-
PDF
- Split View
-
Views
-
Cite
Cite
Eduardo T. Mezquida, Steven J. Slater, Craig W. Benkman, Sage-Grouse and Indirect Interactions: Potential Implications of Coyote Control on Sage-Grouse Populations, The Condor: Ornithological Applications, Volume 108, Issue 4, 1 November 2006, Pages 747–759, https://doi.org/10.1093/condor/108.4.747
- Share Icon Share
Abstract
Coyotes (Canis latrans) are lethally controlled throughout the range of Greater Sage-Grouse (Centrocercus urophasianus) and it has been suggested that such control may benefit sage-grouse. However, the perceived benefits of control are based on the direct effects of coyotes on sage-grouse and largely ignore potential indirect interactions. Here, we summarize some of the evidence for direct effects in a simplified food web including coyotes and sage-grouse. There is very little evidence to suggest that coyotes have much of a direct negative effect on sage-grouse, but there is considerable evidence supporting direct interactions that would lead to positive indirect effects between coyotes and sage-grouse. The three likely forms of positive indirect effects arise because coyotes reduce the potential negative effects resulting from mesopredator release and apparent and exploitative competition. Mesopredator release would adversely affect sage-grouse if a decrease in coyotes allowed an increase in foxes (especially Vulpes vulpes), badgers (Taxidea taxus), and Common Ravens (Corvus corax), mesopredators that prey on sage-grouse eggs and young. A decrease in coyotes is likely to allow jackrabbits (Lepus spp.) to increase, which would cause sage-grouse to suffer from apparent competition if Golden Eagles (Aquila chrysaetos), which are perhaps the most important predator of adult sage-grouse, then increase in response to the increase in jackrabbits. This increase in jackrabbits may also depress the availability of sagebrush (Artemisia spp.) and forbs, leading to an increase in exploitative competition with sage-grouse. For these reasons, we argue that intense and extended lethal coyote control is likely detrimental to sage-grouse conservation.
Centrocercus urophasianus e Interacciones Indirectas: Potenciales Implicaciones del Control de Canis Latrans en las Poblaciones de Centrocercus urophasianus
Los coyotes (Canis latrans) son eliminados como medio de control en todo el rango de distribución de Centrocercus urophasianus y se ha sugerido que este control puede beneficiar a C. urophasianus. Sin embargo, los beneficios observados de este control se basan en los efectos directos de C. latrans en C. urophasianus sin tener en cuenta las potenciales interacciones indirectas. En este estudio, resumimos parte de la evidencia de los efectos directos en una cadena alimenticia simplificada que incluye a C. latrans y a C. urophasianus. La evidencia para afirmar que C. latrans tiene un importante efecto negativo directo sobre C. urophasianus es escasa, pero existe una considerable evidencia que da soporte a las interacciones directas, que llevarían a efectos positivos indirectos entre C. latrans y C. urophasianus. Las tres probables formas de efectos indirectos positivos se producen porque C. latrans reduce los potenciales efectos negativos que surgen de la liberación de los mesodepredadores y de la competencia aparente y por explotación. La liberación de los mesodepredadores afectaría negativamente a C. urophasianus si la disminución de C. latrans llevara a un incremento de Vulpes vulpes, Taxidea taxus y Corvus corax, que depredan los huevos y los jóvenes de C. urophasianus. Una disminución en el número de C. latrans probablemente daría lugar a un incremento de Lepus spp., lo cual causaría que C. urophasianus experimentara competencia aparente si Aquila chrysaetos, que posiblemente es el depredador más importante de los adultos de C. urophasianus, incrementara en respuesta al aumento de Lepus spp. Este incremento de Lepus spp. también podría reducir la disponibilidad de Artemisia spp. y herbáceas, dando lugar a un aumento de la competencia por explotación con C. urophasianus. Por estas razones, argumentamos que un control letal de C. latrans intenso y prolongado es probablemente perjudicial para la conservación de C. urophasianus.
