-
PDF
- Split View
-
Views
-
Cite
Cite
Paramjeet Singh Paliyal, Surajit Mondal, Samar Layek, Piyush Kuchhal, Jitendra Kumar Pandey, Automatic solar tracking system: a review pertaining to advancements and challenges in the current scenario, Clean Energy, Volume 8, Issue 6, December 2024, Pages 237–262, https://doi.org/10.1093/ce/zkae085
- Share Icon Share
Abstract
An automatic solar tracking system is an approach for optimizing the generation of solar power and modifying the angles and direction of a solar panel by considering changes in the position and path of the sun. The performance status of an automatic solar tracking system depends on various factors, including its design, location, and maintenance or repairs. The solar energy from the sun that the Earth intercepts is approximately 1.8 × 1011 MW, which is thousands of times greater than the intensity at which the Earth now uses all other commercially available energy sources combined. Currently, research into automatic solar trackers is on the rise, as solar energy is abundant in nature, but its use in a highly efficient way is still lacking. This paper provides a detailed literature review and highlights some key advancements and challenges associated with state-of-the-art automatic solar tracking systems. The performance of the dual-axis photovoltaic tracking system outperforms that of the stationary systems by more than 27% based on the overall system efficiency. Under diverse weather conditions, the efficiency of the scheduled-based solar tracking systems was enhanced by 4.2% compared with that of the light-dependent resistor-based solar trackers.
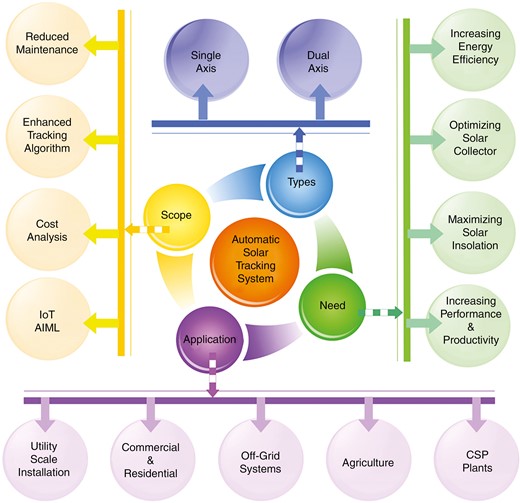
1. Introduction
In the current scenario, the load demand for energy increases daily, and the current resources for feeding the electrical load demands are not sufficient. The electrical load fluctuates throughout the day, and to fulfil these energy demands, renewable energy is highly important because research on renewable energy is trending. Fossil fuels release greenhouse gases such as carbon dioxide, trapping heat and causing global warming. Renewables such as solar, wind, and geothermal energy produce no greenhouse gases that mitigate climate change impacts. As renewable energy is abundant in nature in the form of sunlight and wind, the use of these renewable resources for power production makes the power system efficient and smart. This decentralization makes the grid less vulnerable to single-point failures and improves overall resilience. Solar panels convert sunlight directly into electricity for utility-scale power plants, and solar thermal systems capture heat from the sun for water heating, space heating, and industrial processes. To increase the efficiency of solar panels, a solar tracking strategy is used by automatically adjusting the angle of the panels throughout the day to directly face the sun, and trackers can generate 20%–40% more energy than statically mounted panels can generate [1]. This approach can be particularly advantageous in regions with ample sunshine that benefits the most from increased sun exposure. When space is limited, maximizing energy per unit area becomes crucial; if electricity costs vary depending on the time of day, trackers can optimize generation during peak periods.
An automatic solar tracking system (STS) is an emerging technology that rotates a solar panel or solar concentrator to various positions throughout the day by monitoring the current position and path of the sun. The main aim of any automatic STS is to maximize the amount of sunlight that the solar concentrator or module will receive, resulting in the maximization of the overall energy outputs of the system. Solar tracking can be performed in two ways: single-axis tracking and double-axis tracking. Here, the single-axis STS is used to adjust the angle and position of the solar panel or collector in one direction following the path of the sun (often east to west), and the dual-axis tracker is used to adjust the solar panel and collector in two directions. Many studies on solar energy have analysed solar energy markets in India, as it is the second most populated nation in the world and is experiencing a continuous increase in electric power demand. Consequently, the government is exploring renewable energy sources as viable substitutes for traditional supplies [2].
