-
PDF
- Split View
-
Views
-
Cite
Cite
Ashlyn Swift-Gallant, Lindsay A. Coome, Firyal Ramzan, D. Ashley Monks, Nonneural Androgen Receptors Affect Sexual Differentiation of Brain and Behavior, Endocrinology, Volume 157, Issue 2, 1 February 2016, Pages 788–798, https://doi.org/10.1210/en.2015-1355
- Share Icon Share
Abstract
Testosterone, acting via estrogenic and androgenic pathways, is the major endocrine mechanism promoting sexual differentiation of the mammalian nervous system and behavior, but we have an incomplete knowledge of which cells and tissues mediate these effects. To distinguish between neural and nonneural actions of androgens in sexual differentiation of brain and behavior, we generated a loxP-based transgenic mouse, which overexpresses androgen receptors (ARs) when activated by Cre. We used this transgene to overexpress AR globally in all tissues using a cytomegalovirus (CMV)-Cre driver (CMV-AR), and we used a Nestin-Cre driver to overexpress AR only in neural tissue (Nes-AR). We then examined whether neural or global AR overexpression can affect socio-sexual behaviors using a resident-intruder paradigm. We found that both neural and global AR overexpression resulted in decreased aggressive behaviors and increased thrusting during mounting of intruders, consistent with a neural site of action. Global, but not neural, AR overexpression in males led to an increase in same-sex anogenital investigation. Together, these results suggest novel roles for nonneural AR in sexual differentiation of mice, and indicate that excess AR can lead to a paradoxical reduction of male-typical behavior.
In mammals, sex-typical testosterone signaling throughout the lifespan sexually differentiates the nervous system and behavior. Both androgenic and estrogenic metabolites of testosterone, acting through the androgen receptor (AR) and estrogen receptors, respectively, work perinatally to organize male-typical behaviors in mice and rats. AR is necessary for the display of male-typical sexual and aggressive behaviors (1–4), but it remains unclear to what extent androgens act on AR in neural and nonneural tissues to mediate male-typical behavior (1, 5).
Models of complete AR loss of function, such as the testicular feminization mutation (Tfm) (1) and the complete AR knockout (ARKO) model (2), indicate that AR is necessary for male-typical development of the nervous system and behavior. These global loss of function mutants have an intersex or feminine phenotype for many sexually differentiated traits, including socio-sexual behaviors and their neural substrates. Similarly, when AR is selectively disabled in neural tissue of male mice (Nestin-ARKO), sexual and aggressive behaviors are diminished (3, 4). However, neural-specific ARKO mice exhibit fewer deficits compared with the complete ARKO or Tfm mice. For instance, Juntti et al (4) and Raskin et al (3) did not report any deficits in their analysis of neural-ARKO mice on the olfactory preference test, whereas Tfm male mice display a sex-reversed preference for chemosensory stimuli (6). These differences in behavioral phenotype between global and neural-specific loss of AR function could merely indicate that complete disabling of AR was not achieved in the neural tissue of the neural-ARKO model, or alternatively, could suggest that there are distinct roles for neural and nonneural AR.
Although loss of function mutations have been invaluable for determining the necessity of AR for many male-typical socio-sexual behaviors, the extent of AR function in sexual differentiation can also be studied using variation in sensitivity to androgens. Overexpression of AR can inform us about behavioral phenotypes that can emerge at the high range of gene expression, and also reveal gene functions that are not apparent with loss of function mutations (for review, see Ref. 7).
We therefore developed 2 models of androgen overexpression using the Cre/loxP system to address the contributions of high AR expression in neural and nonneural tissues. The first model overexpresses AR only in neural tissue (Nestin-AR [Nes-AR]) and the second overexpresses AR globally in both neural and nonneural tissue (cytomegalovirus [CMV]-AR). We compared the socio-sexual behaviors of these 2 models using a modified resident-intruder paradigm in which behavioral responses to alternating male and female intruders were measured, and we additionally measured neural activation in response to a male intruder. Our results suggest roles for nonneural AR in the sexual differentiation of socio-sexual behaviors and its neural substrates and indicate that excess AR can lead to a paradoxical reduction of male-typical behaviors.
Materials and Methods
CMV-stop-AR transgene
A floxed stop sequence was obtained from pBS-Neostop (kind gift for Scott Zeitlin, University of Virginia) (12). A NheI site was removed from within this sequence via blunting, before the addition of flanking NheI sites, which were introduced at existing XbaI and KpnI sites using linkers. A NheI fragment containing the loxP Neostop sequence was then ligated between promoter and coding sequence of the p5HBhAR26QcDNA transgene (kind gift of Andrew Lieberman, University of Michigan) (13), yielding CMV-stop-AR (Figure 1). CMV-stop-AR26 transgene was injected into pronuclei of (C57BL/6 × C3H) F2 zygotes in the transgenic facility of the Van Andel Research Institute (Grand Rapids, MI). Zygotes were then implanted into pseudopregnant foster mothers. Resulting transgenic CMV-stop-AR mice were backcrossed onto a C57Bl/6 background more than 10 times before behavioral analysis.
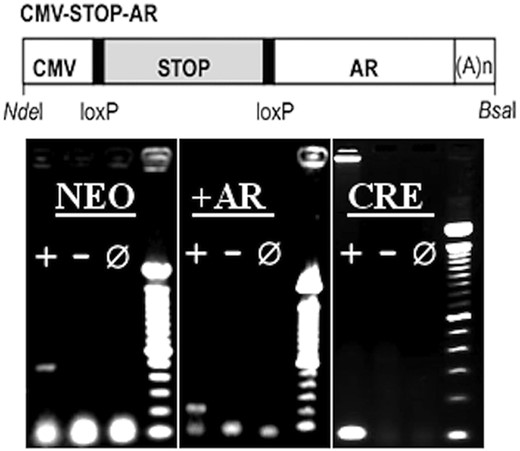
CMV-STOP-AR transgene. Diagram of CMV-STOP-AR transgene. CMV, mini-CMV promoter; STOP, floxed NEO-stop cassette; AR, human AR clone. loxP sites and restriction sites used to isolate transgene from vector backbone are indicated. A(n), polyadenylation sequence. PCR genotyping results: Transgenic positive (+),WT negative (−) and no DNA reactions (0) for all 3 genotyping protocols. The neo protocol indicated the presence of the neo stop of the AR transgene. The cre protocol indicated the presence of Nestin-Cre and CMV-Cre transgenes. +AR indicated the presence of the activated transgene. 100-bp ladder.
Animals
All mice (C57Bl/6 background) were between 2 and 4 months of age at the time of behavioral testing and were 3–4 months of age upon collection of tissue. Experimental animals were singly housed at least 2 weeks before resident-intruder testing, whereas stimulus animals were group housed. All animals were kept on a 12-hour light, 12-hour dark cycle at 21°C–23°C and had ad libitum access to Harlan chow (Harlan Laboratories, Inc). All procedures adhered to Canadian Council on Animal Care Regulations and were approved by the Local Animal Care Committee at the University of Toronto, Mississauga.
