-
PDF
- Split View
-
Views
-
Cite
Cite
Carter T. Atkinson, Julie K. Lease, Robert J. Dusek, Michael D. Samuel, Prevalence of Pox-Like Lesions and Malaria in Forest Bird Communities on Leeward Mauna Loa Volcano, Hawaii, The Condor: Ornithological Applications, Volume 107, Issue 3, 1 August 2005, Pages 537–546, https://doi.org/10.1093/condor/107.3.537
- Share Icon Share
Abstract
Introduced avian pox virus and malaria have had devastating impacts on native Hawaiian forest birds, yet little has been published about their prevalence and distribution in forest bird communities outside of windward Hawaii Island. We surveyed native and non-native forest birds for these two diseases at three different elevations on leeward Mauna Loa Volcano at the Kona Forest Unit of Hakalau Forest National Wildlife Refuge. Prevalence of malaria by both serology and microscopy varied by elevation and ranged from 28% at 710 m to 13% at 1830 m. Prevalence of pox-like lesions also varied by altitude, ranging in native species from 10% at 710 m to 2% at 1830 m. Native species at all elevations had the highest prevalence of malarial antibody and pox-like lesions. By contrast, pox-like lesions were not detected in individuals of four non-native species and only 5% of Japanese White-eye (Zosterops japonicus) was positive for malaria. A significantly high proportion of birds with pox-like lesions also had serological evidence of concurrent, chronic malarial infections, suggesting an interaction between these diseases, dual transmission of both diseases by the primary mosquito vector (Culex quinquefasciatus) or complete recovery of some pox-infected birds without loss of toes. Results from this study document high prevalence of malaria and pox at this refuge. Development of effective disease control strategies will be important for restoration of remnant populations of the endangered ‘Akiapola‘au (Hemignathus munroi), Hawaii Creeper (Oreomystis mana), and Hawaii ‘Akepa (Loxops coccineus coccineus) that still occur on the refuge.
Prevalencia de Lesiones del Tipo de la Viruela Aviar y Malaria en Comunidades de Aves de Bosque en el Volcán Leeward Mauna Loa, Hawai
Resumen. La malaria y la viruela aviar, ambas introducidas, han tenido un impacto devastador sobre las aves nativas de bosque, pero se ha publicado poco sobre su prevalencia y distribución en las comunidades fuera de la isla de Hawai. Muestreamos aves de bosque nativas y no nativas para determinar la presencia de estas dos enfermedades a diferentes elevaciones en el volcán Mauna Loa en la Unidad Forestal de Kona del Refugio de Fauna Silvestre del Bosque Nacional de Hakalau. La prevalencia de malaria determinada a través de serología y microscopía varió con la altitud entre un 28% a 710 m y un 13% a 1830 m. La prevalencia de lesiones del tipo de la viruela aviar también varió con la altitud, entre un 10% a 710 m y un 2% a 1839 m en especies nativas. Las especies nativas tuvieron la más alta prevalencia de anticuerpos contra malaria y lesiones del tipo de la viruela aviar. De modo contrastante, las lesiones del tipo de la viruela aviar no se detectaron en individuos de cuatro especies no nativas y sólo el 5% de los individuos de la especie Zosterops japonicus fue positivo para malaria. Una proporción significativamente alta de las aves con lesiones del tipo de la viruela aviar también presentaron evidencia serológica de infecciones crónicas de malaria simultáneas, sugiriendo una interacción entre estas dos enfermedades, transmisión dual de las enfermedades por el vector principal (el mosquito Culex quinquefasciatus) o recuperación completa en algunas aves infectadas con viruela aviar sin la pérdida de dedos. Los resultados de este estudio documentan altas prevalencias de malaria y viruela aviar en este refugio. El desarrollo de estrategias de control de estas enfermedades será importante para la restauración de las poblaciones remanentes de las especies en peligro Hemignathus munroi, Oreomystis mana, y Loxops coccineus coccineus que aún existen en el refugio.
