study was 5′-GGACTGACTCTTGCGCCCCC-3′. gRNAs were transcribed using the DraI-digested gRNA expression vectors as templates and the MAXIscript T7 kit (Life Technologies). The Cas9 mRNA was transcribed using PmeI-digested Cas9 expression vector and the mMESSAGE mMACHINE T7 ULTRA kit (Life Technologies). Following completion of transcription, the poly (A) tailing reaction and DNase I treatment were performed according to the manufacturer’s instructions. Both the gRNA and the Cas9-encoding mRNA were then purified by LiCl precipitation and redissolved in RNase-free water. Injection mixtures included 500 pg of Cas9 mRNA, and 100 pg of gRNA were coinjected into the 1-cell stage zebrafish embryos. Then the injected embryos were grown. When these zebrafish reached sexual maturity, caudal fins were cut for extracting genomic DNA (see below). Targeted genomic loci were amplified from genomic zebrafish DNA using primers (see below) designed to anneal approximately 260 base pairs and were sequenced for indel occurrence. Forty zebrafish were screened to identify a founder, and the germline mutation frequency was approximately 25%. Mutant sites were verified by comparison with the WT unaffected sequences (chimerism). Chimeric zebrafish were mated onto a Tu background to obtain duox+/− zebrafish. We crossed duox+/− males and duox+/− females to obtain duox+/+, duox+/−, and duox−/− litter mates for all experiments of phenotypic analyses.
PCR and Genotyping
Genomic DNA was extracted from caudal fin clips or whole larvae in a thermal cycler using lysis buffer. The conditions for this procedure were 10 minutes at 98°C and 4°C, +∞. Then caudal fin clips or whole larvae in lysis buffer were digested with proteinase K solution (100 µg/mL) overnight at 55°C in a water bath. Polymerase chain reaction (PCR) was performed using Taq DNA polymerase (LaiFeng) with the following genotyping primer pairs: forward: duox genotype-F 5′-ACTCACAGATGGCAGCTCTT-3′; duox genotype-R 5′-ACAGACCCCTTTGAACCCTT-3′. The PCR products were sequenced for genotyping.
RNA Isolation and Real-time PCR
Total RNA was extracted from pooled whole zebrafish embryos (10 embryos/tube) using TRIzol reagent (Invitrogen), and 1 μg of total RNA was reverse transcribed into cDNA using a PrimeScript™ RT Reagent Kit with gDNA Eraser (TaKaRa). Amplification was carried out using SYBR Premix Ex Taq (TaKaRa) on the ABI QuantStudio 12K Flex Real-Time PCR System (Life Technologies). Relative gene expression was normalized to that of β-actin. Primer pairs were the following: forward: tshba-F: 5′-CAATTACTGTGTGGCTGTCAAC-3′; reverse: tshba-R 5′-CGGTACTCAACTTCCTGATAA-3′; forward: tg-F: 5′-CTTACCTGAAAACGCTGCTTAT-3′; reverse: tg-R 5′-GGGTGAATGTTTTCTCCTTGTA-3′; forward: β-actin-F 5′-CGAGCTGTCTTCCCATCCA-3′; reverse: β-actin-R 5′-TCACCAACGTAGCTGTCTTTCTG-3′. Gene expression data were analyzed by 1-way ANOVA and unpaired Student’s t-test.
Whole Mount In situ Hybridization
For whole mount in situ hybridization (WISH), embryos at 5 dpf were collected and fixed overnight in 4% paraformaldehyde at 4°C. Fixed larvae were washed in PBST (phosphate-buffered saline supplemented with 0.1% Tween 20), then washed in modified destaining solution (10 mL of destaining solution included 5.67 mL of H2O, 3.33 mL of 30% H2O2, and 1 mL of 50% formamide/2× standard saline citrate) that prevents embryonic pigmentation without side effects on TH synthesis. The samples were dehydrated in graded PBST methanol solutions (3:1, 1:1, 1:3) for 10 minutes each and stored in absolute methanol at –20°C. Dehydrated embryos were rehydrated in graded PBST/methanol solutions (1:3, 1:1, 3:1) for 10 minutes each, and then rinsed twice in PBST for 10 minutes each at room temperature (RT). Embryos at 5 dpf were treated with proteinase K solution (100 µg/mL) at RT for 30 minutes, rinsed twice in PBST, and refixed in 4% paraformaldehyde at RT for 30 minutes and hybridized with digoxigenin-labeled antisense RNA probes at 68°C (24, 25). After hybridization, the embryos were washed through a graded series of standard saline citrate (SSC, which is a solution composed of 3 M Sodium chloride and 300 mM tri-Sodium citrate dihydrate, pH 7.0) (2× SSC containing 50% formamide, 2x SSC, 0.2x SSC), for 30 minutes at each concentration. Larval specimens were then incubated overnight with antidigoxigenin-AP Fab fragments diluted 1:10 000 (Roche) at 4°C. Finally, signals were visualized by incubation in BCIP/NBT solution (Vector Laboratories). Whole-mount specimens were observed and photographed under an SMZ25 dissecting microscope (Nikon) and documented using NIS-Elements BR 4.50.00 software (Nikon).