Nestin-Cre and CMV-Cre C57Bl/6 mice were purchased from The Jackson Laboratory (stock 003771; 006054) and were subsequently bred with animals in colony with the CMV-STOP-AR transgene. Subjects included in the behavioral analyses were: 14 wild-type (WT) males, 11 WT females, 12 male mice with CMV-STOP-AR and CMV-Cre (CMV-AR), 12 female CMV-AR mice, 12 male mice with CMV-STOP-AR and Nestin-Cre (Nes-AR), and 10 female Nes-AR mice. For immunohistochemistry (IHC), a subset of 6 animals per group was included in FOS analyses. An additional 6 WT, 6 Nes-AR, and 6 CMV-AR males that were not used in behavioral analyses were included in the AR analyses of muscle, and 6 WT, 5 Nes-AR, and 5 CMV-AR males were included in the analyses of AR in the brain. An additional 36 females were used to examine androgen induced genital masculinization (n = 6 per group; see below for further detail).
Genotyping
We identified the Cre-based transgenes with forward primer, GCG GTC TGG CAG TAA AAA CTA TC, and reverse primer, GTG AAA CAG CAT TGC TGT CAC TT. To identify the presence of the CMV-STOP-AR transgene we amplified the neo stop sequence using forward primer AGG ATC TCC TGT CAT CTC ACC TTG CTC CTG, and reverse primer AAG AAC TCG TCA AGA AGG CGA TAG AAG GCG. Due to the ubiquitous deletion of the Neo stop sequence in mice expressing both CMV-Cre and CMV-STOP-AR, it was also necessary to amplify a unique portion of the CMV-STOP-AR transgene. To do so, we used forward primer ACC GAG GAG CTT TCC AGA AT, and reverse primer CTC ATC CAG GAC CAG GTA GC. Refer to Figure 1 for an example of genotyping results.
Testosterone serum concentrations
We measured testosterone concentrations from serum collected before perfusion, using an ELISA from Enzo Life Sciences (catalog number ADI-901–065). We did so according to the manufacturer's protocol with a sample dilution factor of 5. The assay sensitivity is 5.67 pg/mL, and intraassay coefficient of variation is less than 10%. Cross-reactivity is 14.6% for 19-hydrooxytestosteron, 7.2% for androstendione but less than 1% with any other hormone or metabolite. The average of duplicate readings was used to calculate ng/mL.
Female genital differentiation
As a measure of androgen hypersensitivity in CMV-AR mice, we hormonally manipulated a subset of females, and analyzed their genital development in adulthood. Whole litters were injected sc on postnatal day (PND)1 with either 0.05 mL of testosterone propionate in sesame oil (20 mg/mL), or 0.05 mL of vehicle alone. Between 7 and 14 weeks of age, females were implanted with 2 Silastic tubes, one packed with estradiol dissolved in sesame oil (1.98-mm inner diameter/3.17-mm outer diameter; 50 μg in 0.025 mL) and the other with crystaline dihydrotestosterone (10 mm of DHT; Silastic tube 1.02-mm inner diameter/2.16-mm outer diameter). These implant specifications have been reported to result in male-typical physiological levels of estradiol and dihydrotestosterone in gonadectomized mice (14, 15). Capsules were sealed at both ends (5 mm at each end) using Silastic silicone medical adhesive purchased from Dow Corning. Four to 6 weeks after capsule implants, genitals were photographed. An experimenter blind to genotype and treatment scored genitalia images on a scale of 1–3: 1) indicating typical female genitalia, 2) indicated a protruding clitoris with pale color, and 3) indicated a protruding clitoris with a dark color. This ranked data was analyzed using a Kruskal-Wallis, and Mann-Whitney U tests were used for post hoc analyses.
Resident-intruder paradigm
Male and female experimental mice underwent a total of 6 modified resident-intruder tests, 3 tests with unfamiliar male intruders and 3 tests with unfamiliar female intruders. Experimental mice were tested every second day, and were exposed to a single intruder for each test. The order of presentation in all cases alternated between male and female intruders, and each experimental animal always had a male intruder on the first test. Experimental mice were singly housed 1–2 weeks before the start of behavioral testing, and testing occurred once a day, every other day, until all 6 tests were completed. Bedding was changed once a week. Stimulus males were castrated and given a minimum of 14 days to recover from surgery before the start of behavior testing. Female stimulus mice were gonadally intact. Under red light, behavioral tests lasted 10 minutes in the home cage of experimental mice during the dark phase of the animals' light/dark cycle. We used Noldus Observer XT for scoring of behaviors, including: total duration and number of anogenital investigation, duration and number of face investigations, latency to first mount, number of mounts, number of thrusts, number of boxing bouts, number of tumbling bouts, and duration and number of chasing bouts. A mount was counted whether or not it was followed by intromissions and/or thrusting. In addition to number of mounts and thrusts, we also evaluated number of thrusts per mount; only animals that performed a mount were included in the analysis of thrusting. Tumbling was defined as an aggressive attack in which the experimental animal toppled over the stimulus mouse. We also measured biting, which included all bites and tugging at hair (aggressive grooming) for males (16). Female experimental mice were assessed for aggressive grooming rather than biting, because they did not exhibit other biting behavior. Biting, tail rattling and intromissions as well as dominance-related postures were measured, but data were not analyzed statistically due to their rarity.
Resident-intruder exposure and tissue collection
Ninety minutes before tissue collection, both male and female experimental animals were exposed to an unfamiliar male intruder for 10 minutes. Animals were then intracardially perfused with PBS followed by 4% phosphate-buffered paraformaldyde. Carcasses, seminal vesicles, testes and fat pads were weighed. Brains were extracted and postfixed in the same fixative for 2 hours. Brains were then transferred to 20% sucrose and stored at 4°C until they were sectioned on a sliding microtome fitted with a Physitemp freezing stage. Brains were sectioned at 30 μm at −18°C to −35°C into 4 series. Sections were stored in cryoprotectant at −20°C until histological processing (17). In addition to brains, we collected extensor digitorum longus skeletal muscle to evaluate tissue specificity of AR overexpression, from Nes-AR (n = 6), CMV-AR (n = 6), and WT males (n = 6) were collected as previously described (18). We measured AR-immunoreactivity (AR-ir) in brain and skeletal muscle of male mice. We chose to look only at male tissues in this case because AR-ir can in some cases be ligand-dependent, and the goal of this experiment was simply to validate tissue specificity of the transgene.
Immunohistochemistry
To validate our overexpression models, we measured AR-ir in brain and skeletal muscle. AR IHC closely followed Swift-Gallant and Monks (19). Fresh tissue (skeletal muscle) and fixed tissue (brain) followed a similar AR staining protocol, except fresh tissue was first fixed in 4% phosphate buffered formaldehyde before blocking. One of the 4 series of brain and muscle sections was processed for AR-ir from 6 males from the next genotypes: WT, Nes-AR, and CMV-AR. This tissue was collected from unmanipulated mice that did not undergo the resident-intruder paradigm. For AR-ir counts in the brain, we used the same procedure (same brain regions and plate numbers) as FOS (see Table 1 for further detail).