Introduction
The introduction of mosquito vectors, avian pox (Poxvirus avium), and malaria (Plasmodium relictum) to the Hawaiian Islands is a major factor responsible for the continuing decline and extinction of more than 60 species of forest birds that are endemic to the islands (Warner 1968, van Riper et al. 1986, Jacobi and Atkinson 1995, van Riper et al. 2002). While native thrushes (Myadestes spp.) and subspecies of the native flycatcher (Chasiempis sandwichensis) appear to exhibit some resistance to these diseases (Scott et al. 1986, Atkinson et al. 2001a, Vanderwerf et al. 2001), all 16 species of extant Hawaiian honeycreepers (Drepanidinae) appear to be particularly vulnerable to them. High mortality following natural and experimental infection with avian pox or malaria have been described in Laysan Finches (Telespiza cantans), ‘Anianiau (Hemignathus parvus), ‘Apapane (Himatione sanguinea), Hawaii ‘Amakihi (Hemignathus virens), ‘I‘iwi (Vestiaria coccinea), and Maui ‘Alauahio (Paroreomyza montana; Warner 1968, van Riper et al. 1986, Atkinson et al. 1995, 2000, 2001a, Yorinks and Atkinson 2000, van Riper et al. 2002). In spite of this, published reports about the prevalence and distribution of these diseases in forest bird communities in Hawaii are still limited both in geographic and temporal scope, with most studies focused on windward Hawaii Island (van Riper et al. 1986, 2002, Feldman et al. 1995, Massey et al. 1996, Shehata et al. 2001, Atkinson et al. 2001a).
The leeward slopes of Mauna Loa and Hualalai Volcanoes on the island of Hawaii currently have fragmented populations of three endangered honeycreepers, ‘Akiapola‘au (Hemignathus munroi), Hawaii Creeper (Oreomystis mana), Hawaii ‘Akepa (Loxops coccineus coccineus), and a single remnant population of the ‘Alalā (Corvus hawaiiensis) which is now believed to be extinct in the wild (USFWS 2003a). These areas are considered prime recovery habitats for these endangered species and the U.S. Fish and Wildlife Service and collaborators have released captive reared ‘Alalā near the Kona Forest Unit of Hakalau Forest National Wildlife Refuge (hereafter Kona Forest Unit; U.S Fish and Wildlife Service 2003a, 2003b). Of great concern is the potential impact that locally transmitted avian malaria and pox may have on attempts to restore these birds in otherwise suitable habitat (Jenkins et al. 1989, Massey et al. 1996).
In this study, we determined prevalence of pox-like lesions and avian malaria in native and non-native passerines from the Kona Forest Unit during spring-summer of 1995 as part of a larger investigation on the ecology and transmission of vector-borne avian diseases in the refuge. This area was established as a National Wildlife Refuge in 1998 to protect remaining core habitat of the endangered ‘Alalā and other endangered forest birds on the leeward slope of Mauna Loa Volcano. Development of a management strategy for controlling these introduced diseases will be important for eventual restoration of forest bird communities in this area.
Methods
Native and non-native passerines were captured with mist nets in March, April, and May 1995 at three locations at the Kona Forest Unit (Fig. 1). Each site was sampled for three consecutive days at three consecutive monthly intervals, for a total of 9 days of mist-netting effort at each location. Ten, 9 m long, 30-mm mesh mist nets were suspended approximately 6 m above the ground between metal poles made from 20-mm diameter electrical conduit. The ten mist nets were placed in natural breaks in the vegetation or next to fruiting or flowering plants and were scattered over areas that were roughly 1 hectare in size. The high-elevation site was located near the northern boundary of the refuge (19°24′N, 155°47′W, elevation 1830 m) in heavily grazed, open ohia (Metrosideros polymorpha) forest. The mid-elevation site was located near the center of the refuge (19°23′N, 155°49′W; elevation 1520 m) in heavily grazed, open, ohia–koa (Acacia koa) forest. The low-elevation site was located at the lower, northern boundary of the refuge (19°24′W, 155°51′N; elevation 710 m) in closed ohia forest with a tree fern (Cibotium glaucum) understory.
Mist nets at each location were opened from approximately 0730 to 1500 on each of three consecutive days for each of three monthly sampling periods. Birds were aged and sexed by plumage characteristics, morphometrics, and reproductive condition (Fancy et al. 1993, Lindsey et al. 1998), banded, and bled via jugular venipuncture with sterile, heparinized 28 gauge insulin syringes with half inch needles. Thin blood smears were made immediately after collecting the sample and fixed in absolute methanol. Remaining whole blood was transferred to microhematocrit tubes and stored on wet ice until the end of the day. Tubes were subsequently centrifuged at 10 000 G for 5 min and plasma was collected and frozen for serological analysis. Birds were also examined carefully for swollen or crusty lesions on exposed skin or missing toes which may be indicative of active or old pox infections. Lesions were classified as active based on presence of swellings or crusty scabs or inactive based on presence of missing toes that were otherwise healed (van Riper et al. 2002).