Introduction
Predators often have important direct effects on prey populations as well as dramatic indirect effects that reverberate throughout the entire community (Terborgh et al. 2001, Roemer et al. 2002, Springer et al. 2003, Ripple and Beschta 2004, Knight et al. 2005). Consequently, the importance of predators to the management of ecosystems is receiving increased attention (Estes 1996, Terborgh et al. 1999, Berger et al. 2001, Carroll et al. 2001, Hebblewhite et al. 2005), especially because top predators are particularly vulnerable to extinction (Terborgh et al. 2001) yet have often been the subject of lethal control and eradication efforts. This vulnerability and persecution has enabled investigators to take advantage of recent reintroductions, recolonizations, extinctions, and predator control programs to gain insight into complex relationships in ecological communities and the important direct and indirect effects of top predators (Paine et al. 1990, Henke and Bryant 1999, Berger et al. 2001, Terborgh et al. 2001, Hebblewhite et al. 2005). Nevertheless, in many cases only the direct effects of species interactions (i.e., who eats whom) have been investigated and emphasized. Such a focus is evident in the vast literature on Greater Sage-Grouse (Centrocercus urophasianus), a species of conservation concern, where relatively little mention is made of the potential consequences of indirect interactions. However, given the increasingly recognized importance of indirect interactions in both aquatic and terrestrial systems (Holt and Lawton 1994, Wootton 1994, Menge 1995, Schmitz et al. 2000, Shurin et al. 2002, Schmitz 2003, Werner and Peacor 2003), we believe it is critical to consider potential indirect effects on Greater Sage-Grouse.
In this essay, we outline a simplified web of trophic interactions in sagebrush habitats, discuss the evidence supporting direct and indirect effects in these communities, and argue that intense and extended lethal coyote (Canis latrans) control is likely detrimental to Greater Sage-Grouse conservation because of positive indirect effects between these two species. Although we focus on Greater Sage-Grouse, the same conclusions are likely to apply to the closely related and even more imperiled Gunnison Sage-Grouse (C. minimus) and possibly also to other grouse species. This is not to lessen the importance of other factors (e.g., habitat degradation, fragmentation, and loss [Knick et al. 2003] or disease [Naugle et al. 2004]) contributing to the decline of Greater Sage-Grouse, but to highlight a series of complex interactions that is likely to further contribute to this species' decline.
The three indirect interactions that we hypothesize are mesopredator release, apparent competition, and exploitative competition. In particular, we argue that mesopredator release would occur if a decrease in coyotes allowed foxes (especially red foxes [Vulpes vulpes]), badgers (Taxidea taxus), and Common Ravens (Corvus corax), which prey on Greater Sage-Grouse eggs and young, to increase, causing a decline in Greater Sage-Grouse. If a decrease in coyotes also allowed jackrabbits (Lepus spp.) to increase, then Greater Sage-Grouse could suffer from apparent competition (i.e., negative effects of increased predation that arise if two species share a common predator) if Golden Eagles (Aquila chrysaetos), which are perhaps the most important predator of adult Greater Sage-Grouse, increase in response to the increase in jackrabbits. This increase in jackrabbits may also depress the availability of sagebrush (Artemisia spp.) and forbs, leading to an increase in exploitative competition with Greater Sage-Grouse.
Sage-grouse Natural History and Coyote Control
The Greater Sage-Grouse (hereafter, sage-grouse) is the largest grouse in North America. Sage-Grouse are closely associated with sagebrush, thus their distribution is restricted to sagebrush ecosystems of western North America (Schroeder et al. 1999, Connelly et al. 2004). Sage-Grouse feed almost exclusively on sagebrush (mainly leaves) during late autumn, winter, and early spring, with insects and forbs especially important for juveniles during summer (Schroeder et al. 1999). Nests are usually placed under the cover of sagebrush, and sagebrush is also critical for the winter survival of sage-grouse (Schroeder et al. 1999). Because sagebrush ecosystems in western North America have been drastically reduced since settlement by Europeans more than a century ago (Knick et al. 2003, Connelly et al. 2004), it is not surprising that sage-grouse populations have been declining since the early 1900s, and they currently occupy an estimated 56% of their presettlement (prior to 1800) distribution (Schroeder et al. 2004). Several factors, such as loss of habitat to cultivation, burning, and overgrazing, have been cited as contributing to range reductions and population declines of sage-grouse (Braun 1998, Schroeder et al. 1999). Populations continue to decline despite decades of conservation concern and management efforts (Schroeder et al. 1999, Connelly et al. 2000).
Considerable research effort has focused on different aspects of sage-grouse natural history and on factors potentially detrimental to populations (Connelly et al. 2004). Much of this effort has been devoted to investigating particular problems (e.g., predators, nest predators, and habitat alteration) and their management implications. For example, sage-grouse predators, especially nest predators, have sometimes been claimed to be the major factor limiting sage-grouse populations (Willis et al. 1993, Giesen 1995). However, data quantifying the actual significance of predators are scarce in spite of data on spatial and temporal variation in the predator community. Moreover, lethal predator control is still considered an important management action, even though the importance of different predators on sage-grouse populations is uncertain (Schroeder and Baydack 2001, Connelly et al. 2004). In particular, coyotes are commonly cited as important sage-grouse predators (Willis et al. 1993, Schroeder et al. 1999) and population control of coyotes is currently carried out in different areas and habitats, including sagebrush.