Peptide/Protein Target . | Antigen Sequence (if Known) . | Name of Antibody . | Manufacturer, Catalog Number, and/or Name of Individual Providing the Antibody . | Species Raised in; Monoclonal or Polyclonal . | Dilution Used . |
---|---|---|---|---|---|
AR | Anti-AR | Abcam, Ab133273 (previously epitomics 3165-1) | Rabbit; monoclonal | 0.111111111 | |
FOS | c-fos antibody | Santa Cruz Biotechnology, Inc, SC-52 | Rabbit; polyclonal | 0.388888889 |
Peptide/Protein Target . | Antigen Sequence (if Known) . | Name of Antibody . | Manufacturer, Catalog Number, and/or Name of Individual Providing the Antibody . | Species Raised in; Monoclonal or Polyclonal . | Dilution Used . |
---|---|---|---|---|---|
AR | Anti-AR | Abcam, Ab133273 (previously epitomics 3165-1) | Rabbit; monoclonal | 0.111111111 | |
FOS | c-fos antibody | Santa Cruz Biotechnology, Inc, SC-52 | Rabbit; polyclonal | 0.388888889 |
Peptide/Protein Target . | Antigen Sequence (if Known) . | Name of Antibody . | Manufacturer, Catalog Number, and/or Name of Individual Providing the Antibody . | Species Raised in; Monoclonal or Polyclonal . | Dilution Used . |
---|---|---|---|---|---|
AR | Anti-AR | Abcam, Ab133273 (previously epitomics 3165-1) | Rabbit; monoclonal | 0.111111111 | |
FOS | c-fos antibody | Santa Cruz Biotechnology, Inc, SC-52 | Rabbit; polyclonal | 0.388888889 |
Peptide/Protein Target . | Antigen Sequence (if Known) . | Name of Antibody . | Manufacturer, Catalog Number, and/or Name of Individual Providing the Antibody . | Species Raised in; Monoclonal or Polyclonal . | Dilution Used . |
---|---|---|---|---|---|
AR | Anti-AR | Abcam, Ab133273 (previously epitomics 3165-1) | Rabbit; monoclonal | 0.111111111 | |
FOS | c-fos antibody | Santa Cruz Biotechnology, Inc, SC-52 | Rabbit; polyclonal | 0.388888889 |
Immunohistochemical detection of FOS, an immediate early gene, was used as a marker of neuronal activation in response to a male intruder. One of the 4 series of brain sections was processed for FOS-ir, as previously described (20). Bilateral images were captured at ×400 magnification for 5 brain regions identified using the next plates from the mouse brain atlas (21): bed nucleus of the stria terminalis (BNST) and medial preoptic area (MPOA) (plate 30 and 33), the core and of the shell of the nucleus accumbens (NAcc) (plate 23), plate 21 and 23 of the lateral septum (LS), and the medial amygdala (MePD) (plate 40 and 45). A total of 4 images (bilateral images of 2 plates) were captured for each brain region. These brain regions were selected based on evidence of their involvement in socio-sexual behaviors, and the presence of sex differences (22–26). Immediate early gene activation (FOS) in response to pheromonal cues differs between the sexes in the BNST, MPOA, and MePD. The LS has been linked to both female sexual behavior and maternal aggression. Similarly, the LS, as well as the BNST and MePD, has been shown to activate in response to intermale aggression. Lastly, activation of the NAcc has been linked to the rewarding components of socio-sexual behaviors, and increases in FOS have been reported in the NAcc in response to opposite-sex odors. As described below, we used automated cell counting, and the total number was calculated from the 4 images.
Statistical analyses
For each behavioral measure, a repeated-measures ANOVA compared resident sex, intruder sex, and genotype across all 3 trials. Unless otherwise stated, we conducted post hoc analyses only for significant and marginally significant interactions; Tukey's honest significant difference were conducted for between subject factors and pairwise t-tests were used for within subjects factors with Bonferroni correction. Significance was determined by the critical α-level of less than 0.05, and marginal effects at less than 0.10.
ImageJ 1.47V software was used to automate cell counting for both AR and FOS. To calibrate autocell counting parameters, we first manually scored 3 sample images. Based on these manual counts, we adjusted the automated cell counting parameters to obtain autocounts within 5 ± cells of the manual counts for each sample image. Default settings were used for autocounts with the exception of brightness for the color threshold parameters, which was reduced to 125. The analyze particles function was used to count the masks placed by the color threshold settings, and within the analyze particles parameters, pixels smaller than 10 were excluded. Experimenters were blind to subject sex and genotype while capturing and analyzing the images.
Results
Characterization
AR overexpression in neural and nonneural tissue of our transgenic mice was validated using AR IHC. Obvious increases in AR-ir were apparent throughout the brain of CMV-AR and Nes-AR males compared with WT males, including all regions analyzed for FOS-ir in the present study (see Figure 2A). Results revealed a significant interaction of genotype and brain region, as well as an effect of genotype, F2,16 = 3.827, P = .049. Post hoc tests indicated that overall both CMV-AR, P = .035, and Nestin-AR, P = .034 had approximately 4.5× more AR-ir cells in the brain compared with WT males (see Figure 3). However, analyses evaluating each of the 5 brain regions quantified separately suggest that there is regional variability. A repeated measures ANOVA indicated a brain region by genotype effect, F8,52 = 2.705, P = .014. Post hoc analyses indicate that CMV-AR and Nes-AR males have significantly more AR compared with WT males in the NAcc, P = .017 and P = .027, respectively; LS, P = .033 and P = .015, respectively. Significantly more AR was found in CMV-AR compared with WT males in the MPOA, P = .023, and marginally more in the BNST, P = .088. Although Nes-AR males had significantly more AR in the BNST, P = .021, and marginally more in the MPOA, P = .067. No differences were found between CMV-AR and Nes-AR compared with WT males in the MePD, P = .235 and P = .101, respectively. Specificity of nonneural AR overexpression was confirmed in skeletal muscle, in which CMV-AR males had approximately 3× more AR-ir nuclei, F2,11 = 10.014, P = .003, compared with both WT, P = .004 and Nes-AR males, P = .002 (see Figures 2B and 3).
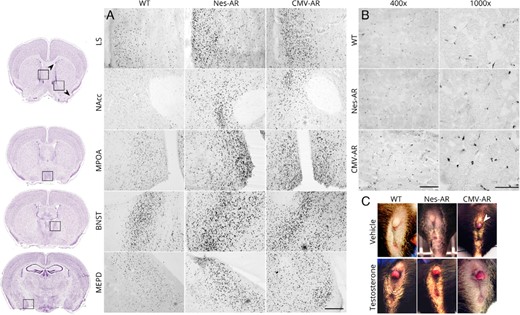
Characterization of AR overexpression models. A, High AR-ir in Nes-AR and CMV-AR male mice compared with WT males in various brain regions (×200 magnification). Scale bar, 200 μm. B, High AR-ir was found in CMV-AR, but not Nes-AR, skeletal muscle compared with WT littermates. Scale bar at ×400 magnification, 100 μm. Scale bar at ×1000 magnification, 50 μm. C, Adult female clitorises after treatment of a vehicle or of testosterone propionate on PND1. Arrow indicates a CMV-AR female with enlarged clitoris after treatment with a vehicle, indicating that the global AR overexpression is functional and sufficient to affect genital development. Image credit, thionin-stained images obtained from the Allen Institute for Brain Science website at www.alleninstitute.org.
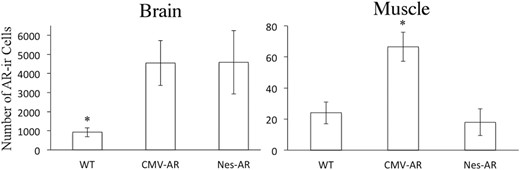
Number of AR-ir cells in brain and skeletal muscle. Both Nes-AR and CMV-AR transgenic males have approximately 4.5× more AR-ir cells in the brain compared with WT male mice. Furthermore, CMV-AR males have more approximately 3× more AR-ir nuclei in skeletal muscle compared with both Nes-AR and WT male mice.
