-
PDF
- Split View
-
Views
-
Cite
Cite
Annett Hoffmann, Gloria-Maria Manjowk, Isabel Viola Wagner, Nora Klöting, Thomas Ebert, Beate Jessnitzer, Ulrike Lössner, Jan-Bernd Stukenborg, Matthias Blüher, Michael Stumvoll, Olle Söder, Konstantin Svechnikov, Mathias Fasshauer, Susan Kralisch, Leptin Within the Subphysiological to Physiological Range Dose Dependently Improves Male Reproductive Function in an Obesity Mouse Model, Endocrinology, Volume 157, Issue 6, 1 June 2016, Pages 2461–2468, https://doi.org/10.1210/en.2015-1966
- Share Icon Share
Obesity has recently been linked with reduced fertility, and the mechanisms underpinning this effect are currently unknown. The adipokine leptin is dysregulated in obesity and affects reproductive tracts; therefore, we investigated the dose-dependent effects of leptin on Leydig cell function and spermatogenesis. Eight-week-old leptin-deficient obese (ob/ob) male mice were treated with subphysiological (0.1- or 0.5-mg/kg body weight [BW]/d) or physiological (3.0-mg/kg BW/d) doses of leptin or saline for 12 weeks (chronic treatment) or 72 hours (acute treatment). We then evaluated male reproductive function markers. Mean testis weight increased significantly in the 0.1- and 3.0-mg/kg BW/d groups compared with saline controls (both P < .05). Intratesticular testosterone levels relative to testis weight significantly increased in the 0.5-mg/kg BW/d group compared with saline controls (P < .05). FSH levels increased in a dose-dependent manner with leptin treatment, whereas LH levels did not change. Leptin treatment significantly up-regulated both mRNA and protein expression of the steroidogenic enzyme cytochrome P450 17A1. Spermatogenesis improved in leptin-treated animals. Significantly more seminiferous tubules were observed in stages I–VIII (P < .01), and there were fewer abnormal seminiferous tubule structures (P < .01). Acute treatment with physiological leptin doses partially improved male reproductive markers without changing BW. Administration of subphysiological to physiological doses of leptin improves Leydig cell function and spermatogenesis.
Obesity plays a major role in the development of metabolic syndrome-related comorbidities such as hypertension, dyslipidemia, and insulin resistance, which reduce quality of life and life span (1). There is mounting evidence supporting the hypothesis that obesity adversely affects male reproductive function (2, 3). It has been shown that male obesity negatively impacts sperm motility (4), alters acetylation status in late round spermatids, and is correlated with increased DNA damage in germ cells (5). Several epidemiological studies have demonstrated a negative correlation between obesity measures and testosterone levels (6–8). However, the factors linking obesity and fertility have yet to be elucidated.
Adipokines, proteins secreted from adipocytes, become dysregulated when weight increases, and have been implicated as key factors in various obesity-related complications (9). Dysregulation of adipokines could also contribute to the molecular mechanisms behind obesity-related male infertility (10). The most prominent adipokines, including adiponectin, leptin, and their associated receptors, have been isolated in male reproductive tracts (10–13). Adiponectin has general insulin-sensitizing and atheroprotective roles, but at the testicular level, it supports male reproductive function by promoting steroidogenesis (13). Leptin, when administered to leptin-deficient mice and humans, generally reduces food intake, body weight (BW), and fat mass (14, 15), and improves fertility in rodents (16, 17).
Treatment with leptin produces different effects on metabolic syndromes depending on the concentration used. At subphysiological levels (ie, doses not sufficient to normalize BW) to physiological levels (ie, doses sufficient to normalize BW), leptin administration improves atherosclerotic disease in leptin-deficient mice compared with littermate controls (18). Other studies have confirmed the atheroprotective effects of low-dose leptin treatment (19, 20). In contrast, high doses of leptin consistently aggravate atherosclerotic disease (21, 22). Subphysiological doses of leptin also stimulate other biological processes, such as lipolysis (23). Notably, leptin demonstrates dose-dependent effects on hypothalamic-pituitary fertility regulation. Subnanomolar concentrations induce GnRH secretion from rat median eminence-arcuate nuclear explants ex vivo; however, at micromolar concentrations, the stimulatory effect is lost (24). Both low leptin levels, such as those seen in congenital leptin-deficiency and lipodystrophy, and high leptin levels, observed in obesity, are associated with impaired fertility (3, 25–28).