Morphometric Analysis
To analysis the survival rate, malformation rate, and hatch rate between duox morphants and control, 600 embryos were injected with standard control morpholino (SD) and 750 embryos were injected duox morpholino (MO). HTA (head–trunk angle) is the angle between the head axis (middle of the ear and eye) and the body axis (along the notochord and parallel to the horizontal myosepta at about somites 5-10), which increases with the stage of development as described by Walpita et al. (26). For measuring HTA at 30 hpf, embryos were hatched manually and were anesthetized in 160 mg/L tricaine methanesulfonate (MS222, Sigma), then larvae were fixed in 3% methylcellulose and placed in lateral position, images were taken and measured by SMZ25 dissecting microscope (Nikon) using NIS elements software to estimate the HTA. For measuring heart rate, embryos at 48 hpf were collected and captured on a 30-second video using a SMZ25 dissecting microscope (Nikon); the heart rate (beats per minute, bpm) was counted manually and multiplied by 2. For measurements of total body length, eye size, and ear size at 5 dpf, larvae were anesthetized in 160 mg/L tricaine methanesulfonate (MS222, Sigma); larvae were fixed in 3% methylcellulose and placed in the lateral position, images were taken, and measured using a SMZ25 dissecting microscope (Nikon) with NIS elements software to estimate the sizes with micrometer precision. Total length was measured as the length of the line between mouth and tip of the tail. The eye and ear areas were measured using NIS elements software as the index for eye size and ear size. For measuring body length and body weight in adult zebrafish, the WT, duox−/− zebrafish and T4 rescued zebrafish were anesthetized in 160 mg/L tricaine methanesulfonate (MS222, Sigma). The body weight and body length (forehead to tail fin) were respectively measured using an analytical balance (minimum scale 0.001 g) and a ruler (minimum scale 1 mm) (27). Morpholinos were injected into embryos at least 3 times and all the above experiments were performed each time. All these parameters were represented as mean ± standard error of the mean.
Immunohistochemistry
Adult zebrafish were fixed whole in 4% paraformaldehyde (PFA) overnight, followed by a decalcification step in 20% EDTA, for 20 days. For characterizing the thyroid follicle, heads were cut at heart level and processed in a Leica EG 1150H tissue processor in preparation for paraffin embedding. The embedding station used was a Leica EG1150H. Samples were cut in horizontal sections at 7 μm thicknesses on a microtome (Thermo Fisher Scientific, microm HM325). Female and male gonads were dissected, fixed in 4% PFA overnight. Sections were prepared for paraffin embedding and sectioned at 10 μm thicknesses. Sections were arranged and held on Superfrost Plus™ slides (Thermo Fisher Scientific). Alternating sections were then taken forward for hematoxylin and eosin (H&E) staining.
Determination of Whole-body Content of Total Thyroid Hormones in Zebrafish
The concentrations of total T4 (TT4) and total triiodotyrosine (TT3) were performed as previously described but with a minor modification (28). Briefly, 200 larvae at 5 dpf were placed in Teflon tubes on ice. The larvae were homogenized in 200 μL of ice-cold PBS using an Ultra-Turrax T8 basic homogenizer (IKA, Staufen, Germany) and sonicated (Sonics and Materials Vibra-Cell at 50% output for 20 seconds). Homogenized samples were centrifuged at 13 000 rpm for 30 minutes at 4°C; supernatant was collected for thyroid hormone measurements. TT4 and TT3 concentrations were measured in duplicate using commercial enzyme-linked immunosorbent assay (ELISA) kits (Cloud Clone Corp, WuHan, China, product no. CEA453Ge for T3 and product no. CEA452Ge for T4) according to the manufacturer’s instructions. Standard curves, and concentrations of TT3 and TT4 were calculated using online analysis software (www.elisanalysis.com). The reported detection ranges for TT4 and TT3 are 3.70 to 300 ng/mL and 123.5 to 10 000 pg/mL respectively. Each group was in triplicate. Thyroid hormone concentrations were represented as the mean from pooled samples of 200 larvae at 5 dpf as previously reported (29, 30).
