-
PDF
- Split View
-
Views
-
Cite
Cite
Subhadra Banerjee, Partha P. Banerjee, Barry R. Zirkin, Terry R. Brown, Regional Expression of Transforming Growth Factor-α in Rat Ventral Prostate during Postnatal Development, after Androgen Ablation, and after Androgen Replacement, Endocrinology, Volume 139, Issue 6, 1 June 1998, Pages 3005–3013, https://doi.org/10.1210/endo.139.6.6060
- Share Icon Share
Abstract
The prostate is a highly heterogeneous organ, composed of different types of epithelial and stromal cells organized regionally along the ductal network. Although androgen-stimulated growth and maintenance of the prostate gland primarily involve epithelial cells, it is unclear whether all epithelial cells are androgen dependent. Moreover, the actions of androgens may not be direct; a number of polypeptide growth factors, including transforming growth factor-α (TGFα), are postulated to mediate androgen action in the rat prostate. In this investigation, using an immunohistochemical technique, we examined the cellular and regional expression of TGFα in the rat ventral prostate during postnatal development to adulthood. TGFα-immunopositive cells were located throughout the ductal epithelium from postnatal days 5–20. By day 45 and thereafter, regional variation in TGFα expression became apparent; epithelial cells in the proximal segment exhibited intense staining, whereas those in the distal segment exhibited negligible staining. These observations were coincident with increased serum testosterone concentrations at puberty. To understand the role of androgen in the expression of TGFα in the epithelial cells of the distal and proximal segments of the adult rat ventral prostate, androgen was withdrawn by castration, and testosterone subsequently was administered. Androgen receptor protein expression decreased after castration and reappeared after androgen replacement in both the distal and proximal segments. TGFα staining was negligible in epithelial cells of the distal segment of intact adult rats, became prominent by 7 days after castration, but then diminished after the administration of testosterone. Western blot analyses revealed the presence of a specific 30-kDa immunoreactive form of TGFα in rat ventral prostate, and its quantity reflected the staining intensities observed in the immunohistochemical studies. These results suggest that TGFα expression is negatively regulated by androgen in epithelial cells of the distal segment. In contrast, staining for TGFα in epithelial cells of the proximal segment did not change with castration or testosterone administration, suggesting that TGFα is not regulated by androgen in this region of the ventral prostate. In summary, TGFα expression is differentially regulated among epithelial cells localized in two different regions of the ventral prostate. We hypothesize that TGFα may function as a survival factor for epithelial cells which, as a consequence of its expression, become androgen independent and thus escape apoptotic cell death after androgen ablation.
THE PROSTATE is a highly heterogeneous cellular organ. In rats, the prostate gland is comprised of four distinct lobes: ventral, dorsal, lateral, and anterior. Each prostatic lobe differs structurally and functionally (1–3), and within a given lobe, the prostatic ducts show substantial regional and cellular heterogeneity represented by several types of epithelial cells (4, 5). Thus, the distal segment of each of the rat prostatic lobes, the segment furthest from the urethra, is lined with columnar epithelial cells, whereas the proximal segment, closest to the urethra, is lined with cuboidal epithelial cells (6). Basal epithelial cells are present in both distal and proximal segments, but the proportion of these cells is much higher in the proximal segment (6).
It is well established that both the growth and maintenance of the prostate gland are androgen-dependent processes (7), but apparently not all epithelial cells are androgen dependent, even though androgen receptors are present in all epithelial cells along the distal-proximal axis in the intact rat (8, 9). In the prostate, androgen receptor expression is under androgenic control (9, 10), with receptor levels decreasing after castration and returning to intact control levels after testosterone replacement (9). We and others demonstrated that withdrawal of androgen from the adult rat by castration induced apoptotic cell death predominantly among epithelial cells in the distal segment, whereas epithelial cells in the proximal segment remained unaffected (11, 12). These results suggested that all prostatic epithelial cells are not equally dependent upon androgen and that there must be some other factor(s) that permits the survival of androgen-independent epithelial cells.
It is widely believed that androgens may not be the direct effectors of androgen action. For example, androgen has no mitogenic effect on normal prostate epithelial cells in culture (13). Rather, a number of soluble growth factors are able to regulate proliferation directly in these cells (13). Moreover, several polypeptide growth factors have been postulated to mediate androgen action in the rat prostate (14–18). Included among these is transforming growth factor-α (TGFα) (18–20), a 50-amino acid peptide that is related to epidermal growth factor (EGF) (21). TGFα and EGF bind to a common EGF receptor to initiate their respective biological activities. The binding affinities of TGFα and EGF for this receptor are similar (21). Although expression of TGFα in the ventral lobe of the rat prostate has been reported in one study (19), another study failed to confirm these findings (20). However, it is clear that TGFα is a growth-promoting agent of LNCaP, DU-145, and PC-3 cells as well as benign human prostatic epithelial cells under tissue culture conditions (22, 23).