To date, the dose-dependent effects of adipokines on in vivo testicular functions, such as steroidogenesis and spermatogenesis, have not been elucidated. To address this knowledge gap, we treated leptin-deficient mice with mouse recombinant leptin using fixed doses ranging from subphysiological to physiological levels. We hypothesized that subphysiological to physiological levels of leptin would improve male reproductive function in a dose-dependent manner by affecting both steroidogenesis through Leydig cell function and spermatogenesis through germ cell and Sertoli cell function.
Materials and Methods
Animal care
The local ethics committee of the state of Saxony (Regierungspräsidium Leipzig) approved all animal-experiment protocols (approval number TVV37/12). All animal experiments were performed in the Medical Experimental Center at the University of Leipzig. All mice were maintained under pathogen-free conditions at 21 ± 1°C on a 12-hour light, 12-hour dark cycle (6 am to 6 pm). The study presented here was part of a larger study elucidating dose-dependent effects of leptin on atherogenesis (18) using ob/ob mice on a low-density lipoprotein (LDL) receptor knockout background.
Animal treatment
Treatments in ob/ob mice started at 8 weeks of age, because male reproductive maturation is complete and gonadotropin levels are stable at this time point (29). We performed 2 sets of treatment experiments. To model chronic leptin administration, BW-matched male mice were separated into 4 groups and treated daily over a period of 12 weeks. We administered saline or 1 of 3 recombinant mouse leptin concentrations (0.1-, 0.5-, or 3.0-mg/kg BW; R&D Systems) ip. To model leptin administration over an acute time frame, BW-matched male mice were separated into 2 groups, and ip saline or 3.0-mg/kg BW recombinant mouse leptin was administered daily over a period of 72 hours. At the end of their respective treatment periods, mice were sacrificed by exsanguination under deep anesthesia with ketamine (WDT) und xylazine (Bayer Health Care).
Measurement of FSH, LH, and intratesticular testosterone
FSH and LH were quantified in plasma samples using a commercial multiplex ELISA (Merck Millipore). Steroids were extracted from mouse testis using the protocol described by Robertson et al (30). Briefly, the tissue was weighed and sonicated. Testosterone was extracted with ethyl acetate (Sigma-Aldrich) and evaporated overnight. The pellet was resuspended in phosphate buffered saline, and intratesticular testosterone was assessed using ELISA (DRG Instruments GmbH) according to the manufacturer's instructions.
Histological analysis
Testes were fixed in 4% paraformaldehyde and processed for paraffin embedding and sectioning. We evaluated the effect of leptin treatment on the spermatogenic process by assessing the morphology of seminiferous tubules. Different spermatogenic stages were identified based on cell types defined by size, shape, and location in the seminiferous tubules as described by Russel et al (31). Ten randomly selected fields of hematoxylin/eosin-stained testis slices were evaluated per animal. Fifty tubules were analyzed per slide at ×20 magnification using a Nikon microscope (Nikon Instruments, Inc).
We quantified the Leydig cell marker cytochrome P450 (CYP)17A1 by performing immunohistochemistry on paraffin-embedded testis sections using a CYP17A1-specific primary antibody (catalog number sc66850; dilution: 1:100; Santa Cruz Biotechnology) and the Dako Real EnVision detection system (Dako) according to the manufacturer's instructions. No fewer than 5 randomly selected fields were evaluated per animal. All slides were photographed and analyzed manually by 2 independent evaluators using a Zeiss Axioskop microscope (Carl Zeiss Microscopy GmbH) along with Cell F image analysis (Olympus) and NIH Image/ImageJ (http://imagej.nih.gov/ij/).
Quantitative real-time RT-PCR analysis
Cyp17a1, Cyp11a1, 17β-hydroxysteroid dehydrogenase B3 (Hsd17b3), insulin-like peptide 3 (Insl3), LH receptor (Lhr), and steroidogenic acute regulatory protein (Star) mRNA levels were determined relative to acidic ribosomal phosphoprotein P0 (36B4) using quantitative real-time RT-PCR and a fluorescent temperature cycler (Roche) as described previously (32). Primer sequences used are summarized in Supplemental Table 1.
Data analysis and statistics
Datasets were analyzed using GraphPad Prism 6 (GraphPad Software). The statistical significance of the leptin treatment groups compared with saline controls was assessed using the Mann-Whitney U test, and P < .05 was considered statistically significant in all analyses.
