-
PDF
- Split View
-
Views
-
Cite
Cite
Casper Bo Jensen, Charles Pyke, Morten Grønbech Rasch, Anders Bjorholm Dahl, Lotte Bjerre Knudsen, Anna Secher, Characterization of the Glucagonlike Peptide-1 Receptor in Male Mouse Brain Using a Novel Antibody and In Situ Hybridization, Endocrinology, Volume 159, Issue 2, February 2018, Pages 665–675, https://doi.org/10.1210/en.2017-00812
- Share Icon Share
Abstract
Glucagonlike peptide 1 (GLP-1) is a physiological regulator of appetite, and long-acting GLP-1 receptor (GLP-1R) agonists lower food intake and body weight in both human and animal studies. The effects are mediated through brain GLP-1Rs, and several brain nuclei expressing the GLP-1R may be involved. To date, the mapping of the complete location of GLP-1R protein in the brain has been challenged by lack of good antibodies and the discrepancy between mRNA and protein, especially relevant in neuronal axonal processes. Here, we present a specific monoclonal GLP-1R antibody for immunohistochemistry with murine tissue and show detailed distribution of GLP-1R expression, as well as mapping of GLP-1R mRNA by nonradioactive in situ hybridization. Semiautomated image analysis was performed to map the GLP-1R distribution to atlas plates from the Allen Institute for Brain Science. The GLP-1R was abundantly expressed in numerous regions, including the septal nucleus, hypothalamus, and brain stem. GLP-1R protein expression was also observed on neuronal projections in brain regions devoid of any mRNA that has not been observed in earlier reports. Taken together, these findings provide knowledge on GLP-1R expression in neuronal cell bodies and neuronal projections.
Glucagonlike peptide 1 (GLP-1) is a neurotransmitter produced in the brain stem and an incretin hormone released from gut endocrine L cells. GLP-1 was shown to have a role in central regulating of feeding in rat and human GLP-1–activated brain areas known to regulate food intake (1). GLP-1 also has an important role in regulating insulin release through activation of the GLP-1 receptor (GLP-1R) expressed on pancreatic β cells, thereby lowering blood glucose levels (2). The GLP-1R is a Gs protein-coupled receptor (3), and mRNA coding for the receptor is widely expressed in both rodents (4) and humans (5). Longer acting GLP-1R agonists (GLP-1RAs) lower overall appetite (6) and bodyweight (7) through mechanisms in the brain (8, 9). In rodent models, GLP-1RAs also affect other central functions, such as neuroprotection, learning, and memory, and has been shown to relieve symptoms in Alzheimer’s and Parkinson disease models (10). A complete characterization of the localization of GLP-1Rs throughout the brain will aid to delineate GLP-1R signaling in the brain, potentially involved in these functions. Although extensive mapping of the receptor mRNA has been conducted previously (11), the mapping of GLP-1R protein distribution by immunohistochemistry (IHC) has been challenged by the lack of specific GLP-1R antibodies (12). Recently, the GLP-1R distribution in the brain was characterized by use of GLP-1R reporter mice (13). Albeit extensive, potential overestimation was emphasized as a result of the nature of the transgene being expressed at any time in development. With the use of the primate-specific GLP-1R monoclonal antibody (MAb; 3F52) (14), we have previously described the GLP-1R distribution in the nonhuman primate brain (15). Here, we present an additional specific GLP-1R MAb (7F38A2) for the use with murine tissue and show the detailed distribution of GLP-1R immunoreactivity in combination with a mapping of GLP-1R mRNA by nonradioactive in situ hybridization (ISH). Both antibodies have been made available through the Developmental Studies Hybridoma Bank (Department of Biology, University of Iowa, Iowa City, IA). The GLP-1R distribution was mapped by image segmentation following semiautomatic registration of the scanned histological slides to digital atlas plates from the Allen Institute for Brain Science (AIBS) mouse brain reference model (16, 17). IHC was performed on brain tissue derived from mice with a complete knockout of the GLP-1R (Glp-1r−/−) to further validate the antibody.
Materials and Methods
Generation of anti-mouse GLP-1R antibody
GLP-1R MAb (7F38A2; 3.6 mg/mL) was generated at Novo Nordisk A/S (Maaloev, Denmark) (Table 1). Glp-1r−/− mice were immunized with baby hamster kidney (BHK) cells, stably transfected with the mouse GLP-1R (mGLP-1R), and antibodies were generated using standard hybridoma technology. The Glp-1r−/− mice were on a C57BL background and were derived from the previously described Glp-1r−/− strain (18), custom bred by Novo Nordisk A/S at Taconic (Ejby, Denmark). The strain is not commercially available. GLP-1R-specific antibodies were identified by image-based screening (ImageXpress; Molecular Devices, Sunnyvale, CA) using the BHK mGLP-1R cells used for immunization and a mock-transfected BHK cell line for counter screen. Following isolation of the 7F38A2 clone and purification of the antibody, binding to mGLP-1R was validated in a flow cytometry experiment using transiently transfected human embryonic kidney mGLP-1R and human embryonic kidney mock cells. Detection was done using an allophycocyanin-labeled anti-mouse antibody (catalog no. 115-136-071; Jackson ImmunoResearch Laboratories, West Grove, PA), diluted 1:500. The 7F38A2 antibody is a mouse immunoglobulin G2aκ, and anti-trinitrophenol is an isotype control. For the immunostaining experiments, the antibody was biotinylated using N-hydroxysuccinimide ester–biotin (catalog no. H1759; Sigma-Aldrich, St. Louis, MO), following the manufacturer’s instructions. The antibody is now available at http://dshb.biology.uiowa.edu/Mab-7F38?sc=9&category=-109.