TGFα is present in the epithelial cells of rat prostate (19, 20) and responds to changes in androgen levels (18, 24). However, until recently, most structure-function studies, including those examining TGFα, have treated the rodent ventral prostate as a homogeneous tissue in which cells can be analyzed within random sections or in tissue homogenates without regard to their location in the prostatic ductal network (18–20, 25, 26). Although such studies have provided important insight, more recent studies have clearly indicated that there is regional heterogeneity within the ventral lobe of the rodent prostate with respect to cell type, androgen responsiveness, gene regulation, and protein synthesis/secretion (1, 4, 6, 27). Herein, we used immunohistochemistry to examine the types of epithelial cells that express TGFα and the regional localization of these cells within the ducts of the rat ventral prostate. Firstly, we determined the patterns of TGFα immunostaining in the distal and proximal segments throughout postnatal development to correlate epithelial cell proliferation and differentiation with TGFα protein expression. We show that TGFα is expressed in both the distal and proximal segments of the ventral prostate during its early postnatal development, but that in the adult it is expressed predominantly in the proximal segment. The decrease in TGFα expression in the distal segment during development correlated with the increasing concentration of serum testosterone at puberty. Secondly, we investigated whether TGFα is differentially regulated by androgen in the columnar vs. cuboidal epithelial cells of prostatic ducts from distal and proximal segments, respectively. To this end, prostatic ducts from castrated and androgen-replaced rats were examined. Androgen receptors were present in epithelial cell nuclei of both the proximal and distal prostatic ducts of intact and castrate-androgen replaced rats, but disappeared from cells in both segments after castration. TGFα staining became prominent in epithelial cells of the distal segment after androgen withdrawal and disappeared after androgen replacement. In contrast, staining for TGFα in epithelial cells of the proximal segment did not change with castration or testosterone administration.
Materials and Methods
Animals
Male and timed pregnant female Sprague-Dawley rats were purchased from Charles River Laboratories (Wilmington, MA). All rats were housed in a temperature-controlled room (22 C) and allowed access to food and water ad libitum. Male rats of 5, 10, 20, and 45 days of age were used for developmental studies. For androgen ablation and replacement studies, adult (90-day-old) male rats were castrated via the abdominal route under metofane anesthesia. Epididymides were removed along with the testes. Some animals were killed 7 days after castration. Others were castrated and subsequently, 7 days later, were administered physiological levels of testosterone by implanting 2.5-cm testosterone-filled SILASTIC brand capsules (Dow Corning, Midland, MI) sc for an additional 7 days. All experimental protocols were approved by the animal care and use committee of The Johns Hopkins University School of Hygiene and Public Health.
Serum testosterone concentration
Rats were killed, and trunk blood was collected and allowed to clot for 2 h at room temperature. The serum was separated by centrifugation and stored frozen (−20 C) until assayed. Serum aliquots of 100 μl were extracted twice with 4 ml anhydrous ethyl ether, and the combined extracts were taken to dryness under nitrogen. The testosterone concentration (nanograms per ml) was determined by RIA (28). The sensitivity of the assay was 0.02 ng/ml.
Dissection of prostatic lobes
The urogenital complex, containing the bladder, seminal vesicles, and prostate, was dissected from the abdominal cavity of each animal and immersed in ice-cold HBSS (Life Technologies, Grand Island, NY), pH 7.4. The ventral prostatic lobe was separated under a dissection microscope. In one portion, the tree-like ducts were physically divided into proximal (arising from the urethra to the first branch point) and distal (from the first branch point to the distal tips) segments from the ventral lobe, fixed in 4% neutral buffered paraformaldehyde for 48 h, and embedded in paraffin. Sections of 4–6 μm were cut, mounted on Vectabond-coated (Vector Laboratories, Burlingame, CA) glass slides, and stored in a desiccated box at 4 C until use. Another portion of the ventral lobe was frozen in liquid nitrogen vapor, embedded in Tissue-Tek OCT compound (Miles, Elkhart, IN), and stored at −70 C. For Western blot analysis, a portion of a whole or microdissected ventral prostate (distal and proximal segments) from intact, castrate, and castrate and testosterone-replaced rats was flash-frozen in liquid nitrogen and stored at −70 C until use.
