-
PDF
- Split View
-
Views
-
Cite
Cite
Delphine Franssen, Arlette Gérard, Benoit Hennuy, Anne-Françoise Donneau, Jean-Pierre Bourguignon, Anne-Simone Parent, Delayed Neuroendocrine Sexual Maturation in Female Rats After a Very Low Dose of Bisphenol A Through Altered GABAergic Neurotransmission and Opposing Effects of a High Dose, Endocrinology, Volume 157, Issue 5, 1 May 2016, Pages 1740–1750, https://doi.org/10.1210/en.2015-1937
- Share Icon Share
Abstract
Rat sexual maturation is preceded by a reduction of the interpulse interval (IPI) of GnRH neurosecretion. This work aims at studying disruption of that neuroendocrine event in females after early exposure to a very low dose of bisphenol A (BPA), a ubiquitous endocrine disrupting chemical. Female rats were exposed to vehicle or BPA 25 ng/kg·d, 25 μg/kg·d, or 5 mg/kg·d from postnatal day (PND)1 to PND5 or PND15. Exposure to 25 ng/kg·d of BPA for 5 or 15 days was followed by a delay in developmental reduction of GnRH IPI studied ex vivo on PND20. After 15 days of exposure to that low dose of BPA, vaginal opening tended to be delayed. In contrast, exposure to BPA 5 mg/kg·d for 15 days resulted in a premature reduction in GnRH IPI and a trend toward early vaginal opening. RNA sequencing analysis on PND20 indicated that exposure to BPA resulted in opposing dose effects on the mRNA expression of hypothalamic genes involved in gamma aminobutyric acid A (GABAA) neurotransmission. The study of GnRH secretion in vitro in the presence of GABAA receptor agonist/antagonist confirmed an increased or a reduced GABAergic tone after in vivo exposure to the very low or the high dose of BPA, respectively. Overall, we show for the first time that neonatal exposure to BPA leads to opposing dose-dependent effects on the neuroendocrine control of puberty in the female rat. A very low and environmentally relevant dose of BPA delays neuroendocrine maturation related to puberty through increased inhibitory GABAergic neurotransmission.
Bisphenol A (BPA), a most ubiquitous endocrine-disrupting chemical (EDC), is used in the production of polycarbonate plastics and epoxy resins (1). In a recent review of 13 studies addressing the issue of BPA effects on female rodent sexual maturation (2), exposure during the first 2 weeks after birth was associated with either normal (3–6) or early vaginal opening (VO) (4, 6–8) depending on the dose of BPA but without consistent dose-response effect. None among those studies found puberty to be delayed. A neuroendocrine mechanism was suggested in some studies (7, 9) and invalidated in others (4). In the 13 studies summarized in the above-mentioned review, BPA was used in the μg/kg·d or mg/kg·d body weight ranges of daily exposure (2). So far, pubertal timing had never been studied after BPA administration in the ng/kg·d range, which is consistent with current human exposure (0.01–1 μg/kg·d) (10). In humans, equivocal data have been obtained. BPA excretion was not associated with changes in pubertal timing in several studies (11–13). Increased urinary BPA levels were observed either in precocious puberty (14) or in relation with late menarche (15). The purpose of the present study was to further elucidate the impact of the dose of BPA on female puberty and the underlying neuroendocrine mechanism with emphasis on rat exposure to low environmentally relevant doses during the early postnatal window. The mechanistic issue was addressed using an ex vivo paradigm of pulsatile GnRH secretion from hypothalamic explants (16) that was shown to be affected by other EDCs in previous studies (17, 18).
The effects of EDCs on female puberty can result either from peripheral effects at the level of estrogen sensitive tissues, eg, breast, uterus, and vagina, or from an activation of the hypothalamic-pituitary-ovarian axis (19, 20). The question arises as to whether early life exposure to BPA can affect the neuroendocrine control of puberty. During perinatal life, a critical window for organization of the central control of the reproductive axis, the female neuroendocrine system is particularly sensitive to sex steroids. In previous studies, we have shown that a single estradiol administration caused central sexual precocity and disrupted the central mechanism of ovulation (21). Also, early postnatal exposure of female rats to the estrogenic insecticide dichlorodiphenyltrichloroethane resulted in early neuroendocrine maturation with precocious VO (17). Neonatal exposure to diethylstilbestrol (DES) for 5 days was also associated with early puberty after a dose of 10 μg/kg·d and, unexpectedly, with a delayed puberty preceded by a delayed maturation of GnRH secretion after 1 μg/kg·d of DES (18).
Because we used exposure to DES for 5 days during the neonatal programming window in our previous study, a similar window of exposure was used initially for BPA. In such conditions, effects on GnRH secretion were observed after exposure to 25 ng/kg·d and 5 mg/kg·d, whereas no effect was seen using 25 μg/kg·d. None of the 3 doses was associated with differences in pubertal timing. Therefore, we studied the effects of a longer exposure (15 d) to the lowest and the highest doses in further experiments. For the first time in investigating puberty, we used a very low dose of BPA of (25 ng/kg·d), which is an equivalent low dose of environmental exposure in humans (10). The delaying effects of this low dose on developmental changes in GnRH secretion and puberty were opposed to the acceleration seen after exposure to 5 mg/kg·d. This contrast provided the rationale for RNA sequencing study to delineate possible opposing changes in mRNA expression of some hypothalamic genes involved in the maturational control of GnRH secretion. It appeared that the most significantly affected genes were related to gamma aminobutyric acid (GABA) neurotransmission, a finding confirmed using quantitative PCR (qPCR). The involvement was interesting given the developmental reduction in inhibitory GABA control of GnRH secretion that was substantiated in the primate in the period preceding the onset of puberty (22, 23) and also shown to occur in the rat using our hypothalamic explant paradigm (24, 25).