Peptide/Protein Target . | Name of Antibody . | Manufacturer, Catalog No. . | Species Raised in, Monoclonal or Polyclonal . | Dilution Used . | RRID . |
---|---|---|---|---|---|
GLP-1R | MAb 7F38A2 | Developmental Studies Hybridoma Bank, MAb 7F38 | Mouse, monoclonal | 1:720 | AB_2618101 |
Peptide/Protein Target . | Name of Antibody . | Manufacturer, Catalog No. . | Species Raised in, Monoclonal or Polyclonal . | Dilution Used . | RRID . |
---|---|---|---|---|---|
GLP-1R | MAb 7F38A2 | Developmental Studies Hybridoma Bank, MAb 7F38 | Mouse, monoclonal | 1:720 | AB_2618101 |
Abbreviation: RRID, Research Resource Identifier.
Peptide/Protein Target . | Name of Antibody . | Manufacturer, Catalog No. . | Species Raised in, Monoclonal or Polyclonal . | Dilution Used . | RRID . |
---|---|---|---|---|---|
GLP-1R | MAb 7F38A2 | Developmental Studies Hybridoma Bank, MAb 7F38 | Mouse, monoclonal | 1:720 | AB_2618101 |
Peptide/Protein Target . | Name of Antibody . | Manufacturer, Catalog No. . | Species Raised in, Monoclonal or Polyclonal . | Dilution Used . | RRID . |
---|---|---|---|---|---|
GLP-1R | MAb 7F38A2 | Developmental Studies Hybridoma Bank, MAb 7F38 | Mouse, monoclonal | 1:720 | AB_2618101 |
Abbreviation: RRID, Research Resource Identifier.
Animals
Male mice (C57BL/6J and Glp-1r−/−) and rats (Sprague-Dawley) were obtained from Taconic and housed five per cage in standard, temperature-controlled conditions with a 12-hour light/dark cycle. The animals had ad libitum access to water and regular chow (No. 1324; Altromin, Brogaarden, Fårevejle, Denmark) unless stated otherwise. Handling and housing of the animals were conducted in accordance with approved national regulations in Denmark, which are fully compliant with internationally accepted principles for the care and use of laboratory animals, and with animal experimental licenses, granted by the Danish Ministry of Justice.
Tissue preparation and sectioning
The animals were anesthetized with isoflurane and transcardially perfused with heparinized (10 U/mL) saline (10 mL), followed by 10% neutral-buffered formalin (10 mL). Brains were removed, immersed into 10% neutral-buffered formalin, and stored at room temperature for 24 hours before being embedded into paraffin. For whole brain receptor mapping, one male mouse was used, and the entire brain was sectioned at 4.5 µm intervals, and all sections were collected. Additionally, two male mice (one C57BL/6J; one Glp-1r−/−) and one male rat (Sprague-Dawley) were used for a follow-up study. Section thickness was 4.5 µm.
IHC
For whole brain receptor mapping, every 20th section was used for IHC. Paraffin sections were dewaxed and rehydrated in double-distilled water. Sections were treated with 0.1% pronase in phosphate-buffered saline at 37°C for 10 minutes and rinsed in Tris-buffered saline (TBS). Sections were then treated with 1% H2O2 in TBS for 15 minutes, washed in TBS with 0.05% Tween (TBS-T), blocked with avidin for 10 minutes (Dako, Glostrup, Denmark), washed with TBS-T, blocked with biotin for 10 minutes (Dako), washed with TBS-T, and preincubated with 3.2 mg/mL poly-l-lysine, 3% bovine serum albumin, 7% donkey serum, and 3% skimmed milk (Dako) for 30 minutes. Sections were incubated overnight with primary biotinylated mGLP-1R antibody (MAb 7F38A2; Novo Nordisk) and then washed three times for 10 minutes each in TBS-T, followed by treatment with Vectastain ABC-HRP Complex (Vector Laboratories, Burlingame, CA) in TBS for 30 minutes and washed again three times for 10 minutes each. Sections were developed with diaminobenzidine (Dako) and counterstained with hematoxylin, rinsed in water, dehydrated, and mounted. Sections for the follow-up study were stained with similar protocol with the exception of an additional tyramide signal amplification step and treatment with Vectastain ABC-HRP Complex, following primary antibody incubation, which this time, was reduced to two hours. All images were obtained using NanoZoomer-XR (Hamamatsu, Hamamatsu City, Japan). In addition, the staining protocol has been optimized for automatic staining, applying the Discovery Ultra staining module (Ventana Medical Systems, Tucson, AZ). For details on this protocol, please refer to the Supplemental Material.
ISH
ISH was performed using the RNAscope platform (19, 20) on neighboring sections to the sections used for whole brain IHC mapping. The staining was performed using a Discovery XT system (Ventana Medical Systems) with VS RNAscope FFPE Red Reagent Kit (catalog no. 320610; Advanced Cell Diagnostics, Newark, CA). In brief, the VS RNAscope protocol was used with the following pretreatment conditions: boiling for two minutes and pretreatment two plus three steps for four minutes each with probes for bacterial dapB (negative control; catalog no. 310048) and mGLP-1R (catalog no. 415896). Images were obtained using the NanoZoomer-XR.
To validate the signal obtained for GLP-1R mRNA, another mGLP-1R probe (Number 415906) nonoverlapping in sequence with Probe Number 415896 was used on adjacent sagittal mouse brain sections. The signals obtained with both probes showed a complete overlap (data not shown).
Image analysis and atlas plate generation
Image segmentation of the IHC and ISH slide-scanner images was performed using a multinomial regression classifier trained on a constructed mosaic image for each staining type. The red, green, blue values of the images were used as input features. Before segmentation, each IHC/ISH image pair was registered to a digital atlas plate, obtained from the AIBS mouse brain reference model (16, 17). The specific atlas plates were manually selected from a collection of 528 plates. The image registration was performed in a coarse to fine manner with an affine transformation, followed by nonlinear B-spline transformation using sum of squared difference as similarity measure. All registrations were computed using Elastix (21). The final atlas plates were constructed by summing a segmented signal inside of a square mask moving across the registered images. A blue circle (IHC) or a red square (ISH) was drawn on the atlas plate if positive signal was detected. The diameter of the marker was based on the signal count, applying a logarithmic scale to show both weak and strong signals. Following the automatic atlas plate generation, manual corrections were performed in regions where the automatic image registration was not sufficiently accurate.