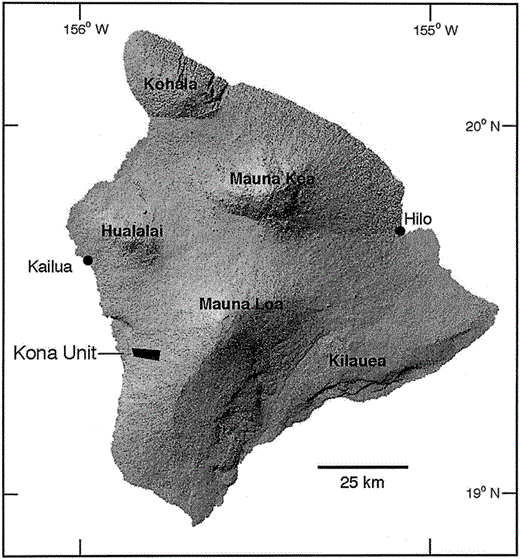
Location of the Kona Forest Unit (Kona Unit) of Hakalau Forest National Wildlife Refuge relative to major mountains and cities (indicated by circles) on the Island of Hawaii. Prevailing tradewinds blow from the northeast, depositing most rainfall on the eastern, windward slopes of Mauna Loa and Mauna Kea Volcanoes
Thin blood smears were stained with 2% Giemsa in 0.01 M phosphate buffer, pH 7.0 for one hour, rinsed with tap water and dried. Smears were scanned for 10 min at 400× to diagnose patent malarial infections. Approximately 30 000 erythrocytes were scanned during this time interval. For slides that were positive for erythrocytic parasites, we estimated parasitemia by counting numbers of erythrocytes in 5 100× fields on areas of the smears with uniform cell distribution and density. The first field was located haphazardly and the remaining 4 fields were selected with a random number table by moving the mechanical stage up or down x number of fields. Erythrocyte counts were then averaged to obtain mean number of erythrocytes per field and total number of fields per slide that would be needed to examine 20 000 erythrocytes. Numbers of fields counted varied from 150 to 250 per slide because of variability in smear thickness and erythrocyte density. Counts of infected erythrocytes were made from this predetermined number of fields for each positive blood smear and expressed as number of infected cells per 20 000 erythrocytes.
An immunoblot analysis of forest bird plasma was done as described previously to detect chronic, low intensity malarial infections (Atkinson et al. 1995, 2001a, 2001b). Briefly, malarial antigen was separated by sodium dodecyl sulfate (SDS) polyacrylamide gel electrophoresis, transferred electrophoretically to polyvinylidene difluoride (PVDF) membranes, and probed with plasma from native and non-native forest birds in a BioRad (Hercules, CA) multiscreen apparatus that divided membranes into 20 adjacent lanes. Membranes were incubated with rabbit antiforest bird immunoglobulin antibody followed by affinity purified alkaline phosphatase-labeled goat antirabbit antibody (Pierce, Rockford, Illinois) and then developed with an alkaline phosphatase substrate kit (BioRad). Controls on each membrane included lanes incubated without primary antibody and lanes incubated with known positive plasma from an experimentally-infected canary. Criteria for judging a blot positive or negative for malaria was reactivity to one or more bands of the following molecular weights: 22, 34, 44, 58, or 170 kDa.
Statistical Analyses
Logistic regression in program LogXact (Metha and Patel 1996) was used to test for association between prevalence of malarial antibody and the independent covariates of elevation, native vs. non-native species, age, and sex. Statistical analyses were conducted sequentially, where each step expanded the number of evaluated covariates, but also reduced the dataset by removing cases with unknown values (e.g., unknown age or sex). In each step all of the covariates were included simultaneously to account for correlation among independent variables. A similar analysis was done for prevalence of pox-like lesions to test for association between lesion prevalence and elevation, native vs. non-native species, age, and sex. We used the chi-squared statistic to evaluate independence of pox-like lesions and serological evidence of malarial infection and the Cochran-Mantel-Haenszel statistic to evaluate this relationship while considering the independent covariates of elevation and sex (Woodward 1999). Results were considered significant when P ≤ 0.05.