Functionality of the CMV-STOP-AR transgene and specificity of expression in CMV-AR and Nes-AR mice was validated by examining response of female genitalia to androgen treatment. CMV-AR females, regardless of PND1 treatment, were found to have hypertrophic clitorises, suggesting that CMV-AR mice have increased sensitivity to androgens in nonneural tissues during the critical period of sexual differentiation (see Figure 2C). Specifically, a Kruskal-Wallis revealed a significant effect of genotype, X2 (2) = 18.825, P = .001. Mann-Whitney U tests confirm that CMV-AR females were significantly different from both WT, U = 20, P = .001, and Nestin-AR females, U = 14, P = .024. Furthermore, no significant difference was found between WT and Nestin-AR females, U = 36, P = .273.
AR overexpression did not alter circulating testosterone concentrations in our transgenic models, F2,15 = 1.602, P = .234 (Table 2). It therefore seems unlikely that a deficit in circulating testosterone is responsible for effects of CMV-AR and Nes-AR on socio-sexual behaviors and neural response to male intruders. No significant effects of genotype were found for body weight, or fat pad weight, although there was a significant effect of testes weight, F2,39 = 4.801, P = .014, such that CMV-AR males had lower testes weight compared with WT, P = .011, but not Nes-AR males, P = .637. Nes-AR did not significantly differ in testes weight compared with WT males, P = .113. Similarly, a trend for genotype was found for seminal vesicles weight, F2,24 = 2.75, P = .078, such that CMV-AR males had lower seminal vesicle weight compared with WT males, P = .066, but not compared with Nes-AR males, P = .473 (see Table 3).
Genotype . | Testosterone, ng/mL . |
---|---|
WT | 5.45 (0.58) |
Nes-AR | 5.08 (0.98) |
CMV-AR | 8.88 (2.63) |
Genotype . | Testosterone, ng/mL . |
---|---|
WT | 5.45 (0.58) |
Nes-AR | 5.08 (0.98) |
CMV-AR | 8.88 (2.63) |
Serum testosterone was measured using an ELISA. No statistical differences were found between male CMV-AR, Nes-AR, and WT mice.
Genotype . | Testosterone, ng/mL . |
---|---|
WT | 5.45 (0.58) |
Nes-AR | 5.08 (0.98) |
CMV-AR | 8.88 (2.63) |
Genotype . | Testosterone, ng/mL . |
---|---|
WT | 5.45 (0.58) |
Nes-AR | 5.08 (0.98) |
CMV-AR | 8.88 (2.63) |
Serum testosterone was measured using an ELISA. No statistical differences were found between male CMV-AR, Nes-AR, and WT mice.
. | Body Weight (g) . | Testes Weight (g) . | Seminal Vesicles (g) . | Fat Pads (g) . |
---|---|---|---|---|
WT | ||||
Male | 29.6 (1.258) | 0.224 (0.007) | 0.351 (0.012) | 0.912 (0.088) |
Female | 23.857 (1.503) | NA | NA | NA |
Nes-AR | ||||
Male | 29.714 (1.50) | 0.2165 (0.006) | 0.329 (0.011) | 0.757 (0.086) |
Female | 25.20 (1.778) | NA | NA | NA |
CMV-AR | ||||
Male | 30.429 (1.50) | 0.199 (0.007) | 0.310 (0.015) | 0.864 (0.086) |
Female | 26.222 (1.326) | NA | NA | NA |
. | Body Weight (g) . | Testes Weight (g) . | Seminal Vesicles (g) . | Fat Pads (g) . |
---|---|---|---|---|
WT | ||||
Male | 29.6 (1.258) | 0.224 (0.007) | 0.351 (0.012) | 0.912 (0.088) |
Female | 23.857 (1.503) | NA | NA | NA |
Nes-AR | ||||
Male | 29.714 (1.50) | 0.2165 (0.006) | 0.329 (0.011) | 0.757 (0.086) |
Female | 25.20 (1.778) | NA | NA | NA |
CMV-AR | ||||
Male | 30.429 (1.50) | 0.199 (0.007) | 0.310 (0.015) | 0.864 (0.086) |
Female | 26.222 (1.326) | NA | NA | NA |
No statistical differences were found between mutant and WT mice on measures of body weight, or fat pad weight. A significant difference for testes weight indicated that CMV-AR males have lower testes weight compared with WT and Nes-AR males, although this effect disappears if corrected for body weight. A marginal effect of seminal vesicle weight suggests that CMV-AR males have smaller seminal vesicles than WT male mice.
. | Body Weight (g) . | Testes Weight (g) . | Seminal Vesicles (g) . | Fat Pads (g) . |
---|---|---|---|---|
WT | ||||
Male | 29.6 (1.258) | 0.224 (0.007) | 0.351 (0.012) | 0.912 (0.088) |
Female | 23.857 (1.503) | NA | NA | NA |
Nes-AR | ||||
Male | 29.714 (1.50) | 0.2165 (0.006) | 0.329 (0.011) | 0.757 (0.086) |
Female | 25.20 (1.778) | NA | NA | NA |
CMV-AR | ||||
Male | 30.429 (1.50) | 0.199 (0.007) | 0.310 (0.015) | 0.864 (0.086) |
Female | 26.222 (1.326) | NA | NA | NA |
. | Body Weight (g) . | Testes Weight (g) . | Seminal Vesicles (g) . | Fat Pads (g) . |
---|---|---|---|---|
WT | ||||
Male | 29.6 (1.258) | 0.224 (0.007) | 0.351 (0.012) | 0.912 (0.088) |
Female | 23.857 (1.503) | NA | NA | NA |
Nes-AR | ||||
Male | 29.714 (1.50) | 0.2165 (0.006) | 0.329 (0.011) | 0.757 (0.086) |
Female | 25.20 (1.778) | NA | NA | NA |
CMV-AR | ||||
Male | 30.429 (1.50) | 0.199 (0.007) | 0.310 (0.015) | 0.864 (0.086) |
Female | 26.222 (1.326) | NA | NA | NA |
No statistical differences were found between mutant and WT mice on measures of body weight, or fat pad weight. A significant difference for testes weight indicated that CMV-AR males have lower testes weight compared with WT and Nes-AR males, although this effect disappears if corrected for body weight. A marginal effect of seminal vesicle weight suggests that CMV-AR males have smaller seminal vesicles than WT male mice.
Behavioral measures
Social investigation
Investigation directed towards the face was not significantly affected by sex and genotype. In contrast, anogenital investigation was altered in CMV-AR, but not Nes-AR, mice. A significant interaction for trial by genotype and intruder sex was found for duration of anogenital investigation, F4,130 = 3.391, P = .011. Overall, CMV-AR mice investigated female intruders less than WT residents, t (52) = 2.229, P = .030. Interestingly, WT and Nes-AR males preferentially investigated the anogenital region of female intruders compared with male intruders, t (13) = 3.629, P = .009 and t (11) = 3.251, P = .024, whereas CMV-AR males investigated male and female intruders equally on trial one, t (11) = 1.271, P = .69 (see Figure 4 and Supplemental Tables 2 and 3), suggesting that overall CMV-AR mice are investigating more than WT mice, p= 0.091.
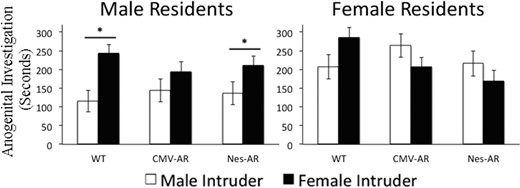
Anogenital investigation of male (white bars) and female (black bars) intruders on trial 1. Male residents (left) investigate female intruders longer than male intruders, with the exception of CMV-AR males; CMV-AR males investigate male and female intruders equally. WT, CMV-AR, and Nes-AR females do not preferentially investigate male or female intruders. *, P < .05; &, P < .09. Error bars, ±SE.
