-
PDF
- Split View
-
Views
-
Cite
Cite
Maria E. Nilsson, Liesbeth Vandenput, Åsa Tivesten, Anna-Karin Norlén, Marie K. Lagerquist, Sara H. Windahl, Anna E. Börjesson, Helen H. Farman, Matti Poutanen, Anna Benrick, Manuel Maliqueo, Elisabet Stener-Victorin, Henrik Ryberg, Claes Ohlsson, Measurement of a Comprehensive Sex Steroid Profile in Rodent Serum by High-Sensitive Gas Chromatography-Tandem Mass Spectrometry, Endocrinology, Volume 156, Issue 7, 1 July 2015, Pages 2492–2502, https://doi.org/10.1210/en.2014-1890
- Share Icon Share
Accurate measurement of sex steroid concentrations in rodent serum is essential to evaluate mouse and rat models for sex steroid-related disorders. The aim of the present study was to develop a sensitive and specific gas chromatography-tandem mass spectrometry (GC-MS/MS) method to assess a comprehensive sex steroid profile in rodent serum. A major effort was invested in reaching an exceptionally high sensitivity for measuring serum estradiol concentrations. We established a GC-MS/MS assay with a lower limit of detection for estradiol, estrone, T, DHT, progesterone, androstenedione, and dehydroepiandrosterone of 0.3, 0.5, 4.0, 1.6, 8, 4.0, and 50 pg/mL, respectively, whereas the corresponding values for the lower limit of quantification were 0.5, 0.5, 8, 2.5, 74, 12, and 400 pg/mL, respectively. Calibration curves were linear, intra- and interassay coefficients of variation were low, and accuracy was excellent for all analytes. The established assay was used to accurately measure a comprehensive sex steroid profile in female rats and mice according to estrous cycle phase. In addition, we characterized the impact of age, sex, gonadectomy, and estradiol treatment on serum concentrations of these sex hormones in mice. In conclusion, we have established a highly sensitive and specific GC-MS/MS method to assess a comprehensive sex steroid profile in rodent serum in a single run. This GC-MS/MS assay has, to the best of our knowledge, the best detectability reported for estradiol. Our method therefore represents an ideal tool to characterize sex steroid metabolism in a variety of sex steroid-related rodent models and in human samples with low estradiol levels.
Serum levels of sex steroid hormones are routinely measured by immunoassay-based techniques in clinical and research settings. However, these assays have a questionable specificity, especially at the lower concentration range (1–4). In this respect, we have shown that immunoassays for serum estradiol show interference by a C-reactive protein-related factor, which can influence the associations between serum estradiol and inflammation-related sex steroid-dependent outcomes (5). Highly specific and sensitive assays for the quantification of sex steroids are required to study the role of sex steroids in disorders such as osteoporosis, breast cancer, prostate cancer, polycystic ovary syndrome, and cardiovascular diseases. More specifically, the need to accurately measure the low levels of serum estradiol present in children, postmenopausal women, and men was recently acknowledged (6). Also, the characterization of the serum sex steroids in genetically modified mouse models requires precise and sensitive methods, taking into account the limited serum volumes available from rodents. Mass spectrometry (MS) represents the most advanced technique for the quantification of sex steroids in serum with the advantage to simultaneously analyze multiple analytes within one sample run with high selectivity, sensitivity, precision, and accuracy.
There are two major differences in sex steroid metabolism between humans and rodents. First, a substantial amount of serum sex steroids is bound with high affinity to SHBG in humans, whereas rodents do not have this carrier protein (7). Second, humans, but not rodents, secrete substantial amounts of C-19 androgen precursors, ie, dehydroepiandrosterone (DHEA) and androstenedione, from the adrenal gland (8). These differences influence the total serum concentrations of sex steroids and sex steroid precursors, which are thus low and difficult to determine in rodents. Using liquid chromatography coupled with tandem MS (LC-MS/MS), detectable levels of T were shown in serum from male and female mice (9). However, the circulating estradiol levels were below the limit of quantification of the assay (<5 pg/mL) both in adult male and female mice (9, 10). Several previous studies have evaluated cyclic changes in serum estradiol levels in female rodents using either direct immunoassays (11) or immunoassay methods using preanalytical extraction, often with chromatography, to increase the assay specificity (12–20). However, to date, no analyses of serum steroids in female rodents according to estrus cycle stage have been performed using sensitive MS-based methods.
The aim of our study, therefore, was to establish a highly sensitive and specific gas chromatography tandem MS (GC-MS/MS) method to assess a comprehensive sex steroid profile (estradiol, estrone, T, DHT, progesterone, androstenedione, and DHEA) in rodent serum in a single run. A major effort was invested in reaching an exceptionally high sensitivity for serum estradiol measurements to be able to analyze this analyte in female rodents.
Materials and Methods
Reagents
Isotope-labeled internal standards of the steroids estradiol (estradiol-2,3,4-13C3), estrone (estrone-2,3,4-13C3), T (testosterone-2,3,4-13C3), DHT (dihydrotestosterone-2,3,4-13C3), progesterone (progesterone-2,3,4-13C3), androstenedione (androstenedione-2,3,4-13C3), and DHEA (dehydroepiandrosterone-2,2,3,4,4,6-d6) were from Sigma-Aldrich. Pentane, ethylacetate, methanol, and heptane were from Merck. Isooctane, toluene anhydrous 99.8%, 1-chlorobutane, and pyridine were from Sigma-Aldrich.
Calibrators
The calibrator stock solutions of each steroid were prepared separately by weighing out material and dissolving in ethyl acetate. Next, the stock solutions of the seven different analytes were pooled to a calibrator standard solution and diluted in methanol. On each day of analysis, seven-point calibration curves, including zero, were prepared from the calibrator standard solutions by dilution in water (1:10), thereby obtaining a range of concentrations as described in Supplemental Table 1. Estradiol, estrone, DHT, progesterone, androstenedione, and DHEA were purchased from Sigma-Aldrich. T was purchased from Fluka. Calibration was performed by determining the peak area ratio between the target analyte and the isotope-labeled internal standard.
Sample preparation
Steroid extraction
Samples consisted of 250 μL serum to which 200 μL deionized water was added (for a final volume of 450 μL) or 450 μL of the calibration standards. After addition of 50 μL internal standards (isotope labeled steroids in methanol) to each sample, 500 μL of 0.5 M ammonium acetate was added. After vortexing, 3 mL 1-chlorobutane was then added to each tube, and the tubes were rotated for 10 minutes. After centrifugation, the organic extracts were collected and purified on Silica SPE columns (Hypersep Si 500 mg; Thermo Scientific), which were first activated with 3 mL water-saturated ethylacetate and conditioned with 3 mL of ethylacetate-pentane-heptane [10:45:45(vol/vol)]. The columns and the adsorbed fraction were then washed with 6 mL ethylacetate-pentane-heptane [10:45:45(vol/vol)]. The analytes of interest were finally eluted using 3 mL ethylacetate-pentane-heptane [50:25:25(vol/vol)], and the organic solvent was evaporated at 35°C.