Statistical Analysis
Continuous, normally distributed data were expressed as the mean ± standard error of the mean. Intergroup comparisons were performed using the Student unpaired t-test (normally distributed data). The D’Agostino and Pearson test was used to check for Gaussian distribution using SPSS 23. P < .05 was considered statistically significant. Diagrams were made using GraphPad Prism 6.
Result
In Vivo Modeling of duox Knockdown Results in Congenital Hypothyroidism Phenotypes
Conservative analysis of DUOX2 protein in humans and Duox protein in zebrafish showed 54% homology in amino acid sequences. In order to investigate the function of duox in vivo, we developed zebrafish models. To test the function of the gene in zebrafish, 1 antisense translation block morpholino was designed complementary to the region of the duox transcript. In order to select the most efficient morpholino concentration to achieve duox knockdown with minimal toxicity, we injected embyros with different concentrations of duox morpholino. We found that 5.6 ng of duox morpholino injected into embryos can lead to the hypothyroid phenotype with minimal toxicity. A higher morpholino concentration (8.4 ng) induced a higher malformation rate and lower survival rate, while lower morpholino concentrations (1.05 ng and 2.1 ng) could not induce the hypothyroid phenotype (Fig. 1 (31)). After injection of 5.6 ng of duox ATG MO, we found that survival rates at 24 hpf, 48 hpf, and 72 hpf, and hatch rates at 48 hpf and 72 hpf were slightly lower than standard control MO-injected embryos; meanwhile, the malformation rate was slightly higher at 48 hpf and 72 hpf compared with standard control (Fig. 1A-1C). We also compared morphological differences between duox knockdown and control larvae and we found that the HTA, as a function of hours of development, was smaller at 30 hpf in duox knockdown embryos than in control, which indicated delayed development in duox knockdown embryos (Fig. 1D). In addition, duox knockdown embryos showed slower heart rate at 48 hpf (Fig. 1E), shorter body length, and smaller eye and ear size (Fig. 1F-1H) at 5 dpf than controls. In order to confirm that the phenotypes in duox knockdown embryos were congenital hypothyroidism, we detected thyroid hormone level by ELISA and found that the T4 and T3 levels were markedly decreased in duox knockdown embryos compared with control at 5 dpf (Fig. 1I and 1J). We furtherly determined tsh and tg expression by Q-PCR and in situ hybridization, and found mRNA expression of tsh and tg significantly increased in duox knockdown embryos compared with control (Fig. 1K-1M). These data indicate that duox knockdown by morpholino resulted in phenotypes of congenital hypothyroidism which were consistent with clinical characteristics of patients with CH.
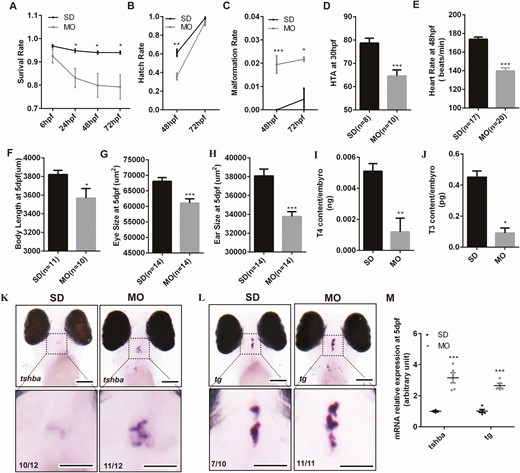
The hypothyroidism phenotypes in zebrafish after duox knockdown by morpholino. The survival rate (A), malformation rate (B), hatch rate (C) between morpholinos (n = 750) and control(n = 600). (D,E) The morphological differences between duox knockdown and control larvae. (D), HTA; (E), heart rate; (F), body length; (G), eye size; (H), ear size; (I,J) thyroid hormone level at 5 dpf larvae by ELISA. Two hundred larvae at 5 dpf for each group were collected in a tube to determine the thyroid hormone level. Each group was in triplicates. (I), T4 level; (J), T3 level; K-M, tsh and tg mRNA expression at 5 dpf between morpholinos and control (K,L) by in situ hybridization and by Q-PCR (M). SD, embryos injected with standard control morpholino. MO, embryos injected with duox morpholino. *P < .05; **P < .01; ***P < .001. Scale bars: 100 μm.
Considering the good response of CH patients to T4 treatment, we wonder whether supplementation of aquarium water with T4 could reverse the phenotypes observed in the duox knockdown mutants. We treated morphants with different concentrations of T4 (5 nM, 10 nM, 50 nM, 100 nM) from 10 hpf, and we found morphants treated with 10 nM T4 can rescue the hypothyroid phenotype. In detail, the HTA at 30 hpf, body length at 5 dpf, and tsh and tg mRNA expression levels in morphants treated with 10 nM T4 can recover to normal levels (Fig. 2 (31)).