Results
Antibody specificity
IHC staining with the GLP-1R antibody revealed high-intensity staining with good resolution and low background (Fig. 1). The antibody was specific for GLP-1Rs expressed in peripheral organs, such as the Brunner’s gland [Fig. 1(a) and 1(b)] and further for mouse [Fig. 1(c)–1(f)] and rat [Fig. 1(g) and 1(h)] brain. The expressional profile of GLP-1R was evaluated throughout the entire mouse brain, and this expression was confirmed in select regions in the rat brain in the hypothalamus and brain stem, showing correspondence between the two species in the regions investigated. However, some discrepancies existed between mouse and rat, where GLP-1R expression could be observed in mouse lateral habenula, nucleus accumbens, and the nucleus of the lateral olfactory tract/cortical amygdalar area, which was absent in rat brain (data not shown). Validation of the antibody has been performed further for GLP-1Rs in the pancreas (22) and kidney (23) and other peripheral GLP-1R-expressing organs, such as the gastrointestinal tract, lung, and thyroid (data not shown).
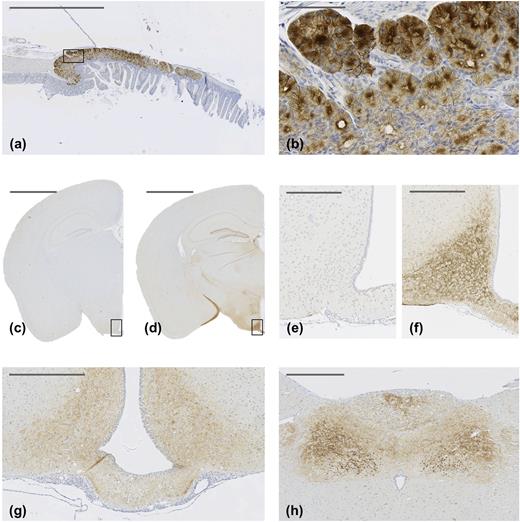
GLP-1R IHC with antibody MAb 7F38A2 on paraffin-embedded mouse Brunner’s gland (a) and (b) and mouse brain (c)–(f). (b) High magnification of the solid line box in (a). (e) and (f) Magnifications of solid line boxed areas in (c) and (d), respectively. (c) and (e) From a Glp-1r−/− mouse; (d) and (f) from a C57BL/6J mouse. (g) and (h) GLP-1R IHC with antibody MAb 7F38A2 on paraffin-embedded rat brain. (g) High magnification image of the hypothalamus, showing positive staining in the arcuate hypothalamic nucleus and median eminence. (h) High magnification image, showing positive staining in the area postrema, dorsal motor nucleus of the vagus nerve, and nucleus of the solitary tract. Original scale bars: (a), (c), and (d) 2000 μm; (b) 100 μm; (e) and (f) 250 μm; (g) and (h) 500 μm.
Distribution of GLP-1R
Overall, GLP-1R protein and mRNA colocalized in most regions; however, some discrepancies were found, such as in the posterior hippocampus, median eminence, and terminal field of accessory olfactory bulb projections. The findings are summarized in Table 2 and on the atlas plate overlays (Figs. 2 and 3 ).
Summary of GLP-1R (IHC and ISH) Location in C57BL/6J Mouse Brain and Comparison With External References
Structure . | Area . | Protein . | mRNA . | Cork et al. (13) (Protein) . | AIBS (mRNA) . | |
---|---|---|---|---|---|---|
Telencephalon | ACB | Nucleus accumbens | + | + | + | + |
AOB | Accessory olfactory bulb | + | + | + | + | |
BMA | Basomedial amygdalar nucleus | + | − | + | + | |
BST | Bed nucleus of stria terminalis | + | + | + | + | |
CeA | Central amygdala | + | + | + | + | |
CeA/AAA | Central amygdala/anterior amygdalar area | + | + | + | + | |
COA | Cortical amygdalar area | + | − | + | + | |
DG | Dentate gyrus | + | + | + | + | |
LPO | Lateral preoptic area | + | + | + | + | |
LS | Lateral septal nucleus | + | + | + | + | |
NLOT/COA | Nucleus of the lateral olfactory tract/cortical amygdalar area | + | − | + | + | |
OV | Vascular organ of the laminae terminalis | + | NA | + | NA | |
PA | Posterior amygdalar nucleus | + | − | + | + | |
SF | Septofimbrial nucleus | + | + | NA | + | |
SFO | Subfornical organ | + | + | + | + | |
TRS | Triangular nucleus of septum | − | + | NA | + | |
TT | Tenia tecta | + | + | + | + | |
Diencephalon | ARH | Arcuate hypothalamic nucleus | + | + | + | + |
DMH | Dorsomedial hypothalamic nucleus | + | + | + | + | |
GENd | Geniculate group, dorsal thalamus | + | − | + | + | |
Hb | Habenula | + | − | + | + | |
LHA | Lateral hypothalamic nucleus | + | + | + | + | |
ME | Median eminence | + | − | NA | + | |
PH | Posterior hypothalamic nucleus | + | − | + | + | |
PP | Peripeduncular nucleus | + | + | NA | + | |
PVH | Paraventricular hypothalamic nucleus | + | + | + | + | |
RCH | Retrochiasmatic area | + | − | + | + | |
SO | Supraoptic nucleus | + | + | NA | + | |
TU | Tuberal nucleus | + | + | NA | + | |
ZI | Zona incerta | + | + | + | + | |
Mesencephalon | APN | Anterior pretectal nucleus | + | + | + | + |
DR | Dorsal raphe | + | − | NA | + | |
MM | Medial mammillary nucleus | + | − | + | + | |
MPT | Medial pretectal area | + | + | + | + | |
MRN | Midbrain reticular nucleus | + | − | NA | + | |
PAG | Periaqueductal gray | + | + | + | + | |
PPT | Posterior pretectal area | + | + | + | + | |
SCs | Superior colliculus, sensory related | − | + | NA | + | |
SCm | Superior colliculus, motor related | + | + | NA | + | |
SCTV | ventral spinocerebellar tract | + | − | NA | + | |
SNr | Substantia nigra, reticular part | + | + | NA | + | |
SNc | Substantia nigra, compact part | + | + | NA | + | |
Pons | NI | Nucleus incertus | + | − | NA | + |
NLL | Nucleus of the lateral lemniscus | + | − | NA | + | |
PB | Parabrachial nucleus | + | + | NA | + | |