Materials and Methods
Animal care and exposure
Female Wistar rats were purchased from the University of Liège. They were housed in standardized conditions (22.8°C, lights on from 6:30 am to 6:30 pm) and mated. Litters were standardized for size and sex ratio on the first postnatal day (PND) of life in order to have 10–12 pups per litter and an identical number of males and females. The day of birth was considered as PND1. Weaning occurred at 21 days of age. In order to avoid a litter effect, a maximum of 2 pups coming from the same dam were studied in a same group for a given experiment. In each litter, female pups were exposed either to corn oil or to one of the 3 BPA doses. The animals were raised in BPA-free cages (polypropylene cages, Ref 1291H006; Tecnilab) and fed EDC- and phytoestrogen-free chow (V135 R/Z low phytoestrogen pellets; SSNIFF Diet). Water was supplied in glass bottles. Beginning on PND1, female pups were sc injected with vehicle (corn oil) or BPA 25 ng/kg·d (consistent with low environmental exposure) or 5 mg/kg·d (10 times below low observed adverse effect level or an intermediate dose of 25 μg/kg·d. BPA (Ref 23,9658; Sigma-Aldrich) was dissolved in ethanol at an initial concentration of 100 mg/mL and then diluted in corn oil. Injections (0.05 mL) were given every 24 hours, between 11 am and 1 pm, from PND1 to PND5 or from PND1 to PND15. All experiments were carried out with the approval of the Belgian Ministry of Agriculture and the Ethical Committee at the University of Liège.
Experimental design
Effects of exposure to BPA from PND1 to PND5 on pubertal timing and GnRH secretion
Ninety female pups from 16 dams were exposed from PND1 to PND5 to corn oil, 25 ng/kg·d, 25 μg/kg·d, or 5 mg/kg·d of BPA. Females were killed on PND15 (n = 16), PND20 (n = 12), or PND25 (n = 8) to study GnRH secretion ex vivo (4 animals/ BPA dose group), whereas 54 animals were followed for VO and estrous cyclicity (n = 6–14/ BPA dose group and 21 controls).
Effects of exposure to BPA from PND1 to PND15 on pubertal timing, GnRH secretion, and hypothalamic gene expression
A total of 104 female pups born from 18 dams were exposed to corn oil, 25 ng/kg·d or 5 mg/kg·d of BPA from PND1 to PND15. Thirty-four females were followed for VO/estrous cyclicity (n = 8–14/group), whereas 46 were killed on PND20 to study GnRH secretion ex vivo. Explants were incubated in control medium or in the presence of 10−4M bicuculline (B7561; Sigma) or muscimol (M1523; Sigma) (n = 4 to 10/group) (25). In 24 females also killed on PND20, the retrochiasmatic hypothalamus was dissected and RNA was extracted for RNA sequencing or real-time PCR (n = 8/control and each BPA dose group). In Supplemental Table 1, the number of animals per experiment is specified.
VO and estrous cyclicity
From PND25, female rats were examined daily for imperforation of the vaginal membrane to determine age at VO. Thereafter, vaginal smears were taken every day in the afternoon until PND80 as described previously (18). A regular cycle was defined by a regular sequence of diestrus 1, diestrus 2, proestrus, and estrus (26).
Hypothalamic explant incubation and GnRH assay
GnRH pulse frequency was studied through calculation of the interpulse interval (IPI) using GnRH release from female rat hypothalamic explants. GnRH secretion was studied after exposure to different doses of BPA from PND1 to PND5 or PND15 (Supplemental Table 1). After exposure to corn oil (control) or different doses of BPA from PND1 to PND5, 16 explants were studied on PND15 (corn oil and BPA 25 ng/kg·d, 25 μg/kg·d, or 5 mg/kg·d) and 12 explants on PND20 after exposure to the low (25 ng/kg·d) or the high (5 mg/kg·d) dose of BPA. Eight explants were also studied on PND25 after exposure to the low dose of BPA (25 ng/kg·d) from PND1 to PND5. After a longer exposure (PND1 to PND15) to the low (25 ng/kg·d) or the high (5 mg/kg·d) dose of BPA, explants were studied on PND20 (8 controls, 16 low dose of BPA, and 22 high dose). The GnRH IPI was studied in the presence of a GABAA receptor agonist, muscimol (10−4M) and an antagonist, bicuculline (10−4M). In previous in vitro studies using hypothalamic explants, a high GABAergic inhibitory tone on GnRH release was revealed by a reduction of the IPI in the presence of the antagonist bicuculline on PND15. On PND25, a lowered GABAergic tone was suggested by the disappearance of bicuculline effects and the occurrence of an increase in GnRH IPI caused by the agonist muscimol (24, 25). We used the same conditions here including bicuculline and muscimol concentrations (10−4M), to investigate the effects of BPA on the regulation of GnRH secretion by GABAergic neurotransmission.
After decapitation, a tissue fragment including the preoptic region and the medial basal hypothalamus was rapidly dissected, as described in Supplemental Materials and Methods. Each explant was transferred into an individual chamber in a static incubator (21). The incubation medium was collected and renewed every 7.5 minutes for a period of 4 hours. The GnRH release in the incubation medium was measured in duplicate using a RIA method with intra- and interassay coefficients of variation of 14% and 18%, respectively (27, 28). The highly specific anti-GnRH antiserum (CR11-B81; final dilution 1:80 000) was kindly provided by Dr V. D. Ramirez (Urbana, IL) (29). The properties of this antibody are given in Table 1. Data below the limit of detection (5 pg/7.5-min fraction) were assigned that value.
