-
PDF
- Split View
-
Views
-
Cite
Cite
John M. Bates, Allozymic Genetic Structure and Natural Habitat Fragmentation: Data for Five Species of Amazonian Forest Birds, The Condor: Ornithological Applications, Volume 102, Issue 4, 1 November 2000, Pages 770–783, https://doi.org/10.1093/condor/102.4.770
- Share Icon Share
Abstract
I studied allozymic variation in five species of Amazonian birds at two geographic scales. At a local scale (all sites within 200 km of one another), I included samples from six sites, three within continuous forest and three in natural forest fragments thought to be several thousand years old. I examined both genetic variation and differentiation to determine whether there were genetic effects related to forest fragmentation. At this local scale, I found little evidence in the allozymic data that clearly suggested genetic structure had been affected in any uniform pattern among species. However, there was genetic differentiation at this level and estimated gene flow (Nmest from private alleles) was low relative to what is reported in other avian studies. At the regional level, I compared samples from sites that were 500–1,500 km from one another from two Amazonian areas of endemism (Inambari and Rondônia). Four of the five species exhibited substantial differentiation between samples from the two areas of endemism, consistent with other studies of genetic differentiation in Neotropical forest understory birds.
Introduction
The Neotropics harbor some of the most species-diverse ecosystems on earth, yet we know little about genetic structure of populations there. Although birds are among the better-studied South American vertebrate groups, intraspecific genetic structure at any scale has been assessed in few of the over 3,000 species that occur on the continent.
Studies focusing on Neotropical forest species often have found greater genetic differentiation among populations than has been observed in better-studied temperate birds (Capparella 1988, Brawn et al. 1996, Brumfield and Capparella 1996). The ultimate reason for the difference may lie with the higher degree of sedentariness of Neotropical species. In contrast to the majority of temperate birds, most Neotropical birds do not migrate seasonally, and although dispersal has almost never been estimated for Neotropical species, it is assumed to be low especially for inhabitants of the forest understory (Greenberg and Gradwohl 1997). Neotropical forest species also are affected by human activity (Canaday 1995) and do not recolonize isolated forest fragments (Willis 1979, Stouffer and Bierregaard 1995). Coupled with data on increased intraspecific genetic structure, these studies suggest that many Neotropical species may be more susceptible to the effects of forest fragmentation than other birds.
The predicted effects of population fragmentation on genetic differentiation and variation are well known (Templeton et al. 1990, Saunders et al. 1991). Populations of sufficiently small size will lose genetic variation over time, and isolated populations receiving no gene flow will eventually differentiate from adjacent populations as a result of genetic drift, natural selection, or some combination of the two.
Allozymic studies have largely been replaced by direct sequencing of DNA; however, allozymes have provided important insight about the effects of population fragmentation on genetic differentiation between and genetic variation within isolated populations. I gathered allozymic data for five sedentary Amazonian understory birds to investigate the effect habitat fragmentation has on genetic structure at a local geographic scale. I present data on each species from six sites within 200 km of one another. Three of these sites are in continuous forest and three are in naturally formed forest fragments isolated from one another and from continuous forest by grassland and savanna. The goal at this scale was to investigate the effects of natural forest fragmentation on genetic structure. I also present data on a regional scale where populations were separated by 500 to 1,500 km.
With these comparisons, I address the following two predictions:
(1) Genetic isolation among populations in fragmented habitats will exceed genetic isolation found in populations separated by similar distances in continuous habitat (distances of less than 200 km). Genetic isolation includes genetic variation that occurs within populations (population variation), and genetic differentiation that occurs among populations (geographic variation). Both aspects provide insights into historic levels of gene flow and other evolutionary processes. At the local geographic scale, levels of genetic variation and differentiation in each of the five species should be a function of patch-size and isolation. Lower genetic variation within samples from forest fragments, as compared to samples from continuous forest, would indicate that forest fragmentation significantly depresses genetic variation owing to either inbreeding effects or reduced immigration or both. Congruent patterns of genetic differentiation in the five species would implicate vicariant (fragmentation) events as important in shaping population structure. Thus, the multiple species approach makes comparisons possible even though the actual history of fragmentation is not known. This is an important point, because although the forest fragments I studied are thought to be on the order of thousands of years old, no data currently pinpoint the timing or pattern of their origin (see below).
(2) Genetic isolation by distance between sites in continuous forest habitat in South American avian taxa will be greater than in comparable North American taxa (distances of 500–1,500 km). In the only study with large sample sizes and multiple populations of Amazonian birds, Capparella (1988) demonstrated substantial intraspecific genetic differentiation in several species across major rivers, suggesting that vicariant events and not simply geographic distance generate genetic differentiation in Amazonia. Such high levels of genetic differentiation are not often found in temperate bird species even at a continental scale (e.g., Gavin et al. 1992). At this regional scale, my study investigates genetic differentiation in additional avian taxa and from a different region of Amazonia than that studied by Capparella.
Methods
Study Sites
The six local-scale collecting sites are in the vicinity of the Serranía de Huanchaca, a 350-m tall plateau of uplifted Brazilian shield located in the northeastern corner of the Department of Santa Cruz, Bolivia (Fig. 1). All sites occur inside the Parque Nacional “Noel Kempff Mercado” or in the adjacent Reserva Forestal Bajo Paraguá (Killeen and Schulenberg 1998). Three sites (1–3) are in naturally formed forest fragments on top of the Serranía. Three other sites (4–6) are in continuous forest along the northern and western sides of the plateau.