PCG | Pontine central gray | + | − | NA | + | |
PG | Pontine gray | + | + | NA | + | |
PRNc | Pontine reticular nucleus, caudal part | − | + | NA | + | |
PRNr | Pontine reticular nucleus | − | + | NA | + | |
PSV | Principal sensory nucleus of the trigeminal | + | + | NA | + | |
SOC | Superior olivary complex | + | − | NA | + | |
Medulla | AMB | Nucleus ambiguus | − | + | NA | + |
AP | Area postrema | + | + | + | + | |
CU | Cuneate nucleus | + | + | NA | + | |
DMX | Dorsal motor nucleus of the vagus nerve | + | − | NA | + | |
ECU | External cuneate nucleus | + | + | NA | + | |
FL | Flocculus (cerebellum) | + | − | NA | + | |
IO | Inferior olivary complex | + | + | + | + | |
LRNm | Lateral reticular nucleus, magnocellular part | + | + | NA | + | |
MARN | Magnocellular reticular nucleus | − | + | NA | + | |
MDRN | Medullary reticular nucleus | − | + | NA | + | |
MV | Medial vestibular nucleus | + | + | NA | + | |
NTS | Nucleus tractus solitarus | + | + | + | + | |
PARN | Parvocellular reticular nucleus | − | + | NA | + | |
SPVC | Spinal nucleus of the trigeminal, caudal part | + | + | NA | + |
Structure . | Area . | Protein . | mRNA . | Cork et al. (13) (Protein) . | AIBS (mRNA) . | |
---|---|---|---|---|---|---|
Telencephalon | ACB | Nucleus accumbens | + | + | + | + |
AOB | Accessory olfactory bulb | + | + | + | + | |
BMA | Basomedial amygdalar nucleus | + | − | + | + | |
BST | Bed nucleus of stria terminalis | + | + | + | + | |
CeA | Central amygdala | + | + | + | + | |
CeA/AAA | Central amygdala/anterior amygdalar area | + | + | + | + | |
COA | Cortical amygdalar area | + | − | + | + | |
DG | Dentate gyrus | + | + | + | + | |
LPO | Lateral preoptic area | + | + | + | + | |
LS | Lateral septal nucleus | + | + | + | + | |
NLOT/COA | Nucleus of the lateral olfactory tract/cortical amygdalar area | + | − | + | + | |
OV | Vascular organ of the laminae terminalis | + | NA | + | NA | |
PA | Posterior amygdalar nucleus | + | − | + | + | |
SF | Septofimbrial nucleus | + | + | NA | + | |
SFO | Subfornical organ | + | + | + | + | |
TRS | Triangular nucleus of septum | − | + | NA | + | |
TT | Tenia tecta | + | + | + | + | |
Diencephalon | ARH | Arcuate hypothalamic nucleus | + | + | + | + |
DMH | Dorsomedial hypothalamic nucleus | + | + | + | + | |
GENd | Geniculate group, dorsal thalamus | + | − | + | + | |
Hb | Habenula | + | − | + | + | |
LHA | Lateral hypothalamic nucleus | + | + | + | + | |
ME | Median eminence | + | − | NA | + | |
PH | Posterior hypothalamic nucleus | + | − | + | + | |
PP | Peripeduncular nucleus | + | + | NA | + | |
PVH | Paraventricular hypothalamic nucleus | + | + | + | + | |
RCH | Retrochiasmatic area | + | − | + | + | |
SO | Supraoptic nucleus | + | + | NA | + | |
TU | Tuberal nucleus | + | + | NA | + | |
ZI | Zona incerta | + | + | + | + | |
Mesencephalon | APN | Anterior pretectal nucleus | + | + | + | + |
DR | Dorsal raphe | + | − | NA | + | |
MM | Medial mammillary nucleus | + | − | + | + | |
MPT | Medial pretectal area | + | + | + | + | |
MRN | Midbrain reticular nucleus | + | − | NA | + | |
PAG | Periaqueductal gray | + | + | + | + | |
PPT | Posterior pretectal area | + | + | + | + | |
SCs | Superior colliculus, sensory related | − | + | NA | + | |
SCm | Superior colliculus, motor related | + | + | NA | + | |
SCTV | ventral spinocerebellar tract | + | − | NA | + | |
SNr | Substantia nigra, reticular part | + | + | NA | + | |
SNc | Substantia nigra, compact part | + | + | NA | + | |
Pons | NI | Nucleus incertus | + | − | NA | + |
NLL | Nucleus of the lateral lemniscus | + | − | NA | + | |
PB | Parabrachial nucleus | + | + | NA | + | |
PCG | Pontine central gray | + | − | NA | + | |
PG | Pontine gray | + | + | NA | + | |
PRNc | Pontine reticular nucleus, caudal part | − | + | NA | + | |
PRNr | Pontine reticular nucleus | − | + | NA | + | |
PSV | Principal sensory nucleus of the trigeminal | + | + | NA | + | |
SOC | Superior olivary complex | + | − | NA | + | |
Medulla | AMB | Nucleus ambiguus | − | + | NA | + |
AP | Area postrema | + | + | + | + | |
CU | Cuneate nucleus | + | + | NA | + | |
DMX | Dorsal motor nucleus of the vagus nerve | + | − | NA | + | |
ECU | External cuneate nucleus | + | + | NA | + | |
FL | Flocculus (cerebellum) | + | − | NA | + | |
IO | Inferior olivary complex | + | + | + | + | |
LRNm | Lateral reticular nucleus, magnocellular part | + | + | NA | + | |
MARN | Magnocellular reticular nucleus | − | + | NA | + | |
MDRN | Medullary reticular nucleus | − | + | NA | + | |
MV | Medial vestibular nucleus | + | + | NA | + | |
NTS | Nucleus tractus solitarus | + | + | + | + | |
PARN | Parvocellular reticular nucleus | − | + | NA | + | |
SPVC | Spinal nucleus of the trigeminal, caudal part | + | + | NA | + |
Presence (+) or absence (−) of GLP-1R protein and mRNA in mouse brain. The two right columns indicate whether GLP-1R was observed as a protein [Cork et al. (13)] or mRNA (AIBS).
