-
PDF
- Split View
-
Views
-
Cite
Cite
Ryo Sasai, Fumitaka Aoto, Takuya Fujimura, Spectroscopic change of rhodamine 3B incorporated in layered titanate/decyltrimethylammonium hybrid solid by exposure of phenol vapors, Chemistry Letters, Volume 54, Issue 4, April 2025, upaf056, https://doi.org/10.1093/chemle/upaf056
- Share Icon Share
Abstract
Phenol, a critical raw material in resins and pharmaceuticals, presents environmental and health risks owing to its high volatility and water solubility. Rapid, on-site detection methods are urgently needed but remain underdeveloped. This study explores the use of decyltrimethylammonium-modified layered titanate hybridized with rhodamine 3B (R3B/C10TMA/LT) as a solid material for phenol detection through spectroscopic changes. Phenol adsorption increased the interlayer spacing from 2.37 nm to 2.71 nm, with an adsorption capacity of 5.2 mmol/g-hybrid (486 mg/g-hybrid). Significant spectroscopic changes, including diffuse reflectance darkening and pronounced photoluminescence quenching, were observed, resulting from interactions between phenol's π-conjugated system and the hybrid material. In contrast, cyclohexanol, a non-aromatic compound, showed minimal interaction, confirming the material's specificity for phenol. Under high humidity, phenol detection remained effective despite a reduced adsorption capacity (3.1 mmol/g-hybrid), demonstrating its robustness under varying conditions. These results underscore the potential of R3B/C10TMA/LT for atmospheric phenol sensing. Future research should focus on developing this material into films or bulk devices for practical applications.
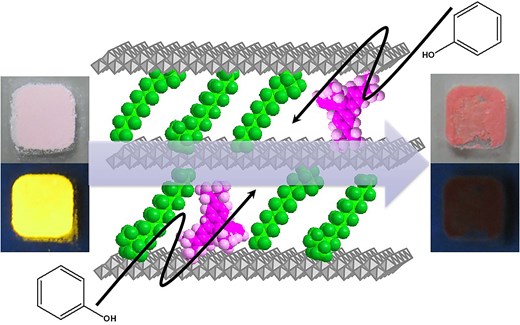
Phenol is a key raw material in various chemical products, including resins and pharmaceuticals. However, its high volatility and water solubility contribute to environmental pollution and chemical hypersensitivity, necessitating effective quantitative detection techniques. Currently, colorimetric methods and liquid chromatography-mass spectroscopy are widely used for accurate phenol quantification. However, these methods involve complex pretreatment procedures and require expensive equipment. Additionally, while several researchers have proposed various detection methods,1 these approaches are unsuitable for rapid, on-site quantitative detection in diverse environmental settings. Solid materials, such as detector tubes, which exhibit spectroscopic changes upon phenol adsorption, offer a promising solution for on-site detection. Such materials must possess high phenol adsorption capacities to be effective. Organic-modified ion-exchangeable layered inorganic compounds (organo-IELICs) are particularly promising as phenol adsorption materials.2–4
By incorporating detection functionalities into organo-IELICs with high adsorption capabilities, it becomes feasible to develop solid sensing materials for various toxic organic compounds, such as phenol. Building on this concept, researchers have extensively studied organo-IELIC/dye hybrid materials for their selective detection abilities toward diverse molecules and ions.5 Previously, the authors reported that butanesulfonate-modified layered double hydroxide hybridized with anionic fluorescein dye (AFD/C4S/LDH) can quantitatively measure relative humidity through changes in photoluminescence (PL) intensity.6,7 Furthermore, this AFD/C4S/LDH hybrid material demonstrated significant PL enhancement when exposed to ammonia gas concentrations exceeding 3 ppm at high relative humidity (above 80%), while the PL intensity decreased linearly with increasing nitrogen dioxide gas concentrations. Similar to the AFD/C4S/LDH hybrid materials, we have also investigated the effect of adsorption of water molecules and basic gas molecules such as ammonia on the PL properties of decyltrimethylammonium-modified layered titanates with rhodamine 3B (R3B/C10TMA/LT).8 In this study, we investigated the phenol detection performance of R3B/C10TMA/LT by studying changes in spectroscopic properties such as optical absorption and emission characteristics when phenol molecules with a high pKa (9.95) were adsorbed on R3B/C10TMA/LT.