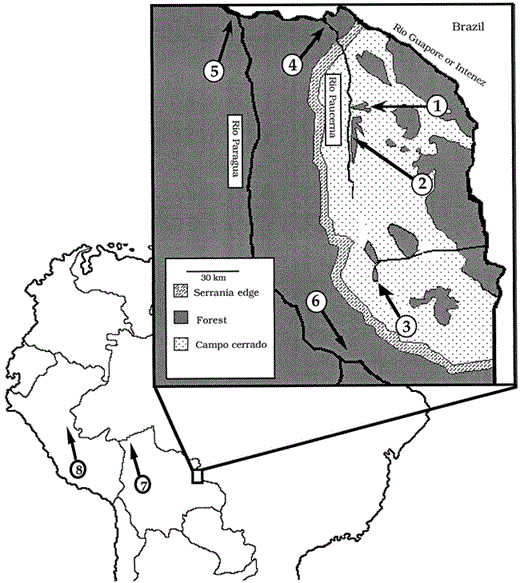
Sample localities at two geographic scales in western Amazonia. Sites 1–8 were sampled for all five taxa. Sites 1–6 are the local-level study sites; sites 7 and 8 provide a regional perspective to genetic structure, as they lie in a different avian area of endemism from sites 1–6 (see text). The inset is of the Serranía de Huanchaca region of the Department of Santa Cruz, Bolivia. Sites 1–3 lie in forest fragments on top of this plateau, sites 4–6 lie in continuous forest below the plateau. Exact locations are: Site 1, 21 km SE of Catarata Arco Iris (13°55′S, 60°45′W); Site 2, 25 km S of Catarata Arco Iris (13°55′S, 60°45′W); Site 3, ca. 45 km E of the town of Florida (14°34′S, 60°40′W); Site 4, west bank of the Río Paucerna, 4 km upstream from its confluence with the Río Itenez (13°43′S, 61°11′W); Site 5, Piso Firme (13°35′S, 60°55′W); Site 6, Los Fierros, 30 km E Aserradero Moira (14°25′S, 61°10′W); Site 7, BOLIVIA, Depto. Pando; ca. 12 km by road S Cobija, ca. 8 km W on road to Mucden (11°9′S, 68°58′W); Site 8, PERU, Depto. Ucuyali; ca. 65 km ENE Pucallpa, (8°8′S, 74°2′W). Additional data on these sites can be found in Bates et al. (1991, 1992), O'Neill et al. (1991), and Parker and Remsen (1987)
The Serranía de Huanchaca is believed to have been more forested in the past (O'Conner et al. 1987), with current forest fragmentation on top of the plateau occurring as a result of erosion of the lateritic crust that once covered it. Today, forest occurs only on remaining lateritic islands. On the plateau's eroded laterite, cerrado vegetation has invaded. Cerrado is a complex South American savanna habitat (Goodland 1971) that shares almost no floral or faunal species with Amazonian forest understory. The age of the laterite suggests that fragmentation could have begun as long as 10,000 years ago (O'Conner et al. 1987); however, the exact ages of the fragments have not been determined. Initially, forest fragments may have remained connected to continuous forest by the presence of gallery forest along streams; today, however, long stretches of these streams are bordered only by cerrado vegetation (Fig. 1). The fragments are currently separated from continuous forest by 5–30 km of cerrado. Avian species composition in the forest fragments at sites 1 (600 ha) and 2 (1,200 ha) is very similar to that of continuous forest sites (Bates and Parker 1998). In contrast, site 3 (350 ha), the smallest forest fragment, lacks many forest species found at other sites, including one of the five study species (see below).
Samples from two additional forest sites in the Department of Pando, Bolivia (site 7) and the Department of Loreto, Peru (site 8, Fig. 1) provide a regional-scale perspective on genetic structure found among sites 1–6. Sites 7 and 8 occur within Cracraft's (1985) South Amazon or Inambari area of endemism, whereas sites 1–6 lie on the western edge of the Rondônia area of endemism. Although there is forest connecting these two centers today, they are hypothesized to have been separated possibly during Pleistocene and earlier (Haffer 1993, Rasäsänen et al. 1995).
Study Taxa
The species studied are common, widespread inhabitants of the Amazonian forest understory. These species are thought to maintain year-round territories on the study sites as they do in other parts of Amazonia (Terborgh et al. 1990) and to have low levels of dispersal relative to migratory temperate passerine birds. They represent three major families of Neotropical suboscines (order Passeriformes). Glyphorynchus spirurus, the Wedge-billed Woodcreeper (Dendrocolaptidae), occurs in lowland forests from southern Mexico to the southern edge of the Amazon basin (all ranges from Peters 1951 or Traylor 1979). Hylophylax poecilinota, the Scale-backed Antbird, and Hypocnemis cantator, the Warbling Antbird (both Thamnophilidae), both range throughout the Amazon basin. Myrmeciza hemimelaena, the Chestnut-tailed Antbird (Thamnophilidae), ranges throughout southern Amazonia and north to southern Colombia on the western edge of the Amazon basin. Leptopogon amaurocephalus, the Sepia-capped Flycatcher (Tyrannidae), occurs from Mexico to Argentina. In Amazonia, the species is locally distributed along the eastern base of the Andes, spreading out across the southern part of the basin to the Rio Tapajos in central Brazil. There is recognized morphologic variation (described subspecies) in several of the taxa, corresponding to the Inambari and Rondônia areas of endemism (see above). For G. spirurus, H. cantator, and L. amaurocephalus, samples from sites 1–6 are representative of one subspecies, and populations from sites 7 and 8 represent another subspecies. In M. hemimelaena, morphologic variation suggests a possible cline (M. L. Isler, pers. comm.) between the two groups of sites (1–6, 7 and 8), and H. poecilinota populations are considered morphologically undifferentiated between the two sets of sites.