Total captures and malaria and pox prevalence by species at each elevation. Malarial prevalence is based on a subsample of 438 native and non-native individuals that were tested by both microscopy and serology. Pox prevalence is based on physical examination of all captured individuals for pox-like lesions. Species are indicated by codes as follows: HAAM = Hawaii ‘Amakihi; ELEP = ‘Elepaio; IIWI = ‘I‘iwi; APAP = ‘Apapane; AKEP = Hawaii ‘Akepa; RBLE = Red-billed Leiothrix; HOFI = House Finch; NOCA = Northern Cardinal; JAWE = Japanese White-eye; NC = none captured; and NB = not bled
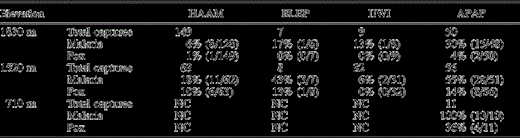
Total captures and malaria and pox prevalence by species at each elevation. Malarial prevalence is based on a subsample of 438 native and non-native individuals that were tested by both microscopy and serology. Pox prevalence is based on physical examination of all captured individuals for pox-like lesions. Species are indicated by codes as follows: HAAM = Hawaii ‘Amakihi; ELEP = ‘Elepaio; IIWI = ‘I‘iwi; APAP = ‘Apapane; AKEP = Hawaii ‘Akepa; RBLE = Red-billed Leiothrix; HOFI = House Finch; NOCA = Northern Cardinal; JAWE = Japanese White-eye; NC = none captured; and NB = not bled
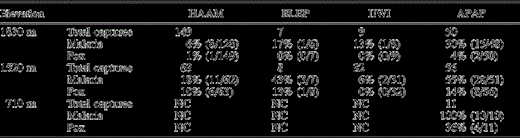
Results
Malaria
We captured 484 birds belonging to 9 species during the study (Table 1), but collected blood samples from only 466 of these individuals. Most birds were captured at the high and mid-elevation sites, even though mist net effort was roughly equal at each area (594 net hours at high elevation, 644 hours at mid-elevation, and 634 hours at low elevation). Native species dominated captures at high and mid-elevations and were absent from the low-elevation site with the exception of ‘Apapane (captured in mist nets) and Hawaii ‘Amakihi (seen and heard, but not captured).
Of the 466 individuals that were bled at least once, 55 (12%) were positive by microscopy for malaria, with most infections detected in ‘Apapane (n = 40), followed by Hawaii ‘Amakihi (n = 9), ‘Elepaio (n = 2), ‘I‘iwi (n = 2), and Japanese White-eye (n = 2). Parasitemia was low and ranged from <1 to 80 infected erythrocytes per 20 000 erythrocytes. Prevalence determined by microscopy varied among sites from 8% (18/ 216) at 1830 m, to 13% (28/209) at 1520 m, to 22% (9/41) at 710 m.
We had sufficient blood to test 438 birds by both microscopy and immunoblotting and these samples were the basis for statistical analysis. Fifty of these individuals were positive by microscopy and 47 of these were positive by immunoblot. Three birds that were positive by microscopy and blot negative included a Japanese White-eye, an ‘Apapane, and an ‘I‘iwi. Using presence of plasma antibody to one or more of the major 22, 34, 44, 58, or 170 kDa antigens as criteria for scoring an immunoblot as positive for malaria (Fig. 2), we found an additional 32 that were serologically positive for malaria but negative by microscopy. This information allowed us to evaluate sensitivity and specificity of the immunoblot using blood smears as a standard for comparison. Sensitivity of the immunoblot (probability of correctly diagnosing malaria in birds with known infections) was 94% (47/50). Specificity of the immunoblot (probability of the test being negative among birds that are negative by microscopy) was 92% (356/ 388).
Overall prevalence of malaria for birds that were tested by both immunoblot and microscopy was 19% (82/438). Malarial prevalence by elevation as measured by both microscopy and serology varied significantly (P < 0.001) from 13% (25/199) at 1830 m, to 23% (46/200) at 1520 m, to 28% (11/39) at 710 m. Prevalence of malarial infections by microscopy and serology was significantly higher (P < 0.001) in native species (23%, 79/351) than in non-native species (3%, 3/87), with no significant interaction (P > 0.25) between elevation and status as a native or non-native species. When malarial prevalence in native species was compared by elevation, ‘Apapane had the highest prevalence of infection at all elevations, followed by ‘Elepaio, Hawaii ‘Amakihi and ‘I‘iwi (Table 1).