Molecular Characteristic of duox Mutant
The morpholino approach was a transient method to the knockdown gene, which could not persist to adult. In order to establish a stable zebrafish line with duox dysfunction, we targeted KO duox of zebrafish by CRISPR/Cas9 and established a 7 bp deletion mutant line for further study (Fig. 2A). The 7 bp deletion resulted in a loss of function by premature termination after the 352th amino acid during Duox protein translation and generated a truncated protein, including the loss of the 2 critical C-terminal NADPH and FAD binding sites, that would be expected to be loss-of-function mutations (Fig. 2B-C). Genotyping of this mutant can be performed via genomic PCR.
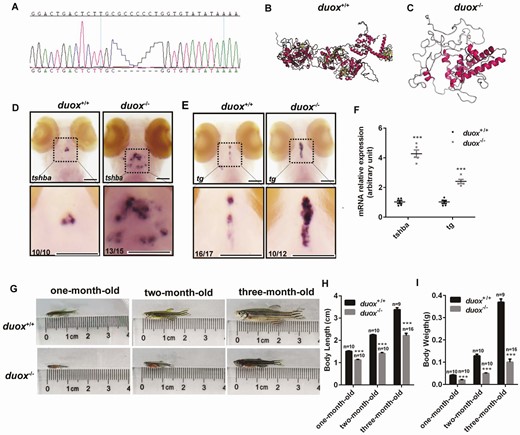
The hypothyroidism phonotype in zebrafish after duox knockout by CRISPR Cas9. (A) A 7 bp deletion mutant line was established verifying by Sanger sequencing. (B,C) Three-dimensional structure of duox protein in WT (B) and duox homozygous mutant line (C). (D-F) tsh and tg mRNA expression at 5 dpf between morpholinos and control by in situ hybridization (D,E) and Q-PCR (F). (G-I) Growth development in duox homozygous mutant and WT (G) including body length (H) and body weight (I). Scale bars: 100 μm.
duox Knockout Mutants Exhibited Phenotypes of Congenital Hypothyroidism
To verify that the duox defect would lead to CH, we analyzed the expression of CH-related genes in duox KO larvae and WT siblings. We performed in situ hybridization to detect mRNA expression of tsh and tg in 5 dpf larvae from duox+/– mating. After photographing whole-mount specimens, the genotype of specimens was identified by PCR and Sanger sequencing. We found that mRNA expression of tsh and tg was significantly increased in KO larvae compared with WT siblings (Fig. 2D and 2E). For detecting mRNA expression of tsh and tg by Q-PCR, we cut the tail of embryos at 3 dpf for genotyping (embryos were still alive after cut tails); the short-tailed embryos were collected at 5 dpf based on the results of genotyping. The results of Q-PCR were consistent with in situ hybridization (Fig. 2F) and were in agreement with the duox knockdown data. To compare morphological differences between WT and duox KO larvae, we measured the body length of duox KO larvae and WT siblings at different stages and wondered how early in development the duox KO mutant exerts its deleterious effects. Interestingly, no apparent growth defect was detected in larvae between the 2 groups at the earlier age before 20 dpf, which was contradicted in duox knockdown larvae. However, duox KO zebrafish exhibited growth retardation resulting in short body length at later developmental stages (from 20 dpf) (Fig. 3 (31)). The phenotypes of impaired growth emerged at the larva–juvenile transition and persisted to adulthood. Quantification of body length and body weight in 1-month-old, 2-month-old, and 3-month-old zebrafish demonstrated that duox KO zebrafish were shorter in body length and lighter in body weight than WT, and the difference became more and more obvious with the development of zebrafish (Fig. 2G-I). In addition, we observed that the homozygous duox mutant adult zebrafish displayed external goiter-like growths in the submandibular area (Fig. 3A and 3B). To confirm whether these goiter-like growths were indeed enlarged thyroids, we fixed zebrafish heads and performed H&E staining on horizontal sections to assess the size and number of thyroid follicles. The results showed that there were more and larger thyroid follicles in KO mutants than in WT (Fig. 3C-3F). Therefore, we confirmed that these growths were indeed of thyroid origin.