Currently, India’s utilization of renewable energy is positioned fifth globally and is increasing, comprising 13.22% of the aggregate energy consumption in the form of solar energy. Hence, in this study, the current investigation involved the modelling of any substantial 20 MW solar photovoltaic (PV) power facility to evaluate its technological and economic efficacy. This evaluation was conducted via the system advisor model (SAM) [3]. In 2022, the global market for solar trackers was valued at $3.2 billion. By 2033, the market is expected to grow, reaching a value of approximately $7.2 billion. It is anticipated that solar tracker sales will increase in tandem with the growing awareness of energy conservation and the shift towards renewable energy sources. A variety of electrical components are utilized by solar trackers, including actuators and sensors, to assist the solar collector in concentrating sunlight to capture energy [4]. This study presents a comprehensive analysis of various solar tracking technologies, categorizing them based on several key parameters, such as the number of axes they utilize, the activity level of the tracking unit, the control strategies employed, and the specific tracking methodologies implemented. This literature review helps readers understand the latest advancements in STS and the variability of their parameters. It also traces the path of the sun and shows how solar insolation changes over time, which is applicable to any latitude or longitude. Additionally, this review compares data based on criteria such as the axis of rotation, tracking method, accuracy, and energy efficiency.
Figure 1 shows the network visualization of the keywords related to STS research papers. This network visualization of the similarities shows how research on STS is trending to optimize and increase the performance of solar panels and solar concentrators. With the help of this figure, how all the keywords are connected can be determined. The maximum number of keywords related to STS used is the maximum number of power point trackers used, which directly indicates that researchers are working more on maximum power point tracking (MPPT), as it is the most repeated keyword in STS-related research articles in the Scopus database [5, 6]. This analysis has been performed by using the visualization of similarities (VOS) viewer and will be beneficial for the reader to know where the research in STS is trending and tilting.
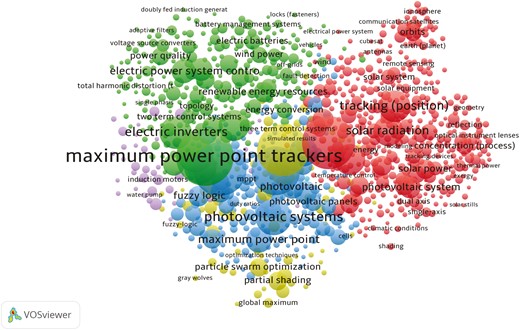
Network visualization of the similarities for the Scopus data, based on keywords related to the STS.
Figure 2 shows that the number of publications per year based on Scopus data with the keywords ‘solar tracking system’, ‘photovoltaics’, and ‘renewable energy’ has increased annually and continues to rise. These data are shown from 2000 to 2024 (June). After conducting the statistical analysis, the plotted figure shows how the research in solar tracking technology is increasing daily to make the solar panels and collectors more efficient and optimize them. As in the current scenario, the energy demand continues to increase, and researchers are continuously investigating renewable energy sources, which can be converted to electricity and utilized to power electrical equipment; this is an abundant and environmentally friendly energy source that meets the criteria. If 0.16% of the Earth’s surface is exploited for solar energy, 20 terawatts of power are produced, which is double what the planet consumes in fossil fuels [7, 8].
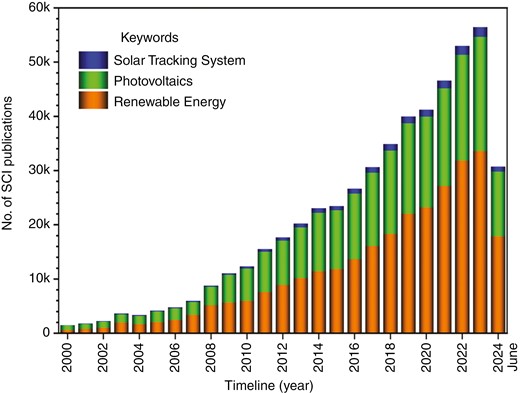
Science Citation Index (SCI) data for the number of publications per year by the keywords ‘solar tracking system’, ‘photovoltaics’, and ‘renewable energy’.
In Fig. 3, an overview of the structural diagram is shown for illustration in this manuscript. Section 1 provides a detailed overview of an automatic STS. Section 1.1 shows the important solar tracking parameters considered. Section 1.2 presents previous similar works on the study of STS. Section 1.3 shows how the sun’s path is traced for applications in solar tracking. Section 2 presents the history of the STS, which describes how solar tracking was used in ancient times. Section 3 presents the classification of the STS, and a comparative analysis of different solar tracking technologies is shown with key findings and performance indices. Section 4 presents the results and discussion based on the solar tracking parameters of the different technologies. Section 5 presents the conclusion and future scope, and Section 6 provides references.