Derivatization
For oximation of the keto-groups, samples were reconstituted in 50 μL of 2% triethylamine in pyridine, and next, 50 μL of a 5 mM pentafluorobenzylhydroxylamine hydrochloride solution in methanol was added. The tubes were incubated at 60°C for 10 minutes and the organic solution was evaporated. Subsequently, hydroxyl-groups were esterificated using 500 μL of a 5% solution of pentafluorobenzoyl chloride and 50 μL of a 1% triethylamine in toluene solution. Samples were incubated for 20 minutes at room temperature. To each sample, 2 mL water was added, and after centrifugation for 5 minutes at 3000 rpm, the upper organic layer was transferred to a GC vial and the sample was dried at 35°C and reconstituted in 100 μL isooctane. The derivatization strategy of combining both oximation and esterification of steroids has been described before (Agilent Application Note, www.chem.agilent.com/library/applications/5990–9478EN.pdf). However, the derivatization protocols are different. In our hands the best performance was achieved when starting with the oximation step instead of the esterification step. We also further refined the reagent solutions, incubation times, and temperatures to obtain the best yield of derivatization products and to be able to expand the number of analytes.
Gas chromatography-tandem mass spectrometry
Analytes dissolved in isooctane were injected on an Agilent 7890A GC with a temperature-programmed injector operating in pulsed splitless mode using an Agilent 7693 autosampler. The injector was equipped with an Agilent Ultra inert liner with glass wool. Analytes were separated on two 50% phenylmethyl polysiloxane (DB-17HT) capillary columns (15 m × 0.25 mm internal diameter, 0.15 m film thickness), interconnected with a back flush system, with helium as the carrier gas at a flow rate of 1.0 mL/min. The GC temperature program started at 90°C, was at hold for 1.5 minutes, then ramped to 190°C at 120°C/min, thereafter to 277°C at 15°C/min, and finally to 330°C at 7°C/min. The GC gradient ended at 15.7 minutes and was thereafter set at hold at 330°C for 6 minutes to allow the column to bake out. Analytes were detected with electron capture negative chemical ionization in multiple reaction monitoring (MRM) mode using an Agilent 7000 triple quadrupole mass spectrometer. Ammonia was used as reagent gas at 1.6 mL/min flow rate. Helium quench gas flow rate was 2.25 mL/min and nitrogen was used as collision gas at 1.5 mL/min flow rate.
The following transitions were used for the quantification: estradiol, 660.1→596.2; estradiol-13C3, 663.1→599.2; estrone, 464.1→400.2; estrone-13C3, 467.1→403.2; T, 677.2→496.2; testosterone-13C3, 680.2→499.2; DHT, 679.2→181.0; dihydrotestosterone-13C3, 682.2→181.0; progesterone, 489.2→459.3; progesterone-13C3, 492.2→462.3; androstenedione, 461.2→431.2; androstenedione-13C3, 464.2→434.2; dehydroepiandrosterone, 482.2→167.0; dehydroepiandrosterone-d6, 488.2→167.0 (Supplemental Table 1). The dwell time for the MRM channels was optimized to achieve a minimum of 15 data points over all analyte peaks and was between 50 and 75 msec. All peaks were automatically integrated using the MassHunter quantitative analysis Workstation Software from Agilent. The Agile integrator was used for estradiol, T, DHT, progesterone, DHEA, and their corresponding internal standards. For estrone, androstenedione, and their corresponding internal standards, the General integrator was used. Calibration samples with accuracy between 80% and 120% were used for the calibration curves. Representative examples of extracted ion chromatograms of target steroids are shown in Figure 1. During method development, it was observed that the oximation step generates two derivatization products. Under the chromatographic conditions used for all ketosteroids, the two isoforms coeluted in one single peak, except for DHT, which eluted as two baseline-separated peaks with seemingly equal intensities (Figure 1). Because of difficulties for the automatic peak integration to handle multiple peaks for one analyte and interference close to the first eluting DHT peak (retention time 16.5 min), we selected the last eluting DHT peak (retention time 16.7 min) to be integrated and used for quantitation. The retention times of the single peaks for the other analytes are reported in Supplemental Table 1. The structures of the steroids in the native and derivatized state are provided in Supplemental Figure 1.
Representative examples of extracted ion chromatograms of target steroids
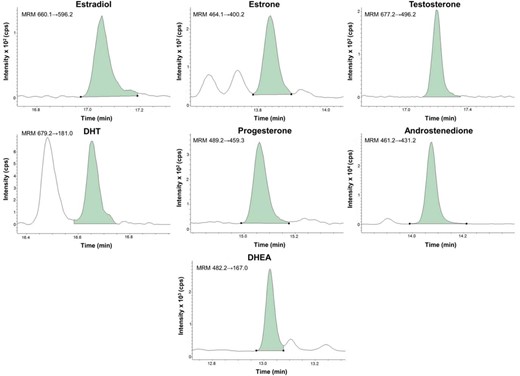
Steroid concentrations in the samples were calculated as estradiol, 8.2 pg/mL; estrone, 8.1 pg/mL; T, 68 pg/mL; DHT, 23 pg/mL; progesterone, 74 pg/mL; androstenedione, 320 pg/mL; and DHEA, 1100 pg/mL.
Evaluation of assay performance
Linearity of the calibration curves was determined by calculating the R2 (Supplemental Table 1). To determine the intraassay and interassay coefficients of variation (CVs), 12 aliquots from two pools of human serum (with low and high levels of the analytes) were analyzed on one occasion and three aliquots were analyzed on four different occasions, respectively (Table 1). Precision analyses were performed using 13C3-labeled (estradiol, estrone, T, DHT, progesterone, androstenedione) or d6-labeled (DHEA) analytes to distinguish from the endogenous analyte levels present in rat serum.