Results
Leptin increases testis weight, intratesticular testosterone, and FSH
Chronic leptin treatment significantly decreased mean BW in a dose-dependent manner from 50.4 g in saline-treated controls to 36.5 g in the 0.1-mg leptin/kg BW/d group, 30.9 g in the 0.5-mg leptin/kg BW/d group, and 25.9 g in the 3.0-mg leptin/kg BW/d group at 12 weeks (P < .0001) (Figure 1A and Supplemental Figure 1A). The leptin-mediated decrease in BW was due to a significant decline in total fat mass rather than total lean mass (Supplemental Figure 1, B and C). Mean testis weight increased significantly in the 0.1- and 3.0-mg/kg BW/d groups (95.1 and 92.4 mg, respectively) compared with 86.5 mg in the saline controls (both P < .05) (Figure 1B).
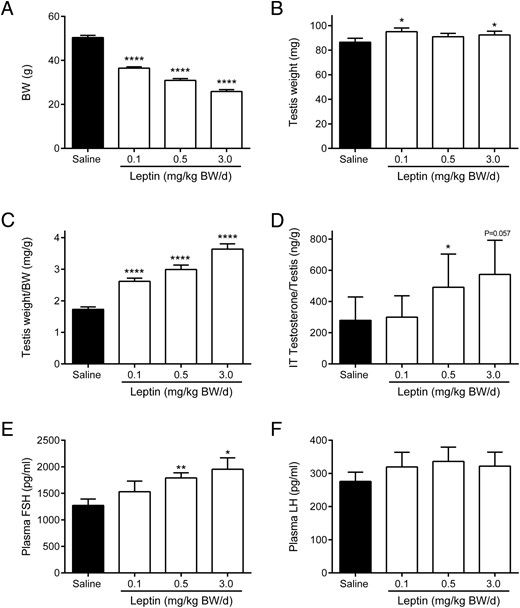
BW (A), absolute testis weight (B), testis weight relative to BW (C), intratesticular (IT) testosterone relative to testis weight (D), and plasma (E) FSH and (F) LH in chronic (12 wk) leptin administration groups. Data are presented as mean ± SE and represent n ≥ 6 per group; *, P < .05; **, P < .01; ****, P < .0001 compared with saline controls.
Testis weight relative to BW significantly increased in a dose-dependent manner in all leptin treatment groups compared with saline controls (P < .0001) (Figure 1C). Intratesticular testosterone relative to testis weight significantly increased to 491.5 ng/g in the 0.5-mg/kg BW/d group compared with 279.5 ng/g in saline controls (P < .05) (Figure 1D). There was also a trend (P = .057) towards higher intratesticular testosterone in the 3.0-mg/kg BW/d group (573.2 ng/g) (Figure 1D).
Plasma FSH levels significantly increased in a dose-dependent manner after leptin treatment from 1273 pg/mL in the saline controls to 1790 pg/mL in the 0.5-mg/kg BW/d group (P < .01) and 1954 pg/mL in the 3.0-mg/kg BW/d group (P < .05) (Figure 1E). In contrast, plasma LH levels were not significantly different between leptin-treated and control animals (Figure 1F).
Leptin increases markers of Leydig cell function
We performed a comprehensive analysis of steroidogenic gene expression to identify the molecular basis for increased testosterone production by Leydig cells after leptin treatment. Lhr expression is necessary for stimulating testosterone production in Leydig cells. Lhr was up-regulated approximately 2-fold in the 3.0-mg/kg BW/d group compared with the saline group (P < .001) (Figure 2A). Star plays a central role in initiating and supporting steroidogenesis in Leydig cells. Star was up-regulated by 1.9-fold in the 0.5-mg/kg BW/d group (P < .01) and 1.8-fold in the 3.0-mg/kg BW/d group (P < .05) (Figure 2B). Both testicular Cyp17a1 (Figure 2C) and Cyp11a1 (Figure 2D) mRNA expression showed dose-dependent up-regulation as high as 2.9-fold in the 3.0-mg/kg BW/d group compared with saline controls (P < .01). Expression of Hsd17b3, a key steroidogenic enzyme, increased 1.5-fold in the 0.5-mg/kg BW/d group (P < .05) and 1.9-fold in the 3.0-mg/kg BW/d group (P < .001) compared with saline controls (Figure 2E). Insl3, a marker of Leydig cell function, was up-regulated 1.7-fold in the 0.5-mg/kg BW/d group compared with saline controls (P < .05) (Figure 2F).