Coyotes have been controlled for protection of livestock, to benefit ungulate or gamebird populations, and, more recently, for conservation of endangered species (Goodrich and Buskirk 1995, Reynolds and Tapper 1996, Phillips and White 2003). The major focus of USDA APHIS Wildlife Services (formerly Animal Damage Control) is the protection of livestock from predators, particularly coyotes (U.S. General Accounting Office 1995). Wildlife Services killed an average of 33 278 coyotes annually between 1998 and 2004 in the six states that could be considered the core of the current sage-grouse distribution (Table 1). Control generally occurs in areas with heavy livestock grazing (U.S. General Accounting Office 1990) and has been estimated to occur on only 11% of western lands (U.S. Fish and Wildlife Service 1978). As a result, the program is not believed to negatively impact overall coyote numbers in the West, but may effectively suppress their numbers in some areas (U.S. Fish and Wildlife Service 1978, U.S. General Accounting Office 1990). Although difficult to quantify, there is likely substantial overlap between sage-grouse habitat and rangelands used for livestock grazing, which are the focus of predator control programs. In addition, the number of coyotes killed by Wildlife Services within the range of the sage-grouse may be small relative to the total number harvested (Table 1). However, reliable estimates of total coyote harvest are difficult to make because of the largely unprotected and unregulated status of coyotes in these states (Table 1). The numbers in Table 1 likely underestimate the total, perhaps by an order of magnitude. For example, the average annual harvest of coyotes in North Dakota was estimated by Wildlife Services at about 4150 individuals between 1998 and 2004, whereas a recent survey of North Dakota hunters and trappers revealed that their total annual harvest (1 July 2004 to 30 June 2005) was approximately 40 000 coyotes (D. Fecske, North Dakota Game and Fish Department, pers. comm.).
Classification of coyotes in the six states encompassing most of the current distribution of Greater Sage-Grouse, and the annual lethal control of coyotes by USDA APHIS Wildlife Services (formerly Animal Damage Control), harvest by hunters and trappers, and total take from these two sources between 1998 and 2004 (data for Montana from Montana Fish, Wildlife and Parks [2005]; data for the other states from USDA APHIS [2005]).
![Classification of coyotes in the six states encompassing most of the current distribution of Greater Sage-Grouse, and the annual lethal control of coyotes by USDA APHIS Wildlife Services (formerly Animal Damage Control), harvest by hunters and trappers, and total take from these two sources between 1998 and 2004 (data for Montana from Montana Fish, Wildlife and Parks [2005]; data for the other states from USDA APHIS [2005]).](https://oup.silverchair-cdn.com/oup/backfile/Content_public/Journal/condor/108/4/10.1093_condor_108.4.747/1/m_i0010-5422-108-4-747-t01.gif?Expires=1748577874&Signature=Wt63lPPf~4wsRm-5DdsmKNP6c9cecI0~t5vFreo7mIAR0jfGb3zrdsWxXnnQzvThBquFZ0Sgta4mY9Ifo5UD0AXn8qIrTUkEs3-rSSf64GSOMyc8~W6uud92G-24kl-T5bHYpojvIbCY6tKSPlL4jgTjOk9DJTh15Tjup8bQo6yElCJGrsSn8Ke6KM27y1pKx3npuUDRvVux7YPgwefODosMjqLhLaUFpY534Lx4rev5VBLEU-6JmUUom~V-qPOrRc~W5B2WPHFw5IVUlIskduvABanyerhy3rWzM1r8j4wJY1i~3F1cCYhIc9eD7iJzq0Q4cucGp-cPP50737rmpQ__&Key-Pair-Id=APKAIE5G5CRDK6RD3PGA)
Classification of coyotes in the six states encompassing most of the current distribution of Greater Sage-Grouse, and the annual lethal control of coyotes by USDA APHIS Wildlife Services (formerly Animal Damage Control), harvest by hunters and trappers, and total take from these two sources between 1998 and 2004 (data for Montana from Montana Fish, Wildlife and Parks [2005]; data for the other states from USDA APHIS [2005]).