. | Estradiol . | Estrone . | T . | DHT . | Progesterone . | Androstenedione . | DHEA . |
---|---|---|---|---|---|---|---|
Sensitivity | |||||||
LLOD, pg/mL | 0.3 | 0.5 | 4 | 1.6 | 8 | 4 | 50 |
LLOQ, pg/mL | 0.5 | 0.5 | 8 | 2.5 | 74 | 12 | 400 |
Precision | |||||||
Intraassay CV | |||||||
QC-low | 2.2% (8.2) | 1.3% (8.1) | 1.6% (68) | 3.0% (23) | 1.9% (74) | 1.9% (321) | 1.0% (1069) |
QC-high | 0.7% (127) | 0.7% (172) | 1.2% (1745) | 3.3% (475) | 0.5% (3770) | 1.5% (365) | 1.2% (8095) |
Interassay CV | |||||||
QC-low | 4.3% (8.2) | 5.5% (8.1) | 2.8% (68) | 4.0% (23) | 6.5% (74) | 12.4% (321) | 7.0% (1069) |
QC-high | 2.1% (127) | 1.6% (172) | 2.8% (1745) | 2.2% (475) | 1.6% (3770) | 6.1% (365) | 3.2% (8095) |
. | Estradiol . | Estrone . | T . | DHT . | Progesterone . | Androstenedione . | DHEA . |
---|---|---|---|---|---|---|---|
Sensitivity | |||||||
LLOD, pg/mL | 0.3 | 0.5 | 4 | 1.6 | 8 | 4 | 50 |
LLOQ, pg/mL | 0.5 | 0.5 | 8 | 2.5 | 74 | 12 | 400 |
Precision | |||||||
Intraassay CV | |||||||
QC-low | 2.2% (8.2) | 1.3% (8.1) | 1.6% (68) | 3.0% (23) | 1.9% (74) | 1.9% (321) | 1.0% (1069) |
QC-high | 0.7% (127) | 0.7% (172) | 1.2% (1745) | 3.3% (475) | 0.5% (3770) | 1.5% (365) | 1.2% (8095) |
Interassay CV | |||||||
QC-low | 4.3% (8.2) | 5.5% (8.1) | 2.8% (68) | 4.0% (23) | 6.5% (74) | 12.4% (321) | 7.0% (1069) |
QC-high | 2.1% (127) | 1.6% (172) | 2.8% (1745) | 2.2% (475) | 1.6% (3770) | 6.1% (365) | 3.2% (8095) |
Abbreviations: QC-low, quality control sample with low concentration; QC-high, quality control sample with high concentration. Values within brackets indicate the concentration for the QC in picograms per milliliter. Sensitivity was evaluated in water, whereas precision was assessed in human serum. The on-column injection amounts for estradiol, estrone, T, DHT, progesterone, androstenedione, and DHEA were for QC-low 103, 101, 850, 288, 925, 4013, and 13 363 fg, respectively, whereas the corresponding values for QC-high were 1588, 2150, 21 813, 5938, 47 125, 4563, and 101 188 fg, respectively.
. | Estradiol . | Estrone . | T . | DHT . | Progesterone . | Androstenedione . | DHEA . |
---|---|---|---|---|---|---|---|
Sensitivity | |||||||
LLOD, pg/mL | 0.3 | 0.5 | 4 | 1.6 | 8 | 4 | 50 |
LLOQ, pg/mL | 0.5 | 0.5 | 8 | 2.5 | 74 | 12 | 400 |
Precision | |||||||
Intraassay CV | |||||||
QC-low | 2.2% (8.2) | 1.3% (8.1) | 1.6% (68) | 3.0% (23) | 1.9% (74) | 1.9% (321) | 1.0% (1069) |
QC-high | 0.7% (127) | 0.7% (172) | 1.2% (1745) | 3.3% (475) | 0.5% (3770) | 1.5% (365) | 1.2% (8095) |
Interassay CV | |||||||
QC-low | 4.3% (8.2) | 5.5% (8.1) | 2.8% (68) | 4.0% (23) | 6.5% (74) | 12.4% (321) | 7.0% (1069) |
QC-high | 2.1% (127) | 1.6% (172) | 2.8% (1745) | 2.2% (475) | 1.6% (3770) | 6.1% (365) | 3.2% (8095) |
. | Estradiol . | Estrone . | T . | DHT . | Progesterone . | Androstenedione . | DHEA . |
---|---|---|---|---|---|---|---|
Sensitivity | |||||||
LLOD, pg/mL | 0.3 | 0.5 | 4 | 1.6 | 8 | 4 | 50 |
LLOQ, pg/mL | 0.5 | 0.5 | 8 | 2.5 | 74 | 12 | 400 |
Precision | |||||||
Intraassay CV | |||||||
QC-low | 2.2% (8.2) | 1.3% (8.1) | 1.6% (68) | 3.0% (23) | 1.9% (74) | 1.9% (321) | 1.0% (1069) |
QC-high | 0.7% (127) | 0.7% (172) | 1.2% (1745) | 3.3% (475) | 0.5% (3770) | 1.5% (365) | 1.2% (8095) |
Interassay CV | |||||||
QC-low | 4.3% (8.2) | 5.5% (8.1) | 2.8% (68) | 4.0% (23) | 6.5% (74) | 12.4% (321) | 7.0% (1069) |
QC-high | 2.1% (127) | 1.6% (172) | 2.8% (1745) | 2.2% (475) | 1.6% (3770) | 6.1% (365) | 3.2% (8095) |
Abbreviations: QC-low, quality control sample with low concentration; QC-high, quality control sample with high concentration. Values within brackets indicate the concentration for the QC in picograms per milliliter. Sensitivity was evaluated in water, whereas precision was assessed in human serum. The on-column injection amounts for estradiol, estrone, T, DHT, progesterone, androstenedione, and DHEA were for QC-low 103, 101, 850, 288, 925, 4013, and 13 363 fg, respectively, whereas the corresponding values for QC-high were 1588, 2150, 21 813, 5938, 47 125, 4563, and 101 188 fg, respectively.
The lower limit of detection (LLOD) was defined as the lowest peak having a signal at > 3 times the noise level. The lower limit of quantification (LLOQ) was defined as the lowest peak that was reproducible with a CV of less than 20% and an accuracy of 80%-120%. At least seven different levels in the lower concentration range of each analyte were evaluated to determine the LLOD and LLOQ (Supplemental Tables 2 and 3). The noise levels for the zero calibrator (including the internal standard solution) were 0.6, 55, 2, 0.4, 50, 300, and 700 counts per second (cps) for estradiol, estrone, T, DHT, progesterone, androstenedione, and DHEA, respectively. The noise levels for a blank specimen (containing rat serum) were 1.6, 140, 1.5, 0.4, 150, 250, and 1600 cps for estradiol, estrone, T, DHT, progesterone, androstenedione, and DHEA, respectively.
The extraction recovery was evaluated with isotope-labeled steroids and calculated as the ratio between samples spiked before and after the SPE-column extraction. Aliquots of serum were spiked with two levels of calibrator standard solutions containing the target analytes to assess accuracy, which was calculated as follows: [(observed value − baseline value)/amount spiked]100 (Table 2 and Supplemental Table 4).