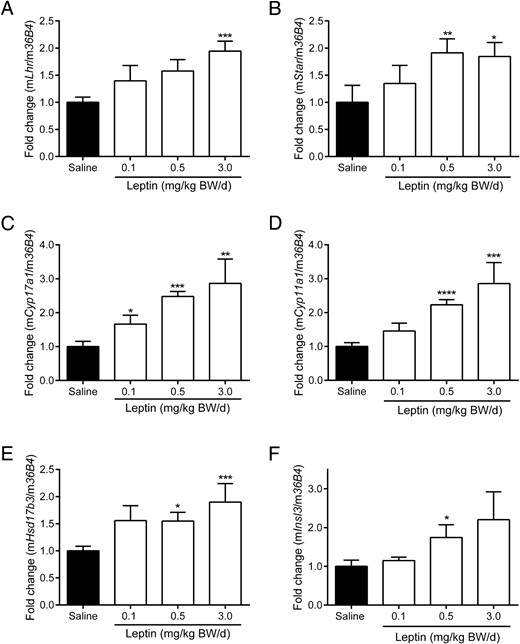
Lhr (A), Star (B), Cyp17a1 (C), Cyp11a1 (D), Hsd17b3 (E), and Insl3 (F) mRNA expression in testis in chronic (12 wk) leptin administration groups. Quantification of mRNA was performed as described in Materials and Methods. Data are presented as mean ± SE and represent n ≥ 7 per group; *, P < .05; **, P < .01; ***, P < .001; ****, P < .0001 compared with saline controls.
Consistent with the transcriptional data, immunohistochemical data showed the mean percent CYP17A1-positive area was 1.4-fold higher in the 3.0-mg/kg BW/d group than in saline controls (P < .05), indicating increased testicular CYP17A1 protein synthesis (Figure 3A).
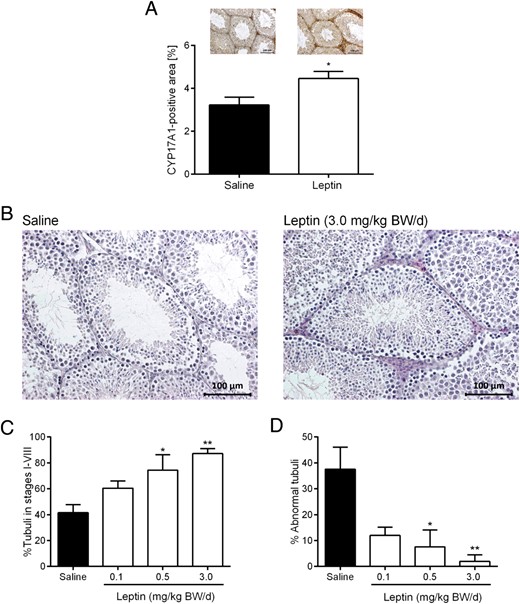
Testicular CYP17A1 protein synthesis (A), hematoxylin and eosin-stained testis sections (B), percent tubules in stages I–VIII (containing all spermatogenic stages including spermatogonia, spermatocytes, round spermatids, and elongated spermatids) (C), and percent abnormal tubules, defined as tubules undergoing spermatogenic arrests (D): 1) premeiotic arrest (only spermatogonia), 2) meiotic arrest (no round or elongated spermatids), or 3) postmeiotic arrest (no elongated spermatids present) in ob/ob mice treated with saline or leptin over 12 weeks. Data are presented as mean ± SE and represent n ≥ 4 per group. Representative images are shown in A and B; *, P < .05; **, P < .01 compared with saline.
Leptin improves markers of spermatogenesis
To assess whether improved Leydig cell function after leptin treatment is associated with improved spermatogenesis markers, we evaluated testes morphology. The lumen of seminiferous tubules appeared hollow in saline controls compared with the 3.0-mg/kg BW/d group (Figure 3B). Leptin significantly increased the percentage of seminiferous tubules in stages I through VIII, (ie, tubules containing spermatogonia, spermatocytes, round spermatids, and elongated spermatids) from 41.6% in controls to 87.2% in the 3.0-mg/kg BW/d group (P < .01) (Figure 3C). There was no significant difference between control and leptin-treated animals in the percentage of seminiferous tubules in stages IX through XII (ie, only excluding round spermatids present) (data not shown). Abnormal tubules were classified as having 1 of 3 different pathologies: 1) premeiotic arrest (only spermatogonia), 2) meiotic arrest (no round or elongated spermatids), and 3) postmeiotic arrest (no elongated spermatids). The fraction of abnormally structured seminiferous tubules significantly decreased in a dose-dependent manner after leptin treatment from 37.6% in control animals to 7.6% in the 0.5-mg/kg BW/d group (P < .05) and 2.0% in the 3.0-mg/kg BW/d group (P < .01) (Figure 3D).