Immunohistochemical localization of TGFα
Tissue sections were deparaffinized, rehydrated, and digested with 0.0025% pronase (Sigma Chemical Co., St. Louis, MO) for 15 min at 37 C. Nonspecific binding was blocked by incubation of sections with 10% normal goat serum in PBS for 30 min at room temperature. Mouse antihuman TGFα monoclonal antibody (Ab-2, Oncogene Science, Uniondale, NY) was used, which cross-reacts with the mature form (5.6 kDa) of rat TGFα (29). The antibody was diluted (2 μg/ml) in 0.2% crystalline grade BSA in PBS, and slides were incubated in a humidified chamber at 4 C for 16–18 h. The sections were then incubated with biotinylated goat antirabbit IgG, stained with avidin-biotin-alkaline phosphatase complex (Vector Laboratories) and Vector red alkaline phosphate substrate (Vector Laboratories), and counterstained with 0.5% methyl green. Negative control slides were prepared in an identical manner, except that the primary antibody was replaced with a similar concentration of purified IgG of the same subtype. TGFα localization was observed by brightfield microscopy, and images were recorded on Ektachrome 64T film (Eastman-Kodak, Rochester, NY).
Immunohistochemical localization of androgen receptor
Frozen tissue sections (5–7 μm) were mounted on poly-l-lysine-coated glass slides, fixed immediately in Zamboni’s fixative for 10 min, and washed three times (10 min each time) in PBS, pH 7.4. Nonspecific binding was blocked by incubation of sections with 10% normal goat serum in PBS for 30 min at room temperature. Rabbit polyclonal antibody raised against a synthetic peptide (near the amino-terminus) specific for the androgen receptor (Novocastra Laboratories, Newcastle upon Tyne, UK) was diluted (1:50) in 0.2% crystalline grade BSA in PBS, and slides were incubated in a humidified chamber at 4 C for 16–18 h. The reaction sites were visualized by incubating the tissue sections with biotinylated second antibody, avidin-biotin-peroxidase complex (Vector Laboratories) and diaminobenzidine reagents (Vector Laboratories). Negative control slides were prepared in an identical manner, except that the primary antibody was replaced with similar concentrations of rabbit IgG. The localization of immunoreactive androgen receptor was observed by light microscopy (Zeiss, Oberkochen, Germany), and images were recorded on Kodak Pan film.
Immunoblot analysis
Frozen tissue samples were homogenized in tissue lysis buffer[ 10 mm Tris (pH 7.4), 150 mm NaCl, 1% Triton X-100, 1% deoxycholate, 0.1% SDS, 5 mm EDTA, 1 mm phenylmethylsulfonylfluoride, 1 mm benzamidine, 0.28 U/ml aprotinin, 50 μg/ml leupeptin, and 0.7 μg/ml pepstatin] and clarified by centrifugation at 14,000 × g for 20 min at 4 C. An aliquot of this supernatant was used for the determination of protein content using the Bio-Rad protein assay reagent (Bio-Rad Laboratories, Hercules, CA) (30). The remaining supernatants were mixed (1:1) with 2 × Laemmli buffer [100 mm Tris-HCl (pH 6.8), 200 mm dithiothreitol, 4% SDS, and 20% glycerol], transferred to a boiling water bath for 5 min, rapidly frozen on dry ice, and stored at −70 C until use.
To obtain fluid from ventral prostates of 90-day-old rats, we used our previously described method (31). In brief, the intact ventral lobe was washed in ice-cold Hanks’ Balanced Salt Solution, blotted onto filter paper, nicked in several locations with a sharp blade, placed in a microcentrifuge tube, and spun at 14,000 × g for 20 min to collect the prostatic fluid. The fluid was transferred to a clean tube and stored at −70 C. An aliquot of the fluid was used for the determination of protein content (30). Fifty microliters of 2× Laemmli buffer were added to 50 μl prostatic fluid and immediately transferred to a boiling water bath for 5 min.
Samples to be analyzed were subjected to SDS-PAGE on a 15% acrylamide gel, run under reducing conditions, using the system described by Laemmli (32). After separation, proteins were electrophoretically transferred to Hybond nitrocellulose membrane (Amersham, Arlington Heights, IL) according to the method of Towbin et al. (33). Transfer was accomplished at 500 mA for 2 h at 4 C. The membrane was initially incubated for 1 h with TBS (20 mm Tris-HCl, pH 7.6, and 137 mm NaCl) containing 0.01% Tween-20 and 5% nonfat dry milk to block nonspecific binding and then incubated for an additional 1.5 h in the presence of TGFα antibody (0.4 μg/ml) with frequent agitation at room temperature. The membrane was then washed with TBS-0.01% Tween-20, incubated with horseradish peroxidase-labeled secondary antibody (1:3000 dilution; Amersham) for 1 h at room temperature, and washed with TBS-0.01% Tween-20. Antibody-binding sites were visualized on Hyperfilm (Amersham) by 5-min exposure, using the ECL detection system (Amersham). High (14.3–200 kDa; Oncogene Science) and low (3.5–29 kDa; Integrated Separation Systems, Natick, MA) molecular mass markers were run on each gel.