Peptide/Protein Target . | Antigen Sequence (if Known) . | Name of Antibody . | Manufacturer, Catalog Number, and/or Name of Individual Providing the Antibody . | Species Raised in; Monoclonal or Polyclonal . | Dilution Used . |
---|---|---|---|---|---|
GnRH | pGlu-His-Trp-Gly-Leu-Arg-Pro-Gly-NH2 | CR11-B81 | Dr V. D. Ramirez (Urbana, IL) | Rabbit; polyclonal | 1:80 000 |
Peptide/Protein Target . | Antigen Sequence (if Known) . | Name of Antibody . | Manufacturer, Catalog Number, and/or Name of Individual Providing the Antibody . | Species Raised in; Monoclonal or Polyclonal . | Dilution Used . |
---|---|---|---|---|---|
GnRH | pGlu-His-Trp-Gly-Leu-Arg-Pro-Gly-NH2 | CR11-B81 | Dr V. D. Ramirez (Urbana, IL) | Rabbit; polyclonal | 1:80 000 |
Peptide/Protein Target . | Antigen Sequence (if Known) . | Name of Antibody . | Manufacturer, Catalog Number, and/or Name of Individual Providing the Antibody . | Species Raised in; Monoclonal or Polyclonal . | Dilution Used . |
---|---|---|---|---|---|
GnRH | pGlu-His-Trp-Gly-Leu-Arg-Pro-Gly-NH2 | CR11-B81 | Dr V. D. Ramirez (Urbana, IL) | Rabbit; polyclonal | 1:80 000 |
Peptide/Protein Target . | Antigen Sequence (if Known) . | Name of Antibody . | Manufacturer, Catalog Number, and/or Name of Individual Providing the Antibody . | Species Raised in; Monoclonal or Polyclonal . | Dilution Used . |
---|---|---|---|---|---|
GnRH | pGlu-His-Trp-Gly-Leu-Arg-Pro-Gly-NH2 | CR11-B81 | Dr V. D. Ramirez (Urbana, IL) | Rabbit; polyclonal | 1:80 000 |
RNA sequencing
RNA sequencing analysis was carried out on total RNA extracted from retrochiasmatic hypothalamus of female rats on PND20 (control, n = 3; BPA 25 ng, n = 3; BPA 5 mg, n = 3). Previous studies have shown that the retrochiasmatic hypothalamus contains neurono-glial networks involved in the regulation of pulsatile GnRH secretion (24, 25, 30). The extraction was done with the RNeasy Mini kit (QIAGEN). Library preparation and sequencing were performed at the Genomics facility of the Interdisciplinary Cluster for Applied Genoproteomics Genomics (University of Liège). RNA integrity was verified on the Bioanalyser 2100 with RNA 6000 Nano chips, RNA integrity number scores were more than 8 for all samples. Illumina Truseq stranded mRNA Sample Preparation kit was used to prepare libraries from 1 μg of total RNA. Poly-A RNAs were purified with polyT-coated magnetic beads and chemically fragmented. They were used as template for first strand synthesis in the presence of random hexamers followed by second strand synthesis. cDNA ends were adenylated at 3′OH extremities before the ligation to adaptors containing the indexes. Finally, the adapters-ligated library fragments were enriched by PCR following Illumina's protocol and purified with Ampure XP magnetic beads. Libraries were validated on Bioanalyser DNA 1000 chip and quantified by qPCR with the KAPA library quantification kit. Sequencing was performed on Illumina NextSeq500. For the data analysis, Fastq files were trimmed for adaptor sequences. The reads were aligned with Tophat 2.0.9 (http://ccb.jhu.edu/software/tophat/index.shtml) to the rat genome. The number of reads per sample was between 11.106 and 22.106. The overall read aligment rate was between 77.7% and 83%. Cufflinks 2.2.0 suite (http://cole-trapnell-lab.github.io/cufflinks/manual/) was used to generate fragments per kilobase of transcript per million fragments mapped values and CuffDiff was used to identify significantly differentially expressed genes between the 3 conditions of treatment (q value, ie, corrected P ≤ .05). The data are presented in log2 (fold change) to allow a representation of an increase as a positive value and a decrease as a negative value on a single plot. More details concerning these softwares and their analytical and statistical methods are given in Supplemental Materials and Methods (31).
Data mining analysis
In order to identify the pathways most affected by BPA, significant RNA sequencing data were uploaded on QIAGEN's Ingenuity Pathway Analysis (IPA) (QIAGEN Redwood City). Canonical pathway analysis was performed on genes with a P < .05 in order to identify the pathways most affected by BPA. More information concerning this software and the analysis are given in Supplemental Materials and Methods.
Real-time PCR
qPCR analysis was used to assess mRNA levels of glutamic acid decarboxylase (GAD)2 and GABA transporter (GAT)2 on PND20 (n = 8/group). Total RNA was extracted from retrochiasmatic hypothalamus of female rats using the RNeasy Mini kit (QIAGEN). A total of 500 ng of RNA for each sample were reverse transcripted using the Transcriptor first strand cDNA synthesis kit (Roche). Real-time qPCRs were performed using FastStart Universal SYBR Green Master (Rox) (Roche) and a LightCycler 480 system (Roche). Samples were run in triplicate. Four microliters of cDNA (previously 5-fold diluted) were added to a mix of 5 μL of SYBR green, 0.3 μL of both forward and reverse primers, and 0.4 μL of nuclease-free water. Cycle threshold (Ct) values were obtained from each individual amplification curve, and the average Ct was calculated for each target gene in each sample. Quantification of relative gene expression was performed according to the 2−ΔΔCt method using β-actin as housekeeping gene (32). The primers sequences and information are provided in Supplemental Table 2.
Statistics
Numerical values were expressed as mean ± SEM. For the analysis of GnRH IPI and mRNA expression (after RT-qPCR), we performed a Student's t test to compare 2 groups of the same age. For data sets containing more than 2 groups of the same age, a one-way ANOVA followed by a Newman-Keuls multiple test was used. To compare more than 2 groups at different ages, a two-way ANOVA followed by a Newman-Keuls multiple test was used. To analyze the age at VO, a Cox regression model was applied. This model is an adaptation of probit analyses commonly used to study pubertal onset in humans (33). Probit analysis is a type of regression used with binomial response variable (in this study: VO, yes/no). Differences with P < .05 were considered to be statistically significant. Statistical analyses were carried out using Statistica software.