![Classification of coyotes in the six states encompassing most of the current distribution of Greater Sage-Grouse, and the annual lethal control of coyotes by USDA APHIS Wildlife Services (formerly Animal Damage Control), harvest by hunters and trappers, and total take from these two sources between 1998 and 2004 (data for Montana from Montana Fish, Wildlife and Parks [2005]; data for the other states from USDA APHIS [2005]).](https://oup.silverchair-cdn.com/oup/backfile/Content_public/Journal/condor/108/4/10.1093_condor_108.4.747/1/m_i0010-5422-108-4-747-t01.gif?Expires=1748577874&Signature=Wt63lPPf~4wsRm-5DdsmKNP6c9cecI0~t5vFreo7mIAR0jfGb3zrdsWxXnnQzvThBquFZ0Sgta4mY9Ifo5UD0AXn8qIrTUkEs3-rSSf64GSOMyc8~W6uud92G-24kl-T5bHYpojvIbCY6tKSPlL4jgTjOk9DJTh15Tjup8bQo6yElCJGrsSn8Ke6KM27y1pKx3npuUDRvVux7YPgwefODosMjqLhLaUFpY534Lx4rev5VBLEU-6JmUUom~V-qPOrRc~W5B2WPHFw5IVUlIskduvABanyerhy3rWzM1r8j4wJY1i~3F1cCYhIc9eD7iJzq0Q4cucGp-cPP50737rmpQ__&Key-Pair-Id=APKAIE5G5CRDK6RD3PGA)
Nevertheless, the effects of the selective culling of coyotes on sage-grouse populations are largely unknown (Slater 2003). If only direct interactions between coyotes and sage-grouse were important, then coyote control would at worst have no impact on sage-grouse and would potentially enhance sage-grouse populations. However, given the growing evidence from other systems indicating that top predators, including coyotes, may benefit species in lower trophic levels by directly or indirectly controlling their predators or competitors (Vickery et al. 1992, Ball et al. 1995, Palomares et al. 1995, Sovada et al. 1995, Crooks and Soulé 1999), coyote control may inadvertently contribute to sage-grouse declines. Consequently, it is critical that we consider the possibility of indirect effects of coyote control on sage-grouse populations.
Direct Trophic Interactions in Sagebrush Habitats
Using published information, including studies conducted outside sagebrush steppe habitat (Table 2), we delineated a food web of direct trophic (mostly consumer–prey) interactions in sagebrush habitats occupied by sage-grouse (Fig. 1). Here, we establish the basic consumer–prey interactions.
Summary of data used to infer interactions between various consumers and their prey or competitors that were used to delineate a food web of trophic interactions in sagebrush habitats occupied by sage-grouse (Fig. 1). Effects of the consumers on the other species were always negative unless noted as positive (+) or neutral (0). We also note whether these effects were determined to be the result of competition (c), interspecific killing (k), predation or herbivory (p), or nest predation (ne), and if this altered density, distribution, or abundance (a). General categories for different habitat types include: ch = chaparral; g = alpine meadow, grassland, grass meadow, prairie, or shortgrass prairie; ow = oak woodland; pw = coniferous woodland or boreal forest; sb = sagebrush; ss = sage-scrub, semi-desert scrub, shrubland, shrubsteppe, or subalpine shrub. We also note whether data were based on observations (obs) or experiments (exp), and the state (if in the United States) or country where the studies were conducted.
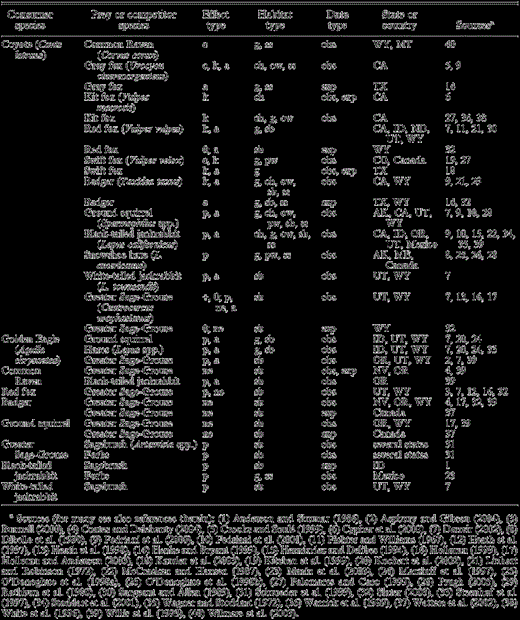
Summary of data used to infer interactions between various consumers and their prey or competitors that were used to delineate a food web of trophic interactions in sagebrush habitats occupied by sage-grouse (Fig. 1). Effects of the consumers on the other species were always negative unless noted as positive (+) or neutral (0). We also note whether these effects were determined to be the result of competition (c), interspecific killing (k), predation or herbivory (p), or nest predation (ne), and if this altered density, distribution, or abundance (a). General categories for different habitat types include: ch = chaparral; g = alpine meadow, grassland, grass meadow, prairie, or shortgrass prairie; ow = oak woodland; pw = coniferous woodland or boreal forest; sb = sagebrush; ss = sage-scrub, semi-desert scrub, shrubland, shrubsteppe, or subalpine shrub. We also note whether data were based on observations (obs) or experiments (exp), and the state (if in the United States) or country where the studies were conducted.
