-
PDF
- Split View
-
Views
-
Cite
Cite
Alexander Suvorov, Laura N. Vandenberg, To Cull or Not To Cull? Considerations for Studies of Endocrine-Disrupting Chemicals, Endocrinology, Volume 157, Issue 7, 1 July 2016, Pages 2586–2594, https://doi.org/10.1210/en.2016-1145
- Share Icon Share
The power of animal models is derived from the ability to control experimental variables so that observed effects may be unequivocally attributed to the factor that was changed. One variable that is difficult to control in animal experiments is the number and composition of offspring in a litter. To account for this variability, artificial equalization of the number of offspring in a litter (culling) is often used. The rationale for culling, however, has always been controversial. The Developmental Origins of Health and Disease concept provides a new context to evaluate the pros and cons of culling in laboratory animal studies, especially in the context of endocrine-disrupting chemicals. Emerging evidence indicates that culling, especially of large litters, can drastically change the feeding status of a pup, which can result in compensatory growth with long-term consequences for the animal, including increased risk of cardio-metabolic diseases. Similarly, culling of litters to intentionally bias sex ratios can alter the animal's behavior and physiology, with effects observed on a wide range of outcomes. Thus, in an attempt to control for variability in developmental rates, culling introduces an uncontrolled or confounding variable, which itself may affect a broad spectrum of health-related consequences. Variabilities in culling protocols could be responsible for differences in responses to endocrine-disrupting chemicals reported across studies. Because litter sex composition and size are vectors that can influence both prenatal and postnatal growth, they are essential considerations for the interpretation of results from laboratory animal studies.
The principal use of animal models in biomedical experiments is to establish causal links between different external factors (exposure to environmental stressors, infectious agents, diet, altered social environments, etc) or internal factors (gene knockouts or knockdowns, induced expression of target proteins, influence of single nucleotide polymorphisms, etc) on different phenotypic outcomes, including disease. The power of animal models is derived from the ability to control most important experimental variables so that observed effects may be unequivocally attributed to only 1 variable, the factor that was changed in the experiment. It is therefore not surprising that tremendous effort is being made to standardize experimental conditions and animal models to control every variable that may potentially have an effect on the analyzed outcome, especially for studies of endocrine-disrupting chemicals (EDCs) (1–3). Within laboratory animal facilities, controlled light and humidity conditions, standard cages and housing materials, standardized food with known quantities of fat, protein and percentage of phytoestrogen-based content, and use of inbred rodent strains (with limited genetic variability) are just a few common examples that illustrate this effort.
One variable that is difficult to control in animal experiments is the number of offspring in a litter. Different rodent strains are known to have relatively large vs small litters, and these strains are often selected bearing this in mind. For example, the outbred CD-1 mouse strain is widely used in studies of EDCs not only because it displays sensitivity to many environmental chemicals but also because it typically produces large litters (4). However, even within this one rodent strain, the number of offspring can vary greatly; Cabaton et al (4) report litter sizes ranging from 1 to 23 pups in untreated female CD-1 mice. This variability has concerned researchers examining developmental exposures or other experiments with developmental models, because differences in litter size may also mean differences in the availability of food resources for each individual pup, differences in social environment, differences in intrauterine exposures to hormones, and differences in maternal care, which taken together may significantly affect rates and trajectories of ontogenesis (5–7).
To control these variables in experiments with laboratory animals, culling is often used. Culling is a procedure of artificial equalization of the number of offspring in a litter. For rats and mice, culling is usually performed on postnatal day 4, and litters are usually reduced to 8 pups, 4 per sex, although this procedure varies across studies. In some studies, pups are moved between litters of the same treatment group to further ensure that all litters are the same size (8). Today, culling procedures are often by default considered a gold standard for developmental experiments and are required or recommended by standardized (guideline) testing protocols, including those used to test for endocrine disruption. For example, adjustment of litter size by “elimination of extra pups” is required, recommended, or is optional in the Organization for Economic Co-operation and Development test guidelines for the developmental and reproductive toxicity assay 426, assay 443, and assays 415, 416, and 421, respectively (see Table 1) (9).