Results
Pubertal timing after exposure to BPA from PND1 to PND5
In agreement with our previous findings (16), maturation of GnRH secretion preceding puberty was characterized by a reduction of the GnRH IPI between PND15 and PND25 (Figure 1) using hypothalamic explants of control female rats ex vivo. Exposure to 25 ng/kg·d of BPA from PND1 to PND5 resulted in a significant increase of GnRH IPI on PND20 and PND25, consistent with delayed maturation. In contrast, exposure to 5 mg/kg·d of BPA resulted in a significant reduction of GnRH IPI on PND15 and PND20, consistent with advanced maturation. The intermediate dose of 25 μg/kg·d did not affect GnRH IPI. Although the low dose of BPA caused a slight delay of age at VO (Figure 1), there was no significant difference between age at VO among the 4 groups (control, BPA 25 ng/kg·d, BPA 25 μg/kg·d, and BPA 5 mg/kg·d). The BPA-exposed animals showed normal estrous cyclicity from VO until PND80. No significant difference in average weight was observed between the control and BPA groups from PND1 to PND90.
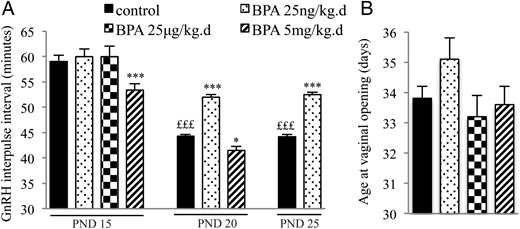
Effects of postnatal BPA exposure for 5 days on pulsatile GnRH secretion and female pubertal timing. A, GnRH IPI in vitro using hypothalamic explants obtained on PND15, PND20, or PND25 from female rats after exposure from PND1 to PND5 to vehicle, 25 ng/kg·d, 25 μg/kg·d, or 5 mg/kg·d of BPA. B, Age at VO in female rats exposed from PND1 to PND5 to vehicle, 25 ng/kg·d, 25 μg/kg·d, or 5 mg/kg·d of BPA. Data are mean ± SEM; *, P < .05 and ***, P < .001 vs control at the same age; £££, P < .001 vs control at PND15.
Pubertal timing after exposure to BPA from PND1 to PND15
After a longer exposure (from PND1 to PND15), the GnRH IPI on PND20 was significantly increased by the low dose of BPA and reduced by the high dose (Figure 2), similarly to the effects observed after a shorter exposure. As illustrated by the cumulative percentage curves in Figure 2A, exposure to the low dose of BPA tended to delay VO, whereas the high dose of BPA tended to advance VO. The Cox regression regarding age at VO revealed a significant difference (P = .016) between the 3 groups (control, BPA 25 ng/kg·d, and BPA 5 mg/kg·d). Pair analysis indicated that the difference was only significant between the groups exposed to the low and high doses of BPA (P = .0043) and not when these exposed groups were compared with controls (Figure 2). The BPA-exposed animals exhibited normal estrous cyclicity from VO until PND80. No significant difference in average weight was observed between the control and BPA groups from PND1 to PND90, suggesting that differences in maturation of GnRH secretion and pubertal timing did not result from differences in nutritional status.
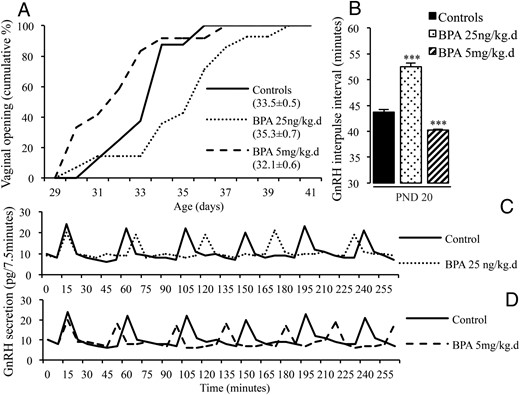
Effects of postnatal BPA exposure for 15 days on pulsatile GnRH secretion and female pubertal timing. A, Cumulative percentage of female rats displaying VO in relation to age in animals exposed from PND1 to PND15 to vehicle or 25 ng/kg·d or 5 mg/kg·d of BPA. Mean age at VO ± SEM for each group is indicated in brackets. B, GnRH IPI in vitro using hypothalamic explants obtained on PND20 from female rats after exposure from PND1 to PND15 to vehicle or 25 ng/kg·d or 5 mg/kg·d of BPA. Data are mean ± SEM. ***, P < .001, vs control group. C and D, Individual representative profiles of GnRH secretion after neonatal exposure to vehicle or BPA 25 ng/kg·d or 5 mg/kg·d.
BPA exposure and changes in hypothalamic mRNA expression
In order to identify the hypothalamic genes possibly involved in the dose-related opposing effects of BPA on GnRH secretion and pubertal timing, a RNA sequencing study was performed using retrochiasmatic hypothalamic extracts obtained on PND20 in female rats. After exposure to BPA 25 ng/kg·d and 5 mg/kg·d (PND1–PND15), the mRNA expression of 34 and 472 genes was significantly affected, respectively (q < 0.05 vs controls). When comparing the 2 BPA doses, 1407 genes were differentially expressed. A bioinformatic analysis (IPA) revealed that GABAA receptor neurotransmission was among the systems most affected by both doses of BPA (Figure 3), based on both the number of affected genes and the statistical significance of changes in expression. The 10 canonical pathways most affected by the 2 doses of BPA are listed in Supplemental Tables 3 and 4. The 2 doses resulted in opposing changes of mRNA expression of genes involved in GABA synthesis (GAD), reuptake (GAT), and signaling (GABAA receptor subunits) (Table 2). Using qPCR on a larger number of samples (n = 8), the increase in mRNA expression of GAD2 was confirmed after 5 mg/kg·d of BPA (1.3 ± 0.07 vs 1.04 ± 0.09 in control). Likewise, the increased mRNA expression of GAT2 was confirmed after 25 ng/kg·d of BPA (1.5 ± 0.27 vs 1.09 ± 0.13 in control).