The R3B/C10TMA/LT hybrid powder was synthesized following a previously reported method.9 For phenol vapor exposure experiments, the R3B/C10TMA/LT hybrid powder was sealed in a container with a phenol crystal sample and left to stand for 24 h at 30 °C, as illustrated in Fig. 1a. To examine the influence of water vapor, water was added to the container under the same conditions (see Fig. 1b). The R3B/C10TMA/LT hybrid powder was characterized using XRD (MiniFlex II powder X-ray diffractometer with Ni-filtered CuKα, 30 kV, 15 mA; Rigaku, Tokyo, Japan), diffuse reflectance spectroscopy (V-670 UV-Vis spectrophotometer attached to an integrating sphere system (ISN-723), JASCO, Tokyo, Japan), and PL spectroscopy (FP-6600 spectrofluorometer, JASCO, Tokyo, Japan).
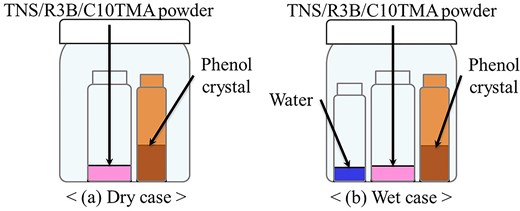
Figure 2 presents the XRD patterns of the R3B/C10TMA/LT hybrid powder before and after phenol adsorption (Fig. 1a). The bottom diffraction (0k0) of the hybrid powder shifts to a lower angle following phenol adsorption, indicating an increase in the TNS interlayer spacing from 2.37 nm to 2.71 nm. This confirms that phenol was adsorbed within the interlayer of the R3B/C10TMA/LT hybrid powder. A comparison of the hybrid powder's weight before and after phenol adsorption revealed an adsorption capacity of 5.2 mmol/g-hybrid (486 mg/g-hybrid). Figure 3 displays the diffuse reflection and PL spectra (excitation wavelength: 520 nm) before and after phenol adsorption. The diffuse reflectance spectrum exhibited a hyperchromic effect upon phenol adsorption, while the PL spectrum showed significant quenching and a marked decrease in PL quantum yield from 0.78 to 0.02. These findings suggest that the R3B/C10TMA/LT hybrid powder can detect phenol molecules in the gas phase through color and luminescence changes.
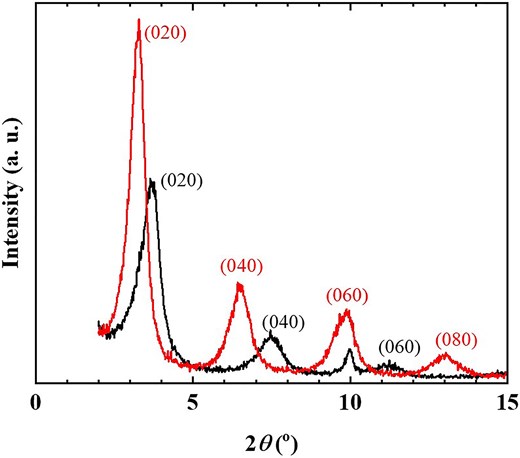
XRD patterns of R3B/C10TMA/LT hybrid powder before (black solid line) and after (red solid line) phenol adsorption experiment using Fig. 1a setting.
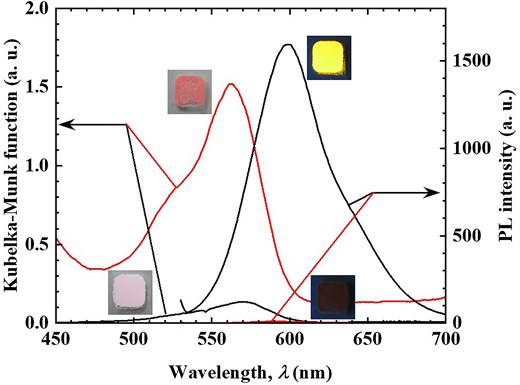
Diffuse reflectance (left axis) and PL (right axis) spectra of R3B/C10TMA/LT hybrid powder before (black solid line) and after (red solid line) phenol adsorption experiment using Fig. 1a setting.