. | Spiked, pg/mL . | Baseline, pg/mL . | Accuracy, % . |
---|---|---|---|
Estradiol | |||
Low | 2.0 | 10.4 | 116 |
High | 120 | 10.4 | 112 |
Estrone | |||
Low | 2.0 | 9.0 | 100 |
High | 120 | 9.0 | 109 |
T | |||
Low | 25.0 | 65.4 | 116 |
High | 1500 | 65.4 | 104 |
DHT | |||
Low | 5.0 | 22.6 | 85 |
High | 300 | 22.6 | 102 |
Progesterone | |||
Low | 50.0 | 78.0 | 105 |
High | 3000 | 78.0 | 103 |
Androstenedione | |||
Low | 100 | 382 | 82 |
High | 750 | 382 | 99 |
DHEA | |||
Low | 50.0 | 946 | 115 |
High | 3000 | 946 | 100 |
. | Spiked, pg/mL . | Baseline, pg/mL . | Accuracy, % . |
---|---|---|---|
Estradiol | |||
Low | 2.0 | 10.4 | 116 |
High | 120 | 10.4 | 112 |
Estrone | |||
Low | 2.0 | 9.0 | 100 |
High | 120 | 9.0 | 109 |
T | |||
Low | 25.0 | 65.4 | 116 |
High | 1500 | 65.4 | 104 |
DHT | |||
Low | 5.0 | 22.6 | 85 |
High | 300 | 22.6 | 102 |
Progesterone | |||
Low | 50.0 | 78.0 | 105 |
High | 3000 | 78.0 | 103 |
Androstenedione | |||
Low | 100 | 382 | 82 |
High | 750 | 382 | 99 |
DHEA | |||
Low | 50.0 | 946 | 115 |
High | 3000 | 946 | 100 |
. | Spiked, pg/mL . | Baseline, pg/mL . | Accuracy, % . |
---|---|---|---|
Estradiol | |||
Low | 2.0 | 10.4 | 116 |
High | 120 | 10.4 | 112 |
Estrone | |||
Low | 2.0 | 9.0 | 100 |
High | 120 | 9.0 | 109 |
T | |||
Low | 25.0 | 65.4 | 116 |
High | 1500 | 65.4 | 104 |
DHT | |||
Low | 5.0 | 22.6 | 85 |
High | 300 | 22.6 | 102 |
Progesterone | |||
Low | 50.0 | 78.0 | 105 |
High | 3000 | 78.0 | 103 |
Androstenedione | |||
Low | 100 | 382 | 82 |
High | 750 | 382 | 99 |
DHEA | |||
Low | 50.0 | 946 | 115 |
High | 3000 | 946 | 100 |
. | Spiked, pg/mL . | Baseline, pg/mL . | Accuracy, % . |
---|---|---|---|
Estradiol | |||
Low | 2.0 | 10.4 | 116 |
High | 120 | 10.4 | 112 |
Estrone | |||
Low | 2.0 | 9.0 | 100 |
High | 120 | 9.0 | 109 |
T | |||
Low | 25.0 | 65.4 | 116 |
High | 1500 | 65.4 | 104 |
DHT | |||
Low | 5.0 | 22.6 | 85 |
High | 300 | 22.6 | 102 |
Progesterone | |||
Low | 50.0 | 78.0 | 105 |
High | 3000 | 78.0 | 103 |
Androstenedione | |||
Low | 100 | 382 | 82 |
High | 750 | 382 | 99 |
DHEA | |||
Low | 50.0 | 946 | 115 |
High | 3000 | 946 | 100 |
Method comparison
The assay for T was validated by the Hormone Standardization Project at the Centers for Disease Control and Prevention (Atlanta, Georgia) using isotope-dilution LC-tandem MS (21). The agreement of assay techniques was visualized using Passing Bablok regression analyses and Bland-Altman analyses and evaluated by calculating the R2.
Animals
The rats and mice were housed in a standard animal facility under controlled temperature (22°C) and photoperiod (12 h light, 12 h dark) and fed standard pellet diet and tap water ad libitum. Animal care was in accordance with institutional guidelines, and the study was approved by the local ethical committee at the University of Gothenburg.
The estrous cycle phase of adult 3- to 4-month-old female C57BL6 mice (Taconic) and Wistar rats (Charles River Laboratories) was determined by a microscopic analysis of the predominant cell types obtained via vaginal smears. In addition, we evaluated serum sex steroid levels in young adult (3–4 mo old) gonadal intact, gonadectomized (gx), and estradiol-treated gx male and female C57BL6 mice. The gx was performed 4 weeks before the termination of the experiment, followed by vehicle or estradiol (830 ng per mouse per day, using slow release pellets; Innovative Research of America) treatment. The serum levels in the young adult mice were also compared with serum levels in 1-year-old C57BL6 mice and in humans (Tables 3 and 4). Finally, serum levels in 3- to 4-month-old female estrogen receptor-α depleted (ERα−/−) mice were compared with young adult random cycling C57BL6 wild-type mice. The rodents were killed by cardiac puncture under isoflurane (rats) or Dormitor/Ketalar (mice) anesthesia and 250 μL of serum was analyzed by GC-MS/MS. Differences in serum sex steroid levels between the different groups were evaluated with a Kruskal-Wallis followed by a Mann-Whitney test. For those samples with sex steroid levels below the respective LLOD, the data were treated as zero in the analyses.
. | Young Adult Mice . | Old Mice . | Premenopausal Women (n = 15) . | |||
---|---|---|---|---|---|---|
Gonadal Intact (n = 44) . | ovx (n = 8) . | ovx + E2 (n = 6) . | ERα−/− (n = 6) . | Gonadal Intact (n = 6) . | ||
Estradiol, pg/mL | 2.7 ± 1.0 | <0.3a | 44.3 ± 8.0b,c | 55.9 ± 10.6b | 3.6 ± 0.7a | 54.9 ± 10.8b |
Estrone, pg/mL | 0.4 ± 0.1 | <0.5 | <0.5 | 64.5 ± 12.0b | 0.3 ± 0.2 | 42.4 ± 4.1b |
T, pg/mL | 51.9 ± 5.5 | 6.9 ± 2.7b | <4b | 1905 ± 247b | 23.5 ± 5.0a | 243.3 ± 26.5b |
DHT, pg/mL | 2.8 ± 0.4 | 3.0 ± 0.7 | <1.6b,c | 39.6 ± 4.3b | 12.8 ± 2.6b | 81.7 ± 8.3b |
Progesterone, pg/mL | 31 323 ± 6108 | 3940 ± 2135b | 674 ± 241b | 3867 ± 724b | 4104 ± 1552b | 78.8 ± 16.5b |
Androstenedione, pg/mL | 42.9 ± 3.5 | NA | NA | NA | NA | 1088 ± 126b |
DHEA, pg/mL | <50 | NA | NA | NA | NA | 5783 ± 1236b |
. | Young Adult Mice . | Old Mice . | Premenopausal Women (n = 15) . | |||
---|---|---|---|---|---|---|
Gonadal Intact (n = 44) . | ovx (n = 8) . | ovx + E2 (n = 6) . | ERα−/− (n = 6) . | Gonadal Intact (n = 6) . | ||
Estradiol, pg/mL | 2.7 ± 1.0 | <0.3a | 44.3 ± 8.0b,c | 55.9 ± 10.6b | 3.6 ± 0.7a | 54.9 ± 10.8b |
Estrone, pg/mL | 0.4 ± 0.1 | <0.5 | <0.5 | 64.5 ± 12.0b | 0.3 ± 0.2 | 42.4 ± 4.1b |
T, pg/mL | 51.9 ± 5.5 | 6.9 ± 2.7b | <4b | 1905 ± 247b | 23.5 ± 5.0a | 243.3 ± 26.5b |
DHT, pg/mL | 2.8 ± 0.4 | 3.0 ± 0.7 | <1.6b,c | 39.6 ± 4.3b | 12.8 ± 2.6b | 81.7 ± 8.3b |
Progesterone, pg/mL | 31 323 ± 6108 | 3940 ± 2135b | 674 ± 241b | 3867 ± 724b | 4104 ± 1552b | 78.8 ± 16.5b |
Androstenedione, pg/mL | 42.9 ± 3.5 | NA | NA | NA | NA | 1088 ± 126b |
DHEA, pg/mL | <50 | NA | NA | NA | NA | 5783 ± 1236b |
Abbreviations: E2, estradiol; NA, not analyzed. Serum sex steroid levels in 3- to 4-month-old (young adult) and 1-year-old (old) female mice in random cycle phase as well as in premenopausal women in the early follicular phase (mean age 31.1 ± 1.5 y, cycle d 1–10). The gonadal intact young adult female mice were also compared with ovx mice and ovx mice treated with E2 (ovx + E2). In addition, the serum levels of ERα−/− mice with disturbed negative feedback regulation of serum sex steroids are shown. Values are given as mean ± SEM.