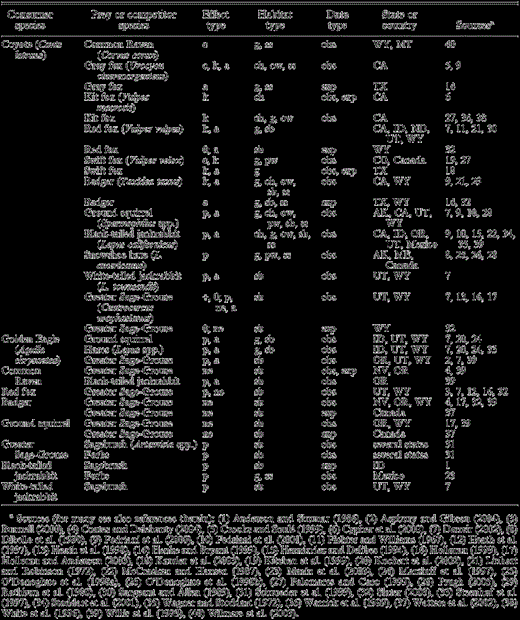
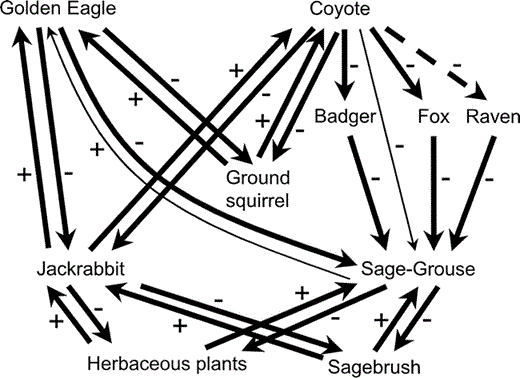
Simplified diagram of trophic interactions in sagebrush habitats occupied by sage-grouse.
Solid lines represent direct interactions and the dashed line represents an indirect interaction. Other indirect interactions are discussed in the text. Plus and minus symbols represent whether the outcome of the interaction for the species at the head of the arrow is beneficial or detrimental, respectively. Thinner arrows indicate apparently weak direct interactions.
A number of studies demonstrate that jackrabbits and rodents (including ground squirrels [Spermophilus spp.]) are the main prey of coyotes in sagebrush and other habitat types (Table 2). In an experimental study testing the effect of coyotes on jackrabbit populations, depressing coyote population densities by 50% resulted in an increase in the population density of jackrabbits (Henke and Bryant 1999). The interpretation that predation by coyotes limits prey abundance is strengthened by evidence that most jackrabbit mortality in sagebrush habitats is caused by coyotes, and that such mortality and variation in the abundance of coyotes relative to the abundance of jackrabbits is sufficient to explain much of the variation in jackrabbit abundance over time (Wagner and Stoddart 1972). Coyotes are also usually cited as a predator of sage-grouse adults and nests (Willis et al. 1993, Schroeder et al. 1999, Connelly et al. 2004). However, coyotes, and mammals in general, seem to have a relatively small impact on adult sage-grouse mortality. For example, Danvir (2002) reported that less than 10% of adult sage-grouse mortality could be attributed to mammals and did not find sage-grouse remains in occasional examinations of coyote scats or den sites. Moreover, coyotes showed little interest in adult sage-grouse while crossing leks and rarely tried to capture them (Danvir 2002). Conversely, mammals are the primary predators of sage-grouse nests, although the relative importance of each mammal species is rarely quantified (Slater 2003). Slater (2003) used indirect evidence (signs of predators and nest and egg remains) to infer predator species at sage-grouse nests and concluded that coyotes were not important nest predators in his study area. Two published studies that identified sage-grouse nest predators using remote-sensing cameras did not record coyotes (Holloran and Anderson 2003, Coates and Delehanty 2004), although the number of unsuccessful nests monitored was too few (n = 7) to draw general conclusions. A study by Willis et al. (1993), that analyzed a long-term data series on coyote abundance and sage-grouse productivity in Oregon, found a significant negative correlation between coyote abundance and the number of chicks per adult sage-grouse. The authors suggested that an increase in coyote abundance following a ban on 1080 poison was one of the main causes of decline in sage-grouse productivity. However, the removal of this broad-acting animal control agent, which caused coyotes to increase, likely also caused Common Ravens (Willis et al. 1993) and other mesopredators (e.g., badgers and foxes), which are better-known nest predators (Table 2), to increase, so the decline in sage-grouse productivity probably reflected a general increase in nest and brood predators.