Results
Serum testosterone concentration
Table 1 shows the serum concentrations of testosterone in Sprague-Dawley rats during postnatal development, after castration, and after testosterone replacement. The serum testosterone concentration in 5-day-old intact rats was 0.19± 0.02 ng/ml, increased to 1.38 ± 0.22 ng/ml by 45 days of age, and attained the adult level of 2.61 ± 0.92 ng/ml by day 90. The serum concentration of testosterone in adult rats was reduced to undetectable levels (<0.02 ng/ml) by 7 days after castration. Seven days after castration, implantation of 2.5-cm testosterone-filled SILASTIC capsules for an additional 7 days elevated the serum testosterone concentration to that in intact adult rats (2.5 ± 0.8 ng/ml).
Serum testosterone concentration in Sprague-Dawley rats during postnatal development, after castration, and after testosterone replacement
Age/treatments . | Serum testosterone (ng/ml) . |
---|---|
Day 5 | 0.19 ± 0.02 |
Day 10 | 0.35 ± 0.03 |
Day 20 | 0.54 ± 0.06 |
Day 45 | 1.38 ± 0.22 |
Day 90 (adult) | 2.61 ± 0.92 |
Castrateda | <0.02 |
Testosterone-replacedb | 2.50 ± 0.80 |
Age/treatments . | Serum testosterone (ng/ml) . |
---|---|
Day 5 | 0.19 ± 0.02 |
Day 10 | 0.35 ± 0.03 |
Day 20 | 0.54 ± 0.06 |
Day 45 | 1.38 ± 0.22 |
Day 90 (adult) | 2.61 ± 0.92 |
Castrateda | <0.02 |
Testosterone-replacedb | 2.50 ± 0.80 |
Ninety-day-old rat castrated for 7 days.
Ninety-day-old rat castrated for 7 days and then implanted with 2.5-cm testosterone-filled SILASTIC capsules for 7 days.
Serum testosterone concentration in Sprague-Dawley rats during postnatal development, after castration, and after testosterone replacement
Age/treatments . | Serum testosterone (ng/ml) . |
---|---|
Day 5 | 0.19 ± 0.02 |
Day 10 | 0.35 ± 0.03 |
Day 20 | 0.54 ± 0.06 |
Day 45 | 1.38 ± 0.22 |
Day 90 (adult) | 2.61 ± 0.92 |
Castrateda | <0.02 |
Testosterone-replacedb | 2.50 ± 0.80 |
Age/treatments . | Serum testosterone (ng/ml) . |
---|---|
Day 5 | 0.19 ± 0.02 |
Day 10 | 0.35 ± 0.03 |
Day 20 | 0.54 ± 0.06 |
Day 45 | 1.38 ± 0.22 |
Day 90 (adult) | 2.61 ± 0.92 |
Castrateda | <0.02 |
Testosterone-replacedb | 2.50 ± 0.80 |
Ninety-day-old rat castrated for 7 days.
Ninety-day-old rat castrated for 7 days and then implanted with 2.5-cm testosterone-filled SILASTIC capsules for 7 days.
Specificity of TGFα antibody
When 100 ng purified EGF or TGFα (Upstate Biotechnology, Lake Placid, NY) were spotted onto a nitrocellulose membrane and probed with the TGFα antibody, only the TGFα was detected (data not shown). To further evaluate the specificity of the TGFα antibody, purified recombinant TGFα and tissue extracts from ventral prostate of intact and castrated rats were analyzed by Western blot. Figure 1A shows that purified recombinant TGFα was detected as a band of 5.6 kDa, whereas a single protein band of approximately 30 kDa was detected in tissue extracts from ventral prostate of intact and castrated rats. When the TGFα antibody was preincubated with 1 μg purified TGFα, the TGFα-immunoreactive bands were undetectable or greatly reduced in intensity (Fig. 1B). Similar results were obtained when the TGFα antibody was preincubated in the presence of 100 μg protein equivalent from ventral prostate tissue extracts of castrated rats (data not shown). Using various amounts of purified recombinant TGFα, we observed that the lower limit for detection of the protein by Western blot was approximately 25–30 ng (data not shown).