Abbreviation: NA, not assessed/reported.
Summary of GLP-1R (IHC and ISH) Location in C57BL/6J Mouse Brain and Comparison With External References
Structure . | Area . | Protein . | mRNA . | Cork et al. (13) (Protein) . | AIBS (mRNA) . | |
---|---|---|---|---|---|---|
Telencephalon | ACB | Nucleus accumbens | + | + | + | + |
AOB | Accessory olfactory bulb | + | + | + | + | |
BMA | Basomedial amygdalar nucleus | + | − | + | + | |
BST | Bed nucleus of stria terminalis | + | + | + | + | |
CeA | Central amygdala | + | + | + | + | |
CeA/AAA | Central amygdala/anterior amygdalar area | + | + | + | + | |
COA | Cortical amygdalar area | + | − | + | + | |
DG | Dentate gyrus | + | + | + | + | |
LPO | Lateral preoptic area | + | + | + | + | |
LS | Lateral septal nucleus | + | + | + | + | |
NLOT/COA | Nucleus of the lateral olfactory tract/cortical amygdalar area | + | − | + | + | |
OV | Vascular organ of the laminae terminalis | + | NA | + | NA | |
PA | Posterior amygdalar nucleus | + | − | + | + | |
SF | Septofimbrial nucleus | + | + | NA | + | |
SFO | Subfornical organ | + | + | + | + | |
TRS | Triangular nucleus of septum | − | + | NA | + | |
TT | Tenia tecta | + | + | + | + | |
Diencephalon | ARH | Arcuate hypothalamic nucleus | + | + | + | + |
DMH | Dorsomedial hypothalamic nucleus | + | + | + | + | |
GENd | Geniculate group, dorsal thalamus | + | − | + | + | |
Hb | Habenula | + | − | + | + | |
LHA | Lateral hypothalamic nucleus | + | + | + | + | |
ME | Median eminence | + | − | NA | + | |
PH | Posterior hypothalamic nucleus | + | − | + | + | |
PP | Peripeduncular nucleus | + | + | NA | + | |
PVH | Paraventricular hypothalamic nucleus | + | + | + | + | |
RCH | Retrochiasmatic area | + | − | + | + | |
SO | Supraoptic nucleus | + | + | NA | + | |
TU | Tuberal nucleus | + | + | NA | + | |
ZI | Zona incerta | + | + | + | + | |
Mesencephalon | APN | Anterior pretectal nucleus | + | + | + | + |
DR | Dorsal raphe | + | − | NA | + | |
MM | Medial mammillary nucleus | + | − | + | + | |
MPT | Medial pretectal area | + | + | + | + | |
MRN | Midbrain reticular nucleus | + | − | NA | + | |
PAG | Periaqueductal gray | + | + | + | + | |
PPT | Posterior pretectal area | + | + | + | + | |
SCs | Superior colliculus, sensory related | − | + | NA | + | |
SCm | Superior colliculus, motor related | + | + | NA | + | |
SCTV | ventral spinocerebellar tract | + | − | NA | + | |
SNr | Substantia nigra, reticular part | + | + | NA | + | |
SNc | Substantia nigra, compact part | + | + | NA | + | |
Pons | NI | Nucleus incertus | + | − | NA | + |
NLL | Nucleus of the lateral lemniscus | + | − | NA | + | |
PB | Parabrachial nucleus | + | + | NA | + | |
PCG | Pontine central gray | + | − | NA | + | |
PG | Pontine gray | + | + | NA | + | |
PRNc | Pontine reticular nucleus, caudal part | − | + | NA | + | |
PRNr | Pontine reticular nucleus | − | + | NA | + | |
PSV | Principal sensory nucleus of the trigeminal | + | + | NA | + | |
SOC | Superior olivary complex | + | − | NA | + | |
Medulla | AMB | Nucleus ambiguus | − | + | NA | + |
AP | Area postrema | + | + | + | + | |
CU | Cuneate nucleus | + | + | NA | + | |
DMX | Dorsal motor nucleus of the vagus nerve | + | − | NA | + | |
ECU | External cuneate nucleus | + | + | NA | + | |
FL | Flocculus (cerebellum) | + | − | NA | + | |
IO | Inferior olivary complex | + | + | + | + | |
LRNm | Lateral reticular nucleus, magnocellular part | + | + | NA | + | |
MARN | Magnocellular reticular nucleus | − | + | NA | + | |
MDRN | Medullary reticular nucleus | − | + | NA | + | |
MV | Medial vestibular nucleus | + | + | NA | + | |
NTS | Nucleus tractus solitarus | + | + | + | + | |
PARN | Parvocellular reticular nucleus | − | + | NA | + | |
SPVC | Spinal nucleus of the trigeminal, caudal part | + | + | NA | + |
Structure . | Area . | Protein . | mRNA . | Cork et al. (13) (Protein) . | AIBS (mRNA) . | |
---|---|---|---|---|---|---|
Telencephalon | ACB | Nucleus accumbens | + | + | + | + |
AOB | Accessory olfactory bulb | + | + | + | + | |
BMA | Basomedial amygdalar nucleus | + | − | + | + | |
BST | Bed nucleus of stria terminalis | + | + | + | + | |