Examples of Organization for Economic Co-operation and Development Test Guidelines for the Developmental and Reproductive Toxicity Assays That Refer to the Adjustment of Litter Size by Elimination of Extra Pups
Test Type . | Culling Requirement . | Description . |
---|---|---|
Test number 426, developmental neurotoxicity study | Required | On or before postnatal day 4, the size of each litter should be adjusted by eliminating extra pups by random selection to yield a uniform litter size for all litters |
Test number 443, extended 1-generation reproductive toxicity study | Recommended | On day 4 after birth, the size of each litter may be adjusted by eliminating extra pups by random selection to yield, as nearly as possible, 5 males and 5 females per litter |
Test number 415, 1-generation reproduction toxicity study | Optional | If standardization is carried out, the following procedure is suggested: on day 4 after birth, the size of each litter may be adjusted by eliminating extra pups by selection to yield, as nearly as possible, 4 males and 4 females per litter |
Test number 416, 2-deneration reproduction toxicity | Optional | Standardization of litter sizes is optional |
Test number 421, reproduction/developmental toxicity screening test | Optional | On day 4 after birth, the size of each litter may be adjusted by eliminating extra pups by random selection to yield, as nearly as possible, 4 or 5 pups per sex per litter depending on the normal litter size in the strain of rats used |
Test Type . | Culling Requirement . | Description . |
---|---|---|
Test number 426, developmental neurotoxicity study | Required | On or before postnatal day 4, the size of each litter should be adjusted by eliminating extra pups by random selection to yield a uniform litter size for all litters |
Test number 443, extended 1-generation reproductive toxicity study | Recommended | On day 4 after birth, the size of each litter may be adjusted by eliminating extra pups by random selection to yield, as nearly as possible, 5 males and 5 females per litter |
Test number 415, 1-generation reproduction toxicity study | Optional | If standardization is carried out, the following procedure is suggested: on day 4 after birth, the size of each litter may be adjusted by eliminating extra pups by selection to yield, as nearly as possible, 4 males and 4 females per litter |
Test number 416, 2-deneration reproduction toxicity | Optional | Standardization of litter sizes is optional |
Test number 421, reproduction/developmental toxicity screening test | Optional | On day 4 after birth, the size of each litter may be adjusted by eliminating extra pups by random selection to yield, as nearly as possible, 4 or 5 pups per sex per litter depending on the normal litter size in the strain of rats used |
Examples of Organization for Economic Co-operation and Development Test Guidelines for the Developmental and Reproductive Toxicity Assays That Refer to the Adjustment of Litter Size by Elimination of Extra Pups
Test Type . | Culling Requirement . | Description . |
---|---|---|
Test number 426, developmental neurotoxicity study | Required | On or before postnatal day 4, the size of each litter should be adjusted by eliminating extra pups by random selection to yield a uniform litter size for all litters |
Test number 443, extended 1-generation reproductive toxicity study | Recommended | On day 4 after birth, the size of each litter may be adjusted by eliminating extra pups by random selection to yield, as nearly as possible, 5 males and 5 females per litter |
Test number 415, 1-generation reproduction toxicity study | Optional | If standardization is carried out, the following procedure is suggested: on day 4 after birth, the size of each litter may be adjusted by eliminating extra pups by selection to yield, as nearly as possible, 4 males and 4 females per litter |
Test number 416, 2-deneration reproduction toxicity | Optional | Standardization of litter sizes is optional |
Test number 421, reproduction/developmental toxicity screening test | Optional | On day 4 after birth, the size of each litter may be adjusted by eliminating extra pups by random selection to yield, as nearly as possible, 4 or 5 pups per sex per litter depending on the normal litter size in the strain of rats used |
Test Type . | Culling Requirement . | Description . |
---|---|---|
Test number 426, developmental neurotoxicity study | Required | On or before postnatal day 4, the size of each litter should be adjusted by eliminating extra pups by random selection to yield a uniform litter size for all litters |
Test number 443, extended 1-generation reproductive toxicity study | Recommended | On day 4 after birth, the size of each litter may be adjusted by eliminating extra pups by random selection to yield, as nearly as possible, 5 males and 5 females per litter |
Test number 415, 1-generation reproduction toxicity study | Optional | If standardization is carried out, the following procedure is suggested: on day 4 after birth, the size of each litter may be adjusted by eliminating extra pups by selection to yield, as nearly as possible, 4 males and 4 females per litter |
Test number 416, 2-deneration reproduction toxicity | Optional | Standardization of litter sizes is optional |
Test number 421, reproduction/developmental toxicity screening test | Optional | On day 4 after birth, the size of each litter may be adjusted by eliminating extra pups by random selection to yield, as nearly as possible, 4 or 5 pups per sex per litter depending on the normal litter size in the strain of rats used |
The rationale for culling, however, has always been controversial. Almost 2 decades ago, the necessity of litter size adjustment was the subject of scientific debate (5, 10–12). In the 1996 edition of the Guide for the Care and Use of Laboratory Animals, culling was recommended as one way to ensure that groups of cohoused animals were provided sufficient space within the cage (13), and in the 2011 edition of the Guide, it was still noted that culling provides one method of “intensive management of available space to allow for the safety and well-being of the breeding group” (14).