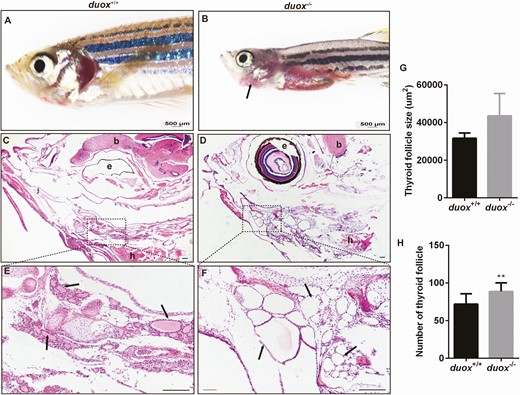
Thyroid morphology in duox mutant and wildtype zebrafish. (A,B) Adult mutant zebrafish exhibited a visible external goiter-like growths (arrows), while wildtype did not. (C-F) Hematoxylin and eosin stain in horizontal sectioning of thyroid follicles (arrows). (G-H0 Quantification of the size of thyroid follicles and the number of thyroid follicles (n = 5, respectively). The size of thyroid follicles was larger and the number of thyroid follicles were more in KO mutants than WT, which showed hyperplastic thyroid follicle in mutant line. e, eye; b, brain; h, heart. scale bars: 500 μm for A-B, 200 μm for C-F; **P < .01.
duox Mutants Exhibited Extrathyroidal Abnormal Phenotypes
Except the hypothyroid phenotype presented in duox mutants, the number of extrathyroidal phenotypes that were distinct from those seen in the WT and heterozygous siblings were also displayed. We found duox KO adults lacked barbels, which are anterior sensory appendages, although these were normally visible in WT siblings (Fig. 4A-B). One apparent phenotype was erythema (redness) in the opercular region of mutants. The redness was apparent in juvenile fish and sustained throughout the lifetime in duox mutants (Fig. 4B). We also found that the erythema allowed for phenotypic identification of homozygous duox mutants from their heterozygous and WT siblings, with 100% accuracy, as confirmed via genotyping. In addition, developmental differences could also be observed in the tail fin. The homozygous duox mutants partially displayed misshapen or damaged fins. We found that more than half exhibited fins similar to the WT (9 out of 16); some mutants showed partial divisive fins (3 out of 16) and 2 out of 16 presented completely divisive fines; others showed damaged fins, which manifested as ragged margins and tears (2 out of 16) (Fig. 4D-4H). The most apparent and significant phenotype was that the homozygous duox mutants were darker than their WT siblings. In zebrafish Danio rerio, the adult pattern comprises dark stripes of black melanophores and a few iridescent iridophores, alternating with light “interstripes” of yellow/orange xanthophores and abundant iridophores, all within the hypodermis, between the epidermis and myotome. The number of melanophores in interstripes was significantly increased in the homozygous duox mutants compared with their WT siblings ((Fig. 4J-K, P). The difference in pigmentation also contributed to phenotypic identification of homozygous duox mutants from their heterozygous and WT siblings. We also found scales developed late in duox mutants and were arranged more densely than in WT siblings (Fig. 4M and 4N). As shown in Fig. 4Q-4R, the distances between the posterior edges of adjacent scales (width of scales) in mutants was shorter and the scale was smaller than that of WT siblings.
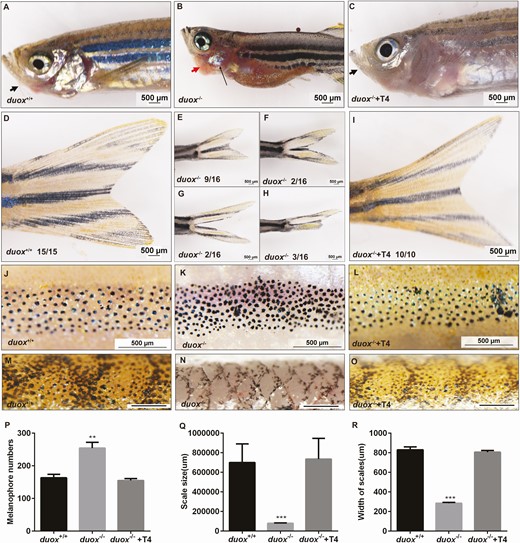
duox–/– mutants exhibited extrathyroidal abnormal phenotypes and could be rescued by T4 treatment. (A-C) duox–/– mutants showed goiter (red arrowheads) and lack barbels (black arrowheads). Erythema in the thoracic region was prominent among duox–/– mutants (black arrow). (D-I) duox–/– mutants partially displayed misshapen or damaged fins. (J-L) flank region showing the distribution of melanophores in WT, duox–/– siblings and duox–/– rescue group. (M-O) Scale morphology showed scales development in duox/– mutants was delayed. (P) Quantification of melanophores. (Q-R) Quantification of the distances between posterior edges of adjacent scales (width of scales) and scale size. All these abnormal phenotypes could be rescued by T4 treatment (C, I, L, O-R). **P < .01, ***P < .001; Scale bars: 500 μm.