CeA | Central amygdala | + | + | + | + | |
CeA/AAA | Central amygdala/anterior amygdalar area | + | + | + | + | |
COA | Cortical amygdalar area | + | − | + | + | |
DG | Dentate gyrus | + | + | + | + | |
LPO | Lateral preoptic area | + | + | + | + | |
LS | Lateral septal nucleus | + | + | + | + | |
NLOT/COA | Nucleus of the lateral olfactory tract/cortical amygdalar area | + | − | + | + | |
OV | Vascular organ of the laminae terminalis | + | NA | + | NA | |
PA | Posterior amygdalar nucleus | + | − | + | + | |
SF | Septofimbrial nucleus | + | + | NA | + | |
SFO | Subfornical organ | + | + | + | + | |
TRS | Triangular nucleus of septum | − | + | NA | + | |
TT | Tenia tecta | + | + | + | + | |
Diencephalon | ARH | Arcuate hypothalamic nucleus | + | + | + | + |
DMH | Dorsomedial hypothalamic nucleus | + | + | + | + | |
GENd | Geniculate group, dorsal thalamus | + | − | + | + | |
Hb | Habenula | + | − | + | + | |
LHA | Lateral hypothalamic nucleus | + | + | + | + | |
ME | Median eminence | + | − | NA | + | |
PH | Posterior hypothalamic nucleus | + | − | + | + | |
PP | Peripeduncular nucleus | + | + | NA | + | |
PVH | Paraventricular hypothalamic nucleus | + | + | + | + | |
RCH | Retrochiasmatic area | + | − | + | + | |
SO | Supraoptic nucleus | + | + | NA | + | |
TU | Tuberal nucleus | + | + | NA | + | |
ZI | Zona incerta | + | + | + | + | |
Mesencephalon | APN | Anterior pretectal nucleus | + | + | + | + |
DR | Dorsal raphe | + | − | NA | + | |
MM | Medial mammillary nucleus | + | − | + | + | |
MPT | Medial pretectal area | + | + | + | + | |
MRN | Midbrain reticular nucleus | + | − | NA | + | |
PAG | Periaqueductal gray | + | + | + | + | |
PPT | Posterior pretectal area | + | + | + | + | |
SCs | Superior colliculus, sensory related | − | + | NA | + | |
SCm | Superior colliculus, motor related | + | + | NA | + | |
SCTV | ventral spinocerebellar tract | + | − | NA | + | |
SNr | Substantia nigra, reticular part | + | + | NA | + | |
SNc | Substantia nigra, compact part | + | + | NA | + | |
Pons | NI | Nucleus incertus | + | − | NA | + |
NLL | Nucleus of the lateral lemniscus | + | − | NA | + | |
PB | Parabrachial nucleus | + | + | NA | + | |
PCG | Pontine central gray | + | − | NA | + | |
PG | Pontine gray | + | + | NA | + | |
PRNc | Pontine reticular nucleus, caudal part | − | + | NA | + | |
PRNr | Pontine reticular nucleus | − | + | NA | + | |
PSV | Principal sensory nucleus of the trigeminal | + | + | NA | + | |
SOC | Superior olivary complex | + | − | NA | + | |
Medulla | AMB | Nucleus ambiguus | − | + | NA | + |
AP | Area postrema | + | + | + | + | |
CU | Cuneate nucleus | + | + | NA | + | |
DMX | Dorsal motor nucleus of the vagus nerve | + | − | NA | + | |
ECU | External cuneate nucleus | + | + | NA | + | |
FL | Flocculus (cerebellum) | + | − | NA | + | |
IO | Inferior olivary complex | + | + | + | + | |
LRNm | Lateral reticular nucleus, magnocellular part | + | + | NA | + | |
MARN | Magnocellular reticular nucleus | − | + | NA | + | |
MDRN | Medullary reticular nucleus | − | + | NA | + | |
MV | Medial vestibular nucleus | + | + | NA | + | |
NTS | Nucleus tractus solitarus | + | + | + | + | |
PARN | Parvocellular reticular nucleus | − | + | NA | + | |
SPVC | Spinal nucleus of the trigeminal, caudal part | + | + | NA | + |
Presence (+) or absence (−) of GLP-1R protein and mRNA in mouse brain. The two right columns indicate whether GLP-1R was observed as a protein [Cork et al. (13)] or mRNA (AIBS).
Abbreviation: NA, not assessed/reported.
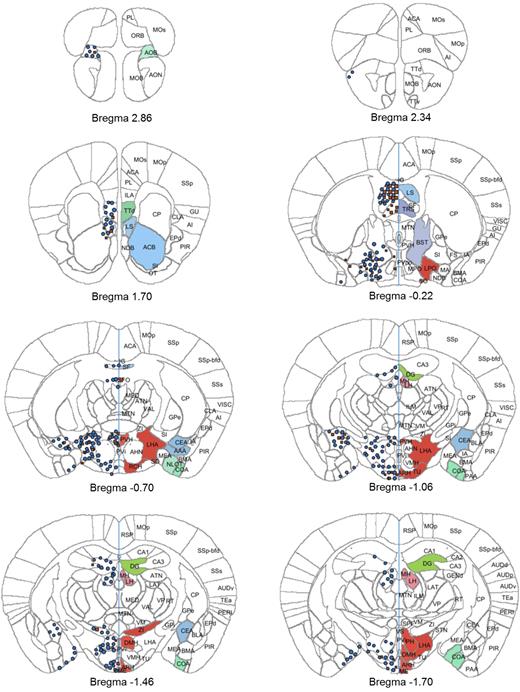
Atlas plates (bregma 2.86 to −1.70) showing distribution of signals for GLP-1R ISH (red squares) and GLP-1R IHC (blue circles). The distribution is overlaid onto digital atlas plates constructed from the AIBS mouse brain atlas. The distribution of GLP-1R mRNA and protein is indicated on the left half of the atlas plates, and brain regions with positive staining for either GLP-1R mRNA or protein are highlighted with a solid color on the right half of the atlas plates. Brain region abbreviations follow the nomenclature of the AIBS mouse brain reference atlas (24).