Foxes, badgers, and ravens appear to be major sage-grouse nest predators (Table 2), although information is scarce on their relative importance. Slater (2003), using indirect evidence, identified some instances of nest predation by red foxes, but the incidence was low, presumably because fox abundance was low during the study. He inferred badgers as the main predator of sage-grouse nests in his study. Both red foxes and badgers were also recorded as nest predators in studies using remote-sensing cameras (Holloran and Anderson 2003, Coates and Delehanty 2004). Red foxes seemed to be responsible for most sage-grouse killed by mammals in another study (Danvir 2002). The negative impact of red foxes on populations of three forest grouse species in Sweden is especially well documented (Lindström et al. 1994). Ravens have also been reported as sage-grouse nest and chick predators (Willis et al. 1993 and references therein). The impact of ravens on sage-grouse nest success can be considerable (Batterson and Morse 1948, Coates and Delehanty 2004) and changes in raven abundance have been suggested as an important factor limiting sage-grouse productivity (Willis et al. 1993, Coates and Delehanty 2004). Ground squirrels were the primary predators in a study using artificial nests (Watters et al. 2002). Although recorded at sage-grouse nests in the study by Holloran and Anderson (2003), ground squirrels were not involved in nest predation and may not be able to penetrate sage-grouse eggs (Coates and Delehanty 2004). Consequently, we do not show a direct interaction between ground squirrels and sage-grouse in Figure 1.
Golden Eagles (Aquila chrysaetos) are likely the most important predators of adult sage-grouse (Patterson 1952, reviewed by Schroeder et al. 1999). Golden Eagles are one of three raptor species in Europe whose diets regularly contain grouse as a major component (Valkama et al. 2005) and have been found to be the main predators of male sage-grouse on leks (Aspbury and Gibson 2004 and references therein). They have also been documented to kill females and juveniles (Willis et al. 1993, Danvir 2002; Fig. 1). Danvir (2002) reported a negative correlation between winter eagle count and sage-grouse population change, which suggests that predation by eagles depresses sage-grouse winter survival. However, jackrabbits and ground squirrels are the main prey items for eagles in various habitats (Table 2) during both the breeding and nonbreeding seasons. Moreover, the abundance of jackrabbits and ground squirrels, but not sage-grouse, has been shown to influence eagle reproduction (Table 2). Thus, there appears to be asymmetry in the relationship between sage-grouse and Golden Eagles. Sage-Grouse may at times be limited by eagle predation, but eagles are unlikely to be limited by the abundance of sage-grouse (Fig. 1). This type of asymmetry has been found in relationships between other species of raptors and grouse, where alternative prey limit raptors (Redpath and Thirgood 1999, Thirgood et al. 2000).
As mentioned above, sagebrush comprises most of the sage-grouse diet, although forbs are important for juveniles and adults in summer (Fig. 1, Table 2). Jackrabbits feed mainly on grasses and forbs, and also browse sagebrush and other shrub species (Fig. 1, Table 2). Anderson and Shumar (1986) found that during a population peak, jackrabbits reduced sagebrush cover by up to 34%. Although this difference was not statistically significant (the authors attributed this to small samples), jackrabbits caused a significant decrease in total vascular plant cover. Similarly, Danvir (2002) indicated that winter browsing by high densities of jackrabbits caused extensive defoliation and sometimes killed sagebrush plants.