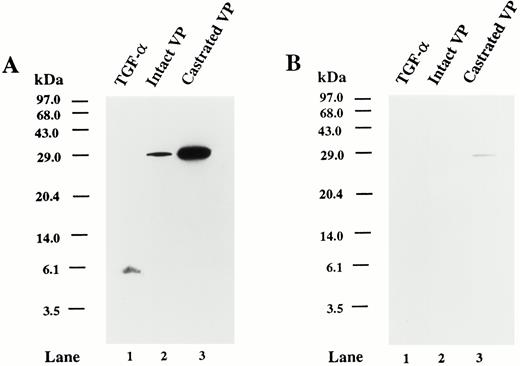
Specificity of TGFα antibody. A, Western blot analysis of the TGFα proteins using recombinant human TGFα and tissue extracts of rat ventral prostate. Autoradiogram shows the immunoreactivity of recombinant human TGFα (50 ng protein; lane 1), ventral prostate extract from 90-day-old intact rat (100 μg protein; lane 2), and ventral prostate extract from 90-day-old rat from 7 days after castration (100 μg protein; lane 3). The blot was probed with TGFα antibody. B, Replicate Western blot probed with TGFα antibody preabsorbed with 1 μg purified recombinant TGFα. The positions of the molecular mass markers (kilodaltons) are indicated.
Immunohistochemical localization of TGFα during postnatal development of the ventral prostate
During the early postnatal period (days 5 and 10), the cellular compositions of the distal and proximal segments were similar. On postnatal day 5, when prostatic ducts contained many epithelial cells but had not yet formed a glandular lumen (Fig. 2A), and on day 10, when the epithelial cells were organized as a tube with a small lumen (Fig. 2B), cells that were immunopositive for TGFα were located throughout the ductal epithelium. On day 20, epithelial cells in the distal segment were columnar (Fig. 2C), whereas the proximal epithelial cells appeared cuboidal (Fig. 2D). The epithelial cells of the distal segment stained for TGFα expression, and an abundance of highly stained secretory material was present within the lumen. The cuboidal epithelial cells of the proximal segment also were intensely stained, but there was no staining within the lumen. By day 45, however, regional specificity of staining became apparent. Columnar epithelial cells in the distal segment exhibited negligible staining for TGFα, although staining of luminal secretions remained intensely positive (Fig. 2E). In contrast, the cuboidal epithelial cells in the proximal segment continued to exhibit intense staining for TGFα (Fig. 2F). Throughout the period of postnatal development until day 45, stromal cells did not stain for TGFα.
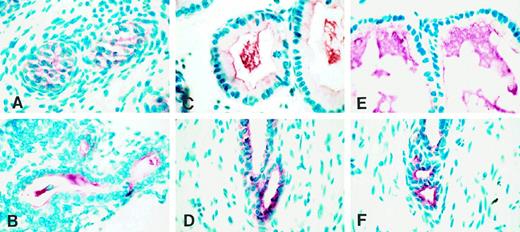
Immunohistochemical localization of TGFα in paraformaldehyde-fixed rat ventral prostate during postnatal development at different ages: day 5 (A), day 10 (B), day 20 (C, distal segment; D, proximal segment), and day 45 (E, distal segment; F, proximal segment). Red color indicates TGFα staining, and blue stain indicates nuclear staining using methyl green.
Immunohistochemical localization of androgen receptor in the ventral prostate of adult intact, castrate, and castrate plus testosterone-replaced rats
Because one goal was to investigate the role of androgen in the expression of TGFα in the epithelial cells of distal and proximal segments of adult rat ventral prostate, we examined androgen receptor protein expression in intact, castrate, and castrate plus androgen-replaced rats. In the intact adult rat, epithelial cells of the distal and proximal segments showed intense nuclear staining for androgen receptor (Fig. 3, A and B). In both segments, some stromal cells were also androgen receptor positive (Fig. 3, A and B). As expected, nuclear androgen receptor staining intensity decreased dramatically in epithelial and stromal cells in both distal and proximal segments 7 days after castration (Fig. 3, C and D). However, 7 days after testosterone administration to castrated rats, nuclear androgen receptor staining reappeared in the columnar epithelial cells of the distal segment (Fig. 3E) and cuboidal epithelial cells of the proximal segment (Fig. 3F). At this time, androgen receptor immunoreactivity was evident again in some stromal cells in both the distal and proximal segments (Fig. 3, E and F).