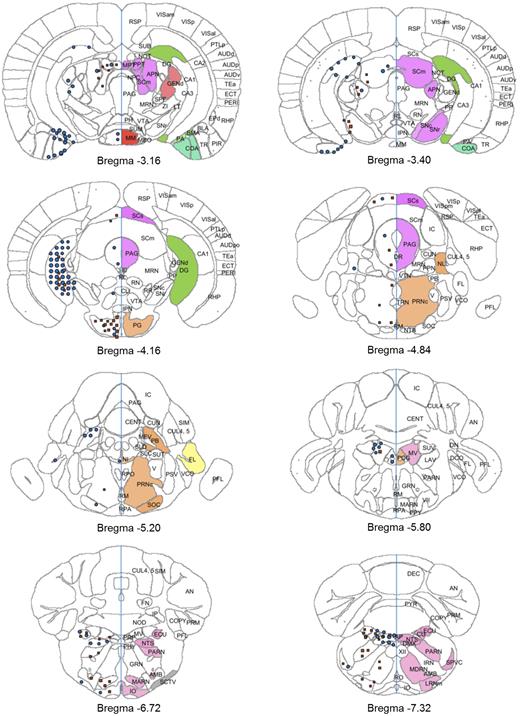
Atlas plates (bregma −3.16 to −7.32) showing distribution of signals for GLP-1R ISH (red squares) and GLP-1R IHC (blue circles). The distribution is overlaid onto digital atlas plates constructed from the AIBS mouse brain atlas. The distribution of GLP-1R mRNA and protein is indicated on the left half of the atlas plates, and brain regions with positive staining for either GLP-1R mRNA or protein are highlighted with a solid color on the right half of the atlas plates. Brain region abbreviations follow the nomenclature of the AIBS mouse brain reference atlas (24).
Telencephalon
In agreement with previous reports, GLP-1R protein-positive cells were identified in the olfactory bulb, amygdala, dentate gyrus, septal nucleus, preoptic area, septofimbrial nucleus, triangular nucleus of septum, tenia tecta, and vascular organ of the laminae terminalis, nucleus accumbens, and bed nucleus of stria terminalis. Furthermore, protein was observed in the subfornical organ and in the terminal field of accessory olfactory bulb projections (the latter is included in the cortical amygdalar area in Table 2), where mRNA expression was absent (Table 2). The specificity of the antibody was validated further by antibody staining on brain sections from Glp-1r−/− mice, especially in those regions where protein expression differed from mRNA expression. The data from the Glp-1r−/− mice showed complete absence of GLP-1R immunoreactivity, further establishing this antibody as specific toward the GLP-1R and confirming the presence of GLP-1R protein in these regions (Fig. 4).
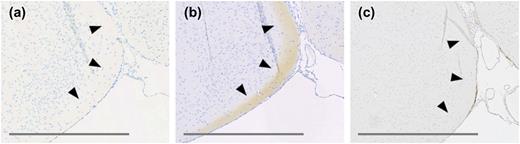
(a) and (b) High magnification images of adjacent sections of C57BL/6J mouse brain tissue focusing on the terminal field of accessory olfactory bulb projections. (a) mGLP-1R ISH showing lack of signal. (b) GLP-1R IHC with antibody MAb 7F38A2 showing positive staining. (c) GLP-1R IHC with antibody MAb 7F38A2 on a corresponding section from a Glp-1r−/− mouse brain showing negative staining. Original scale bars, 400 μm.
Diencephalon
In the diencephalon, GLP-1R protein and mRNA were observed in cells in zona incerta, tuberal nucleus, peripeduncular nucleus, and hypothalamus in the arcuate nucleus, dorsomedial hypothalamic nucleus, lateral hypothalamic nucleus, paraventricular hypothalamic nucleus, and supraoptic nucleus. Furthermore, GLP-1R protein was observed in median eminence as fibers only with no mRNA expression. The same pattern of presence of GLP-1R protein in the absence of mRNA expression was observed in the geniculate group of thalamus, posterior hypothalamic nucleus, habenula, and retrochiasmatic area (Table 2). When comparing mRNA expression reported by AIBS (25) with data obtained in the current study, several areas expressing GLP-1R mRNA in the AIBS atlas were negative in the current study (Table 2).
Mesencephalon
GLP-1R was observed in the anterior pretectal nucleus, medial and posterior pretectal area, periaqueductal gray, and substantia nigra. GLP-1R protein was also observed in the motor-related part of the superior colliculus, whereas mRNA expression could be observed in both the motor-related and the sensory-related part of the superior colliculus. Further discrepancies were observed in the dorsal raphe, medial mammillary nucleus, midbrain reticular nucleus, and ventral spinocerebellar tract, where the receptor was observed as protein on fibers only, lacking mRNA expression.
Pons
In pons, GLP-1R protein and mRNA expression could be observed in the pontine gray, parabrachial nucleus, and principal sensory nucleus of the trigeminal. Very few brain regions were positive for both protein and mRNA, and only protein was observed in the nucleus incertus, nucleus of the lateral lemniscus, and superior olivary complex, whereas only mRNA was observed in the pontine reticular nucleus.
Medulla
In medulla, GLP-1R was observed in the area postrema, cuneate nucleus, inferior olivary complex, lateral reticular nucleus, nucleus tractus solitarus, medial vestibular nucleus, and spinal nucleus of the trigeminal. GLP-1R protein but not mRNA was observed in the dorsal motor nucleus of the vagus nerve and in flocculus, residing in the cerebellum, whereas mRNA was evident in the nucleus ambiguus and reticular nucleus.