Allozymes and Data Analysis
Adult individuals of all species were captured in mist nets and prepared as study skins or skeletal specimens (deposited in the Louisiana State University Museum of Natural Science, LSUMNS). Samples of heart, liver, and breast muscle collected from each specimen were preserved in liquid nitrogen (−196°C) less than 30 min after death. Once transported to the LSUMNS, samples were stored in an ultracold freezer at −70°C until prepared for biochemical analysis.
For allozymic analysis, homogenates of each sample were prepared combining subsamples of each tissue type. The majority of each tissue was saved for extraction of mitochondrial DNA (Bates 1993). Methods for starch gel electrophoresis followed those of Richardson et al. (1986) and Murphy et al. (1990). The buffer systems employed were Amine-citrate, Tris-citrate (pH 8.0), lithium hydroxide, and Poulik (following Murphy et al. 1990). The computer program BIOSYS-1 (Swofford and Selander 1981) was used to calculate allele frequencies, heterozygosity values, departures from Hardy-Weinberg equilibrium, Nei's (1978) and Rogers' (1972) genetic distances (D), and to construct UPGMA phenograms from matrices of Rogers' D (genetic distance matrices, tables of gene frequencies and UPGMA trees are available from the author or from The Field Museum archives). The degree of genetic population structure was estimated by computing Wright's (1978)FST, using a computer program written by G. F. Barrowclough, which corrects for sampling bias due to finite sample size. I also used this program to calculate hierarchical FST values. Populations were grouped for these analyses into samples from isolated fragments (sites 1–3), continuous forest (sites 4–6), fragments and nearby continuous forest (sites 1–6), and across broad geographic distances (sites 1–8).
I tested for concordance between geographic distance and Rogers' D using Mantel's (1967) test in the computer program NTSYS-pc, version 1.40 (Rohlf 1988). This was done by comparing matrices of Rogers' (1972),D for all five species with matrices of straight-line geographic distances between sites (Gabriel networks; Gabriel and Sokal 1969). Because gallery forests may persist along streams as cerrado habitat expands, gallery forests could have served as corridors for avian dispersal between fragments and forest. Thus, the most recent gallery forest connection between forest fragments and continuous forest sites has probably not been a straight-line connection. To account for this possibility, I constructed a second geographic distance matrix considering stream drainages as connections between sites. To take into account multiple comparisons of matrices, I used corrected probability values by dividing the original probability value by the number of comparisons (Douglas and Endler 1982). In the case of the regional-scale geographic analysis, the corrected probability value was 0.05/7 or 0.007, corresponding to a t-value of 2.58. The probability values for the local geographic level also were adjusted accordingly (for both H. cantator and L. amaurocephalus, the number of comparisons possible for these species at the regional and local geographic levels was six and four, respectively). Finally, Mantel's tests were used to compare genetic distance matrices between the five species at the local geographic level (sites 1–6).
Estimating gene flow
Results
Numbers of Loci and Sample Sizes
I collected allozyme data for 23–30 presumptive loci for most individuals of all species (tables of allele frequencies available from the author or from The Field Museum archives). For the three species in which only 23–25 loci were scored, at least 5 additional loci were examined for a portion of the sample (generally 25 individuals); although in these cases, all individuals surveyed were monomorphic, I did not include these other loci in analyses. I excluded one locus from analyses of two species (LA-2 for Myrmeciza hemimelaena and for Glyphorynchus spirurus) because scoring of individuals was inconsistent.
Sample sizes at sites varied (Table 1), and in two species, no samples were collected at one site. Hypocnemis cantator did not occur at site 3 (see below), and Leptopogon amaurocephalus was recorded at site 6 (Bates et al. 1991), but not collected.
Three measures of genetic variation (heterozygosity, percentage of polymorphic loci, and numbers of alleles per locus) for allozyme data from the six Huanchaca region study sites (sites 1–6), with mean sample sizes per locus for each study site
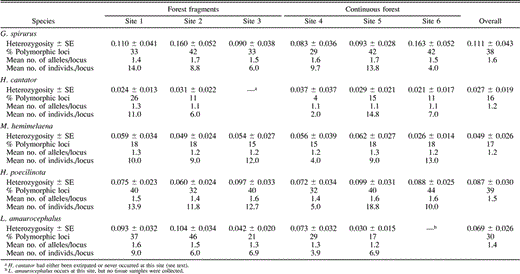
Three measures of genetic variation (heterozygosity, percentage of polymorphic loci, and numbers of alleles per locus) for allozyme data from the six Huanchaca region study sites (sites 1–6), with mean sample sizes per locus for each study site
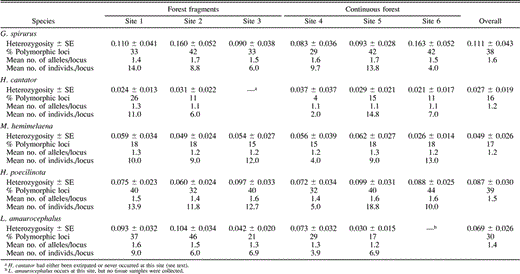
Overall Allozymic Variation
Heterozygosity varied among species (all individuals in a species pooled), from 11.1 ± 4.3 in G. spirurus to 2.7 ± 1.9 in H. cantator (Table 1). The mean percentage of polymorphic loci among sites 1–6 ranged from 16% and 17% for H. cantator and M. hemimelaena, respectively to 38% and 39% in G. spirurus and Hylophylax poecilinota, respectively. Leptopogon amaurocephalus was intermediate with 30% of loci being polymorphic. As with heterozygosity and percentage of polymorphic loci, values for the mean number of alleles per locus were higher in G. spirurus, H. poecilinota, and L. amaurocephalus than in M. hemimelaena and H. cantator (Table 1).