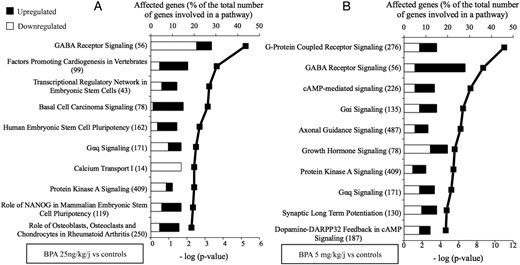
Hypothalamic pathways affected by 25 ng/kg·d or 5 mg/kg·d of BPA. A, Top 10 canonical pathways derived from IPA based on genes that are significantly down- or up-regulated in the retrochiasmatic hypothalamus on PND20 after 15 days of exposure to BPA 25 ng/kg·d. B, Top 10 canonical pathways derived from IPA based on genes that are significantly down- or up-regulated in retrochiasmatic hypothalamus on PND20 after 15 days of exposure to BPA 5 mg/kg·d. The pathways are ranked based on -log (P value) in order of decreasing significance that is illustrated by the bold line. The black and the white bars refer to the percentage of up-regulated and down-regulated genes that is calculated relative to the total number of genes involved in each pathway (given in the brackets).
Genes Related to GABA Neurotransmission Showing Significant Changes in Hypothalamic mRNA Expression After Exposure to Low or High BPA Doses From PND1 to PND15
Gene . | FPKM Control . | Down-Regulated log2 (Fold Change) . | P value . | Up-Regulated log2 (Fold Change) . | P value . | ||
---|---|---|---|---|---|---|---|
BPA 25 ng vs Control . | BPA 5 mg vs Control . | BPA 25 ng vs Control . | BPA 5 mg vs Control . | ||||
GAD1 | 65.87 | −0.19 | .05665 | 0.35 | .00065 | ||
GAD2 | 52.05 | −0.24 | .01670 | 0.44 | .00005 | ||
GAT2 | 5.34 | −0.60 | .0006 | 0.39 | .00885 | ||
GABRA2 | 16.69 | −0.18 | .10665 | 0.45 | .0015 | ||
GABRB3 | 17.95 | −0.16 | .1294 | 0.63 | .00075 | ||
GABRD | 20.44 | −1.09 | .00005 | 0.23 | .04135 | ||
GABRE | 6.49 | −0.15 | .1965 | 0.71 | .00005 | ||
GABRG2 | 43.47 | −0.04 | .73085 | 0.40 | .00075 | ||
GABRG3 | 3.79 | −0.88 | .0003 | 0.61 | .00405 | ||
GABRQ | 5.67 | −0.21 | .07385 | 0.70 | .00005 |
Gene . | FPKM Control . | Down-Regulated log2 (Fold Change) . | P value . | Up-Regulated log2 (Fold Change) . | P value . | ||
---|---|---|---|---|---|---|---|
BPA 25 ng vs Control . | BPA 5 mg vs Control . | BPA 25 ng vs Control . | BPA 5 mg vs Control . | ||||
GAD1 | 65.87 | −0.19 | .05665 | 0.35 | .00065 | ||
GAD2 | 52.05 | −0.24 | .01670 | 0.44 | .00005 | ||
GAT2 | 5.34 | −0.60 | .0006 | 0.39 | .00885 | ||
GABRA2 | 16.69 | −0.18 | .10665 | 0.45 | .0015 | ||
GABRB3 | 17.95 | −0.16 | .1294 | 0.63 | .00075 | ||
GABRD | 20.44 | −1.09 | .00005 | 0.23 | .04135 | ||
GABRE | 6.49 | −0.15 | .1965 | 0.71 | .00005 | ||
GABRG2 | 43.47 | −0.04 | .73085 | 0.40 | .00075 | ||
GABRG3 | 3.79 | −0.88 | .0003 | 0.61 | .00405 | ||
GABRQ | 5.67 | −0.21 | .07385 | 0.70 | .00005 |
Abbreviations: GABR, GABA receptor; FPKM, fragments per kilobase million (digital value of expression level).