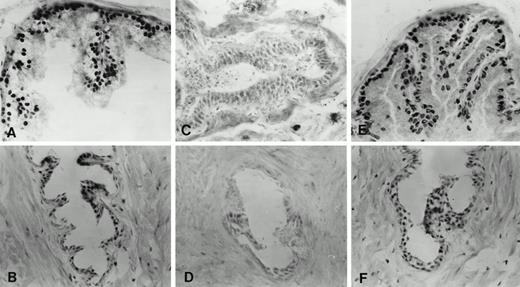
Immunohistochemical localization of androgen receptor in frozen sections of ventral prostate of adult intact (90-day-old), castrate, and castrate plus testosterone-replaced rats. A and B, Distal and proximal segments, respectively, of intact rat. C and D, Distal and proximal segments, respectively, 7 days after castration. E and F, Distal and proximal segments, respectively, after castration for 7 days followed by testosterone replacement (2.5-cm testosterone-filled capsule) for an additional 7 days.
Immunohistochemical localization of TGFα in the ventral prostate of adult intact, castrate, and castrate plus testosterone-replaced rats
The adult architecture of the ventral prostate was established by 90 days of age. At this time, the regional differences in TGFα staining seen on day 45 became more striking. Thus, the distal segment contained columnar epithelial cells, which, as on day 45, exhibited little TGFα staining (Fig. 4A). In a few of these cells, TGFα was localized at the supranuclear region, but unlike the situation on day 45, the luminal contents stained variably for TGFα. In contrast, cuboidal epithelial cells in the proximal segment stained intensely (Fig. 4B).
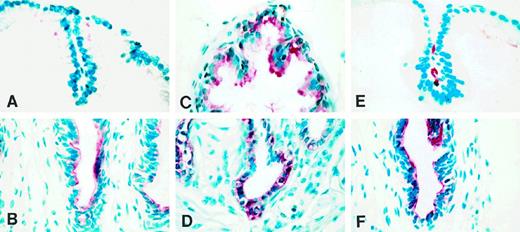
Immunohistochemical localization of TGFα in paraformaldehyde-fixed ventral prostate of adult intact (90-day-old), castrate, and castrate plus testosterone-replaced rats. A and B, Distal and proximal segments, respectively, of intact rat. C and D, Distal and proximal segments, respectively, 7 days after castration. E and F, Distal and proximal segments, respectively, after castration for 7 days followed by testosterone replacement (2.5-cm testosterone-filled capsule) for an additional 7 days.
The regulation of TGFα expression in rat ventral prostate by androgen was examined in 90-day-old rats. In contrast to the situation in the intact adult, in which epithelial cells of the distal segment were negligibly stained for TGFα, 7 days after castration these cells expressed high levels of TGFα (Fig. 4C), but appeared cuboidal rather than tall columnar as in intact animals. Cells in the proximal segment were positive, as was the case before castration (Fig. 4D). Seven days after testosterone administration to castrated rats, columnar epithelial cells reappeared in the distal segment (Fig. 4E), coincident with which TGFα staining disappeared from the cells of this segment. In contrast, cuboidal epithelial cells in the proximal segment continued to express TGFα (Fig. 4F). Stromal cells were devoid of staining throughout the experimental manipulations.
Western blots of immunoreactive TGFα in the ventral prostate of adult intact, castrate, and castrate plus testosterone-replaced rats
As we have seen regional differences in TGFα immunohistochemical localization in rat ventral prostate and its regulation by androgen, we also employed Western blots to semiquantitatively determine the levels of TGFα expression and to identify the immunoreactive protein(s) present in the microdissected distal and proximal segments of the ventral prostate of adult intact, castrate, and castrate plus testosterone-replaced rats. A single immunoreactive protein of approximately 30 kDa was detected in all tissue extracts prepared from the ventral prostate (Fig. 5). The level of TGFα was lower in the distal segment than in the proximal segment of intact rats (Fig. 5A). Seven days after castration, TGFα levels increased in the distal segment, but after testosterone replacement for 7 days, TGFα levels in the distal segment declined to the intact control level. By contrast, TGFα levels in the proximal segment did not change after castration or the subsequent administration of androgen.
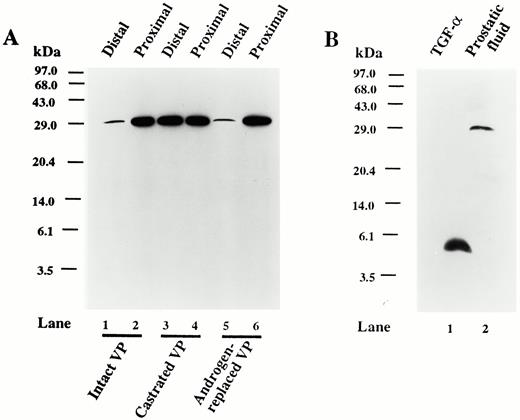
A, Western blot analysis of the TGFα proteins from ventral prostate tissue extracts from distal and proximal segments of 90-day-old intact (lanes 1 and 2), 7-day castrated (lanes 3 and 4), and 7-day castrated plus 7-day testosterone-replaced (2.5-cm testosterone-filled capsule; lanes 5 and 6) rats. Equal amounts (100μ g) of protein were loaded in each lane. B, Western blot analysis of purified recombinant TGFα (100 ng protein; lane 1) and fluid from the ventral prostate of an intact 90-day-old rat (100 μg protein; lane 2). The positions of the molecular mass markers (kilodaltons) are indicated.