In contrast to anogenital investigation, which was affected by global AR overexpression, no genotype effects were found for investigation of the face region of male or female intruders (intruder sex by genotype by trial: F4,120 = 0.753, P = .558).
Copulation
Sexual receptivity of the stimulus females was assessed to ensure that there were no biases between groups. We calculated the lordosis quotient for all males that mounted a minimum of 10 times on trial 3. A Kruskal-Wallis evaluating lordosis quotient scores from the 3 genotype groups confirmed that there were no differences in stimulus female receptivity between groups, X2 (2) = 1.367, P = .505.
As expected, we observed a significant interaction for trial, intruder sex and resident sex, F2,130 = 16.324, P < .0001. Post hoc analyses indicated that male resident showed an increase in mounting of female (trial 1 vs 2, P = .002, trial 2 vs 3, P = .001 and trial 1 vs 3 P = .001), but not male intruders across trials (trial 1 vs 2, P = .579, trial 2 vs 3, P = .213 and trial 1 vs 3 P = .136), whereas female residents did not show an differences over trials for male intruders (trial 1 vs 2, P = .144, trial 2 vs 3, P = .696 and trial 1 vs 3 P = .140), although there is a trend for an increase between trial 2 and 3, P = .061, but not between trial 1 and 2 or between trial 1 and 3 for female intruders, P = .378 and P = .426, respectively (see Table 4). Genotype did not affect mounting.
Genotype . | Resident Sex . | Trial 1 . | Trial 2 . | Trial 3 . | |||
---|---|---|---|---|---|---|---|
Male Intruder . | Female Intruder . | Male Intruder . | Female Intruder . | Male Intruder . | Female Intruder . | ||
WT | Male | 1.71 (0.62) | 7.29 (1.56) | 2.71 (1.33) | 13.64 (2.65) | 1.50 (0.76) | 17.28 (1.85) |
Female | 1.09 (0.55) | 3.73 (0.79) | 1.73 (0.89) | 5.27 (1.41) | 2.09 (0.86) | 3.46 (0.94) | |
Nes-AR | Male | 1.17 (0.82) | 7.42 (1.76) | 2.00 (0.91) | 7.63 (2.20) | 3.75 (1.12) | 20.00 (3.08) |
Female | 1.3 (0.52) | 2.5 (1.27) | 0.50 (0.27) | 4.50 (1.42) | 0.90 (0.69) | 1.70 (0.92) | |
CMV-AR | Male | 1.17 (0.60) | 6.08 (1.87) | 1.58 (1.01) | 9.08 (2.62) | 3.17 (1.29) | 20.17 (3.32) |
Female | 1.67 (1.07) | 2.91 (1.21) | 2.00 (0.85) | 2.67 (0.77) | 1.00 (0.46) | 3.33 (1.62) |
Genotype . | Resident Sex . | Trial 1 . | Trial 2 . | Trial 3 . | |||
---|---|---|---|---|---|---|---|
Male Intruder . | Female Intruder . | Male Intruder . | Female Intruder . | Male Intruder . | Female Intruder . | ||
WT | Male | 1.71 (0.62) | 7.29 (1.56) | 2.71 (1.33) | 13.64 (2.65) | 1.50 (0.76) | 17.28 (1.85) |
Female | 1.09 (0.55) | 3.73 (0.79) | 1.73 (0.89) | 5.27 (1.41) | 2.09 (0.86) | 3.46 (0.94) | |
Nes-AR | Male | 1.17 (0.82) | 7.42 (1.76) | 2.00 (0.91) | 7.63 (2.20) | 3.75 (1.12) | 20.00 (3.08) |
Female | 1.3 (0.52) | 2.5 (1.27) | 0.50 (0.27) | 4.50 (1.42) | 0.90 (0.69) | 1.70 (0.92) | |
CMV-AR | Male | 1.17 (0.60) | 6.08 (1.87) | 1.58 (1.01) | 9.08 (2.62) | 3.17 (1.29) | 20.17 (3.32) |
Female | 1.67 (1.07) | 2.91 (1.21) | 2.00 (0.85) | 2.67 (0.77) | 1.00 (0.46) | 3.33 (1.62) |
Male mice mounted more frequently than female mice, and female intruders were mounted more than male intruders, as expected. No differences were found between mutant and WT mice.
Genotype . | Resident Sex . | Trial 1 . | Trial 2 . | Trial 3 . | |||
---|---|---|---|---|---|---|---|
Male Intruder . | Female Intruder . | Male Intruder . | Female Intruder . | Male Intruder . | Female Intruder . | ||
WT | Male | 1.71 (0.62) | 7.29 (1.56) | 2.71 (1.33) | 13.64 (2.65) | 1.50 (0.76) | 17.28 (1.85) |
Female | 1.09 (0.55) | 3.73 (0.79) | 1.73 (0.89) | 5.27 (1.41) | 2.09 (0.86) | 3.46 (0.94) | |
Nes-AR | Male | 1.17 (0.82) | 7.42 (1.76) | 2.00 (0.91) | 7.63 (2.20) | 3.75 (1.12) | 20.00 (3.08) |
Female | 1.3 (0.52) | 2.5 (1.27) | 0.50 (0.27) | 4.50 (1.42) | 0.90 (0.69) | 1.70 (0.92) | |
CMV-AR | Male | 1.17 (0.60) | 6.08 (1.87) | 1.58 (1.01) | 9.08 (2.62) | 3.17 (1.29) | 20.17 (3.32) |
Female | 1.67 (1.07) | 2.91 (1.21) | 2.00 (0.85) | 2.67 (0.77) | 1.00 (0.46) | 3.33 (1.62) |
Genotype . | Resident Sex . | Trial 1 . | Trial 2 . | Trial 3 . | |||
---|---|---|---|---|---|---|---|
Male Intruder . | Female Intruder . | Male Intruder . | Female Intruder . | Male Intruder . | Female Intruder . | ||
WT | Male | 1.71 (0.62) | 7.29 (1.56) | 2.71 (1.33) | 13.64 (2.65) | 1.50 (0.76) | 17.28 (1.85) |
Female | 1.09 (0.55) | 3.73 (0.79) | 1.73 (0.89) | 5.27 (1.41) | 2.09 (0.86) | 3.46 (0.94) | |
Nes-AR | Male | 1.17 (0.82) | 7.42 (1.76) | 2.00 (0.91) | 7.63 (2.20) | 3.75 (1.12) | 20.00 (3.08) |
Female | 1.3 (0.52) | 2.5 (1.27) | 0.50 (0.27) | 4.50 (1.42) | 0.90 (0.69) | 1.70 (0.92) | |
CMV-AR | Male | 1.17 (0.60) | 6.08 (1.87) | 1.58 (1.01) | 9.08 (2.62) | 3.17 (1.29) | 20.17 (3.32) |
Female | 1.67 (1.07) | 2.91 (1.21) | 2.00 (0.85) | 2.67 (0.77) | 1.00 (0.46) | 3.33 (1.62) |
Male mice mounted more frequently than female mice, and female intruders were mounted more than male intruders, as expected. No differences were found between mutant and WT mice.