Genes Related to GABA Neurotransmission Showing Significant Changes in Hypothalamic mRNA Expression After Exposure to Low or High BPA Doses From PND1 to PND15
Gene . | FPKM Control . | Down-Regulated log2 (Fold Change) . | P value . | Up-Regulated log2 (Fold Change) . | P value . | ||
---|---|---|---|---|---|---|---|
BPA 25 ng vs Control . | BPA 5 mg vs Control . | BPA 25 ng vs Control . | BPA 5 mg vs Control . | ||||
GAD1 | 65.87 | −0.19 | .05665 | 0.35 | .00065 | ||
GAD2 | 52.05 | −0.24 | .01670 | 0.44 | .00005 | ||
GAT2 | 5.34 | −0.60 | .0006 | 0.39 | .00885 | ||
GABRA2 | 16.69 | −0.18 | .10665 | 0.45 | .0015 | ||
GABRB3 | 17.95 | −0.16 | .1294 | 0.63 | .00075 | ||
GABRD | 20.44 | −1.09 | .00005 | 0.23 | .04135 | ||
GABRE | 6.49 | −0.15 | .1965 | 0.71 | .00005 | ||
GABRG2 | 43.47 | −0.04 | .73085 | 0.40 | .00075 | ||
GABRG3 | 3.79 | −0.88 | .0003 | 0.61 | .00405 | ||
GABRQ | 5.67 | −0.21 | .07385 | 0.70 | .00005 |
Gene . | FPKM Control . | Down-Regulated log2 (Fold Change) . | P value . | Up-Regulated log2 (Fold Change) . | P value . | ||
---|---|---|---|---|---|---|---|
BPA 25 ng vs Control . | BPA 5 mg vs Control . | BPA 25 ng vs Control . | BPA 5 mg vs Control . | ||||
GAD1 | 65.87 | −0.19 | .05665 | 0.35 | .00065 | ||
GAD2 | 52.05 | −0.24 | .01670 | 0.44 | .00005 | ||
GAT2 | 5.34 | −0.60 | .0006 | 0.39 | .00885 | ||
GABRA2 | 16.69 | −0.18 | .10665 | 0.45 | .0015 | ||
GABRB3 | 17.95 | −0.16 | .1294 | 0.63 | .00075 | ||
GABRD | 20.44 | −1.09 | .00005 | 0.23 | .04135 | ||
GABRE | 6.49 | −0.15 | .1965 | 0.71 | .00005 | ||
GABRG2 | 43.47 | −0.04 | .73085 | 0.40 | .00075 | ||
GABRG3 | 3.79 | −0.88 | .0003 | 0.61 | .00405 | ||
GABRQ | 5.67 | −0.21 | .07385 | 0.70 | .00005 |
Abbreviations: GABR, GABA receptor; FPKM, fragments per kilobase million (digital value of expression level).
BPA exposure and GABAergic regulation of GnRH release
Based on the RNA sequencing results, we studied whether GABAergic regulation of pulsatile GnRH secretion was affected by BPA. Exposure to the low BPA dose from PND1 to PND15 resulted in a significant increase of GnRH IPI on PND20 (51.7 ± 0.9 vs 43.5 ± 0.0 min), whereas the high dose caused a significant reduction of GnRH IPI (39.4 ± 0.5 min), confirming the data of earlier experiments. The increase in GnRH IPI that occurred after 25 ng/kg·d of BPA likely involved increased GABAergic tone, because it was no longer observed (Figure 4) when hypothalamic explants were incubated in the presence of bicuculline, a GABAA receptor antagonist (45.8 ± 0.9 vs 51.65 ± 0.9 min, bicuculline vs control). Moreover, muscimol did not affect the increase in GnRH IPI seen after exposure to 25 ng/kg·d of BPA. The reduction in GnRH IPI that occurred after 5 mg/kg·d of BPA (43.8 ± 1.3 vs 40 ± 1.25 min, muscimol vs control) was no longer observed when hypothalamic explants were incubated in the presence of muscimol, a GABAA receptor agonist. The GABAA receptor antagonist did not affect the reduction in GnRH IPI seen after exposure to 5 mg/kg·d of BPA (Figure 4). Taken together, these data confirm that postnatal exposure to a very low dose of BPA accounts for delayed maturation of GnRH secretion through increased GABAergic tone whereas a high dose of BPA accounts for early maturation through reduced GABAergic tone.
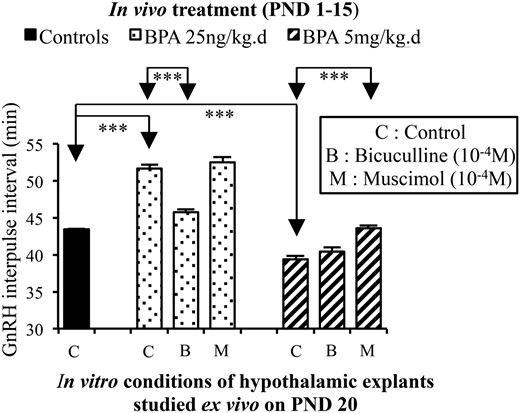
Effects of in vivo exposure to BPA on GABAergic regulation of GnRH secretion in vitro. GnRH IPI was calculated using secretion from hypothalamic explants obtained on PND20 from female rats exposed in vivo to vehicle (corn oil) or 25 ng/kg·d or 5 mg/kg·d of BPA from PND1 to PND15. The in vitro conditions involve control or presence of a GABAA receptor antagonist (bicuculline) or a GABAA receptor agonist (muscimol) both used at 10−4M concentration shown previously to be effective in similar conditions. A reduction in GnRH IPI by bicuculline and absent effects of muscimol indicated a high tone of GABAergic inhibition of GnRH release. Absence of bicuculline effects and increase in GnRH IPI by muscimol indicated a low tone of GABAergic inhibition of GnRH release. ***, P < .001. Data are mean ± SEM.
Discussion
We provide here the first evidence that BPA can have opposing dose-dependent effects on the neuroendocrine control of puberty in the female rat. Postnatal exposure to a high dose of BPA led to accelerated maturation of GnRH secretion and a trend toward early onset of puberty. Conversely, neonatal exposure to a very low dose of BPA led to a delay in developmental acceleration of GnRH secretion and a trend toward delayed VO. Based on these opposing effects on neuroendocrine maturation, we hypothesized that some hypothalamic genes could be differentially affected by a low vs high dose of BPA. An RNA sequencing analysis showed opposing variations in mRNA expression of genes related to GABA neurotransmission with confirmation using qPCR. Using GABA agonist and antagonist, a decrease or an increase of GABA inhibitory tone on pulsatile GnRH secretion was shown after exposure to a high or low dose of BPA, respectively.