Allozymic Variation at the Local Geographic Scale
Levels of intraspecific allozymic variation at study sites 1–6 were not consistent with predictions for forest fragment populations relative to continuous forest populations. Two of the three standard measures of genetic variation (heterozygosity and number of alleles per locus) showed no reductions in the isolated populations of the forest fragments (site 1–3, Table 1). In fact, heterozygosity was often higher in the forest fragments than in continuous forest. The third measure, the percentage of polymorphic loci, was often lower than average in the three forest fragment populations (particularly for site 3) relative to the continuous forest sites (Table 1). However, low percentages of polymorphic loci for forest fragments were no lower than those for some of the continuous forest populations of the same species.
Hierarchical FST Analyses
FST values at the local level (sites 1–6) were markedly lower than values at the regional level (sites 1–8), and FST values calculated from less inclusive groupings to assess the effects of forest fragmentation at the local level were, with few exceptions, lower still (Table 2). For H. poecilinota, the FST value for forest fragments (sites 1–3) was higher than the FST value for continuous forest sites (sites 4–6, Table 2), a result consistent with forest fragmentation leading to increased genetic isolation of populations. For L. amaurocephalus, the FST value for the forest fragments was slightly higher than that for continuous forest sites. However, in the other three species, FST values for forest fragments were not higher than FST values for continuous forest sites. In G. spirurus, for example, the FST value for the three continuous forest sites is almost twice the value for forest fragments.
Hierarchical FST values (± SE), corrected for small sample size, calculated across several geographic levels. Gs = G. spirurus, Hc = H. cantator, Mh = M. hemimelaena, Hp = H. poecilinota, La = L. amaurocephalus.
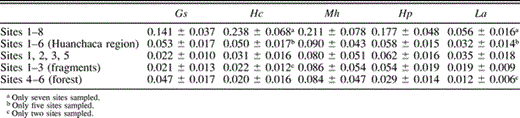
Hierarchical FST values (± SE), corrected for small sample size, calculated across several geographic levels. Gs = G. spirurus, Hc = H. cantator, Mh = M. hemimelaena, Hp = H. poecilinota, La = L. amaurocephalus.
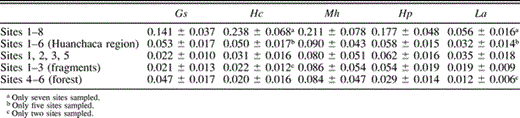
At the regional scale (sites 1–8), FST values ranged from 0.238 in H. cantator to 0.056 in L. amaurocephalus (Table 2). Values for the other three species exceeded 0.140, suggesting substantial differentiation at the regional level, which can be attributed primarily to allele frequency shifts between populations from the Rôndonian region (sites 1–6) and sites from the Inambari region (sites 7 and 8). In H. cantator for instance, large frequency shifts occur between populations from sites 1–6 and those from sites 7 and 8 at GOT-1, PGM-2, and SOD-1. The population at site 8 is apparently fixed for a unique allele at GOT-1. In M. hemimelaena, the samples from sites 7 and 8 are fixed for an allele (“B”) at GPI that appears at a frequency of 0.008 at sites 1–6 (once in the 114 alleles surveyed). Glyphorynchus spirurus populations from sites 7 and 8 exhibit a substantial frequency shift in alleles at LA-1 from those occurring in populations from sites 1–6.
Genetic Distances
At the regional geographic scale (sites 1–8), Mantel's tests on Rogers' (1972) genetic distances resulted in significant t-values (P < 0.01) for four of the five species. Thus, there is a strong correlation between genetic (Rogers' D) and Gabriel (straight-line) geographic distances at this scale for all species except L. amaurocephalus (Table 3). When sites 1–6 were compared alone, none of the five species showed significant t-values, revealing no relationship between genetic distance and the straight-line geographic distances between sites (Table 3). The Mantel's tests comparing local-scale genetic distances and a geographic distance matrix that reflected corridors of riparian vegetation along streams as possible routes of immigration and emigration also resulted in no significant t-values for any of the five species. For the species, Mantel's tests were significant for 2 of 10 comparisons between pairs of matrices of Rogers' (1972)D (between M. hemimelaena and H. cantator; and between H. poecilinota and L. amaurocephalus; Table 4).