P < .05 vs gonadal intact young adult mice;
P < .01 vs gonadal intact young adult mice;
P < .01 vs ovx young adult mice.
. | Young Adult Mice . | Old Mice . | Premenopausal Women (n = 15) . | |||
---|---|---|---|---|---|---|
Gonadal Intact (n = 44) . | ovx (n = 8) . | ovx + E2 (n = 6) . | ERα−/− (n = 6) . | Gonadal Intact (n = 6) . | ||
Estradiol, pg/mL | 2.7 ± 1.0 | <0.3a | 44.3 ± 8.0b,c | 55.9 ± 10.6b | 3.6 ± 0.7a | 54.9 ± 10.8b |
Estrone, pg/mL | 0.4 ± 0.1 | <0.5 | <0.5 | 64.5 ± 12.0b | 0.3 ± 0.2 | 42.4 ± 4.1b |
T, pg/mL | 51.9 ± 5.5 | 6.9 ± 2.7b | <4b | 1905 ± 247b | 23.5 ± 5.0a | 243.3 ± 26.5b |
DHT, pg/mL | 2.8 ± 0.4 | 3.0 ± 0.7 | <1.6b,c | 39.6 ± 4.3b | 12.8 ± 2.6b | 81.7 ± 8.3b |
Progesterone, pg/mL | 31 323 ± 6108 | 3940 ± 2135b | 674 ± 241b | 3867 ± 724b | 4104 ± 1552b | 78.8 ± 16.5b |
Androstenedione, pg/mL | 42.9 ± 3.5 | NA | NA | NA | NA | 1088 ± 126b |
DHEA, pg/mL | <50 | NA | NA | NA | NA | 5783 ± 1236b |
. | Young Adult Mice . | Old Mice . | Premenopausal Women (n = 15) . | |||
---|---|---|---|---|---|---|
Gonadal Intact (n = 44) . | ovx (n = 8) . | ovx + E2 (n = 6) . | ERα−/− (n = 6) . | Gonadal Intact (n = 6) . | ||
Estradiol, pg/mL | 2.7 ± 1.0 | <0.3a | 44.3 ± 8.0b,c | 55.9 ± 10.6b | 3.6 ± 0.7a | 54.9 ± 10.8b |
Estrone, pg/mL | 0.4 ± 0.1 | <0.5 | <0.5 | 64.5 ± 12.0b | 0.3 ± 0.2 | 42.4 ± 4.1b |
T, pg/mL | 51.9 ± 5.5 | 6.9 ± 2.7b | <4b | 1905 ± 247b | 23.5 ± 5.0a | 243.3 ± 26.5b |
DHT, pg/mL | 2.8 ± 0.4 | 3.0 ± 0.7 | <1.6b,c | 39.6 ± 4.3b | 12.8 ± 2.6b | 81.7 ± 8.3b |
Progesterone, pg/mL | 31 323 ± 6108 | 3940 ± 2135b | 674 ± 241b | 3867 ± 724b | 4104 ± 1552b | 78.8 ± 16.5b |
Androstenedione, pg/mL | 42.9 ± 3.5 | NA | NA | NA | NA | 1088 ± 126b |
DHEA, pg/mL | <50 | NA | NA | NA | NA | 5783 ± 1236b |
Abbreviations: E2, estradiol; NA, not analyzed. Serum sex steroid levels in 3- to 4-month-old (young adult) and 1-year-old (old) female mice in random cycle phase as well as in premenopausal women in the early follicular phase (mean age 31.1 ± 1.5 y, cycle d 1–10). The gonadal intact young adult female mice were also compared with ovx mice and ovx mice treated with E2 (ovx + E2). In addition, the serum levels of ERα−/− mice with disturbed negative feedback regulation of serum sex steroids are shown. Values are given as mean ± SEM.
P < .05 vs gonadal intact young adult mice;
P < .01 vs gonadal intact young adult mice;
P < .01 vs ovx young adult mice.
. | Young Adult Mice . | Old Mice . | Old Men (n = 49) . | ||
---|---|---|---|---|---|
Gonadal Intact (n = 9) . | orx (n = 14) . | orx + E2 (n = 14) . | Gonadal Intact (n = 15) . | ||
Estradiol, pg/mL | <0.3 | <0.3 | 20.7 ± 3.1a,b | <0.3 | 23.0 ± 1.6a |
Estrone, pg/mL | <0.5 | <0.5 | <0.5 | <0.5 | 29.4 ± 1.9a |
T, pg/mL | 8235 ± 1055 | <4a | <4a | 957 ± 246a | 3170 ± 246a |
DHT, pg/mL | 168 ± 24 | <1.6a | <1.6a | 31 ± 6a | 281 ± 23c |
Progesterone, pg/mL | 680 ± 93 | 3428 ± 432a | 113 ± 22a,b | 617 ± 118 | 40.3 ± 3.6a |
Androstenedione, pg/mL | 207 ± 31 | NA | NA | NA | 517 ± 31a |
DHEA, pg/mL | <50 | NA | NA | NA | 1057 ± 108a |
. | Young Adult Mice . | Old Mice . | Old Men (n = 49) . | ||
---|---|---|---|---|---|
Gonadal Intact (n = 9) . | orx (n = 14) . | orx + E2 (n = 14) . | Gonadal Intact (n = 15) . | ||
Estradiol, pg/mL | <0.3 | <0.3 | 20.7 ± 3.1a,b | <0.3 | 23.0 ± 1.6a |
Estrone, pg/mL | <0.5 | <0.5 | <0.5 | <0.5 | 29.4 ± 1.9a |
T, pg/mL | 8235 ± 1055 | <4a | <4a | 957 ± 246a | 3170 ± 246a |
DHT, pg/mL | 168 ± 24 | <1.6a | <1.6a | 31 ± 6a | 281 ± 23c |
Progesterone, pg/mL | 680 ± 93 | 3428 ± 432a | 113 ± 22a,b | 617 ± 118 | 40.3 ± 3.6a |
Androstenedione, pg/mL | 207 ± 31 | NA | NA | NA | 517 ± 31a |
DHEA, pg/mL | <50 | NA | NA | NA | 1057 ± 108a |
Abbreviations: E2, estradiol; NA, not analyzed. Serum sex steroid levels in 3- to 4-month-old (young adult) and 1-year-old (old) male mice as well as in 79-year-old men. The gonadal intact young adult male mice were also compared with orx mice and orx mice treated with E2 (orx + E2). Values are given as mean ± SEM.