No general consensus emerged whether the advantages of culling outweigh the disadvantages (or vice versa). The Developmental Origins of Health and Disease concept provides a new context to evaluate the pros and cons of culling in laboratory animal studies. In this minireview, we discuss litter adjustment procedures in light of modern advances in biomedical science with a particular focus on Developmental Origins of Health and Disease and the study of EDCs.
To Cull, or Not To Cull: Traditional Arguments
Arguments in favor of culling
Arguments in favor of culling were summarized by Agnish and Keller (10) and are generally based on concerns over unequal growth and development of pups in small and big litters and the desire to control this unintended inequality. In numerous studies, pup weight at birth or at weaning has been shown to be inversely proportional to litter size (5, 6). In fact, this observation is likely made in studies in which litter size and body weight at early stages of development (eg, in the first few days of postnatal life) are measured and correlated, even if culling occurs at later time points.
It has also been proposed that the elimination of competition by culling gives every pup equal access to milk and results in rapid equalization of body weights (15). Several studies have shown higher weight variability in large litters compared with small litters or litters that were culled during early postnatal development (16, 17). Culling large rat litters to 8 pups reduced variability by 50% compared with unculled litters (10).
Nutrient deficiency in large litters may delay postnatal developmental, including later onset of developmental landmarks, delayed sexual maturation, and alterations in reflex, locomotive, emotional, and memory development (5, 18, 19). Females reared in big litters, which typically have smaller body weights, themselves produce smaller litters (6). Litter size has also been shown to affect maternal behavior. Mothers with big litters commit infanticide more often compared with those with small litters; mothers with big litters also spend less time with their pups (reviewed in Ref. 10). Given that maternal behavior is an important determinant of pups' development (20) and may program stress susceptibility in pups (21), culling provides a means by which any indirect effect of litter size can be controlled.
Finally, although feasibility concerns are rarely discussed when considering experimental design, the reality is that larger litters require significantly more time to weigh, collect and process tissues, make behavioral observations, and collect other outcomes of interest. Palmer and Ulbrich raised the notion that culling leads to reduced workload, as well as reduced laboratory costs (11). These factors need to be acknowledged, especially when very large experiments (eg, n = 20 litters/treatment) are conducted, as is often the case for reproductive and developmental (guideline) toxicity tests of EDCs.
Arguments against culling
Some of the traditionally considered disadvantages of culling have been discussed in detail elsewhere (11). In short, culling leads to an artificial equalization of variance, which contradicts the goals of developmental toxicology and other studies of health outcomes. In fact, many studies have reported that environmental toxicants, including EDCs, increase the variance measured in biological endpoints (22); this change in variance is an important outcome that should not be dismissed.
Artificial equalization of litter size by culling hinders or makes it impossible to detect many reproductive and developmental parameters and endpoints such as the ability of exposed dams to rear large litters, the rate of pup mortality from birth to weaning in exposed and control litters, and true litter weight. There are also concerns about the ability to observe and calculate the prevalence of low-frequency developmental abnormalities and functional deficits. According to the Food and Drug Administration Guidance for Industry Reproductive and Developmental Toxicities, “an increased frequency of rare events in drug-exposed animals increases concern for reproductive or developmental toxicity in humans” (23). Culling, however, decreases the chances of detection of such events by decreasing the number of animals to be surveilled throughout the litter's lifetime.