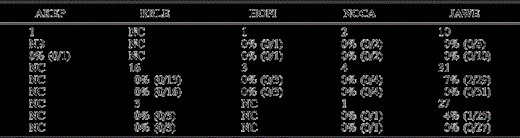
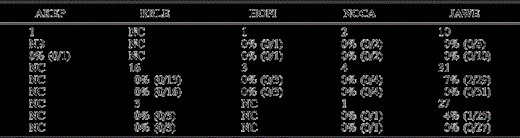
Birds with unknown age classifications (n = 83) were removed from the analysis and malarial prevalence as measured by microscopy and serology was evaluated against status as native or non-native species, elevation and age. In this analysis there was a significantly higher (P < 0.001) prevalence in native birds, a significant decline (P < 0.001) in prevalence with elevation, and malarial infections occurred more frequently (P < 0.001) in after hatch-year (AHY) birds (32%, 74/231) than in hatch-year (HY) birds (2%, 2/124). There was no significant interaction (P > 0.35) between age and elevation, so HY birds had a lower prevalence of infection at all elevations. When birds with unknown age or sex (n = 178) were removed from the analysis there was a significantly higher (P < 0.001) prevalence in native birds, a significant decline (P < 0.001) in prevalence with elevation, higher (P < 0.001) prevalence AHY birds, and malarial prevalence was higher (P = 0.02) in males (33%, 57/171) than in females (20%, 18/89).
Our final analysis considered only native birds with known age and sex (n = 236, Table 2). There was a significant decline (P < 0.001) in prevalence with elevation from 100% (10/10) at low elevation to 39% (42/109) at mid-elevation to 19% (22/117) at high elevation. Malarial prevalence was higher (P < 0.001) in AHY birds (43%, 72/167) than in HY birds (3%, 2/ 69), and higher (P = 0.03) in males (38%, 56/ 148) than in females 20% (18/88). There were no significant interactions between sex and elevation (P > 0.6) or between sex and age (P > 0.15).
A total of 34 birds were sampled 2 or more times at intervals of 1 to 3 months, allowing us to determine whether malarial transmission was occurring during the sampling period. We had sufficient plasma to run immunoblots and examine smears from 30 of these individuals. There were no cases where a bird changed infection status from negative to positive and only one case, a Japanese White-eye, that changed infection status from immunoblot-positive to immunoblot-negative.
Pox
Of 484 birds that were captured during the study, 22 (5%) had pox-like lesions on the legs or feet. Of these 22 cases, 13 (59%) were classified as inactive lesions based on missing toes or portions of toes and nine (41%) were classified as active lesions based on presence of small wart-like, smooth swellings either alone on the legs or feet or in combination with missing toes. We did not observe lesions on the beak or soft tissues around the eyes or cases of wet or diphtheritic pox on the mucous membranes of the oral cavity. Three birds with pox-like lesions were captured on at least two occasions in sequential months. In all three cases the birds had missing toes and the location or size of the lesions did not change.
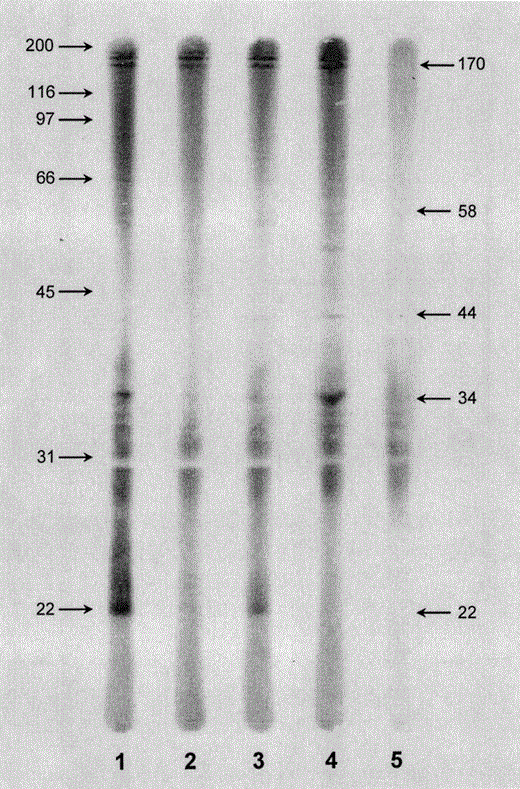
Immunoblot analysis of plasma samples from wild honeycreepers at the KFU that were positive for malaria by microscopy. Lanes 1, ‘Apapane; Lane 2, ‘Elepaio; Lane 3, Hawaii ‘Amakihi; Lane 4, ‘I‘iwi; Lane 5, negative control incubated with rabbit antiforest bird immunoglobulin, goat antirabbit IgG alkaline phosphatase conjugate and enzymatic substrates alone. Molecular weight markers (kDa) are indicated on left. Major antigens (22, 34, 44, 58, and 170 kDa) are marked on the right
Native species had a higher prevalence (6%, 22/386) of pox-like lesions (P = 0.01) than non-native birds (0/98). For native species, prevalence of lesions by elevation varied (P < 0.001) from 2% (4/216) at 1830 m, to 9% (14/159) at 1520 m, to 40% (4/11) at 710 m (Table 1). As was the case with malaria, most pox-like lesions occurred on ‘Apapane (n = 14), followed by Hawaii ‘Amakihi (n = 7), and ‘Elepaio (n = 1). All infected individuals that could be accurately aged were older than one year. Pox-like lesions were more frequent (P < 0.001) in AHY natives (10%, 20/196) than in HY natives (0/130). Pox-like lesions were more frequent in native AHY males (13%, 16/119) than females (6%, 4/65); however, these prevalences were similar (P > 0.2) when the differences related to elevation were considered.