We observed a marginal 3-way interaction between intruder sex, trial, and genotype, F4,130 = 2.25, P = .067, for thrusting (see Figure 5 and Table 5). Both WT and Nes-AR residents displayed a marginal increase in thrusting of a female intruder between trial 1 and 2, t (28) = 1.903, P = .067 and t (22) = 1.944, P = .065, but then plateaued, and showed no difference in thrusting between trial 2 and 3, t (28) = 0.806, P = .427 and t (22) = 0.149, P = .883. Conversely, CMV-AR residents displayed an increase in thrusting across trials. Although there were no differences found between trial 1 and 2, and trial 2 and 3, CMV-AR residents displayed an increase between trial 1 and 3, t (24) = 2.384, P = .025. Thrusting of male intruders did not differ across trials. Because females displayed little thrusting overall, we analyzed male residents separately to see if males were driving these effects. After sexual experience, on trial 3, CMV-AR male residents thrusted female intruders more than WT male residents, P = .077. To evaluate whether these results truly reflect experience effects, we tested whether trial 1 or trial 2 was a covariate of thrusting on trial 3. We found that trial 2, F1,71 = 12.963, P = .001, but not trial 1, F1,69 = 1.012, P = .318), was a significant covariate, suggesting that the animals that were thrusting on trial 2 were also thrusting on trial 3, consistent with an effect of experience. Conversely, Nes-AR males thrusted male intruders more than WT males on trial 3, t (28) = 2.089, P = .046, although they did not differ from WT males in thrusting of female intruders (for antibodies, please see Table 1).

Thrusting of male (white bars) and female (black bars) intruders, and number of thrusts per mount on trial 3. Overall male residents displayed more thrusting of female intruders compared with female residents. CMV-AR mice show an increase in number of thrusts per mount of female intruders, and differ significantly from WT and Nes-AR mice. Nes-AR males thrusted male intruders more compared with WT males. a, effect of genotype; error bars, ±SE.
Genotype . | Resident Sex . | Trial 1 . | Trial 2 . | Trial 3 . | |||
---|---|---|---|---|---|---|---|
Male Intruder . | Female Intruder . | Male Intruder . | Female Intruder . | Male Intruder . | Female Intruder . | ||
WT | Male | 1.00 (0.68) | 10.00 (2.95) | 2.14 (0.98) | 24.57 (7.92) | 0.14 (1.17) | 27.71 (9.05) |
Female | 1.55 (0.78) | 3.82 (3.33) | 0.55 (1.10) | 16.50 (8.93) | 3.73 (1.32) | 3.18 (10.21) | |
Nes-AR | Male | 0.25 (0.73) | 5.21 (3.19) | 1.08 (1.06) | 18.58 (8.56) | 3.33 (1.12) | 25.25 (8.76) |
Female | 1.90 (0.81) | 6.60 (3.49) | 0.40 (1.16) | 9.70 (9.37) | 0.40 (1.39) | 0.90 (10.70) | |
CMV-AR | Male | 0.67 (0.74) | 3.92 (3.12) | 1.58 (1.06) | 20.17 (8.56) | 1.83 (1.27) | 58.67 (9.77) |
Female | 1.00 (0.74) | 7.58 (3.19) | 2.75 (1.05) | 8.17 (8.15) | 0.67 (1.22) | 5.17 (5.91) |
Genotype . | Resident Sex . | Trial 1 . | Trial 2 . | Trial 3 . | |||
---|---|---|---|---|---|---|---|
Male Intruder . | Female Intruder . | Male Intruder . | Female Intruder . | Male Intruder . | Female Intruder . | ||
WT | Male | 1.00 (0.68) | 10.00 (2.95) | 2.14 (0.98) | 24.57 (7.92) | 0.14 (1.17) | 27.71 (9.05) |
Female | 1.55 (0.78) | 3.82 (3.33) | 0.55 (1.10) | 16.50 (8.93) | 3.73 (1.32) | 3.18 (10.21) | |
Nes-AR | Male | 0.25 (0.73) | 5.21 (3.19) | 1.08 (1.06) | 18.58 (8.56) | 3.33 (1.12) | 25.25 (8.76) |
Female | 1.90 (0.81) | 6.60 (3.49) | 0.40 (1.16) | 9.70 (9.37) | 0.40 (1.39) | 0.90 (10.70) | |
CMV-AR | Male | 0.67 (0.74) | 3.92 (3.12) | 1.58 (1.06) | 20.17 (8.56) | 1.83 (1.27) | 58.67 (9.77) |
Female | 1.00 (0.74) | 7.58 (3.19) | 2.75 (1.05) | 8.17 (8.15) | 0.67 (1.22) | 5.17 (5.91) |
Genotype . | Resident Sex . | Trial 1 . | Trial 2 . | Trial 3 . | |||
---|---|---|---|---|---|---|---|
Male Intruder . | Female Intruder . | Male Intruder . | Female Intruder . | Male Intruder . | Female Intruder . | ||
WT | Male | 1.00 (0.68) | 10.00 (2.95) | 2.14 (0.98) | 24.57 (7.92) | 0.14 (1.17) | 27.71 (9.05) |
Female | 1.55 (0.78) | 3.82 (3.33) | 0.55 (1.10) | 16.50 (8.93) | 3.73 (1.32) | 3.18 (10.21) | |
Nes-AR | Male | 0.25 (0.73) | 5.21 (3.19) | 1.08 (1.06) | 18.58 (8.56) | 3.33 (1.12) | 25.25 (8.76) |
Female | 1.90 (0.81) | 6.60 (3.49) | 0.40 (1.16) | 9.70 (9.37) | 0.40 (1.39) | 0.90 (10.70) | |
CMV-AR | Male | 0.67 (0.74) | 3.92 (3.12) | 1.58 (1.06) | 20.17 (8.56) | 1.83 (1.27) | 58.67 (9.77) |
Female | 1.00 (0.74) | 7.58 (3.19) | 2.75 (1.05) | 8.17 (8.15) | 0.67 (1.22) | 5.17 (5.91) |
Genotype . | Resident Sex . | Trial 1 . | Trial 2 . | Trial 3 . | |||
---|---|---|---|---|---|---|---|
Male Intruder . | Female Intruder . | Male Intruder . | Female Intruder . | Male Intruder . | Female Intruder . | ||
WT | Male | 1.00 (0.68) | 10.00 (2.95) | 2.14 (0.98) | 24.57 (7.92) | 0.14 (1.17) | 27.71 (9.05) |
Female | 1.55 (0.78) | 3.82 (3.33) | 0.55 (1.10) | 16.50 (8.93) | 3.73 (1.32) | 3.18 (10.21) | |
Nes-AR | Male | 0.25 (0.73) | 5.21 (3.19) | 1.08 (1.06) | 18.58 (8.56) | 3.33 (1.12) | 25.25 (8.76) |
Female | 1.90 (0.81) | 6.60 (3.49) | 0.40 (1.16) | 9.70 (9.37) | 0.40 (1.39) | 0.90 (10.70) | |
CMV-AR | Male | 0.67 (0.74) | 3.92 (3.12) | 1.58 (1.06) | 20.17 (8.56) | 1.83 (1.27) | 58.67 (9.77) |
Female | 1.00 (0.74) | 7.58 (3.19) | 2.75 (1.05) | 8.17 (8.15) | 0.67 (1.22) | 5.17 (5.91) |
Interestingly, we also found a significant effect of genotype on number of thrusts per mount, F2,61 = 3.533, P = .035, on trial 3, suggesting that CMV-AR males are thrusting more per mount compared with both WT, t (27) = 1.962, P = .06, and Nes-AR residents, t (23) = 1.895, P = .071.