t-values from Mantel tests of matrices of Rogers' (1972) genetic distances and geographic distances (km) between sites. Correlation coefficients are given in parentheses. The matrices compared are available from the author. Comparisons at the local scale were made using a Gabriel network of straight-line geographic distances between sites, and by measuring the distance between sites based on connections along streams. For the streamside matrix, birds are assumed to use streamside vegetation as corridors for movement between sites, rather than straight-line paths
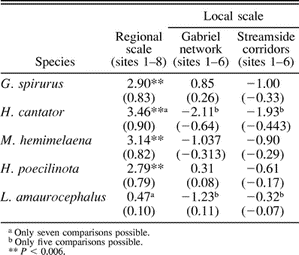
t-values from Mantel tests of matrices of Rogers' (1972) genetic distances and geographic distances (km) between sites. Correlation coefficients are given in parentheses. The matrices compared are available from the author. Comparisons at the local scale were made using a Gabriel network of straight-line geographic distances between sites, and by measuring the distance between sites based on connections along streams. For the streamside matrix, birds are assumed to use streamside vegetation as corridors for movement between sites, rather than straight-line paths
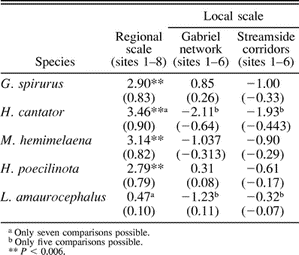
t-values from Mantel tests for matrix comparisons of Rogers' (1972) genetic distances from sites in the Serranía de Huanchaca region (sites 1–6). Correlation coefficients are given in parentheses. The matrices compared are available from the author. Gs = Glyphorynchus spirurus, Hc = Hypocnemis cantator, Mh = Myrmeciza hemimelaena, Hp = Hylophylax poecilinota, La = Leptopogon amaurocephalus.
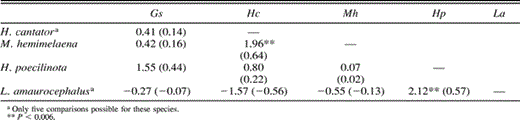
t-values from Mantel tests for matrix comparisons of Rogers' (1972) genetic distances from sites in the Serranía de Huanchaca region (sites 1–6). Correlation coefficients are given in parentheses. The matrices compared are available from the author. Gs = Glyphorynchus spirurus, Hc = Hypocnemis cantator, Mh = Myrmeciza hemimelaena, Hp = Hylophylax poecilinota, La = Leptopogon amaurocephalus.
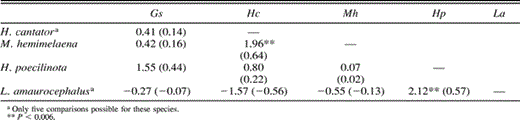
In UPGMA phenograms of Rogers' (1972)D values (not shown), populations from sites 7 and 8 fall outside populations from the Huanchaca region (sites 1–6) for all species except L. amaurocephalus. In L. amaurocephalus, some populations from the Huanchaca region (sites 1–6) appear phenetically closer to populations from sites 7 and 8 than they are to other sites of the Huanchaca. At the local geographic level, we would expect to see continuous forest sites cluster together relative to the forest fragments if forest fragmentation leads to increased differentiation in forest fragments. This occurs only in H. cantator, but not in the other species. For H. cantator and M. hemimelaena, whose distance matrices were significantly correlated using Mantel's test (Table 4), it appears from the phenograms that the significant Mantel's test is due primarily to site 1 being the most divergent of the five sites for which data are available for both taxa. A clear explanation of the other significant between-species Mantel's test, between H. poecilinota and L. amaurocephalus (Table 4), does not emerge from an examination of their respective phenograms (not shown) which are incongruent.
Estimates of Dispersal Using Private Alleles
Values of Nmest for all five species (Table 5) were less than 2.6 immigrants/generation. Jackknifed Nmest values revealed a greater range of values for Nmest, demonstrating that some samples do have disproportionate effects. However, only in G. spirurus and L. amaurocephalus did any jackknifed values exceed 3.0. For H. cantator and M. hemimelaena, Nmest values were less than 1.0. Private alleles for L. amaurocephalus were particularly numerous in forest fragments, and this species had more private alleles overall (14) than the other species (G. spirurus was next highest with 9).
Allozymic data, private alleles, and values of Nmest based on the mean frequency of private alleles [p(1)] for this study and for other studies of passerine birds conducted over local geographic areas. The equations used are those appropriate to the mean sample size of the data set, following Slatkin and Barton (1989). Values in parentheses for the study species give the lowest and highest Nmest from a jackknife analysis in which each population was sequentially removed (see text)
![Allozymic data, private alleles, and values of Nmest based on the mean frequency of private alleles [p(1)] for this study and for other studies of passerine birds conducted over local geographic areas. The equations used are those appropriate to the mean sample size of the data set, following Slatkin and Barton (1989). Values in parentheses for the study species give the lowest and highest Nmest from a jackknife analysis in which each population was sequentially removed (see text)](https://oup.silverchair-cdn.com/oup/backfile/Content_public/Journal/condor/102/4/10.1093_condor_102.4.770/1/m_i0010-5422-102-4-770-t05.gif?Expires=1749660744&Signature=l~rWm7-lkdl70TxRNq~dIaYe~oRQWDnV7cN2xmNbZ2PyADIHFLGVs~ItRowzfW6QRk~soVFOdgTX4eqkJtpgGJRtaZboWmyf4fTFULPydtx9eWhgpPWHQ~6R5KCsYSWj585C2ByHJsiraIyuS8MQtMo~2gMFyhNe-Mj4vbveEboGRuIbsMFplSvYoWFoDRg~QgpeL0ZAaGI2tsg4FAgQCIt5QI3qxLSrBTLhi~ddHMo8hR8BCsnaV-VuqDibg6AeOUbFwkqaDYElhCUc8l--HYz4oobJuuMSjHH~k6w4x3KiTAVBNcIL9fm2HyOlkvWfTShIBQFcD5jT4OCX3mzrJQ__&Key-Pair-Id=APKAIE5G5CRDK6RD3PGA)