Prevalence of malaria in native species by age class and sex. Only birds that could be aged or sexed by morphometrics, plumage characteristics, or reproductive condition are included here. All individuals were tested by both microscopy and serology. HY = hatch-year, AHY = after hatch-year, and NC = none captured

Prevalence of malaria in native species by age class and sex. Only birds that could be aged or sexed by morphometrics, plumage characteristics, or reproductive condition are included here. All individuals were tested by both microscopy and serology. HY = hatch-year, AHY = after hatch-year, and NC = none captured

Of the native AHY birds that could be tested for malaria by microscopy and serology, 12% (20/167) had pox-like lesions and 43% (72/167) had serological evidence of chronic malarial infections. A significantly higher (P < 0.001) proportion (11%, 18/167) of birds than expected had concurrent pox-like lesions and antibody to avian malaria. This strong association of pox-like lesions and antibody to avian malaria was also consistent between males and females and among elevations.
Discussion
We documented altitudinal distribution of malaria and pox-like infections on the leeward slopes of Mauna Loa Volcano with a combination of microscopy, serology and presumptive diagnosis of pox infection based on presence or absence of lesions. Four species of endangered forest birds at the refuge are at high risk of exposure to malaria and pox: ‘Alalā, ‘Akiapola‘au, Hawaii Creeper, and Hawaii ‘Akepa. Captive-reared ‘Alalā that were reintroduced into the wild at sites along the southern boundary of the refuge at an elevation of 1500 m acquired malaria soon after release (Massey et al. 1996). Likewise, Jenkins et al. (1989) also reported acute malarial and pox infections in ‘Alalā nestlings and adults from North and South Kona near the refuge, but did not provide details about the elevation of the nest location. There are no well documented reports of pox and malaria infection in Hawaii Creeper, Hawaii ‘Akepa, and ‘Akiapola‘au, but all three of these species are limited to the highest elevation forests on the island and are currently very rare on the refuge (D. Ball, pers. comm.).
Van Riper et al. (1986, 2002) reported the distribution of pox and malaria from sea level to 2000 m on windward Mauna Loa Volcano from a sample of birds captured in the late 1970s. Prevalence was determined by microscopic examination of Giemsa-stained blood smears to diagnose malarial infections and occurrence of missing toes and swellings to make presumptive diagnoses of pox infections. Our findings are in general agreement with those reported from the windward side of the mountain. Native species are the primary reservoirs of pox and malaria infection, intensities of malarial infection were low, and prevalence by species were comparable, but higher using more sensitive diagnostic methods. Unlike van Riper et al. (1986), we captured ‘Apapane across the entire altitudinal range of our study and observed prevalences of infection as high as 100% for malaria in low elevation captures of this species. ‘Apapane typically undergo altitudinal migrations to follow ohia bloom and might be expected to receive higher exposure to this disease than other more resident species such as ‘Amakihi and ‘Elepaio (van Riper et al. 1986). High mobility coupled with high susceptibility to the parasite (Yorinks and Atkinson 2000) make them exceptional reservoir hosts for the parasite. Interestingly, Nielsen (2000) found active ‘Apapane nests as low as 600 m on the refuge. Nesting success in these ‘Apapane did not differ significantly from those at higher elevation, in spite of high prevalence of malaria in mosquitoes captured near nest sites. Given high prevalences of antibody identified in this study, it is likely that these ‘Apapane are immune survivors of prior acute infections (Yorinks and Atkinson 2000). It is possible that resistance to malaria is evolving in these birds as a result of higher transmission rates and selective pressures at low elevations.