Finally, some concerns have been raised about how the extent of culling might affect access to nutritional resources. In the laboratory, rat and mouse litters are often reduced to 8 pups, a number that is much smaller than the average litter size in most standard laboratory rodent strains (11, 24). Importantly, this number is comparable with the number of pups typical of litters from wild mice and rats (25, 26), whereas years of artificial selection towards increased litter sizes have significantly modified endocrine regulation of reproduction in laboratory animals (27). These modifications are likely responsible for the mother's ability to maintain a match between milk production and litter size at birth, a correlation found in many studies (15, 24, 28, 29). Thus, artificial reduction of litters in laboratory rodents may result in overfeeding of pups which after culling receive access to milk supply intended for larger litters.
When Culling Induces Artificial Disruptions to Sex Ratios
Studies from litter-bearing rodents including mice and rats have demonstrated how very small differences in hormone exposures during in utero development, due to transport of hormones from neighboring fetuses, can influence behaviors, physiology, and organ morphology (reviewed in Ref. 31). This role of intrauterine position has been proposed as a mechanism by which phenotypic variability could be produced in siblings with relatively similar genetic makeup, increasing the likelihood that some pups would thrive and reproduce in differing environments (32). Although litter makeup during prenatal development is well understood, few studies have examined how the postnatal litter composition can affect behavior, physiology, and morphology.
One factor that has received attention is the influence of sex ratio within the litter on maternal behaviors. Mouse dams rearing all male litters show higher levels of maternal care for multiple measures, including time spent nursing, nest building, and licking of pups (33). Similarly, rat mothers lick male pups more than female pups (34, 35), and the level of licking from the mother influences male offspring sexual performance; reductions in maternal licking during early development are associated with longer interintromission intervals, longer ejaculation latencies, and longer postejaculation intervals (34). These results suggest that artificial changes to the sex ratio via culling can not only alter how the mother interacts with her pups but also influence the sexual behavior of pups in adulthood.
Additional studies have characterized the influence of the litter composition on behavior of male mice (36). After experimental manipulation of sex ratios in litters, adult males reared in male-biased litters exhibited significantly more mounting behaviors toward sexually receptive females compared with males reared in mixed-sex litters (37). Because there were no differences in fertility measures between males reared in all-male vs mixed-sex litters, the authors interpreted these findings to indicate that males from male-biased litters experienced less efficient copulation. Hard and Larsson similarly showed that males reared in all-male litters had decreased reproductive performance compared with males raised in mixed-litters, although with increased exposure to females (eg, 1 wk of testing), these effects were ameliorated (38).
Juvenile female mice are typically more social than males, and altering the sex ratio of litters can increase these dimorphisms (39). Female-biased litters engage in more social behaviors during the early juvenile period, but these behaviors decrease with age, whereas mixed-sex and male-biased litters display fewer of these behaviors, but they increase in frequency with age. Similarly, solitary play is observed more often in females raised only with other females compared with females from mixed-sex litters.
The effects of litter sex ratios extend beyond behaviors of the individual littermates. Male rats from male-biased litters are perceived as more attractive mates for novel females compared with males that were raised in mixed-sex litters, suggesting that the litter make-up can alter the endocrine physiology of the male (37). Brain physiology is also distinguishable based on the sex ratio of the litter. Male rats raised in male-biased litters have decreased metabolic capacity in brain regions (measured by expression of cytochrome oxidase, a marker of oxidative phosphorylation) compared with males raised in mixed litters, although the extent of the difference is specific to the brain nuclei (40). Litter composition also influences sexual maturation of female mice, as females from litters with 0 or 1 male littermate attained puberty earlier, measured by timing of vaginal opening and first estrus, compared with litters with 25%–75% females (41). Here, the author calculated that litter sex composition accounted for 8% of the total variation in pubertal timing. Still other effects of sibling sex ratios have been observed for a range of phenotypes including pain reactivity and response to analgesics (reviewed in Ref. 33).