Allozymic data, private alleles, and values of Nmest based on the mean frequency of private alleles [p(1)] for this study and for other studies of passerine birds conducted over local geographic areas. The equations used are those appropriate to the mean sample size of the data set, following Slatkin and Barton (1989). Values in parentheses for the study species give the lowest and highest Nmest from a jackknife analysis in which each population was sequentially removed (see text)
![Allozymic data, private alleles, and values of Nmest based on the mean frequency of private alleles [p(1)] for this study and for other studies of passerine birds conducted over local geographic areas. The equations used are those appropriate to the mean sample size of the data set, following Slatkin and Barton (1989). Values in parentheses for the study species give the lowest and highest Nmest from a jackknife analysis in which each population was sequentially removed (see text)](https://oup.silverchair-cdn.com/oup/backfile/Content_public/Journal/condor/102/4/10.1093_condor_102.4.770/1/m_i0010-5422-102-4-770-t05.gif?Expires=1749660744&Signature=l~rWm7-lkdl70TxRNq~dIaYe~oRQWDnV7cN2xmNbZ2PyADIHFLGVs~ItRowzfW6QRk~soVFOdgTX4eqkJtpgGJRtaZboWmyf4fTFULPydtx9eWhgpPWHQ~6R5KCsYSWj585C2ByHJsiraIyuS8MQtMo~2gMFyhNe-Mj4vbveEboGRuIbsMFplSvYoWFoDRg~QgpeL0ZAaGI2tsg4FAgQCIt5QI3qxLSrBTLhi~ddHMo8hR8BCsnaV-VuqDibg6AeOUbFwkqaDYElhCUc8l--HYz4oobJuuMSjHH~k6w4x3KiTAVBNcIL9fm2HyOlkvWfTShIBQFcD5jT4OCX3mzrJQ__&Key-Pair-Id=APKAIE5G5CRDK6RD3PGA)
Discussion
Overall Genetic Variation
Mean heterozygosity in birds has been estimated to be 4.5–6.5% (Barrowclough 1983, Evans 1987). Two study species, Glyphorynchus spirurus (11.1 ± 4.3%) and Hylophylax poecilinota (8.7 ± 3.0%) had levels of mean heterozygosity that were somewhat higher than this, whereas heterozygosity for Hypocnemis cantator (2.7 ± 1.9%) was low. Values for the other two species, Myrmeciza hemimelaena (4.9 ± 2.6%) and Leptopogon amaurocephalus (6.9 ± 2.6%) were typical. The percentage of polymorphic loci and the number of alleles per locus showed similar patterns, with G. spirurus, H. poecilinota, and L. amaurocephalus exhibiting greater overall variation than H. cantator and M. hemimelaena (Table 1). As with levels of heterozygosity, the mean percentages of polymorphic loci at the local level (sites 1–6) in G. spirurus (38%), H. poecilinota (39%), and L. amaurocephalus (30%) also were higher than the 22–24% average determined for birds (Corbin 1987, Evans 1987).
Effects of Forest Fragmentation on Genetic Structure
I predicted that if gene flow was sufficiently low among populations from forest fragments and continuous forest relative to gene flow among sites in continuous forest, then genetic variation should be lost in the forest fragment populations relative to the genetic variation in continuous forest populations. In addition, increased genetic differentiation also may have developed in forest fragment populations. Although data sets for all five species exhibit some aspects consistent with genetic effects due to forest fragmentation, shared patterns of decreased genetic variation and increased genetic differentiation in forest fragment populations were not observed. In every analysis (measures of genetic variation, hierarchical FST, Mantel's tests), one to several species did not exhibit the predicted pattern, and it was not always the same species that deviated (Table 6).
Summary of the agreement with predictions concerning the genetic effects of forest fragmentation for allozymic variation and differentiation in five Amazonian forest birds from the Serranía de Huanchaca region (sites 1–6). Y = data for the species match predictions, N = data do not match predictions. Gs = G. spirurus, Hc = H. cantator, Mh = M. hemimelaena, Hp = H. poecilinota, La = L. amaurocephalus.
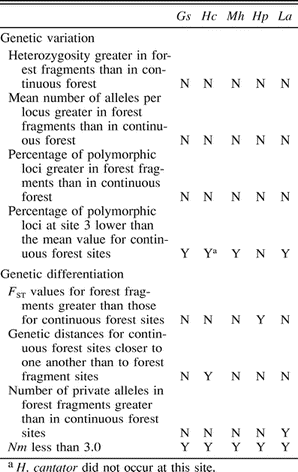
Summary of the agreement with predictions concerning the genetic effects of forest fragmentation for allozymic variation and differentiation in five Amazonian forest birds from the Serranía de Huanchaca region (sites 1–6). Y = data for the species match predictions, N = data do not match predictions. Gs = G. spirurus, Hc = H. cantator, Mh = M. hemimelaena, Hp = H. poecilinota, La = L. amaurocephalus.
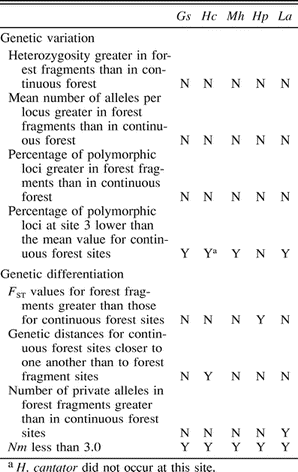
In spite of a lack of a pattern common to all species, aspects of these data sets that are consistent with forest fragmentation affecting genetic structure merit consideration because “all-or-none” predictions might be too strict. These aspects include lower than average percentages of polymorphic loci in the smallest forest fragment (site 3) and increased genetic differentiation among forest fragment populations relative to continuous forest populations in some species. I also mention the absence of H. cantator from site 3 here, because this site is the one that would be predicted to lose species first under forest fragmentation models.