P < .01 vs gonadal intact young adult mice;
P < .01 vs orx young adult mice;
P < .05 vs gonadal intact young adult mice.
. | Young Adult Mice . | Old Mice . | Old Men (n = 49) . | ||
---|---|---|---|---|---|
Gonadal Intact (n = 9) . | orx (n = 14) . | orx + E2 (n = 14) . | Gonadal Intact (n = 15) . | ||
Estradiol, pg/mL | <0.3 | <0.3 | 20.7 ± 3.1a,b | <0.3 | 23.0 ± 1.6a |
Estrone, pg/mL | <0.5 | <0.5 | <0.5 | <0.5 | 29.4 ± 1.9a |
T, pg/mL | 8235 ± 1055 | <4a | <4a | 957 ± 246a | 3170 ± 246a |
DHT, pg/mL | 168 ± 24 | <1.6a | <1.6a | 31 ± 6a | 281 ± 23c |
Progesterone, pg/mL | 680 ± 93 | 3428 ± 432a | 113 ± 22a,b | 617 ± 118 | 40.3 ± 3.6a |
Androstenedione, pg/mL | 207 ± 31 | NA | NA | NA | 517 ± 31a |
DHEA, pg/mL | <50 | NA | NA | NA | 1057 ± 108a |
. | Young Adult Mice . | Old Mice . | Old Men (n = 49) . | ||
---|---|---|---|---|---|
Gonadal Intact (n = 9) . | orx (n = 14) . | orx + E2 (n = 14) . | Gonadal Intact (n = 15) . | ||
Estradiol, pg/mL | <0.3 | <0.3 | 20.7 ± 3.1a,b | <0.3 | 23.0 ± 1.6a |
Estrone, pg/mL | <0.5 | <0.5 | <0.5 | <0.5 | 29.4 ± 1.9a |
T, pg/mL | 8235 ± 1055 | <4a | <4a | 957 ± 246a | 3170 ± 246a |
DHT, pg/mL | 168 ± 24 | <1.6a | <1.6a | 31 ± 6a | 281 ± 23c |
Progesterone, pg/mL | 680 ± 93 | 3428 ± 432a | 113 ± 22a,b | 617 ± 118 | 40.3 ± 3.6a |
Androstenedione, pg/mL | 207 ± 31 | NA | NA | NA | 517 ± 31a |
DHEA, pg/mL | <50 | NA | NA | NA | 1057 ± 108a |
Abbreviations: E2, estradiol; NA, not analyzed. Serum sex steroid levels in 3- to 4-month-old (young adult) and 1-year-old (old) male mice as well as in 79-year-old men. The gonadal intact young adult male mice were also compared with orx mice and orx mice treated with E2 (orx + E2). Values are given as mean ± SEM.
P < .01 vs gonadal intact young adult mice;
P < .01 vs orx young adult mice;
P < .05 vs gonadal intact young adult mice.
Results
Assay performance characteristics
Calibration curves were linear for all steroids (Supplemental Table 1). Evaluation of the sensitivity of the assay revealed that the LLOD for estradiol, estrone, T, DHT, progesterone, androstenedione, and DHEA when using 250 μL of water was 0.3, 0.5, 4, 1.6, 8, 4, and 50 pg/mL, respectively, whereas the corresponding values for the LLOQ were 0.5, 0.5, 8, 2.5, 74, 12, and 400 pg/mL, respectively (Table 1). Rather similar sensitivities were observed when using 250 μL of rat serum as matrix, revealing that the LLOD for estradiol, estrone, T, DHT, progesterone, androstenedione, and DHEA was 0.3, 0.4, 4, 1.5, 8, 3, and 25 pg/mL, respectively, whereas the corresponding values for the LLOQ were 0.5, 0.75, 8, 3.75, 56, 6, and 50 pg/mL, respectively (Supplemental Tables 2 and 3). Evaluation of the precision revealed that both the intra- and interassay CVs were less than 16% for all analytes both when determined using a low and a high level human control sample (Table 1) and when determined using a female and a male rat control sample (Supplemental Table 2). The extraction recoveries during the preparation of the samples were found to be greater than 80% for all steroids investigated (data not shown). The extraction recovery does not influence the absolute quantification of the analytes because internal standards are added to every sample before the initiation of the extraction. Accuracy was consistent and reproducible for each steroid quantified over two spiking levels tested when evaluated in human serum: estradiol, 112%-116%; estrone, 100%-109%; T, 104%-116%; DHT, 85%-102%; progesterone, 103%-105%; androstenedione, 82%-99%; and DHEA, 100%-115% (Table 2). This was also the case when evaluated in rat serum: estradiol, 89%-112%; estrone, 108%; T, 105%-114%; DHT, 88%-102%; progesterone, 92%-103%; androstenedione, 105%-111%; and DHEA, 102%-103% (Supplemental Table 4).
We participated in the international Hormone Standardization Project for serum T run by the CDC. Thirty-nine serum samples were analyzed during one certification year (2013), and we successfully passed the performance criterion of ±6.4% mean bias to the CDC T reference method in adult male and female serum over the concentration range of 25–10 000 pg/mL (calculated mean bias was −1.0% and R2 = 1.0). The excellent performance of the Gothenburg serum T assay vs the CDC reference method is displayed in a Passing-Bablok regression (Figure 2A) and a Bland-Altman plot (Figure 2B).
Assay validation of the Gothenburg GC-MS/MS vs the CDC reference LC-MS/MS method for serum T

A, Passing-Bablok regression for the serum T analysis (n = 39) using the Gothenburg GC-MS/MS and the CDC LC-MS/MS. The slope (solid black line) is calculated with 95% confidence bands (dashed black lines) and line of identity (dotted gray line). B, Bland-Altman plot of the difference in T concentrations between the Gothenburg GC-MS/MS and CDC LC-MS/MS against the mean of the two assays. The solid line represents the mean difference and the dashed lines the 95% limits of agreement.