Discussion
The reliable detection of the GLP-1R expression in tissues using IHC has been hampered by a lack of specific antibodies, thus limiting well-validated histological mapping studies to the detection of GLP-1R mRNA using ISH (12, 26, 27) and protein using in situ ligand binding with low spatial resolution (28). In this study, an extensive mapping of GLP-1R mRNA and protein expression in the mouse brain is reported. For localization of GLP-1R protein, a GLP-1R MAb was used, which is highly specific for mGLP-1R, as demonstrated by the complete absence of reactivity in mice lacking the GLP-1R. The localization of GLP-1R protein was combined with detection of GLP-1R mRNA on adjacent sections using nonradioactive ISH, enabling a higher accuracy for anatomical localization compared with a radioactivity-based approach. The expression patterns observed showed good alignment between protein and mRNA expression but also revealed some regions only positive for GLP-1R protein and encompassing axonal projections. This is a well-described phenomenon in the brain where protein and gene expression do not always correlate (29–31), possibly in situations where protein can be transported away from the nuclear processing site along the axonal processes. This phenomenon necessitates caution when interpreting potential GLP-1–interacting brain regions and neurons based on receptor mRNA expression alone, as such data will underestimate the number of GLP-1–binding sites. The distribution of GLP-1R protein in the mouse brain has previously been described using a transgene Glp-1r reporter mouse (13). When comparing GLP-1R protein expression detected using the antibody in the current study with the study by Cork et al. (13), there is a high level of agreement, with a few exceptions, where some positive regions were seen using IHC but not reported by Cork et al. (13). Of note, several of these regions were regions lacking mRNA expression, indicating the presence of protein exclusively on projections and terminals. Thus, IHC seems an easier approach to obtain high sensitivity for GLP-1R expression, including axonal processes, without requiring generation of reporter mice. Interestingly, the concern for artifactual overexpression of GLP-1R in the Glp-1r reporter mouse as a result of accumulated gene transcription during embryogenesis does not seem to be a problem in the case of the GLP-1R, as all regions reported positive in the reporter mouse were also seen with antibody staining.
The comparison of mRNA expression in the current study with that reported by AIBS revealed several areas where mRNA was reported to be present by AIBS (e.g., nucleus of the trapezoid body, septohippocampal nucleus, dorsal peduncular area, and tegmental reticular nucleus) but not observed in our study (and hence, not included in Table 2). These inconsistencies are likely a result of a higher specificity of the RNAscope ISH platform used here, as demonstrated by the full overlap of signals obtained with two nonoverlapping probes for GLP-1R. Another explanation could be the more extensive sampling of the mouse brain in studies reported by AIBS, potentially revealing subnuclei with limited GLP-1R expression that was not picked up in this study.
To verify that the antibody could also be applied to rat tissue, select sections from rat brain were stained and examined. Although there was a good agreement with respect to localization of the GLP-1R between mouse and rat on sections with hypothalamus and brain stem, it must be emphasized that the entire rat brain was not analyzed, and a discrepancy between mouse and rat in GLP-1R localization in the brain was detected in some regions. In general, more GLP-1R protein was observed in the mouse brain compared with the rat brain. A large part of the additional GLP-1R protein was seen in mouse axonal processes, such as in the medial habenula and cortical amygdalar areas, which were absent in the rat. Thus, to characterize the GLP-1R fully in the rat brain, a full mapping is needed rather than the assumption of complete correspondence between species.
With the consideration of the relative small fraction of neurons in the hindbrain that produce GLP-1, a remarkable abundance of GLP-1Rs was found to be expressed throughout the brain, indicating potential diverse actions of this neurotransmitter. Indeed, central GLP-1 activity has been reported to affect functions, such as feeding behavior, body weight and temperature, malaise, fluid homeostasis, stress response, and learning and memory (32, 33). One of the most abundant GLP-1R populations was found in the caudal division of the lateral septal nucleus, a population that is little understood with respect to GLP-1 actions. The caudal part of the lateral septal nucleus has been characterized as abundant in somatostatin neurons, which has been shown to act inhibitory in septal nucleus (34, 35). With further building on this inhibitory mechanism, other studies have suggested that GLP-1R-expressing neurons in lateral septal nucleus are GABAergic and connected to GLP-1 actions on behaviors associated with reward (36, 37). Septal projections are extensive and include innervation of the hypothalamus, supramammillary nucleus, hippocampus, thalamus, and ventral tegmental area (38). Thus, the involvement in reward signaling may only be one of many GLP-1–mediated behaviors, initialized from this area.
Another area with high expression of GLP-1R-positive fibers was in the dentate gyrus, which is the principal input to the rest of the hippocampus, forming the “tri-synaptic hippocampal circuit” associated with episodic memory consolidation and formation (39). The dentate gyrus is capable of generating new granule cells in the adult brain (40, 41), which has been linked to memory formation and maintenance, as well as neuroprotection (42, 43). Hence, GLP-1R expression in this area could be coupled to some of the beneficial effects reported with GLP-1 regulation of memory and Alzheimer’s disease (10) and neuroprotection (33).
A high expression of GLP-1R was also identified on fibers projecting to the median eminence. The median eminence is usually associated with the integration of the hypothalamic pituitary axis, serving as an area of release from paraventricular hypothalamic neurons to the bloodstream. On top of this, literature suggests the median eminence to be a site for uptake of peripheral peptides, such as leptin and ghrelin from the blood (44, 45), through receptor-specific uptake; this could be extended to include GLP-1RAs. Indeed, all circumventricular organs, including the median eminence, have a high expression of GLP-1R, suggesting interaction with peripheral GLP-1 in these regions.
In summary, we report here a full mapping of GLP-1R protein and mRNA in the mouse brain using IHC with a specific MAb and ISH, respectively. The strength of using a fully validated and specific antibody is emphasized by the ability to detect GLP-1R protein in regions devoid of mRNA and in neuronal projections, where it was not observed using a transgenic reporter system.
Abbreviations:
- AIBS
Allen Institute for Brain Science
- BHK
baby hamster kidney
- GLP-1
glucagonlike peptide 1
- Glp-1r−/−
glucagonlike peptide 1 receptor knockout
- GLP-1RA
glucagonlike peptide 1 receptor agonist
- IHC
immunohistochemistry
- ISH
in situ hybridization
- MAb
monoclonal antibody
- mGLP-1R
mouse glucagonlike peptide 1 receptor
- TBS
Tris-buffered saline
- TBS-T
Tris-buffered saline with 0.05% Tween.
Acknowledgments
The technical assistance of Pia G. Mortensen, Bettina Brandrup, and Suzanne K. Møller is gratefully acknowledged.
Disclosure Summary: C.B.J., C.P., M.G.R., L.B.K., and A.S. are employed by Novo Nordisk A/S, which markets the GLP-1RA liraglutide for the treatment of diabetes and weight management, and they hold minor stock portions as part of an employee-offering program. A.B.D. has nothing to disclose.