Researchers that work with rodents are aware that sex ratios across many litters will average out to approximately 50:50 ratios of males and females, but individual litters will deviate from this significantly. The question that experimenters must consider is whether culling litters, and perhaps even mixing pups from multiple litters to reconstitute litters with the sex make-up desired, is the best thing to do. Culling in a manner that deliberately alters the sex ratio (eg, to remove male pups because female reproductive organs are the endpoint of interest) could reduce variability between pups from different litters by controlling for the influence of litter composition. However, this act could also unintentionally alter the endpoint of interest directly, for example, by altering the timing of puberty, or could alter the endpoint of interest indirectly, for example, by altering the attractiveness of the animal which influences downstream fertility endpoints. An alternative approach would be to include sex ratio as a covariate in statistical analyses, or to limit experiments to only those animals derived from litters with relatively even sex ratios. Because even small changes in the sex ratio of the litter after culling can influence physiological and behavioral outcomes, these details need to be fully reported in any study (8).
Culling and Developmental Programing by Catch-Up Growth
Just as culling litters to bias sex ratios can influence the physiology and behaviors of offspring, we similarly propose that culling alone, distinct from alterations in sex ratios, can disrupt metabolic endpoints in offspring. Specifically, we hypothesize that culling induces catch-up growth in developing rodents, resulting in a long-term shift of metabolic phenotypes that ultimately affects many developmental and health endpoints. Our hypothesis capitalizes on the following knowledge: 1) milk production by mothers correlates with litter size at birth; 2) culling of the litter will provide each pup with higher milk resources than would occur without culling, resulting in overfeeding; 3) a sudden switch from restricted nourishment, as is experienced by large litters during gestation, to overfeeding, as is experienced when large litters are culled in early postnatal development, results in catch-up growth; and 4) catch-up growth during early development results in long-term programing of metabolic health and increases risk for many long-term morbidities and mortalities.
In rats, artificial reduction of litter size to 3 pups is a well-established method to induce overfeeding, which results in hyperglycaemia, rapid fat accumulation, and obesity (42, 44). During later life, these rats develop hyperinsulinemia, hyperphagia, high body weight, impaired glucose tolerance, increased blood pressure (BP), and insulin-dependent dopamine-related behavior (42, 44, 45). Are these changes relevant for cases of moderate culling when litter size is reduced less drastically, to 8–10 pups? The Sprague Dawley rat dataset from the Huntingdon Research Center (11) describes distribution of body weight at weaning in relation to litter size at birth. These measures were examined for more than 200 unculled and culled litters combined (Figure 1). Unsurprisingly, pup weight in unculled litters negatively correlates with litter size, similar to what has been reported in many other studies (5, 5, 6). However, surprisingly, in culled litters, this correlation is positive, and the linear trend line is much steeper. This suggests that pups from bigger litters (13–16 pups) likely experience minor nutritional deficits during in utero development and early postnatal life, but after culling, milk resources are available that were designated for almost twice as many pups. This switch from undernourishment to overfeeding induces rapid catch-up growth during the suckling period and results in a significant increase in pups' body weight at weaning. Nutritional availability does not change drastically after culling of smaller litters, thus their growth remains relatively steady from gestation through weaning. In this case, these pups have lower body weights at weaning compared with their low birth weight (LBW) conspecifics from big litters.
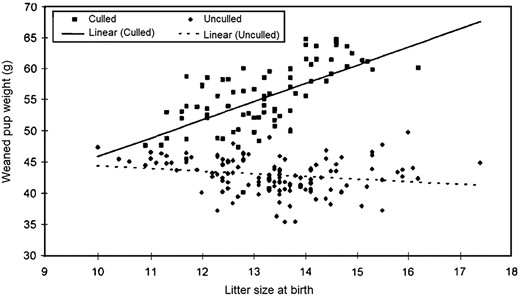
Group mean litter size at birth and weaned pup weight (from Ref. 11). These data demonstrate that pups experience catch-up growth after culling to 8 pups by postnatal day 4. The intensity of catch-up growth positively correlates with initial litter size. As a result, by the time of weaning, pups from the largest culled litters have attained the heaviest body weights.