Because the TGFα antibody reacted with the secretory products in the prostatic lumen of several tissue sections, shown in Figs. 2 and 4, we were interested to determine whether TGFα was secreted into the prostatic lumen, and if so, whether the immunoreactive protein was the same as that seen in tissue extracts. Figure 5B shows that prostatic fluid also contains a 30-kDa TGFα protein, and therefore, the immunoreactive protein observed in the lumen of prostatic sections by immunohistochemistry is consistent with the protein expressed within epithelial cells.
Discussion
The present study shows that TGFα is expressed throughout the development of the ventral prostate, from postnatal day 5 to adulthood. This result corroborates the observation by Cunha and his associates that TGFα messenger RNA is present in the neonatal rat prostate (34). However, our results differ from the previous study of Taylor and Ramsdell (19), in which a sheep polyclonal antiserum directed against the mature TGFα 50-amino acid sequence was used for the localization of TGFα in rat ventral prostate during its postnatal development (days 8–42). They did not detect TGFα immunoreactivity in the ventral prostate of 10- and 21-day-old rat pups, although it was present in prostatic epithelial cells at 28 and 35 days of age (19). In contrast to this previous study (19), we found TGFα immunoreactivity in the epithelial cells throughout postnatal development (days 5–45) in both distal and proximal segments using a mouse monoclonal antiserum specific for the mature form of TGFα (29).
During postnatal development, the rat ventral prostate attains a highly branched, convoluted, tree-like structure, with regional differences in cellular architecture along the prostatic ducts. The regional cellular heterogeneity cannot be appreciated by examination of random sections. The dissection method we used enabled us to visualize both the distal and proximal segments. In this regard, the regional variability in TGFα protein expression in the prostatic ducts was particularly noteworthy; with developmental age, staining intensity became more prominent in the proximal, but disappeared from the distal, segment. It seems likely that the contradictory results of previous studies, reporting either the presence of TGFα in epithelial cells of the adult ventral prostate (19) or its absence (20), may have resulted from the examination of different regions of the ventral lobe.
Regional variation in TGFα staining became strikingly apparent in the adult rat ventral prostate. We found that TGFα protein expression appeared almost exclusively in the cuboidal epithelial cells of the proximal segment, with very little in the columnar epithelial cells of the distal segment. In contrast, both cuboidal and columnar epithelial cells in these segments stained positively for nuclear androgen receptor. Therefore, we asked whether TGFα was regulated differently by androgen in epithelial cells of the proximal and distal segments despite the apparent ubiquitous expression of androgen receptor? In the distal segment, castration resulted in high levels of TGFα expression in the epithelial cells, and the administration of physiological levels of testosterone reduced this expression. In striking contrast, androgen levels had no effect on the prominent TGFα expression in the proximal segment. These results suggest that TGFα is negatively regulated by androgen in epithelial cells of the distal segment, which is consistent with a recent study that reported the induction of TGFα messenger RNA in the ventral lobe after castration (18), but in contrast, TGFα expression in epithelial cells of the proximal segment appears not to be regulated by androgen. It is apparent that different regulatory mechanisms determine TGFα expression that are cell type and/or segment specific.
The differences in TGFα expression in the distal and proximal segments suggest region-specific roles for TGFα in the adult ventral prostate. A definitive function for TGFα in the prostate has not yet been established, although it is postulated to play a role in autocrine growth regulation of epithelial cells. For example, overexpression of TGFα in a number of organs, including the anterior lobe (coagulating gland) of the prostate, was shown to result in tissue enlargement, primarily due to epithelial cell hyperplasia (35, 36), suggesting that TGFα acts as a mitogen. In addition, Cunha and his colleagues have shown a profound inhibition of androgen-dependent prostatic ductal morphogenesis and growth in serum-free organ cultures of neonatal rat prostates using a neutralizing antibody to TGFα (34). This would predict that TGFα should be expressed primarily in the distal segment where cell proliferation is most prominent, but, as we observed, it is not.