‘I‘iwi are particularly vulnerable to malaria and we found only 2 of 39 individuals with malarial infections (Atkinson et al. 1995). One of these birds was smear-positive and serologically negative for malaria, suggesting that it had a recent acute infection (Atkinson et al. 2001b). Otherwise, the low prevalence of infection in both hatch-year and after-hatch-year ‘I‘iwi from areas where prevalence is much higher in other species implies that few ‘I‘iwi survive exposure, and is consistent with experimental studies documenting high susceptibility of this species to malaria (Atkinson et al. 1995, van Riper et al. 1986). By contrast, non-native species challenged with Hawaiian isolates of P. relictum under controlled conditions either resist infection or develop short-lived, low intensity parasitemias with no subsequent mortality (Atkinson et al. 1995, van Riper et al. 1986).
We found significant differences in prevalence of malaria by both age class and sex among native birds. Adult birds had a higher prevalence based on microscopy and serology than hatch-year individuals and males had a higher prevalence than females. Mist netting and blood collection was performed during the breeding season when mosquito numbers are lowest at elevations above 1000 m (LaPointe 2000). In addition, malaria transmission and epizootics at mid-elevation vary annually depending on vector abundance and related weather conditions and other factors (Ahumada et al. 2004). Thus it is not surprising that most hatch-year individuals were negative for malaria. It is not clear to us, however, why males had higher prevalence than females when previous studies suggest males suffer higher morbidity and mortality after infection with malaria (Atkinson et al. 1995). Higher prevalence in males was consistent for all native species that we captured, with the exception of ‘Elepaio where the sample size was small. Potential explanations include behavioral differences that increase exposure of males to mosquito vectors that transmit disease, or differences in susceptibility to predation during acute phase infections (Yorinks and Atkinson 2000).
We found a pattern of pox-like lesions in native and non-native species that was very similar to what we observed with malaria. Lesions were limited to native species and a higher prevalence of both old and active lesions occurred at low and mid-elevations where mosquito vectors are more active. Prevalence of pox-like lesions was also higher in adults than hatch year birds indicating increasing exposure to disease with age. In contrast to malaria, pox-like lesions did not occur more frequently in males than in females; however, the lower overall prevalence of lesions and smaller sample size may have limited our ability to detect significant differences. Of particular interest was the high prevalence of pox-like lesions in adult native birds that were serologically positive for malaria. This indicates a much higher frequency of pox-like lesions in birds with chronic malarial infections than would be expected if the two diseases were transmitted independently. Van Riper et al. (1986) reported a similar association between birds with lesions and birds that were positive by microscopy on windward Mauna Loa and suggested that prior infection with pox could lower host resistance and make birds more susceptible to malaria. Since older birds are more likely to have survived acute malarial infections and to be infected with pox, one potential explanation for the association between these diseases is that prevalence of malarial antibody reflects longer potential exposure to pox infection. Other possibilities include simultaneous transmission of both diseases by mosquito vectors that carry both pathogens, differential mortality among pox, malaria, and pox-malaria infected birds, or recovery of some fraction of pox-infected birds without the loss of toes or development of permanent lesions that would allow an accurate presumptive diagnosis in the field. Because these results indicate an interaction between malaria and pox infections, closely linked field and laboratory studies will be required to determine whether malaria or pox infections occur first, which diseases have a significant impact on mortality, and whether one disease increases the risk of concurrent infection.