A number of underlying biological mechanisms have been proposed to explain how early life malnutrition and subsequent catch-up growth could contribute to adult metabolic diseases. Studies from rodents have shown that malnutrition in fetal development alters blood flow away from the developing kidneys and liver to increase blood flow to the brain. This response, termed the “thrifty phenotype” allows individuals to survive challenging environments. David Barker proposed that problems would arise when these individuals were “programmed” to consume as many calories as possible in adulthood (46), especially when excess nutrients are available (47). Thus, if developing organisms experience a period of nutritional deficit during the prenatal or early postnatal period of development, they often demonstrate an accelerated compensatory growth when they gain improved access to nutrients (48). This catch-up growth may itself result in long-term programing of an organism for metabolic and other abnormalities (49, 50). This hypothesis, designated as “grow now, pay later” by Metcalfe and Monaghan (50), suggests that there are evolutionary tradeoffs between the immediate benefits of accelerated growth (increased survival and reproduction) and the delayed adverse effects of this acceleration (metabolic diseases). As an illustrative example, rate of death from coronary disease was higher in human males who had LBW but an average or above average body weight at the age of 7 years compared with those with low body weight at this age (51). Many effects of LBW on metabolic, cardiovascular, and other health endpoints observed in human and animal studies may be attributed to catch-up growth, which is increasingly viewed as a long-term health hazard (52).
Another body of evidence linking rapid compensatory growth with adverse outcomes emerges from understanding that catch-up growth is characterized by different rates of growth of tissues and organs than typically occurs during normal development. Several reports suggest that catch-up growth induces a disproportionally faster rate of body fat growth (53). For example, in LBW infants, an increase in skinfold thickness, a measure of body fat composition, develops before body weight catch-up. In neonates, this fat accumulation is associated with rapid increase of IGF-1 and lipoprotein lipase concentrations (54). In experiments with juvenile rats, after growth arrest induced by 2 weeks of heavily restricted food intake followed by a return to normal diet, decreased thermogenesis was observed associated with accelerated fat deposition that was also accompanied with abnormally high plasma insulin during the catch-up period (55). Further, animals experiencing catch-up growth showed decreased insulin-stimulated glucose utilization in skeletal muscles but increased insulin-stimulated glucose utilization in adipose tissue (52). The authors suggest that “coordinated induction of skeletal muscle insulin resistance and adipose tissue insulin hyperresponsiveness, might be a central event in the link between catch-up growth, hyperinsulinemia and risks for later metabolic syndrome” (52).
Determining the long-term consequences of growth compensation is likely to be context-specific. For example, rats that experienced growth restriction during gestation have improved glucose tolerance compared with controls at 12 weeks of age, but glucose tolerance deteriorated faster than controls as animals age (56), developing frank diabetes in older adulthood (57). In experiments in which rat dams were fed a diet with reduced protein content, the longevity of male offspring was significantly increased if growth retardation was restricted to the period of suckling but decreased if growth retardation was restricted to gestation (56). Finally, when catch-up growth was induced via cold temperature in juvenile 3-spined sticklebacks, all fish attained the same average adult size, but catch-up growth led to a 14.5% reduction in median lifespan (58). These experiments also support the central role of catch-up growth, rather than nutrient deficiency itself, in long-term health reprograming.
It is challenging to separate effects of early life nutritional deficits from the effects of increased growth in a catch-up phase. The prevailing role of catch-up growth rather than nutritional deficit in programing of cardio-metabolic health in humans was recently illustrated by a systematic review and cryptanalysis of 39 population-based studies (59). LBW and catch-up growth were analyzed together with the following cardio-metabolic risk factors or diseases: body mass index, waist circumference, body weight, BP, cholesterol levels (low-density lipoprotein, high-density lipoprotein, and total), triglycerides, insulin resistance, insulin, glucose, impaired glucose tolerance, diabetes, hypertension, metabolic syndrome, coronary heart disease, cardiovascular disease, and type 2 diabetes. Significant associations were observed for all reported cardiovascular disease risk factors with catch-up growth (79.6%) and LBW (58.5%). Thus, postnatal catch-up growth of malnourished neonates is likely a more important risk factor for cardiovascular and metabolic disease than LBW alone (81).