As detailed in the Supplemental Table 5, a number of studies have reported that GABA neurotransmission could be affected in different brain areas after exposure to BPA during early life (34–41). GABAergic tone appeared to be increased after BPA exposure in some studies (34, 37) or reduced in others (36, 38, 39). In a recent study close to our conditions using whole brain extracts from animals exposed to different doses of BPA from gestational day 8 to PND16, Cabaton et al (36), investigated brain composition by nuclear magnetic resonance spectroscopy on PND21. In this non targeted metabolomics study, these authors reported that 25 μg/kg·d of BPA could reduce GABA concentrations in the brain. Interestingly enough they also used a very low BPA dose of 25 ng/kg·d, and it appears that opposing effects were obtained with the low dose ie, increase in GABA concentrations (N. Cabaton and D. Zalko, personal communication). These opposing dose effects are consistent with the present study. Also, such an observation in the whole central nervous system could suggest that the effects reported here about a specific neuroendocrine function could be part of a much larger effect of BPA in the brain. Opposing dose effects of BPA are supported by in vitro studies of GABA-induced currents in hippocampal neurons that are inhibited or potentiated by high or low BPA concentrations, respectively (41). It has been shown that GnRH secretion can be regulated by GABA in 2 manners (42). Synaptic receptors generate fast (phasic) postsynaptic currents and extrasynaptic receptors generate a persistent (tonic) current controlling neuronal excitability. The latter GABAA receptors accounting for tonic current involve the δ-subunit. Among the genes encoding the GABAA receptor subunits, mRNA expression of δ-subunit is the only one repressed after exposure to 5 mg/kg·d of BPA and one among 2 subunit genes showing increased mRNA expression after 25 ng/kg·d of BPA (Table 2). This is consistent with dose-dependent dual effects of BPA on tonic GABA neurotransmission. Only a few studies addressed the neuroendocrine events that can precede or follow the changes in GABAergic tone (Supplemental Table 5). Either 2 separate mechanisms involving different factors or opposing variations of a common factor could explain the opposing effects of BPA on GABA neurotransmission. An insight into a possible upstream mechanism was recently provided by a study reporting that delayed neonatal maturation of a chloride cotransporter after in utero exposure to BPA contributes to activate GABA neurotransmission through reduction of intraneuronal chloride concentrations (40). The gene encoding this cotransporter (Kcc2) was not affected in our RNA sequencing study performed on PND20. Studies performed in younger animals with emphasis on epigenetic mechanisms are warranted because such mechanisms were found to account for BPA alteration of Kcc2 expression (40) and DNA methyltransferase 1 mRNA expression was altered after BPA exposure in another study (39). In the present study, DNMT1 mRNA expression in the female hypothalamus was found to increase significantly at PND20 after exposure to 5 mg/kg·d of BPA (data not shown). Such effects consistent with involvement of epigenetic mechanisms could suggest that BPA has organizational together with activational effects. The issue is complex in the reproductive axis, because some effects are likely occurring at other levels including the gonads (43, 44).
Changes in mRNA expression of the components of GABAergic neurotransmission (GAD, GAT, and GABA receptor subunits) can be viewed either as part of the altered GABAergic tone or as a downstream reaction to the altered GABAergic tone. In the first case, increased GAD and reduced GAT expression will be associated with increased GABAergic tone (and conversely) such as found for GAD mRNA in the basolateral amygdala (39). In the present study, the inverse observation was made because reduced GAD and increased GAT expression were associated with increased GABAergic tone (and conversely). Such findings can be consistent with effects downstream from the increased GABA tone because it was shown in neuronal culture that GABA could down-regulate GAD expression and activity (45) and up-regulate GAT activity (46). Confirmation of such an explanation would require a time-lapse study of GAD and GAT expression during and after exposure to different doses of BPA. A prerequisite to the interpretation of changes in GAD and GAT mRNA expression was to clarify whether the GABAergic control of GnRH secretion was increased or reduced. An answer was obtained indirectly using the GABAA receptor agonist muscimol and antagonist bicuculline. Interestingly, Zhou et al (38) also found that the GABAAR agonist was effective in conditions of BPA-induced reduction of GABAergic tone using another paradigm, whereas the antagonist was effective in control conditions. A body of literature shows that GABA is a major contributor to the prepubertal inhibition of GnRH secretion in several species (23). However, there is evidence of stimulatory GABA in adult rats and mice (47, 48) and in the fetal hypothalamus (49). Because GABA has dual effects on GnRH neurosecretion, one could hypothesize that BPA resulted in increased or reduced GnRH secretion through antagonism of inhibitory or stimulating GABA inputs. In such event however, the effects of muscimol and bicuculline would have been opposed. In addition, dual GABA effects could be determined by the developmental stage and the neuronal apparatus (GnRH neurons vs impinging regulatory neurons). Here, opposing effects were obtained in similar conditions of age and hypothalamic structure.
Based on RNA sequencing data, G protein-coupled receptors represent another mechanistic component that bioinformatics analysis identified as a candidate system possibly involved. This was particularly interesting given the critical stimulating role of G protein-coupled receptor 54 and its ligand kisspeptin in the neuroendocrine control of puberty and reproduction (50, 51). However, neither Kiss1 nor GPR54 mRNA expression appeared to be affected in the present RNA sequencing analysis. We reported recently that DES exposure (1 and 10 μg/kg·d) reduced KISS1 expression in the hypothalamus (18). In the present study, however, exposure to BPA did not affect KISS1 mRNA level in the mediobasal hypothalamus (data not shown). After neonatal exposure of female rats to 0.1 or 0.5 mg/kg·d of BPA for 5 days, Navarro et al (9) reported reduced KISS1 mRNA expression on PND30, and Patisaul et al (52) found reduced kisspeptin immunoreactivity in the arcuate nucleus after neonatal exposure to 50 mg/kg·d of BPA for 4 days. Because kisspeptin is a gatekeeper of GnRH neuron stimulation, these findings are in contrast to the early VO that was reported after neonatal or early postnatal exposure to BPA doses ranging 0.05–100 mg/kg·d (4, 7, 8). These discordant findings could suggest that BPA effects on kisspeptin are not causal in the mechanism of early sexual maturation and might be reactive to that process, such as discussed about GAT and GAD mRNA expression.