The percentage of polymorphic loci has been shown theoretically (Nei et al. 1975) and empirically (Leberg 1992) to be the most sensitive of the three common measures of genetic variation (heterozygosity and mean number of alleles per locus are the other two) to population declines. Percentages of polymorphic loci were lower in site 3 samples than the averages for continuous forest sites for three of the four species found there (G. spirurus, M. hemimelaena, and L. amaurocephalus,Tables 1 and 6). Site 3 is the smallest forest fragment, and all things being equal, these populations would be expected to lose genetic variation more rapidly than those in the larger fragments or continuous forest. However, in each of the three species, a population from a continuous forest site also exhibited a low percentage of polymorphic loci.
The absence of H. cantator from site 3 is noteworthy, because this species favors treefalls, light gaps, and forest edge more so than other study species (Stotz and Bierregaard 1989). These habitats were as plentiful at site 3 as at other sites (pers. observ.). This species also is one of a few forest birds that persists in 10-ha man-made fragments near Manaus, Brazil (Stouffer and Bierregaard 1995), and it was common at the other five study sites in the Serranía de Huanchaca region (Bates and Parker 1998). Furthermore, absence of H. cantator at site 3 contrasted with the abundance of another thamnophilid, Dysithamnus mentalis (Plain Antvireo) at the site. Dysithamnus mentalis resembles H. cantator in foraging ecology and morphology (pers. observ.), and it was one of the most common understory species in the fragment; yet, it was unrecorded in extensive surveys at the other five sites (Bates and Parker 1998). It is possible that H. cantator has gone extinct in the smallest forest fragment, although there is no indication that this would be for genetic reasons.
Different aspects of the data suggest increased genetic differentiation in the forest fragments for three of the species. The FST value calculated for the three forest fragments in H. poecilinota is higher than the value for continuous forest populations (Table 2). This is also true in L. amaurocephalus, however, standard errors associated with these values suggest that they are not different. Forest fragment populations in L. amaurocephalus also have a high number of private alleles relative to those from the one continuous forest site with enough individuals to use in the estimations. This is also true in H. cantator. In addition, branching patterns in the UPGMA phenogram for H. cantator (not shown), show the forest fragment populations to be more differentiated from one another (basal) than continuous forest populations. In spite of these observations, the allozymic data do not clearly suggest that forest fragmentation has affected genetic structure for any of the species.
Genetic Structure Within Sedentary Neotropical Taxa
The second prediction was that genetic differentiation in the species that I studied would be greater than in comparable less sedentary temperate zone taxa. Hackett and Rosenberg (1990) summarized intraspecific genetic distance data for allozyme studies of sedentary Neotropical species and emphasized that these genetic distances were often greater than values reported between congeneric species of temperate birds (Barrowclough 1980). Mean Nei's (1978) distance values between the Huanchaca sites (1–6) and sites 7 and 8 are similar to intraspecific values for other Neotropical birds (Hackett and Rosenberg 1990). At this regional scale, all species were not equally differentiated, as L. amaurocephalus exhibited much less differentiation among sites than the other four species. This species had the lowest FST value when sites 1–8 were compared (Table 2), and it was the only species that did not show a significant Mantel's test when genetic distances were compared to geographic distances for sites 1–8 (Table 4). In addition, it was the only species in which population samples from sites 7 and 8 did not fall outside the cluster of sites 1–6 in a UPGMA phenogram (not shown). That L. amaurocephalus populations from the two areas of endemism (Inambari and Rondônia) are much less differentiated than are populations of the other study species suggests that L. amaurocephalus populations may be connected through greater gene flow between these sites. Alternatively, L. amaurocephalus may have spread across this region more recently, and its populations have not had time to differentiate. These allozyme results are mirrored in mitochondrial DNA variation (Bates 1993).
Genetic Structure at the Local Geographic Level
My results also indicate substantial levels of genetic differentiation among populations at the local scale, even among sites in continuous forest. Avian allozymic studies are not often conducted at such scales, because most birds are thought to be good dispersers, and levels of allozymic divergence uncovered by many regional avian studies have been low. Thus, detecting meaningful allozymic structure at a local level in birds appears unlikely.
In another region of the Amazon Basin, Capparella (1987, 1988) demonstrated significant genetic structure in five of six Amazonian birds whose ranges were bisected by the Amazon River or its tributary, the Napo River. He also presented data for five of these species from three sites within 85 km of one another that were not separated by a major river. Comparing my results to those of Capparella permits assessment of local allozymic structure in Amazonian birds from two different regions. Capparella reported levels of heterozygosity and percentage of polymorphic loci similar to values published for other birds (Barrowclough 1983, Corbin 1987). In my study, two species had higher than average levels of overall genetic variation, and three species had average or below average levels of variation.