Indirect Effects of Coyote Control on Sage-grouse and Sagebrush Communities
One likely effect of coyote removal in sagebrush habitats is an increase in abundance of mesopredators such as foxes, badgers, and ravens, which in turn are important sage-grouse nest predators. Evidence supporting the mesopredator release hypothesis has grown in the last decade (Palomares et al. 1995, Rogers and Caro 1998, Courchamp et al. 1999, Crooks and Soulé 1999, Schmidt 2003), and a fair number of observational and experimental studies document direct (interspecific killing) as well as indirect (behavioral avoidance) effects of coyote presence on fox and badger populations (Table 2). The interaction between coyotes and ravens is less well studied (Table 2). However, coyotes dominate ravens at carcasses (Wilmers et al. 2003), and carrion, especially during winter (Boarman and Heinrich 1999), is an important food resource for ravens that may influence winter survival. Thus, competition between coyotes and ravens might be a potential mechanism influencing raven populations, as suggested for other avian scavengers that compete with ravens for carrion (Boarman and Heinrich 1999). Another mechanism by which fox, badger, and raven abundances could increase under coyote removal would be through the expected increase in prey availability (i.e., jackrabbits and ground squirrels). Willis et al. (1993) and Feldhamer et al. (2003) describe both these prey types as part of the diet of foxes, badgers, and ravens, although these direct interactions are not shown in Figure 1. Coyote abundance is often correlated with jackrabbit and rodent abundances, and a coyote removal experiment documented an increase in abundance of badgers, foxes, and jackrabbits (Henke and Bryant 1999).
The potential increase in jackrabbit and ground squirrel abundances with the removal of coyotes would also positively influence Golden Eagle populations. Higher predation rates as a result of more eagles are another potential negative indirect effect of coyote removal on sage-grouse. This is similar to the negative indirect effects between feral pigs (Sus scrofa) and the island fox (Urocyon littoralis) mediated through a shared predator (Golden Eagles) on the California Channel Islands (Roemer et al. 2002). A comparable example is where high densities of rabbits (Oryctolagus cuniculus) led to increased numbers of Northern Goshawks (Accipiter gentilis) and increased predation on Ring-necked Pheasants (Phasianus colchicus; Kenward 1986). This negative effect is the result of apparent competition (Holt 1977), whereby two prey share a common predator and an increase in one prey species causes an increase in both the predator and predation on the other prey species, depressing its population. There is considerable evidence for the importance of alternative prey for raptors on predation rates on grouse (reviewed by Valkama et al. 2005), and such an interaction has even been considered in their management. Smith et al. (2001) suggested that Red Grouse (Lagopus l. scoticus) populations would benefit if habitat structure were altered to reduce the densities of alternative prey for Hen Harriers (Circus cyaneus), thereby reducing harrier abundance and predation on grouse.
Evidence for and recognition of the importance of apparent competition is increasing (Schmitt 1987, Holt and Lawton 1994, Bonsall and Hassell 1997, Roemer et al. 2002, Morris et al. 2004). The strength of this potential indirect effect on sage-grouse is unclear, given that an increase in mesopredator populations could buffer the jackrabbit and ground squirrel increases expected with coyote removal. Nonetheless, all mesopredators, jackrabbits, and rodents increased in a coyote control experiment (Henke and Bryant 1999), indicating that an increase in mesopredators does not necessarily reduce jackrabbit and rodent abundances. The above-mentioned cascade of effects caused by a reduction in coyote numbers might negatively impact sage-grouse populations through a decrease in nest and brood success (because of increased fox, badger, and raven predation as a result of mesopredator release) and an increase in adult and juvenile predation (from eagle predation as a result of apparent competition). Danvir (2002) provides a possible example for positive indirect effects of coyotes on sage-grouse. He found a nearly 500% increase in sage-grouse lek counts following cessation of coyote control and occupation of almost all available habitat on the study area by coyote pairs. Danvir (2002) suggested that the presence of coyotes positively affected sage-grouse indirectly by reducing the density of sage-grouse nest predators and regulating the abundance of adult sage-grouse predators, although in the end he did not consider these interactions to be the cause of the increase in sage-grouse, instead emphasizing habitat manipulations.