T4 Treatment Could Rescue the Hypothyroidism Phenotype and Extrathyroidal Abnormal Phenotypes
Interestingly, we found duox KO mutants were infertile in adulthood, unlike their heterozygous and WT siblings, which were able to breed normally. Female mutants were found not to lay eggs regardless of mating with mutant, heterozygous, or WT males. Similarly, mutant males failed to cross with females, regardless of genotype. Similarly, we used 10 nM T4 to reverse the phenotypes observed in the duox KO mutants as in the morpholino approach. Embryos from duox heterozygous self-crosslinking were firstly classified into 2 groups. One group embryo (contained WT, heterozygous, homozygous) was treated with 10 nM T4 from 10 hpf. At 1 month old, all T4-treated zebrafish were genotyped and homozygous mutants continued with T4 treatment while the WT and heterozygous zebrafish were discarded. The other group of embryos (containing WT, heterozygous, homozygous) was normally raised without T4 (Fig. 5A). We observed the phenotype associated with duox loss of function after 3 months of treatment with T4 to consider whether these phenotypes could be reversed. We found body length and body weight were reversed in treated homozygous zebrafish compared with untreated homozygous zebrafish at 2 months old and 3 months old, especially at 3 months old, the body size of treated homozygous zebrafish could be rescued at the same level as the WT siblings (Fig. 5B-5D). Strikingly, we found that mutant males and females were able to spawn with each other after 3 consecutive months of treatment with T4, although the number of eggs was less than that from WT (Fig. 5E), which indicated the fertility of duox KO mutants could be partially rescued after T4 treatment. When we stopped T4 treatment, the fertility gradually decreased. After stopping treatment for 3 weeks, the duox KO mutants were infertile again and fertility could be restored when T4 treatment was continued (Fig. 5E). We crossed T4-treated female KO mutants with male KO mutants, and the fertile eggs were classified into 2 groups (with or without T4 treatment). At 5 dpf, we checked whether the levels of tsh and tg could be normalized following treatment with T4. The results showed that mRNA expression of tsh and tg could be normalized following T4 treatment by Q-PCR (Fig. 5F). Interestingly, the growth development of duox–/–-F3 at a very early age (before 5 dpf) was the same as duox–/–-F2 and WT larvae while after 10 dpf the body length decreased significantly in duox–/–-F3 compared with duox–/–-F2 and WT larvae, while the development of duox–/–-F2 at this stage was not affected (Fig. 5G). We speculated that the dosage of 10 nM T4 replacement may be insufficient, which led to maternal hypothyroidism. Such a consequence may explain the difference between duox–/–-F3 (parents were hypothyroidism) and duox–/–-F2 (parents were euthyroid).
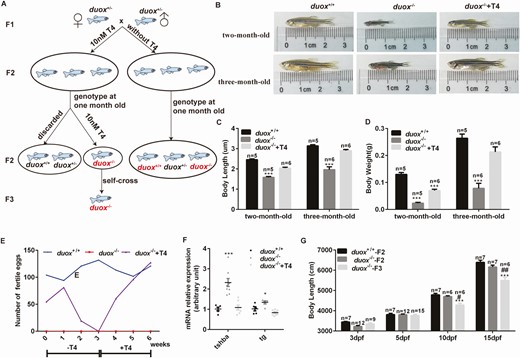
T4 treatment could rescue the phenotype of hypothyroidism in duox–/– mutants. (A) The flow chart of T4 treatment. Embryos from duox heterozygous self-crosslinking were firstly classified into 2 groups. One group embryo (contained wild type, heterozygous, homozygous) was treated with 10 nM T4 at 10 hpf. At 1 month old, all T4 treated zebrafish were genotyped and homozygous mutants were continued with T4 treatment while wildtype and heterozygous were discarded. The other group embryo (contained wildtype, heterozygous, homozygous) was normally raised without T4 and genotyped at 1 month old. Font in red indicates corresponding zebrafish were analyzed and compared. (B-D) body length and body weight were reversed in treated homozygous zebrafish compared with untreated homozygous zebrafish. (E) fertility of duox–/– mutants could also be partially rescued after T4 treatment. T4 treatment should be continued and cannot be stopped over 2 weeks. (F) tsh and tg mRNA expression at 5 dpf by Q-PCR, which showed that the mRNA expression of tsh and tg can be normalized following T4 treatment. (G) body length at different stages. *P < .05, ***P < .001, compared with duox+/+. #P < .05; ##P < .01, compared with duox–/–-F2.