We also investigated mRNA levels of Sertoli cell markers, including inhibin B (Inhbb), sex determining region Y-box (Sox)9, and vimentin (Vim), as well as germ cell markers, including DEAD box protein (Ddx)4, kit oncogene (Kit), and cAMP-responsive element modulator Crem). None of these markers were significantly affected by leptin treatment (Supplemental Figure 2).
Acute leptin treatment improves markers of male gonadal function independent of BW changes and metabolic improvements
Leptin-deficient ob/ob mice were treated daily with physiological leptin doses (3.0-mg/kg BW) over 72 hours to assess whether leptin influences male gonadal function independent of BW changes and metabolic improvements. Acute leptin administration had no significant effect on BW, testis weight, glycated hemoglobin A1c, fasting glucose, or cholesterol and triglycerides, including the LDL, high-density lipoprotein, and very-LDL fractions (Supplemental Figure 3). However, treatment with leptin for 72 hours induced a 2-fold increase in Lhr mRNA levels compared with controls (P < .01) (Figure 4A). Testicular mRNA expression of Cyp17a1 (Figure 4C), Cyp11a1 (Figure 4D), and Hsd17b3 (Figure 4E), all of which are downstream targets of LHR, was significantly up-regulated after leptin administration compared with saline controls (all P < .05). There was a trend towards increased Star mRNA levels (P = .10) (Figure 4B). No change was observed in Insl3 mRNA levels (Figure 4F) or plasma FSH and LH levels (Figure 4, G and H) under these conditions.
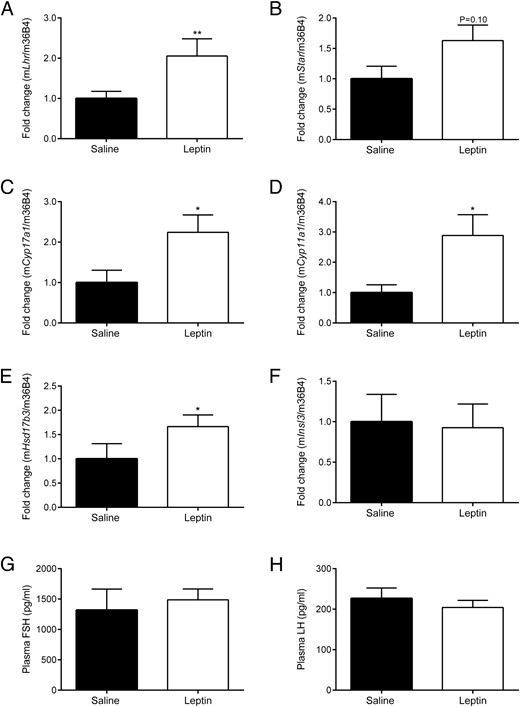
Influence of acute leptin administration (3.0-mg/kg BW/d for 72 h) on (A) Lhr, (B) Star, (C) Cyp17a1, (D) Cyp11a1, (E) Hsd17b3, and (F) Insl3 mRNA expression in testis and plasma (G) FSH and (H) LH levels. Quantification of mRNA expression and plasma FSH and LH concentrations was performed as described in Materials and Methods. Data are presented as mean ± SE and represent n ≥ 6 per group; *, P < .05; **, P < .01 compared with saline controls.