How, then, does the proposed mitogenic function of TGFα relate to its high level of expression in the proximal segment? We propose that TGFα may have the ability to protect epithelial cells from death, in addition to a role in cell proliferation. In fact, it has been shown in cell culture that TGFβ1-induced cell death can be counterbalanced by mitogenic factors such as basic fibroblast growth factor and EGF (37, 38). Perhaps this is a role that TGFα plays in the proximal segment of the ventral prostate. Indeed, our unpublished results show that the cell survival factor, Bcl-2, is expressed predominantly in the proximal segment, but staining intensity increases in the distal segment after castration. We suggest that epithelial cells in the proximal segment may express several factors, including TGFα, to aid in their survival, as these cells are required for tissue renewal.
In fact, we found that TGFα protein expression appeared in all surviving epithelial cells of the distal segment by 7 days postcastration. Our previous results demonstrated that cell death (apoptosis) was maximal by 2–3 days after castration, resulting in a 60% loss of cells by 1 week (12). Thus, the absence of TGFα expression in the epithelial cells of the distal segment before seven days postcastration (data not shown) is correlated with the loss of cells, whereas TGFα expression after 7 days postcastration is correlated with the survival of the remaining epithelial cells, now of a cuboidal type. This interpretation is consistent with observations in the proximal segment in which all epithelial cells are TGFα positive, and very little cell death occurs in response to androgen withdrawal (12). Taken together, these observations make it reasonable to suggest that TGFα may protect epithelial cells from death.
Similar to our immunohistochemical results, our Western blot analysis demonstrated a region-specific increase in the level of TGFα after castration and a decrease in TGFα level after androgen replacement to castrated rats. However, the immunoreactive protein detected in the prostatic tissue extracts had a molecular mass of approximately 30 kDa and was not the 5.6-kDa protein representing the mature form of TGFα, although the antibody we used reacts with both mature and precursor forms of TGFα. Because we estimated the sensitivity of our TGFα Western blot system to be in the range of 25–30 ng, only small amounts of the mature form of TGFα must be present in rat ventral prostate. However, higher molecular mass forms of TGFα are not unusual and have been reported previously. For example, higher molecular mass forms comprised the predominant TGFα activity extracted from lysates or supernatants of human rhabdomyosarcoma (18 kDa) (39), melanoma (22.5 kDa) (40), mammary carcinoma cells (30 and 68 kDa) (41), human platelets (24 kDa) (42), the urine of cancer patients (30 kDa) (43), human prostate carcinoma cells (18 kDa) (23), rat hepatocellular carcinoma cells (18–21 kDa) (44), and rat submandibular gland (15–20 kDa) (45). Several of these higher molecular mass forms of TGFα have been tested and found to have biological activity (41, 44, 46–48). Therefore, it is reasonable to propose that the 30-kDa TGFα of rat ventral prostate would be biologically active. Moreover, similar to many human carcinomas, normal rat ventral prostate also secretes a higher molecular mass form of TGFα in the prostatic fluid. Because there are no reports that show the mature form (5.6 kDa) or any other molecular mass forms of TGFα in rat prostate or in prostatic fluid to be biologically active, it will be interesting to determine whether further processing of the 30-kDa TGFα is required for its biological activity. It will be of interest to investigate whether the 30-kDa form of TGFα is biologically equipotent and/or resistant to proteolytic degradation compared with mature TGFα, and whether lobe-specific or age-dependent changes occur in the processing of TGFα to differentially affect its activity in different regions of the prostate.
Our understanding of the regional cellular heterogeneity in the prostatic ductal system is more clear than before, as we now recognize that in the same ductal system in which all prostatic epithelial cells are exposed to the same level of circulating androgen, all cells (columnar vs. cuboidal) are not responding in the same manner. These observations suggest that the effect of androgen on the epithelial cells of prostatic ducts may vary profoundly based on their regional localization. Whether similar regional heterogeneity in stromal cells exists in the prostatic ducts is not known at this point and will be the subject of future investigation.
In summary, our results show for the first time that regional heterogeneity in the expression of TGFα in epithelial cells exists in the rat ventral prostate. Although androgen receptors are present in epithelial cells of the proximal segment, these cells appear to be minimally androgen dependent based upon their survival after androgen ablation. We speculate that TGFα expression may be indicative of epithelial cell androgen independence. Our findings suggest a possible role for TGFα in the androgen-independent growth of prostatic cells in diseases such as cancer and benign prostatic hyperplasia, even as serum testosterone levels decrease with age and androgen ablation therapy is implemented.
References
Author notes
This work was supported by NIH Grant P01-AG08321 and Population Center Grant P30-HD-06268.