Except the CH-related phenotype in duox KO mutants could be rescued by T4 treatment, extrathyroidal abnormal phenotypes could also be rescued, such as the development of barbels, epidermis, scales, tail fins, and pigment (Fig. 4C, 4I, 4L, and 4O-4R). After T4 treatment, the morphology of duox mutants almost recovered to normal. As a result, we could not distinguish the genotype of the treatment group from the different phenotypes of their WT siblings or heterozygous siblings.
To further investigate the role of duox in fertility, we stained the gonads of zebrafish from each group with H&E. We found that the development of ovary and testis in WT and duox KO with T4-treated zebrafish was similar in adults, and contained various stages of reproductive cells. Oocytes in duox KO mutants were naive and immature, containing only primary oocytes and follicular epithelium oocytes, and lacking mature oocytes (Fig. 6A-C). Testis in duox mutants contained spermatagonia and spermatocytes, and lacked spermatozoa (Fig. 6D-F). These results suggested that the development of reproductive cells in KO mutants was immature, which explains the infertility of KO mutants and the recovery of their fertility after long-term T4 treatment. Thyroid hormones played a role in developing and maturing of reproductive cells.
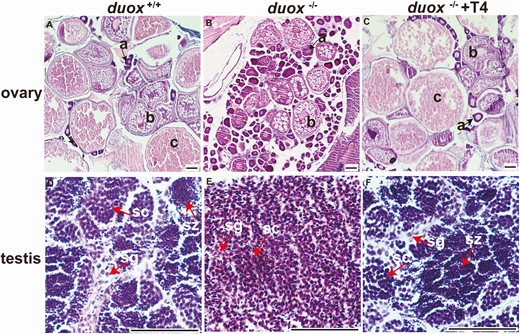
Female and male gonads from zebrafish of each group. (A-C) Female ovarian dysmorphology in 4-month-old zebrafish. (A) adult wild-type ovary stained with hematoxylin and eosin, showing oocytes at various stages of development. (B) Oocytes in duox mutants were naive and immature, which only contained primary oocyte and follicular epithelium oocyte, lack of mature oocyte. (C) Ovarian dysmorphology in duox mutants after T4 rescue was similar as wild-type ovary and contained developing and mature oocytes. (D-F) Male testicular dysmorphology in 4-month-old zebrafish. (D) Adult wild-type testis stained with hematoxylin and eosin, showing spermatogenesis at various stages. (E) Testis in duox mutants only contained spermatagonia and spermatocyte, lack of spermatozoa. (F) Testicular dysmorphology in duox mutants after T4 rescue was similar as wild-type testis. a, primary oocyte (arrows); b, follicular epithelium oocyte; c, mature oocyte; sg, spermatagonia; sc, spermatocyte; sz, spermatozoa. (Scale bars: 50 μm for sections of testis; 100 μm for sections of ovaries).
Discussion
In this study, we generated zebrafish in which the duox gene was knocked down by a morpholino or knocked out by CRISPR Cas9 to understand the deleterious actions of mutant duox during development. Both methodologies showed the hypothyroid phenotype, but some differences existed. Using the morpholino approach, morphants exhibited developmental delay and cardiac edema in embryos and in embryo–larva transition. In contrast to these findings, we did not detect any discernible morphological abnormalities in the embryos and early larvae in homozygous mutant fish. We detected growth impairment of homozygous mutant zebrafish only in the larva–juvenile transition beginning at 20 dpf, which persisted to adulthood. The first visible abnormal development was a hypoplastic epidermis at the larva–juvenile transition of homozygous mutant zebrafish with the appearance of a “‘red belly,’” which persisted to adulthood. It is not immediately clear what may account for the difference between the findings in morphants and the findings in KO mutants in this study. One possibility could be the different methodologies used to induce the expression of mutants in the embryos of zebrafish.
During the completion of this manuscript, many studies have reported the phenotypic consequences of hypothyroidism in adult zebrafish. Among the various adult phenotypes displayed by the homozygous duox mutants, most have been previously observed. Treatment of zebrafish larvae with the NADPH oxidase inhibitor following pharmacological disruptions in thyroid hormone synthesis phenocopied the hypothyroid phenotypes with hyperplastic goiter caused by impaired TH synthesis (32). In mutant strains, the hypothalamic–pituitary–thyroid axis in zebrafish also showed hypothyroid phenotypes. For example, knockdown of type 3 iodothyronine deiodinase (Dio3) perturbed both embryonic and early larval development in zebrafish (33), which were similar to those of knockdown duox in our study. In mutant strains in thyroid hormone transporters, oatp1c1–/– and oatp1c1–/– X mct8–/– adults exhibited an enlarged thyroid gland (goiter) (34). Thyroid ablation and tshr mutant strains display alterations in pigmentation; other abnormal phenotypes such as growth retardation, delayed development of scales, thyroid hyperplasia/external goiter, and infertility have also been reported (35), which is consistent with the phenotype of the 7-bp deletion mutant line in our study.