Aggressive behaviors
As expected, intruder sex by resident sex interactions were found for all aggressive measures indicating that male residents were more aggressive toward male intruders compared with female intruders, whereas female residents showed little aggressive behaviors towards either male and female intruders (see Figure 6): boxing, F1,130 = 20.767, P < .001; tumbling, F1,130 = 18.485, P < .001; and number of chasing bouts, F1,130 = 13.397, P = .001 (see Supplemental Tables 7 and 8).
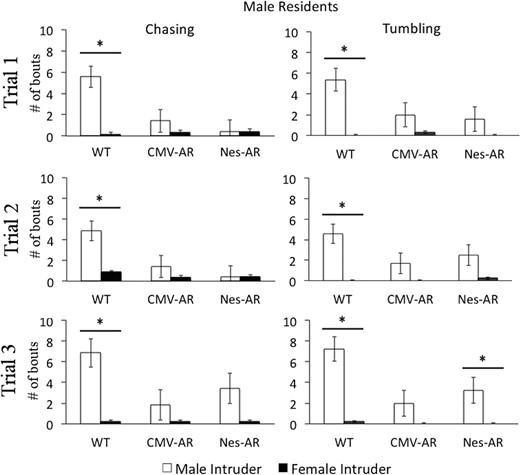
Aggressive behaviors displayed by male residents towards male (white bars) and female (black bars) intruders across trials 1–3. On all trials, WT male residents chase and tumble with male intruders more than female intruders. Both CMV-AR and Nes-AR male residents chase and tumble less with male intruders compared than WT male residents, and do not aggress more with a male intruder compared with female intruder, with the exception that Nes-AR males tumble more with male intruders on trial 3. *, P < .05; &, P < .1. Error bars, ±SE.
Both neural and global overexpression of AR decreased chasing behaviors (see Figure 6 and Supplemental Table 5). There was an interaction of intruder sex and genotype, F2,130 = 6.255, P = .003, and a main effect of genotype, F2,65 = 6.825, P = .002 for number of chasing bouts. Overall CMV-AR and Nes-AR residents chased less than WT residents, P = .001 and P = .009. CMV-AR residents did not differ from Nes-AR residents, P = .767. Interestingly, although WT residents showed an increase in chasing a male intruders compared with female intruders on all trials (trial 1: t (16) = 2.923, P = .03; trial 2: t (16) = 3.159, P = .024; trial 3: t (16) = 2.414, P = .084), CMV-AR, and Nes-AR males did not show a difference in chasing of male and female intruders on trial 1, t (12) = 1.429, P = .537, and t (12) = 1.468, P = .504, trial 2, t (12) = 1.817, P = .5291, and t (12) = 0, P = 1, nor on trial 3 t (12) = 1.00, P = 1, and t (12) = 2.044, P = .189.
Similarly, both neural and global overexpression of AR resulted in decreased tumbling bouts (see Figure 5). A significant interaction was found between intruder sex and genotype, F2,130 = 3.925, P = .025, as well as a significant effect of genotype, F2,65 = 3.36, P = .041 for number of tumbling bouts. CMV-AR residents displayed significantly less tumbling compared with WT residents, P = .021, and Nes-AR residents displayed marginally less tumbling compared with WT residents, P = .069. WT residents displayed tumbling significantly more with male intruders compared with female intruders across all trials (trial 1: t (16) = 3.59, P = .009; trial 2: t (16) = 3.572, P = .009; trial 3: t (16) = 3.268, P = .015). CMV-AR residents did not show a difference in tumbling of a male vs female intruder on any trial (trial 1: t (12) = 2.002, P = .204; trial 2: t (12) = 1.261, P = .699; trial 3: t (16) = 1.056, P = .942). In contrast to chasing bouts, Nes-AR residents did tumble with male intruders compared with female intruders on trial 3, t (12) = 3.108, P = .027, and marginally on trial 2, t (12) = 2.463, P = .09, but did not show a difference in tumbling with male or female intruders on trial 1, t (12) = 2.13 P = .171.
Aggressive grooming was displayed by less than half of experimental females on any given trial, and thus, in this case, nonparametric tests were performed. Kruskal-Wallis test revealed a significant effect of genotype for aggressive grooming of female intruders on the first trial, X2 (2) = 8.851, P = .012, but no differences were found on trial 2, X2 (2) = 1.674, P = .433, or trial 3, X2 (2) = 1.12, P = .571. A genotype effect of aggressive grooming was also found for trial 2 with a male intruder, X2 (2) = 6.848, P = .033, but this effect was not found on trial 1, X2 (2) = 0.118, P = .942, or trial 3, X2 (2) = 4.585, P = .101. Mann-Whitney U tests were used to compare genotypes after significant Kruskal-Wallis tests. These post hoc analyses revealed that CMV-AR females displayed less aggressive grooming towards female intruders on trial 1 compared with both WT, U = 54, P = .041, and Nestin-AR females, U = 24, P = .002. Both CMV-AR and Nestin-AR females displayed less aggressive grooming towards male intruders on trial 2 compared with WT females, U = 48, P = .026 and U = 42, P = .047. Similar to the male genotype differences on chasing and tumbling, these results suggest that CMV-AR females and Nestin-AR females perform less aggressive grooming compared with WT females.
FOS immunohistochemistry
CMV-AR mice exhibited altered neural activation in the LS in response to male intruders. FOS analyses indicated a marginal genotype by resident sex interaction, F2,47 = 3.063, P = .058. Post hoc tests suggest that CMV-AR mice do not have the typical sexually differentiated FOS response in the LS to male intruders; no pairwise comparisons were statistically significant, although there was a marginal effect for CMV-AR males having fewer FOS-ir cells than Nes-AR males, P = .057 (see Figure 7).
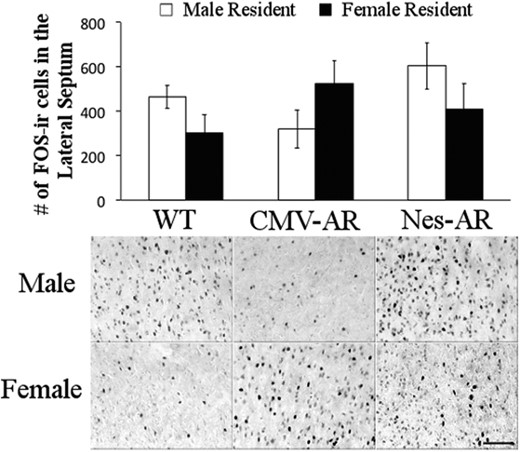
Neural activity in response to male intruder. FOS-ir cells in the lateral septum of male residents (white bars) and female residents (black bars). Ninety minutes before collection of tissue, all experimental mice were exposed to a male intruder for 10 minutes. A significant sex by genotype interaction, such that CMV-AR male mice have a decrease in FOS-ir cells, whereas CMV-AR females have an increase in FOS-ir cells. Scale bar, 100 μm. Error bars, ±SE.
Additionally, a marginal effect of sex was found in the NAcc, such that males had more FOS-ir cells than females, F1,47 = 3.773, P = .059.
Discussion
The present study compared socio-sexual behaviors in a model of global AR overexpression with a model of neural-only AR overexpression. Results agree with previous reports that neural AR contributes to male-typical aggressive and socio-sexual behaviors. Interestingly, several effects were observed in CMV-AR but not Nes-AR mice, suggesting novel roles for nonneural AR in sexual differentiation of socio-sexual behavior. Notably, CMV-AR males exhibited less male-typical anogenital investigation, and both male and female CMV-AR mice showed increased thrusting during mounts.