Culling and Studies of EDCs
Like the effects of intrauterine position, studies of EDCs have illustrated that developing vertebrates are highly responsive to even slight changes in hormone concentration or hormone action (60–62). Studies from genetic knockout mice have similarly revealed interactions between altered hormone signaling and sex ratios of the litter during postnatal development (63, 64). An increasing number of studies have shown that a subset of EDCs, termed metabolism-disrupting chemicals, can alter body weight, responses to high-fat diets, induce type 2 diabetes, and alter food consumption behaviors, among other effects (65). Thus, the culling of litters during the study of EDCs raises a number of important issues:
Because even slight differences in culling (eg, culling to 8 pups in one study vs culling to 10 pups in another study) could induce inconsistencies in morphology, physiology, and behaviors (8), information about culling, including the number of pups in the final reconstituted litter and the final sex ratio of the litter and the degree to which it deviates from the prenatal (intrauterine) sex ratio and initial litter size, should be reported and considered in statistical analyses (Table 2).
Differences in culling should be acknowledged as potential sources of inconsistencies between studies, especially when EDC “replication” studies are attempted.
Studies using genetic knockout animals should consider both the genetic make-up and the sex ratio of pups within litters when drawing conclusions.
Variability itself should be viewed as an endpoint, because EDCs can increase variability in biological endpoints without necessarily altering the mean values. These effects may be more difficult to see when litters are culled.
Rare diseases and other abnormalities may be missed during toxicity assessments of EDCs if litters are culled.
Suggested Cofactors to Be Included in Statistical Models for the Analysis of the Data From Developmental Experiments Designed With and Without Culling
. | Litter Size and Composition-Related Factors Affecting Developmental Trajectories . | Known Biological Parameters Affected . | Cofactors to Be Included in Statistical Model . |
---|---|---|---|
No culling | Availability of nutrients during embryonic and suckling periods | Body weight, onset of developmental landmarks, timing of sexual maturation, reflex, locomotive, emotional, and memory development, fecundity | Litter size |
Litter sex environment | Social behavior, brain physiology, sexual maturation and sexual performance, maternal behavior, sex steroids secretion | Sex ratio in litter | |
Culling | Availability of nutrients before culling | Body weight, onset of developmental landmarks, timing of sexual maturation, reflex, locomotive, emotional, and memory development, fecundity | Litter size before culling |
Sex environment before culling | Sexual maturation, sex steroids secretion | Sex ratio before culling | |
Overfeeding and catch-up growth | Body weight, body composition, glucose and lipid metabolism, insulin secretion, BP, bone density, response to stress, attention, depression, lifespan | Litter size before and after culling | |
Sex environment after culling | Social behavior, brain physiology, sexual maturation and sexual performance, maternal behavior, sex steroids secretion, morphology and physiology | Sex ratio after culling |
. | Litter Size and Composition-Related Factors Affecting Developmental Trajectories . | Known Biological Parameters Affected . | Cofactors to Be Included in Statistical Model . |
---|---|---|---|
No culling | Availability of nutrients during embryonic and suckling periods | Body weight, onset of developmental landmarks, timing of sexual maturation, reflex, locomotive, emotional, and memory development, fecundity | Litter size |
Litter sex environment | Social behavior, brain physiology, sexual maturation and sexual performance, maternal behavior, sex steroids secretion | Sex ratio in litter | |
Culling | Availability of nutrients before culling | Body weight, onset of developmental landmarks, timing of sexual maturation, reflex, locomotive, emotional, and memory development, fecundity | Litter size before culling |
Sex environment before culling | Sexual maturation, sex steroids secretion | Sex ratio before culling | |
Overfeeding and catch-up growth | Body weight, body composition, glucose and lipid metabolism, insulin secretion, BP, bone density, response to stress, attention, depression, lifespan | Litter size before and after culling | |
Sex environment after culling | Social behavior, brain physiology, sexual maturation and sexual performance, maternal behavior, sex steroids secretion, morphology and physiology | Sex ratio after culling |
Suggested Cofactors to Be Included in Statistical Models for the Analysis of the Data From Developmental Experiments Designed With and Without Culling
. | Litter Size and Composition-Related Factors Affecting Developmental Trajectories . | Known Biological Parameters Affected . | Cofactors to Be Included in Statistical Model . |
---|---|---|---|
No culling | Availability of nutrients during embryonic and suckling periods | Body weight, onset of developmental landmarks, timing of sexual maturation, reflex, locomotive, emotional, and memory development, fecundity | Litter size |