Serum steroids in female rodent samples
Because no previous study has reported the cyclical changes in serum sex steroids in female rodents using sensitive MS-based methods, we first used our developed GC-MS/MS assay to measure the steroid profile in serum samples of mice and rats according to the estrous cycle stage. Serum levels of estradiol, estrone, T, DHT, progesterone, and androstenedione were clearly above the LLOD of the respective assay in diestrus and proestrus for both rats (Figure 3) and mice (Figure 4). Serum levels of T, DHT, progesterone, and androstenedione were also measurable in estrus and metestrus in both rats and mice. A majority of the estrus samples was below the LLOD for estradiol (<0.3 pg/mL) and estrone (<0.5 pg/mL) in both rats and mice (Figures 3 and 4), whereas the levels of these two estrogens were low, but in general above the LLOD, in metestrus. In both rats and mice, the highest estradiol and estrone levels were observed in proestrus. In rats, serum T levels were highest in proestrus and lowest in estrus and a similar but nonsignificant trend was observed in mice. Unexpectedly, we observed the highest serum DHT levels in metestrus compared with estrus in both rats and mice. The serum levels of progesterone were highest in estrus in rats. Furthermore, the highest serum levels of androstenedione were seen in proestrus and the lowest in estrus for rats, whereas no significant differences were seen in mice. Serum levels of DHEA were below the LLOD in both female and male mice and in female rats (Tables 3 and 4 and data not shown).
Serum sex steroids according to estrous stage in female rats

Cyclical changes in serum levels of estradiol (A), estrone (B), T (C), DHT (D), progesterone (E), and androstenedione (F) in 3- to 4-month-old female Wistar rats were analyzed by GC-MS/MS using 250 μL of serum. Estrous cycle phase was determined by a microscopic analysis of the predominant cell types obtained via vaginal smears (diestrus, n = 9; proestrus, n = 10; estrus, n = 6; metestrus, n = 7). Values are given as mean ± SEM. a, P < .05, A, P < .01 vs proestrus; b, P < .05, B, P < .01 vs estrus; c, P < .05, C, P < .01 vs metestrus.
Serum sex steroids according to estrous stage in female mice

Cyclical changes in serum levels of estradiol (A), estrone (B), T (C), DHT (D), progesterone (E), and androstenedione (F) in 3- to 4-month-old female C57BL6 mice were analyzed by GC-MS/MS using 250 μL of serum. Estrous cycle phase was determined by microscopic analysis of the predominant cell types obtained via vaginal smears (diestrus, n = 5; proestrus, n = 10; estrus, n = 17; metestrus, n = 12). Values are given as mean ± SEM. a, P < .05, A, P < .01 vs proestrus; b, P < .05, B, P < .01 vs estrus; c, P < .05, C, P < .01 vs metestrus.
Ovariectomy (ovx) resulted in serum levels of estradiol and estrone below the LLOD and significantly reduced serum levels of T and progesterone compared with female mice in random cycle phase (Table 3). Treatment of the ovx mice with estradiol increased serum estradiol levels substantially and decreased serum T, DHT, and progesterone compared with the ovx mice.
Serum levels of estradiol and DHT were slightly increased, whereas serum levels of T and progesterone were slightly reduced in 1-year-old female mice compared with young adult random cycling female mice (Table 3).
It was previously reported that the negative feedback regulation of sex steroids is disturbed in female mice with ERα gene inactivation (22–24). Accordingly, serum levels of estradiol, estrone, T, and DHT were substantially increased in female ERα−/− mice compared with wild-type mice (Table 3).
Serum steroids in male mouse samples
As expected, the serum levels of T and DHT were significantly higher, whereas the serum levels of progesterone were lower in young adult male mice compared with female mice in random cycle phase (P < .01; Tables 3 and 4). Estradiol and estrone concentrations were below the LLOD of our assay in male mice (Table 4). Orchidectomy (orx) significantly reduced the serum T and DHT concentrations but increased the serum progesterone levels compared with gonadal intact male mice (Table 4). Treatment of orx mice with estradiol resulted in high serum estradiol levels and reduced serum progesterone levels compared with orx mice. In addition, old male mice displayed reduced T and DHT compared with young adult male mice (Table 4).
Comparison of serum steroids between mouse and human samples
Finally, we compared serum sex steroid concentrations in human and mouse serum analyzed by our established GC-MS/MS method. Serum levels of estradiol and estrone were substantially higher in both premenopausal women (in the early follicular phase) and older men compared with young adult female and male mice, respectively (P < .01; Tables 3 and 4). Serum concentrations of T, DHT, androstenedione, and DHEA were higher, whereas serum progesterone was lower in premenopausal women in the early follicular phase compared with young adult female mice in random cycle phase (Table 3).
Discussion
We have developed a highly sensitive and specific GC-MS/MS method for the quantification of a comprehensive sex steroid profile in rodent serum in a single run. Our method is specific, accurate, and precise for the seven target steroids, including estradiol, estrone, T, DHT, progesterone, androstenedione, and DHEA. The GC-MS/MS assay has, to the best of our knowledge (9, 10, 25), the best sensitivity reported so far for estradiol, enabling serum estradiol measurements in rodents according to the estrous cycle phase, distinction between serum pools from gonadal intact and castrated animals, and analysis of human samples with very low estradiol levels. Taken together, this method is an improvement over previous methods for measuring sex steroid levels in rodents and represents a valuable contribution with respect to reference intervals in mice and rats.
In this study, we report for the first time estradiol and estrone levels in female rodents according to estrous cycle phase as analyzed by sensitive and specific MS methodology. The estradiol levels are highest in the follicular phase of the estrous cycle and should thus preferably be measured in rodents during the diestrus or proestrus stages. In contrast, the serum levels of estradiol are very low in the estrus phase (<0.3 pg/mL). Many previous studies have reported measurable immunoassay-based estradiol levels in female rodents, but the assays used have not even been able to distinguish between the estradiol levels in gonadal intact and ovx female mice, strongly suggesting that these findings are not reliable due to the low specificity of the estradiol immunoassays in the lower concentration range (1–4). Murine serum estradiol concentrations were below the LLOQ of the assay [5 pg/mL (9) and 2.8 pg/mL (10)] in two previous studies using specific MS-based methods, except for a recent study that applied an LC-MS/MS-based method with a sensitivity of 2.5 pg/mL and detected measurable estradiol levels in female mice at the diestrus stage (25). The estradiol concentrations reported in this study using the established GC-MS/MS method are lower than the previously reported levels in rodents measured by either direct immunoassays (11) or more appropriate indirect immunoassay-based methods using preanalytical extraction (12–20), supporting the notion that the latter assay types overestimate the estradiol levels, especially at low levels. The much lower estradiol levels in rodents compared with humans can at least partly be explained by the fact that humans, but not rodents, express SHBG and secrete substantial amounts of C-19 sex steroid precursors from the adrenal gland (8).
Serum levels of both T and DHT were higher in proestrus than in estrus in female rats. Notably we observed an increase in the levels of the potent androgen DHT in metestrus vs estrus in both mice and rats. Further studies are warranted to determine the physiological role of this DHT increase during metestrus.
Although serum estradiol levels were readily measurable in male mice treated with estradiol, gonadal intact male mice had estradiol levels below the detection limit (<0.3 pg/mL) of our very sensitive assay, suggesting that male mice have extremely low circulating estradiol levels. In addition, this highlights the putative importance of local aromatization of androgens into estrogens in the target tissues because the physiological significance of estrogenic effects in several tissues in male rodents is well recognized (26–29). Future studies analyzing the tissue estradiol levels in different sex steroid-responsive organs in male rodents are clearly warranted. We expect that the estradiol levels, produced by the local aromatization of androgens, in certain tissues might be higher than the circulating estradiol levels in male mice.