Several studies have reported an effect of BPA on pubertal onset in female rodents. Taken as a whole, those studies show that BPA effects depend markedly on the age window and dose of exposure, with possible nonlinear dose-response relationship (2, 53). To our knowledge, our study is the first one demonstrating the impact of such a low and environmentally relevant dose of BPA on the neuroendocrine mechanism of sexual maturation. Twenty-five ng/kg·d of BPA affect the frequency of GnRH secretion and mRNAs expression of several hypothalamic genes. The effect of the very low dose of BPA on frequency of GnRH secretory pulses is robust and reproducible in our model. Moreover, it is consistent with the trend toward delayed VO that is seen subsequently. The amplitude of GnRH secretory pulses is not affected (data not shown). Using the ex vivo paradigm of hypothalamic explant, changes in amplitude of GnRH release were only apparent after in vitro stimulation with secretagogues such as glutamate or glutamate receptor agonists (21, 54). Because we aimed at changes in endogenously driven pulsatile secretion of GnRH in the present study, GnRH secretagogues have not been used.
The neuroendocrine system appears to be exquisitely sensitive to BPA endocrine disruption that may not be manifested phenotypically. When the female rats are postnatally exposed to 25 ng/kg·d for 5 or 15 days, age at puberty is not significantly different from controls but the frequency of GnRH secretion is significantly decreased. Presumably, the effects on maturation of GnRH secretion evidenced at PND20 and PND25 are not lasting enough or sufficient to affect significantly pubertal timing about 10 days later. We made a similar observation in male rats exposed for 5 days after birth (2). Thus, absence of phenotypic changes in some of the previous studies does not necessarily mean absence of effect. Such high neuroendocrine sensitivity to BPA, functionally evidenced through changes in pulsatile GnRH secretion, is also consistent with a significant change in hypothalamic mRNA expression of 34 genes after exposure to that very low dose of BPA.
The study of mRNAs expression showed some limitations. Our RNA sequencing analysis was limited to 3 samples for each group and confirmed on 8 samples only for some of the genes involved in GABA synthesis, reuptake, and signaling. However, the incubation of control or BPA-exposed hypothalamic explants with a GABA receptor agonist or antagonist robustly confirmed the effects of BPA on GABAergic neurotransmission. A short exposure of 5 or 15 days differs from the constant human exposure throughout life. However, such short exposures allow the delineation of a window of sensitivity to BPA. Also, the effects of BPA on puberty are unlikely to result from direct effects on target peripheral tissues such as the female genital tract because the exposure ended several weeks before puberty and BPA is a nonpersisting chemical. Accordingly, the data are likely to reflect alterations of functions organized during early postnatal life.
Human exposure to BPA varies between 0.01 and 1 μg/kg·d (10). Because we have studied a very low dose of 25 ng/kg·d, we chose sc injections in order to guarantee accuracy of the exposure. This route of administration was used as well in all the other studies with BPA exposure in early postnatal life (reviewed in Ref. 2). Although not relevant to the human route of exposure, sc injections have been shown to lead to similar serum concentration as oral administration (55). The question remains as to whether such a low level of exposure makes a difference from contamination through food, water, and cages. We have attempted to control for this through housing conditions and the control animals exposed to corn oil were raised in similar conditions.
Postnatal life (5–15 first days after birth) has been shown to be particularly sensitive to the effects of BPA on the neuroendocrine mechanism of puberty (2). Further studies could underscore the significance of early postnatal life as a critical window by comparing the effects of a similar exposure to BPA in adulthood. Questions remain as to whether a longer and sustained exposure involving the whole prenatal life could result in effects similar to those reported here. Moreover, further studies should aim at delineation of the genetic mechanisms that take place upstream and downstream to GABA neurotransmission and account for divergent effects on pubertal timing.
In conclusion, our study shows that alteration of pubertal timing by BPA in the rat is not necessarily towards precocity. Instead, delayed maturation of GnRH secretion can be caused by environmentally relevant exposure with the mechanistic involvement of GABA neurotransmission. Taking together our data and the literature, it appears that the effects of BPA are not limited to the neuroendocrine control of the reproductive axis. BPA seems to affect GABA neurotransmission in multiple brain areas. Although rodent data do not necessarily apply as such to human health, the involvement of GABA shown in the present study points to the likelihood of detrimental effects of early life exposure to BPA in humans. GABA plays indeed a critical role in brain development and function and disorders of human behaviors including autism (56) that are possibly more frequent after early exposure to BPA (57–59). The Unites States Environmental Protection Agency has considered that a daily exposure lower than 50 μg/kg·d was “safe” (60). European Food Safety Authority very recently proposed to set the daily tolerable intake at 4 μg/kg·d (61). This study reinforces the idea that defining a threshold for BPA effects is misleading not only because doses much below the so-called safe limit can interfere with the hormonal system but also because a low and a high dose can have opposing effects.
Acknowledgments
We thank Professor Delvenne for the assistance with Papanicolaou staining and the Dr V. D. Ramirez (Urbana, IL) for providing the CR11-B81 anti-GnRH antiserum.
This work was supported by Fonds National de la Recherche Scientifique (Belgium), the Belgian Society for Pediatric Endocrinology and Diabetology, and the Fonds Léon Frédéricq.
Disclosure Summary: The authors have nothing to disclose.
For Counterpoint see page 1736
Abbreviations
- BPA
bisphenol A
- Ct
cycle threshold
- DES
diethylstilbestrol
- EDC
endocrine-disrupting chemical
- GABA
gamma aminobutyric acid
- GAD
glutamic acid decarboxylase
- GAT
GABA transporter
- IPA
Ingenuity Pathway Analysis
- IPI
interpulse interval
- PND
postnatal day
- qPCR
quantitative PCR
- VO
vaginal opening.
References
Author notes
J.-P.B. and A.-S.P. contributed equally to this work.