Studies of neural-specific ARKO mice have demonstrated the necessity of neural AR for male-typical copulatory behaviors (3, 4). Less explored is the idea that nonneural AR can influence sexual behaviors. In light of the central role of the genitals in mediating sexual sensation, and the evidence for latent organizational actions on CMV-AR female genitals (Figure 2), it is tempting to speculate that this tissue is the nonneural AR target influencing thrusting behavior in these animals. Increased accessibility of the genitals due to hypertrophy, increases in genital sensation and/or increased masculinization of associated neural structures might contribute to increased sexual reward and reinforcement with multiple trials. The hypothesis that androgens can act primarily on the genitals to cause sexual differentiation of copulatory behavior goes back to the pioneers of this field, most notably Frank Beach (eg, Ref. 27; for review, see Ref. 28) but has largely been discounted in favor of overwhelming evidence that steroid hormones alter the structure and function of the nervous system. Although we cannot rule out synergistic actions of neural and nonneural AR in masculinizing thrusting in CMV-AR mice, our findings provide evidence that Beach's hypothesis may be at least partly correct.
Interestingly, global, but not neural AR overexpression in males increased same-sex anogenital investigation. This finding might be explained either by a sensory alteration, and/or a motivational alteration. It seems unlikely that transgenics were unable to discriminate between males and females because both global and neural overexpression models exhibit different social behaviors to male and female intruders. Similarly, global, but not neural, loss of AR function mutations disrupted male-typical preference for female olfactory cues (3, 4, 6). However, it is possible that global overexpression mice cannot differentiate between receptive (in behavioral estrus) females compared with nonreceptive females. This inability to discriminate or indifference to these cues could potentially explain the increased thrusting behavior in the global overexpression mice. Although the reason behind the increase in same-sex anogenital investigation remains unresolved in the current study, our results provide further evidence that nonneural AR contributes to the male-typical anogenital investigation.
Loss of function AR mutations generally result in reduced male-typical socio-sexual behaviors (1–4). One would therefore predict that AR overexpression would result in a hypermasculine socio-sexual phenotype. Curiously, in many cases we observed alterations in socio-sexual phenotype similar to those associated with loss of AR function. A decrease in male-typical behaviors was observed for both aggressive and sexual behaviors as well as an increase in anogenital investigation. It is unlikely that the transgene AR is nonfunctional, or that it has simple dominant negative properties, because CMV-AR females exhibit clear evidence for masculinized genitals (Figure 2), a hallmark developmental action of AR. Reductions in male-typical outcomes due to AR overexpression therefore suggest a nonlinear relationship between AR signaling and male-typical sexual differentiation in which either insufficient activation or excessive activation results in loss of masculine phenotype. In both instances, loss of AR function then leads to a decrease in male-typical behaviors and/or an increase in female-typical behaviors that are typically suppressed in males. Similar examples of loss of AR function due to overexpression have been reported. For example, AR overexpression selectively in skeletal muscle results muscle weakness and atrophy (18), and patterns of muscle gene expression which are typical of ARKO models (29). Similarly, a paradoxical increase in AR in the MePD is observed in noncopulating rats (30). Furthermore, as discussed below, supraphysiological levels of androgens can result in same-sex mate preference in males, a female-typical behavior that results with low androgen exposure (10, 11, 33, 34).
There is considerable interest in the biological mechanisms that support attraction to opposite sex cues. A current theoretical model of same sex orientation posits that relatively low androgen signaling in early development promotes androphilia, and relatively high androgen signaling promotes gynephilia (for review, see Refs. 31, 32). Paradoxical loss of AR function might explain the apparent increase in androphilia in CMV-AR males. In these males, the time spent investigating a female intruder was not decreased compared with WT and Nes-AR males, but the time spent investigating a male partner increased was increased compared with WT and Nes-AR males. Interestingly, others have proposed that androphilia may be produced by supraphysiological androgen signaling. For example, Henley et al (11) reported that, in rats, supraphysiological levels of androgens suppress male typical sexual behaviors and increase preference for same-sex partners (10, 11, 33, 34). Hypersensitivity to androgens has also been proposed to play a role in sexual orientation in humans. For example, homosexual men have been shown to have increases in androgen-dependent measures, such as larger penile size and hypermasculine auditory evoked potentials (35, 36).
Taken together with studies of loss of function AR mutants, the current study highlights the importance of both neural and nonneural AR in sexual differentiation of socio-sexual behaviors. However, several questions remain unresolved. Firstly, these experiments draw inferences based on AR manipulations that are present throughout development, as well as in adulthood. This not only raises the possibility of compensatory effects, but more to the point does not distinguish between organizational and/or activational effects of neural and/or nonneural AR. Although neither the mutants in the current study nor Nestin-ARKO mice differ in adult circulating testosterone, it remains possible that subtle differences in hypothalamic pituitary gonadal regulation either in adulthood or through development might contribute to altered socio-sexual behavior. Future studies should therefore address the importance of developmental testosterone action in the observed effects. Another important limitation of these models relates to difficulty with spatial resolution in the models. It is possible that there is regional variability in the efficacy of the genetic manipulation. In the present study, we do not find statistical increases of AR in CMV-AR and Nes-AR males in the MePD, and previous reports of Nestin-ARKO mice indicate that these knockouts exhibit some residual AR-ir in a number of brain regions (4). It is possible that these regional differences in AR might contribute to the observed effects, and it seems entirely likely that AR action in normal development is disproportionately localized to key brain regions in light of large regional differences in AR expression in the nervous system. Spatially restricted genetic approaches might shed light on this question. Lastly, pathology, such as motoneuron and muscle degeneration, has been previously reported with AR overexpression models (18); however, it should be noted that this is unlikely to explain the current findings. The overexpression models which exhibit neural pathology overexpressed AR to a much greater degree (∼100-fold), and there was a clear threshold, in which slightly lower levels of overexpression did not result in frank pathology. The present models only exhibit 3- to 4.5-fold increases in AR in neural and nonneural tissues, and we observed no obvious clinical or histological signs of pathology.
In conclusion, the present study suggests that nonneural AR has a role in the sexual differentiation of socio-sexual behaviors and their neural substrates, and that excess AR may lead to loss of male-typical behaviors. Discrepancies in anogenital investigation and copulatory behaviors between global and neural AR overexpression models suggest that nonneural sites of action should be considered. For example, AR in nonneural tissues surrounding and/or part of the vomeronasal organ could be explored as a site of action in male-typical anogenital investigation, and genital tissue could be explored as a site of action for male-typical thrusting behaviors. Future investigation may also investigate the mechanism by which increased AR leads to loss of function.
Acknowledgments
We thank Kelyn Montano for her contributions to behavioral testing.
This work was supported by the National Sciences and Engineering Research Council of Canada (NSERC) Discovery Grant RGPIN 312458 and the Canadian Institutes of Health Research Operating Grant JNM-116637 (to D.A.M.) and by a doctoral Canadian Graduate Scholarship NSERC grant (A.S.-G.).
Disclosure Summary: The authors have nothing to disclose.
Abbreviations
- AR
androgen receptor
- AR-ir
AR-immunoreactivity
- ARKO
AR knockout
- BNST
bed nucleus of the stria terminalis
- CMV
cytomegalovirus
- IHC
immunohistochemistry
- LS
lateral septum
- MePD
medial amygdala posterior division
- MPOA
medial preoptic area
- NAcc
nucleus accumbens
- Nes
Nestin
- PND
postnatal day
- Tfm
testicular feminization mutation
- WT
wild type.