We also used the established GC-MS/MS method to evaluate the impact of gonadectomy on circulating sex hormones levels in mice. As expected, but not previously reliably shown in mice, levels of estradiol and estrone were significantly reduced after ovx, reaching levels below the detection limit of the GC-MS/MS assay. Thus, this assay can clearly distinguish between serum estrogens in gonadal intact and ovx female mice. In addition, ovx substantially reduced serum levels of T and progesterone, demonstrating that a major part of the circulating levels of these hormones are secreted by the ovaries in female mice. However, ovx mice still had moderate levels of progesterone and low but measurable levels of T and DHT, suggesting that the murine adrenal gland, at least after ovx, has the capacity to contribute to circulating levels of progesterone, T, and DHT. Adrenocortical cells in the adult mouse normally lack cytochrome P450 17α-hydroxylase/C17-C20 lyase, a dual-function enzyme required for the synthesis of cortisol and androgen precursors. Consequently, corticosterone is the principal glucocorticoid secreted by the mouse adrenal cortex, and under normal conditions, androgenic steroids are not produced in this tissue (8, 30, 31). Because progesterone is a precursor of corticosterone, it is not surprising that the adrenal gland contributes to circulating progesterone in mice (8). In mice, gonadectomy has been reported to induce expression of cytochrome P450 17α-hydroxylase/C17-C20 lyase and thereby androgen synthesis in the adrenal cortex (30, 32–34), possibly explaining the low but measurable levels of T and DHT in ovx female mice in the present study. The potential role of the adrenals as a contributing endocrine gland to circulating T and DHT in female rodent should be further evaluated by analyzing the tissue-specific sex steroid profile of the adrenal gland in gonadal intact and ovx rodents.
As expected, the serum levels of progesterone were lower in male gonadal intact mice compared with random cycling female mice. Notably, orx increased serum progesterone levels in male mice, reaching very similar levels as found in ovx female mice, suggesting that the adrenal gland is a major contributor to circulating progesterone in male mice and that a testicular factor inhibits adrenal progesterone secretion in gonadal intact male mice.
It is well established that the phenotype of female estrogen receptor (ER)-α knockout mice is confounded by a disrupted negative feedback on the hypothalamic-pituitary axis. This was demonstrated by increased serum levels of estradiol and T, which were measured by conventional immunoassays in these mice (22–24). In this study, we can confirm these high levels of serum estradiol and T in female mice with inactiviation of ERα as measured by MS. In addition, these findings can be extended to estrone and DHT because these sex steroids levels were also elevated in the ERα knockout mice compared with wild-type mice. This demonstrates that ERα is involved in the negative feedback regulation of not only the circulating estradiol and T concentrations but also of estrone and DHT in mice.
The limitation of GC-based methods requiring large sample volumes has been overcome because our method quantifies the different steroids from 250 μL of rodent serum, avoiding the need to pool serum samples. To accurately measure serum estradiol and estrone concentrations in female rodents at the highest sensitivity, 250 μL of serum is required. However, 50 μL of male rodent serum is sufficient to obtain a sex steroid profile because the levels of estradiol and estrone are below the LLOD of the assay, and this volume results in adequate sensitivity for the analysis of serum T, DHT, progesterone, and androstenedione concentrations. Nevertheless, it is a clear limitation that the 250 μL of mouse serum required for analyzing female sex steroid concentrations entails the killing of the mice. Also, because there is no validated reference method available for the measurement of rodent sex hormone concentrations, a limitation of the present study is that such a method comparison using rodent serum was not performed.
An important advantage of our established MS-based method is its multianalyte capability, allowing multiple sex steroids to be quantified from a single sample. In addition, even though the GC-MS/MS method developed is more laborious and time consuming compared with LC-MS/MS assays, its sensitivity is noticeably higher. The high sensitivity of the developed GC-MS/MS method relies on a number of factors. First, the chosen extraction strategy, based on a combination of liquid extraction of steroid hormones from serum to 1-chlorobutane and subsequent cleanup on solid phase extraction columns, generates very clean extracts with low content of interfering components. Furthermore, in our hands, the derivatization of steroid hormones with pentafluoro-groups produces stable derivatives with very favorable ionization properties for subsequent use in negative chemical ionization mode. The pentafluoro-derivatives give high signal to noise ratios and low fragmentation, ie, highly abundant ion pairs for MRM could be found for most transitions. In addition, the ionization efficiency with ammonia as reagent gas was higher for all analytes compared with methane, and, therefore, ammonia was used in the present method. The signal was reduced for all analytes by more than 80% when methane was used compared with ammonia. The sensitivity, as evaluated by the signal to noise ratio, was improved for estradiol, estrone, T, and progesterone by 40%, 53%, 103%, and 133%, respectively, when ammonia was used instead of methane, whereas no substantial improvement was observed for androstenedione, DHEA, or DHT. The GC separation was also optimized to generate the highest sensitivity: a high temperature column with low bleed and thin film was chosen and was operated with flushback technology to prevent high boiling components to enter the analytical GC column. After the GC gradient, a high temperature bake-out step was added to further clean up the column between injections. This resulted in an efficient separation of analytes and interfering compounds as well as high signal to noise ratios. Lastly, we use a high-end triple quadrupole mass detector with helium quench to remove interfering neutrals, which results in low background and high sensitivity and specificity.
In conclusion, we have established a highly sensitive and specific GC-MS/MS method to assess a comprehensive sex steroid profile in serum in a single run. Our method will provide a valuable tool to characterize the sex steroid metabolism in a variety of sex steroid-related rodent models and allow the analysis of sex steroid concentrations in human samples with low estradiol levels.
Acknowledgments
We thank Annelie Carlsson for her excellent technical assistance.
Current affiliation for E.S.-V.: Reproductive Endocrinology and Metabolism, Department of Physiology and Pharmacology, Karolinska Institute, Stockholm SE-177 71, Sweden.
This work was supported by the Swedish Research Council, the Swedish Foundation for Strategic Research, an Avtal om Läkarutbildning och Forskning research grant from the Sahlgrenska University Hospital, the Lundberg Foundation, the Torsten and Ragnar Söderberg's Foundation, and the Novo Nordisk Foundation.
Disclosure Summary: The authors have nothing to disclose.
Abbreviations
- cps
counts per second
- CV
coefficient of variation
- DHEA
dehydroepiandrosterone
- ER
estrogen receptor
- ERα−/−
ER-α depleted
- GC
gas chromatography
- GC-MS/MS
GC tandem MS
- gx
gonadectomized
- LC-MS/MS
liquid chromatography-MS/MS
- LLOD
lower limit of detection
- LLOQ
lower limit of quantification
- MRM
multiple reaction monitoring
- MS
mass spectrometry
- orx
orchidectomy
- ovx
ovariectomy.
References
Author notes
M.E.N. and L.V., and H.R. and C.O. contributed equally to this work.