Discussion
Here, we show for the first time that administration of subphysiological to physiological levels of leptin improves male gonadal function markers in leptin-deficient mice in a dose-dependent manner. Our findings suggest that leptin exposure increases intratesticular testosterone levels and testis weight. These data also show that testis weight increased despite the animals' weight loss after leptin treatment. These findings are consistent with previous work by Mounzih et al who demonstrated that testis weight is significantly increased in leptin-deficient rodents after 60 days of leptin treatment (17). In this study, we aimed to elucidate the mechanisms by which leptin improves Leydig cell function. We show that leptin treatment induces Lhr gene expression in the testis. Because LH stimulates testosterone production and supports steroidogenic genes expression in Lhr-expressing Leydig cells (33), we hypothesize that leptin improves steroidogenesis through LH response in Leydig cells. We demonstrate that leptin induces mRNA expression of Star, which regulates the first steps of steroidogenesis (34), as well as genes associated with the sequential conversion of steroid precursors to testosterone, including Cyp17a1, Cyp11a1, and Hsd17b3 (35), in a dose-dependent manner. Leptin treatment induces Insl3, also known as Leydig insulin-like hormone, which is an indicator of differentiation status and functional Leydig cells (36). Given our results and those of previous studies, it seems likely that leptin improves Leydig cell function by inducing key steroidogenic enzymes. Additionally, these improvements are probably not dependent on metabolic and BW changes because Lhr, Cyp17a1, Cyp11a1, and Hsd17b3 were up-regulated in ob/ob mice treated with leptin for 72 hours. These in vivo results are consistent with those seen in an in vitro mouse Leydig cell model (MA-10 cells, data not shown). In that system, leptin significantly up-regulated Lhr mRNA by 1.4-fold, providing further evidence that leptin directly induces Lhr expression in Leydig cells.
Peptide/Protein Target . | Antigen Sequence (if Known) . | Name of Antibody . | Manufacturer, Catalog Number, and/or Name of Individual Providing the Antibody . | Species Raised in; Monoclonal or Polyclonal . | Dilution Used . |
---|---|---|---|---|---|
CYP17A1 | Epitope corresponding to amino acids 331-410 mapping near the C terminus of CYP17A1 of mouse origin | CYP17A1 (M80) | Santa Cruz Biotechnology, CYP17A1 (M-80):sc-66850 | Rabbit; polyclonal | 1:100 |
Peptide/Protein Target . | Antigen Sequence (if Known) . | Name of Antibody . | Manufacturer, Catalog Number, and/or Name of Individual Providing the Antibody . | Species Raised in; Monoclonal or Polyclonal . | Dilution Used . |
---|---|---|---|---|---|
CYP17A1 | Epitope corresponding to amino acids 331-410 mapping near the C terminus of CYP17A1 of mouse origin | CYP17A1 (M80) | Santa Cruz Biotechnology, CYP17A1 (M-80):sc-66850 | Rabbit; polyclonal | 1:100 |
Peptide/Protein Target . | Antigen Sequence (if Known) . | Name of Antibody . | Manufacturer, Catalog Number, and/or Name of Individual Providing the Antibody . | Species Raised in; Monoclonal or Polyclonal . | Dilution Used . |
---|---|---|---|---|---|
CYP17A1 | Epitope corresponding to amino acids 331-410 mapping near the C terminus of CYP17A1 of mouse origin | CYP17A1 (M80) | Santa Cruz Biotechnology, CYP17A1 (M-80):sc-66850 | Rabbit; polyclonal | 1:100 |
Peptide/Protein Target . | Antigen Sequence (if Known) . | Name of Antibody . | Manufacturer, Catalog Number, and/or Name of Individual Providing the Antibody . | Species Raised in; Monoclonal or Polyclonal . | Dilution Used . |
---|---|---|---|---|---|
CYP17A1 | Epitope corresponding to amino acids 331-410 mapping near the C terminus of CYP17A1 of mouse origin | CYP17A1 (M80) | Santa Cruz Biotechnology, CYP17A1 (M-80):sc-66850 | Rabbit; polyclonal | 1:100 |
In the chronic leptin administration experiments, ob/ob mice treated with leptin at subphysiological (0.5-mg/kg BW/d) to physiological (3.0-mg/kg BW/d) levels for 12 weeks showed a significant increase in plasma FSH levels, whereas plasma LH levels remained relatively unchanged. These results are consistent with those of Barash et al in which ob/ob animals treated with leptin over 2 weeks had significantly increased FSH, but not LH, levels (16). Unlike the chronic experiments, acute leptin administration did not affect plasma FSH and LH concentrations in ob/ob mice despite the significant change in Lhr, Cyp17a1, Cyp11a1, and Hsd17b3 mRNA expression. These findings support the hypothesis that some of the recovery observed in the chronic leptin administration group could be due to recovery of gonadotropic function rather than the direct effects of leptin on Leydig cells alone. Furthermore, chronic leptin-mediated decreases in body fat, seen with both subphysiological and physiological leptin levels, could also play a role in reproductive outcomes.