Coyote and jackrabbit populations also fluctuate in apparent predator-prey cycles (Wagner and Stoddart 1972). Such population cycling could have important consequences for sage-grouse. For example, when jackrabbit populations decline, Golden Eagles may feed more intensively on sage-grouse, causing sage-grouse populations to decline and perhaps cycle with a slight lag relative to jackrabbit cycles. This would be similar to results found in studies of other raptors and grouse (reviewed by Valkama et al. 2005). For example, predation on forest grouse by goshawks in the boreal forests of Canada and by Common Buzzards (Buteo buteo) in western Finland increased following declines of snowshoe hare (Lepus americanus) and vole (Microtus spp.) populations, respectively (Doyle and Smith 2001, Martin et al. 2001, Reif et al. 2001). Such yearly fluctuations would lead to variation in the importance of indirect effects of coyotes on sage-grouse and to variation in findings among studies (Stoddart et al. 2001). However, if these indirect effects are important, future studies could take advantage of natural population cycles to test for indirect effects.
In addition to these proposed predator-mediated indirect effects, another potential positive indirect effect of coyotes on sage-grouse may arise through a reduction in exploitative competition, whereby lower densities of jackrabbits result in an increase in the amount of vegetation that is vital for sage-grouse survival (Fig. 1). Wagner (1988) proposed a similar argument that sustained reduction of coyote numbers caused greater competition between jackrabbits and livestock for available forage. Although exploitative competition is a conceivable indirect interaction between jackrabbits and sage-grouse, and decreases in coyotes would likely intensify this interaction, it is unclear if this is an important interaction for sage-grouse because studies to date indicate only that such a mechanism is plausible (Table 2). A study using exclosures preventing jackrabbits but not sage-grouse from entry would potentially provide a simple means to test this hypothesis.
Finally, some studies indicate that gray wolves (Canis lupus) can limit coyote numbers (Bekoff and Gese 2003, Paquet and Carbyn 2003). If wolves depress the abundance of coyotes, then the importance of the interactions we described above could be an artifact of the elimination by humans of wolves from much of their former habitat. However, it is unclear that wolves consistently depress coyote numbers (Bekoff and Gese 2003). Moreover, wolves were likely uncommon in sagebrush habitats because the large ungulates on which wolves rely (Paquet and Carbyn 2003) were uncommon in sagebrush habitats in the Great Basin prior to European settlement (Mack and Thompson 1982). Indeed, wolves have “always been scarce” in Nevada (Young and Goldman 1944:30), which has more sagebrush habitat than any other state (Knick et al. 2003). Consequently, we believe that wolves were unlikely to have had much of an impact on coyote populations across most sagebrush habitats either before or after European settlement.
Conclusions
Coyotes likely reduce the potential negative effects of three indirect interactions that could adversely affect sage-grouse populations. Coyotes reduce the number of mammalian nest predators that eat sage-grouse eggs and young (mesopredator release). By limiting the number of jackrabbits, coyotes reduce the number of Golden Eagles preying on sage-grouse (apparent competition) and perhaps reduce the number of competitors eating plants consumed by sage-grouse (exploitative competition). Although the evidence for direct interactions that would lead to these indirect effects is substantial (Table 2), carefully controlled studies (e.g., Schmitt 1987) are necessary to rigorously test for the hypothesized positive indirect effects of coyote presence on sage-grouse populations. Nevertheless, we believe it is imperative to consider our proposed mechanisms of interaction when managing for sage-grouse even in the absence of studies testing for indirect effects. The types of direct interactions found between species in the sagebrush community commonly lead to the indirect effects that we are postulating (mesopredator release: Palomares et al. 1995, Rogers and Caro 1998, Crooks and Soulé 1999, Schmidt 2003; apparent competition: Schmitt 1987, Holt and Lawton 1994, Bonsall and Hassell 1997, Roemer et al. 2002, Morris et al. 2004; exploitative competition: Denno et al. 1995, Schluter 2000), and some data are consistent with such positive indirect effects of coyotes on sage-grouse (Danvir 2002). We have not intended to argue that ceasing coyote control and enhancing coyote populations will alone stop further declines in sage-grouse. Healthy coyote populations and the elimination of coyote control measures, however, might greatly enhance the long-term prospects for sage-grouse populations.
Acknowledgments
ETM was supported by a postdoctoral fellowship from Ministerio de Educacion Cultura y Deportes of Spain. CWB is grateful to R. B. Berry for sending a copy of a report that instigated this study and for funding that allowed CWB to think about such things. During the writing of this paper, CWB was supported in part by a grant from the National Science Foundation (DEB-0502944). J. L. Beck, T. Christiansen, D. Dobkin, J. Estes, D. MacDonald, and two anonymous reviewers made helpful comments on a previous draft of this manuscript, and S. Buskirk and M. Kauffman provided critical references.
Literature Cited
Author notes
Corresponding author. E-mail: [email protected]