I found local-level FST values to indicate substantial allozymic differentiation even between continuous forest sites (4–6). FST values among these sites ranged from 0.019 in L. amaurocephalus to 0.086 in M. hemimelaena (Table 2). Capparella (1987) also reported local-level FST values for five species that, with the exception of the Black-faced Antbird (Myrmoborus myotherinus, FST = 0.002), exceeded 0.01 and ranged up to 0.098 in the Golden-headed Manakin (Pipra erythrocephala). Capparella's value for his three population samples of G. spirurus (0.073) exceeded the value (0.047) that I calculated for sites 4–6 in this species. These values are comparable to those between different subspecies and from sites hundreds of kilometers apart in other avian studies (Barrowclough and Johnson 1988). From a subset of Hackett's (1993) allozyme data (her Table 1), I calculated FST values for population samples of a subspecies of Bicolored Antbird (Gymnopithys leucaspis castanea) collected at the same three Peruvian sites Capparella studied. The FST value for these populations also is high (0.291 ± 0.089). Thus, in three different studies, Amazonian suboscines of the forest understory exhibit high levels of genetic differentiation, even over small geographic distances. It is not yet known whether this level of genetic differentiation also occurs in other avian taxa in the Amazon such as non-passerines and oscine passerines, or in other ecological groups of birds such as canopy-inhabiting species. High levels of differentiation are found in Amazonian rodents (Patton et al. 1994).
Estimated Levels of Gene Flow at the Local Geographic Scale
Estimates of gene flow (Nm) in this study are among the first for any Neotropical birds. Although all samples used in the estimates are from sites within 200 km of one another, Nm was found to be less than 1.0 for H. cantator and M. hemimelaena, and less than 3.0 in the other three species (Table 5). These estimates are low compared to Nm values reported in other avian studies. For instance, Grant and Little (1992) reported Nm (calculated from private alleles) to be 9.1 for Greywing Francolins (Francolinus africanus) in a 60-km area, and Randi and Alkon (1994) reported Nm values of 6–12 for Chukars (Alectoris chukar) from five sites in Israel within a 300-km area. Local-level estimates of Nm for other passerine birds are lacking, but I calculated values for several species from the literature (Table 5). These estimates of Nm are, with one exception (G. spirurus from Peru), also higher than values in my study.
Estimates of Nm greater than 1.0 have been considered sufficient to maintain a panmictic population (Franklin 1980, Slatkin 1985); however, the generality of this value as a cutoff has been questioned (Varvio et al. 1986, Wright 1987). Judging from Table 5 and from other allozyme studies (Gavin et al. 1992, Johnson and Marten 1992, Groth 1993), values based on private alleles rarely are less than 3.0 in birds, even when samples come from geographically distant areas and from morphologically differentiated populations. Thus, the values of Nm I observed suggest gene flow at the local scale could be low enough to permit differentiation of populations.
Implications of Genetic Structure in Amazonian Birds
I found little support for the prediction that natural forest fragmentation affects genetic structure as measured by allozymes; however, all species (with the possible exception of L. amaurocephalus) exhibited substantial levels of genetic differentiation at both the regional and local geographic levels relative to most birds. Mitochondrial DNA, which evolves at a faster rate than allozymes, provides evidence that populations of three of the species (the three antbirds) may indeed exhibit genetic effects related to the forest fragmentation studied here (Bates 1993).
In addition to providing genetic information within populations, Avise (1989) has suggested that molecular data can be useful for conservation biology because they can identify “phylogenetic discontinuities within and among species.” Patterns of allozymic divergence at the regional level are consistent with a genetic break between the Rondônian and Inambari regions for four of the five species. This high level of differentiation is seen in mitochondrial DNA data for the same individuals (Bates 1993), and these levels of divergence are as great as those found between many morphologically differentiated bird species. Substantiation of regional “phylogenetic discontinuities” will require sampling additional populations between those I studied; however, my data are consistent with results for other Amazonian species in which genetic differentiation is much greater than that among comparable populations of many other birds. The sedentary nature of these birds may be the primary reason for this structure; however, an additional factor may be a relatively longer history in much of their present day distributions (compared to temperate species, Dobzhansky 1950). From a conservation perspective, if the high species diversity of tropical regions such as Amazonia is mirrored by levels of genetic structure similar to what I report, we may be underestimating the biotic richness of regions we already suspect are among the richest on earth.
Acknowledgments
Field work in Bolivia was funded by grants to J. V. Remsen from J. H. McIlhenny, and by the National Geographic Society (grant #4089–89), with additional support from P. Machris. I thank all the many people in Bolivia for their support and guidance, especially the staff of the Parque Nacional Noel Kempff Mercado under the direction of N. Ruiz and G. Cerro Grande; A. Moscoso V. of the Dpto. de Vida Silvestre, Centro de Desarrollo Forestal; T. R. de Centurión and her staff at the Museo de Historia Natural “Noel Kempff Mercado,” and E. Quintela and his family. For help in the field, I thank A. P. Capparella, M. D. Carreño, A. Castillo, T. J. Davis, M. C. Garvin, M. and P. Isler, H. Justiniano, C. A. Marantz, T. A. Parker III, G. H. Rosenberg, M. Sánchez S., D. C. Schmitt, C. G. Schmitt, and A. Yépez. Lab work was conducted in the Museum of Natural Science at Louisiana State University (LSU), with funding from the Alexander Wetmore Fund of the American Ornithologists' Union, the LSU chapter of Sigma Xi, and an NSF grant to R. M. Zink. J. V. Remsen, R. M. Zink, and S. J. Hackett commented on earlier drafts of the manuscript. Finally, I thank the late Theodore A. Parker III for his enthusiasm and encouragement during the course of this project.
Literature Cited
Author notes
Present address: Zoology Department, Field Museum of Natural History, Roosevelt Road at Lake Shore Drive, Chicago, IL 60605, [email protected]