Litter sex environment | Social behavior, brain physiology, sexual maturation and sexual performance, maternal behavior, sex steroids secretion | Sex ratio in litter | |
Culling | Availability of nutrients before culling | Body weight, onset of developmental landmarks, timing of sexual maturation, reflex, locomotive, emotional, and memory development, fecundity | Litter size before culling |
Sex environment before culling | Sexual maturation, sex steroids secretion | Sex ratio before culling | |
Overfeeding and catch-up growth | Body weight, body composition, glucose and lipid metabolism, insulin secretion, BP, bone density, response to stress, attention, depression, lifespan | Litter size before and after culling | |
Sex environment after culling | Social behavior, brain physiology, sexual maturation and sexual performance, maternal behavior, sex steroids secretion, morphology and physiology | Sex ratio after culling |
. | Litter Size and Composition-Related Factors Affecting Developmental Trajectories . | Known Biological Parameters Affected . | Cofactors to Be Included in Statistical Model . |
---|---|---|---|
No culling | Availability of nutrients during embryonic and suckling periods | Body weight, onset of developmental landmarks, timing of sexual maturation, reflex, locomotive, emotional, and memory development, fecundity | Litter size |
Litter sex environment | Social behavior, brain physiology, sexual maturation and sexual performance, maternal behavior, sex steroids secretion | Sex ratio in litter | |
Culling | Availability of nutrients before culling | Body weight, onset of developmental landmarks, timing of sexual maturation, reflex, locomotive, emotional, and memory development, fecundity | Litter size before culling |
Sex environment before culling | Sexual maturation, sex steroids secretion | Sex ratio before culling | |
Overfeeding and catch-up growth | Body weight, body composition, glucose and lipid metabolism, insulin secretion, BP, bone density, response to stress, attention, depression, lifespan | Litter size before and after culling | |
Sex environment after culling | Social behavior, brain physiology, sexual maturation and sexual performance, maternal behavior, sex steroids secretion, morphology and physiology | Sex ratio after culling |
Concluding Remarks
Culling is used in rodent experiments to control litter size, a variable that influences the rate of development and therefore affects many health-related endpoints including body weight, onset of developmental landmarks, sexual maturation, neurodevelopment, female fecundity, and embryo mortality. Emerging evidence indicates that culling, especially of large litters, can drastically change the feeding status of a pup, which can result in compensatory growth with long-term consequences for the animal including increased risk of metabolic diseases. Similarly, culling of litters to intentionally bias sex ratios can alter the animal's behavior and physiology, with effects observed on a wide range of outcomes.
In an attempt to control for variability in developmental rates, culling introduces an uncontrolled variable which itself may affect a broad spectrum of health-related consequences (Table 2). Thus, the question “to cull or not to cull?” may be converted into a more rational and biologically oriented question: What is easier to control in a rodent experiment, the natural variability associated with differences in litter sizes and sex ratios, or the downstream consequences of altering these factors, many of which may not be known? When researchers are interested in endocrine endpoints, including metabolism, it is likely to be easier to leave litters unculled in favor of larger litter sizes with variable sex ratios. This type of variability is intrinsic to animal models and effects of treatments on variability may shed important light on how EDCs and other environmental factors influence development.
The evidence presented in this minireview indicates that litter size and sex composition are factors that do not change their vectors throughout prenatal development, the suckling period, and juvenile development. Therefore, these factors may be easily introduced in multivariate statistical models as adjustment factors. If culling cannot be avoided, statistical models may need to be adjusted to account for aspects such as sex ratios and catch-up growth.
Acknowledgments
This work was supported by start-up funds from the University of Massachusetts (A.S.). L.N.V. was supported by start-up funds from the University of Massachusetts and by the National Institute of Environmental Health Sciences of the National Institutes of Health Award K22ES025811.
Disclosure Summary: L.N.V. has been reimbursed for travel expenses by organizations, including SweTox, Israel Environment Fund, Beyond Benign, the Mexican Endocrine Society, Advancing Green Chemistry, ShiftCon, United States Environmental Protection Agency, CropLife America, the World Federation of Scientists, BeautyCounter, and many universities, to speak about endocrine-disrupting chemicals. A.S. has nothing to disclose.