While microscopy is still considered the most definitive test for diagnosing malaria, sensitivity of the method for detecting chronic, subpatent parasitemias is extremely low, potentially missing up to 75% of these infections (Jarvi et al. 2002). Recent application of both enzyme-linked immunosorbant assays (ELISA) and immunoblotting methods for serological detection of antibodies to P. relictum and PCR methodology for amplification of parasite DNA has significantly improved diagnostic accuracy for avian malaria (Feldman et al. 1995, Massey et al. 1996, Atkinson et al. 2001a, Jarvi et al. 2002). Like microscopy, however, all three of these methods can yield false positive and negative results depending on the stage and duration of infection, parasitemia, and antibody titer in the peripheral circulation, and specificity of immunoreagents and PCR primers (Jarvi et al. 2002). We minimized false positives and negatives by using microscopy to identify early acute infections without detectable circulating antibody and serology to identify older chronic infections with low peripheral parasitemias and high levels of antibody, but even this combination of tests may have missed some infections—particularly those that are less than 2–3 days old where parasites have not yet invaded circulating erythrocytes (Garnham 1966). Available evidence from experimental studies of Hawaiian forest birds and other passerines indicates that they likely remain infected for life with chronic, low-level infections that stimulate humoral and cellular immunity to reinfection (Atkinson et al. 2001a, 2001b, Bishop et al. 1938, Jarvi et al. 2002). This prolonged immune response forms the basis for the high sensitivity and specificity of immunoblotting and we feel that antibody-based serological methods used in conjunction with microscopy or PCR are powerful epidemiological tools for studying the prevalence and incidence of avian malaria in forest bird populations.
This study documents primary reservoir hosts and elevational distribution of vector-transmitted avian malaria and pox virus at the Kona Forest Unit. Disease prevalence on the refuge is relatively high with up to 48% of ‘Apapane, 31% of ‘Elepaio, 10% of Hawaii ‘Amakihi, and 5% of ‘I‘iwi carrying acute or chronic malarial infections. Climate in this area is drier than windward Mauna Loa and affected strongly by the blockage of prevailing trade winds by Mauna Loa and Hualalai Volcanoes. Unlike the heavy orographic rainfall that occurs on the windward side of the island, moisture patterns on the leeward side are driven primarily by daytime surface heating and upslope winds that give rise to convective rainfall at elevations between 600 and 1800 m (Giambelluca and Schroeder 1998). The result is a band of mesic-wet ohia forest with annual precipitation of approximately 1.5– 2 m that becomes increasingly dry at elevations above 1500 m and below 600 m. The slope of Mauna Loa Volcano is also steeper on the leeward side of the mountain, with altitude changes of approximately 150 m per km vs. changes of 60–70 m per km on the windward side. These relatively steep slopes compress the available forest bird habitat into a steep gradient where short flight distances can bring forest birds to lower-elevation habitats where disease risks may be higher.
Our work provides baseline data for future efforts to control both of these diseases. The most feasible method for reducing transmission is by a reduction of mosquito populations through treatment or elimination of larval habitats on the refuge and adjacent lands. This will be especially challenging because of the steep altitudinal gradient that places high-elevation habitats in close proximity to mid-elevations where mosquitoes are more abundant. Also challenging is dense vegetation and the presence of recent lava flows and fence lines that provide natural and manmade corridors for movement of mosquitoes up the mountainside. Moreover, large numbers of feral pigs (Sus scrofa) both on the refuge and adjacent ranchlands create abundant habitats for mosquito larvae by felling and hollowing tree ferns for their starchy cores, leaving open troughs on the forest floor that catch and hold rainwater (CTA, unpubl. data). In spite of this, vector control through source reduction may be more feasible here than in most natural areas on the island because volcanic substrates on Mauna Loa are highly porous, slopes have no well-defined stream drainages, and almost all available water for mosquito development is associated with feral animals or human infrastructure created by the ranching industry (CTA, unpubl. data). Based on current distributional data and evidence of low-elevation reproduction, there is some evidence that resistance to these diseases is evolving in ‘Apapane on the refuge (Nielson 2000); however, rarer endangered species continue to decline and existing populations of ‘Akepa, Hawaii Creeper, and ‘Akiapola‘au face local extinction if disease transmission and other limiting factors are not controlled.
Acknowledgments
Research reported here was done prior to purchase of the Kona Forest Refuge by the U.S. Fish and Wildlife Service and we acknowledge the support and cooperation of the former landowners, Cynthia Salley and Nohea Santimer in granting access to the site. We also thank the Student Conservation Association and numerous dedicated Resource Assistants and interns who assisted with field work and data collection, including Marsha Dusek, Jennifer Goergen, Maureen Henry, Kerry Kenwood, Melissa Luanglue, J. Stuart Niven, Jennifer Osgood, and Sara Racciatti. This research was made possible in part by financial support from the U.S. Geological Survey, Wildlife and Invasive Species Programs. Any use of trade, product, or firm names in this publication is for descriptive purposes only and does not imply endorsement by the U.S. Government.
Literature Cited