The signaling mechanisms by which subphysiological to physiological levels of leptin mediate their effects on male gonadal function need to be investigated in future studies. Leptin is known to act through well-established pathways and expression of targets, including signal transducer and activator of transcription 3 and p44/42 mitogen-activated protein kinase phosphorylation, and suppressor of cytokine signaling-3 (37); however, in our study, these targets were not up-regulated in the testis after either chronic or acute leptin treatment (data not shown). These data indicate that leptin might mediate its effects on testicular function using alternative pathways.
Although leptin has beneficial effects on male reproductive function at subphysiological to physiological levels, supraphysiological leptin levels, such as those seen with common obesity, might directly impair Leydig cell function. This hypothesis would explain why hyperleptinemic mice have decreased reproductive parameters, including testosterone, testis weight, Leydig cell number, and offspring number (38). Tena-Sempere et al convincingly demonstrated that recombinant leptin significantly inhibits chorionic gonadotropin-stimulated testosterone secretion, which is accompanied by impaired gene expression of Star and Cyp11, but not Hsd17b3, in rat testis tissue ex vivo (39). Acute incubation of rat Leydig cells with recombinant leptin also significantly inhibits human chorionic gonadotropin-stimulated testosterone production ex vivo (40). Thus, leptin could have differential effects on Leydig cell function depending on leptin concentration, treatment duration, and experimental design; ie, in in vivo vs ex vivo settings.
Here, we show that subphysiological to physiological leptin doses improve spermatogenesis markers. Leptin significantly increased the number of tubules in the first half of the spermatogenic cycle (stages I–VIII) compared with saline-treated mice and acted in a dose-dependent manner. Leptin-treated animals had significantly decreased abnormal tubules compared with controls. However, germ cell markers, such as Ddx4, Kit, and Crem (41), and Sertoli cell markers, including Inhbb, Sox9, and Vim (41), were not significantly modulated by leptin treatment in our study. This result is consistent with a previous report by Mounzih et al, in which they show that seminiferous tubules become more abundant and contain mature germ cells after leptin treatment of ob/ob mice (17). Leptin levels in seminal plasma positively correlate with sperm concentration and motility in humans (10), and leptin significantly increases progressive and total sperm motility, acrosome reaction, and nitric oxide production in sperm (42, 43). Based on our results and those of previous studies, subphysiological to physiological leptin levels appear to improve both spermatogenesis and Leydig cell function. Whether these observed leptin-mediated changes in male gonadal function translate into an effect on actual offspring is currently unknown and should be addressed in the future.
In summary, leptin administered at subphysiological to physiological levels improves reproductive function via improved Leydig cell function, increased intratesticular testosterone, and improved spermatogenesis parameters. Further studies are needed to elucidate the effects of supraphysiological leptin concentrations, such as those seen in common obesity, on reproductive function markers.
Acknowledgments
We thank Eva Jung, Eva Böge, Claudia Gebhardt, and Jessica-Michelle Jannicke of the University of Leipzig for their excellent technical assistance.
This work was supported by Deutsche Forschungsgemeinschaft Grants SFB 1052/1, C06 (to M.F.), B01 (to M.B.), and B04 (to N.K.); the Federal Ministry of Education and Research (BMBF), Germany Grants FKZ: 01EO1001 (IFB Adiposity Diseases, Projects K6a-87) (to M.F.) and FKZ: 01EO1501 (to N.K.); the Deutsche Gesellschaft für Andrologie (S.K.); and the Deutsche Hochdruckliga e.V. (M.F.). I.V.W. is supported by the Integrated Research and Treatment Center (IFB) Adiposity Diseases, a European Society for Paediatric Endocrinology Research Fellowship, Samariten Stiftelsen, and Skällskapet Bårnavard.
Disclosure Summary: The authors have nothing to disclose.
Abbreviations
- BW
body weight
- CYP
cytochrome P450
- Hsd17b3
17β-hydroxysteroid dehydrogenase B3
- Insl3
insulin-like peptide 3
- LDL
low-density lipoprotein
- Lhr
LH receptor
- Star
steroidogenic acute regulatory protein.
References
Author notes
A.H., G.-M.M., and I.V.W. contributed equally to this work.
M.F. and S.K. contributed equally to this work.