This remarkable spectroscopic change is likely associated with the π-conjugated system of phenol. To investigate this, the adsorption of cyclohexanol, a non-aromatic cyclic alcohol, was examined under the same conditions as those in Fig. 1a. The amount of cyclohexanol adsorbed was estimated at 0.24 mmol/g-hybrid (24.0 mg/g-hybrid) based on the mass change before and after adsorption, significantly lower than that of phenol, yet adsorption was still observed. Figure 4 presents the diffuse reflection and PL spectra (excitation wavelength: 520 nm) before and after cyclohexanol adsorption. Although a slight increase in diffuse reflectance intensity was noted, no significant darkening effect was observed, unlike in the case of phenol. Similarly, the PL spectrum exhibited no substantial quenching, and the PL quantum yield remained largely unchanged (0.75). These minor variations can be attributed to solvent effects. Despite the relatively large adsorption quantity, these results suggest that cyclohexanol does not interact with R3B and thus does not induce any spectroscopic changes. These findings indicate that the interaction between the π-conjugated system of phenol and R3B plays a crucial role in altering the spectral properties of R3B due to phenol adsorption. Given the high pKa value of cyclohexanol (pKa = 16), it is unlikely to exist as an interlayer anion or form ion pairs with R3B, ruling out electrostatic interactions. In contrast, phenol has a lower pKa value (9.95), and considering that TNS provides a basic environment, phenol may exist as a phenolate ion in the interlayer. If phenolate ions are responsible for the observed spectral changes, similar effects should occur when water molecules are adsorbed, even at low phenol concentrations. To test this hypothesis, phenol adsorption experiments were conducted under high-humidity conditions, as shown in Fig. 1b. Figure 5 presents the diffuse reflection and PL spectra (excitation wavelength: 520 nm) before and after phenol adsorption under high humidity. Regardless of humidity, the diffuse reflection spectrum exhibited a darkening effect, and significant PL quenching was observed. These results qualitatively confirm that this material can detect phenol molecules in the atmosphere irrespective of humidity. However, the amount of phenol adsorbed under high-humidity conditions was measured at 3.1 mmol/g-hybrid (293.5 mg/g-hybrid), lower than the adsorption capacity under dry conditions. This finding demonstrates that the R3B/C10TMA/LT hybrid powder maintains high phenol detection sensitivity even in humid environments.
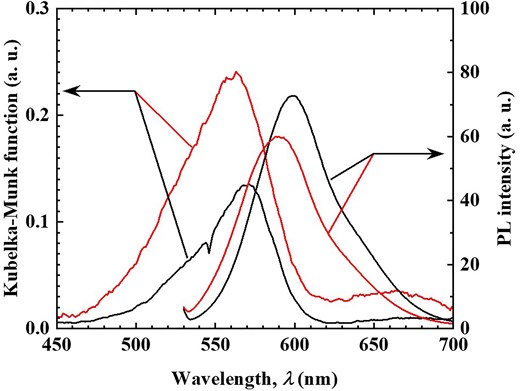
Diffuse reflectance (left axis) and PL (right axis) spectra of R3B/C10TMA/LT hybrid powder before (black solid line) and after (red solid line) cyclohexane adsorption experiment using Fig. 1a setting.
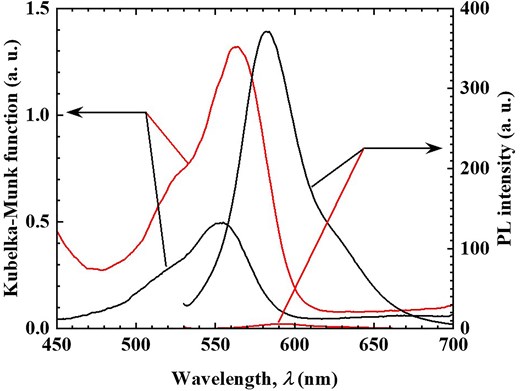
Diffuse reflectance (left axis) and PL (right axis) spectra of R3B/C10TMA/LT hybrid powder before (black solid line) and after (red solid line) phenol adsorption experiment under water vapor using Fig. 1a setting.
In summary, the R3B/C10TMA/LT hybrid powder detects phenol molecules in the atmosphere by altering its spectroscopic properties. The detection mechanism is likely driven by the interaction between photoexcited R3B and phenolate ions. To fully assess its applicability, further investigation is needed to determine whether the hybrid powder enables quantitative phenol detection and how its sensitivity is influenced by relative humidity.
Funding
This work was partially supported by two programs (2010 and 2013) funded by the Shimane Industrial Promotion Foundation and Cosmetology Research Foundation.
References
Author notes
Conflict of interest statement. None declared.