Thyroid hormones are responsible for normal growth and development during pre- and postnatal life. In humans, it has been reported that hypothyroidism is associated with decreased libido or impotence; hypothyroid males have shown hypoactive sexual desire, delayed ejaculation, erectile dysfunction, and premature ejaculation; after euthyroidism was restored, these symptoms showed significant improvement (36-38). Krassas et al. reported that hypothyroidism had an adverse effect on human spermatogenesis, with sperm morphology the only parameter that was significantly affected (39). One study showed that male patients with infertility and hypothyroidism were more often euthyroid men (40). For hypothyroid women of reproductive age, hypothyroidism results in altered ovarian function, menstrual irregularities, subfertility, and higher miscarriage rates (41, 42). Zebrafish exhibit an elaborate premating ritual consisting of a series of behaviors to attract mates (43). Male zebrafish exhibit typical courtship behaviors toward females, including chase (following or swimming alongside female), tail-nose (touching the female’s side or tail with nose or head), zig-zag (tail sweep and circle along female’s body), encircle (circling around or in front of the female), and quiver (rapid tail oscillation against the female’s side). The only female courtship behavior is egg laying (oviposition). These mating behaviors can be recorded on videos, and then viewed and analyzed using VideoPad Editor as described before (44). Chopra et al. investigating the phenotypes associated with 2 nonsense mutant alleles in duox in zebrafish found adult females do contain eggs but failed to breed (45). The methimazole-treated fathead minnow did not show an increase in the number of spermatozoa and a decrease in the number of spermatogonia compared with the untreated group, which suggests that hypothyroidism affects spermatogenesis (46).
Intriguingly, we also noted duox KO mutants lose fertility regardless of whether males or females. After KO mutants were treated with T4 from birth to adult, the fertility of both males and females improved. This phenomenon indicated that hypothyroidism is associated with fertility as previously described (46). Interestingly, when we started T4 treatment at different time/age, from 6 hpf at the earliest to 20 dpf at the latest, the development and fertility of treated mutants also returned to normal. Although we do not currently know the reason for the similar outcome of KO mutants with different onset treatment time, a potential cause may be due to the low level of thyroid hormone needed for growth and development before the juvenile stage in zebrafish. It has previously been noted that, in zebrafish, the peaks in thyroid hormones at 21 dpf for T4 and 10 dpf for T3 are likely to be associated with transition from the larval to juvenile stage (47).
Our study found that the fertility of zebrafish is dependent on thyroid hormone; T4 treatment should be continued and cannot be stopped over the age of 2 weeks in hypothyroid zebrafish in order to achieve fertility. Strikingly, we found thyroid hormones played a role in development and maturing of reproductive cells. Reproductive cells in KO mutants were immature, while after long-term T4 treatment, reproductive cells in KO mutants could develop into mature oocytes or sperms.
Conclusion
In this study, we generated hypothyroid zebrafish through knockdown duox by morpholino or KO duox by CRISPR Cas9, both mutants displayed hypothyroid phenotypes, which could be rescued by T4 treatment. Thyroid hormones played a role in developing and maturing of reproductive cells. Our work indicated that duox mutant zebrafish may provide a model for human congenital hypothyroidism.
Abbreviations
- CH
congenital hypothyroidism
- dpf
days post fertilization
- DUOX
dual oxidase
- ELISA
enzyme-linked immunosorbent assay
- H&E
, hematoxylin and eosin
- hpf
hours post fertilization
- KO
knockout
- PBST
phosphate-buffered saline supplemented with 0.1% Tween 20
- PCR
polymerase chain reaction
- ROS
reactive oxygen species
- RT
room temperature
- T3
triiodotyrosine
- T4
thyroxine
- WISH
whole mount in situ hybridization
- WT
, wildtype.
Acknowledgment
We would like to thank the aquarium staff in the zebrafish unit for their care and support of the fish.
Financial Support: This work was supported by Chinese National Key Research Program (2017YFC1001801 to H.-D. S.) and the National Natural Science Foundation of China (81770786 to H.-D. S., 81661168016 to H.-D. S., 81870537 to S.-X. Z., 82070816 to S.-X. Z.) and Shanghai Science and Technology Committee (19140904200 to M. D.) and Shanghai Municipal Education Commission Two-hundred Talent (20161318 to to S.-X. Z.).
Additional Information
Disclosures: The authors have nothing to disclose.
Data Availability
All data generated or analyzed during this study are included in this published article or in the data repositories listed in References.
References
Author notes
The first 3 authors should be regarded as joint first authors.