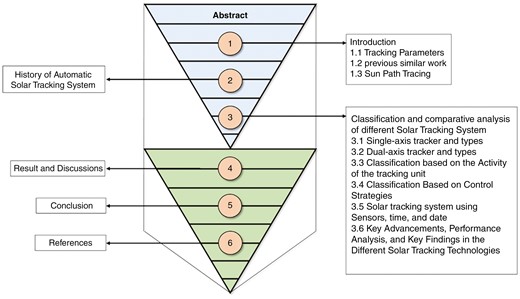
Overview structure diagram and illustration of the study sequence.
1.1 Solar tracking parameters
The azimuth and elevation angles are the main sun-tracking parameters that are essential for maximizing solar energy acquisition. Solar panels are directed toward the sun as it moves across the sky during the day because the azimuth angle tells us the horizontal direction of the sun concerning the observer’s position on Earth. In contrast, the elevation angle refers to the vertical angle of the sun above the horizon and is used to tilt solar panels to maximize the amount of solar energy that reaches them [9]. STS can guarantee that panels are always positioned optimally for maximum energy production by dynamically adjusting these angles based on variables such as the time of day, date, latitude, and even weather. This greatly improves the efficiency and output of solar power installations. Table 1 presents the parameters that should be considered before framing the solar tracking strategy.
Factors to be considered . | Description . | References . |
---|---|---|
Tracking method | To determine the tracking method axis or dual axis: • 25%–30% performance gain for a single axis. • Bumps up the performance by another 5%–10% in dual axis. | [10] |
Tracking accuracy | • Determined by energy demands, available solar resources, and precision requirements. • Higher precision ranging from 0.5° to 1° often leads to increased energy output but may increase complexity and cost. | [11] |
Tracking range | • Single axis typically covers 180° from east to west. • Dual axis has a greater range for tracking azimuth and elevation of the sun. • 180°–360° for azimuth, allowing for full east‒west tracking. • The elevation range can span from 0° to 90°. | [12] |
Mounting type | • Fixed axis in which panels are tilted along a fixed axis. • Polar-aligned where panels are aligned parallel to the Earth’s axis. | [13] |
Sensor type | Sensors like LDRs, photodiodes, or digital sun sensors provide feedback for the tracking system to adjust panel position. | [14] |
Control system | The controller and software receive sensor information to adjust panel position using motors or actuators. | [15] |
Power supply | It can be powered by the panels themselves or a separate source like batteries or smaller solar panels. | [16] |
Durability and resistance | Materials and construction should withstand various weather conditions including wind, rain, snow, and temperature fluctuations. | [17] |
Maintenance and servicing | Accessibility to components, lubrication needs, recalibration requirements, and overall ease of maintenance should be considered during design. | [18] |
Cost and budget | Installation costs, ongoing maintenance, and potential return on investment through increased energy production. | [19] |
Factors to be considered . | Description . | References . |
---|---|---|
Tracking method | To determine the tracking method axis or dual axis: • 25%–30% performance gain for a single axis. • Bumps up the performance by another 5%–10% in dual axis. | [10] |
Tracking accuracy | • Determined by energy demands, available solar resources, and precision requirements. • Higher precision ranging from 0.5° to 1° often leads to increased energy output but may increase complexity and cost. | [11] |
Tracking range | • Single axis typically covers 180° from east to west. • Dual axis has a greater range for tracking azimuth and elevation of the sun. • 180°–360° for azimuth, allowing for full east‒west tracking. • The elevation range can span from 0° to 90°. | [12] |
Mounting type | • Fixed axis in which panels are tilted along a fixed axis. • Polar-aligned where panels are aligned parallel to the Earth’s axis. | [13] |
Sensor type | Sensors like LDRs, photodiodes, or digital sun sensors provide feedback for the tracking system to adjust panel position. | [14] |
Control system | The controller and software receive sensor information to adjust panel position using motors or actuators. | [15] |
Power supply | It can be powered by the panels themselves or a separate source like batteries or smaller solar panels. | [16] |
Durability and resistance | Materials and construction should withstand various weather conditions including wind, rain, snow, and temperature fluctuations. | [17] |
Maintenance and servicing | Accessibility to components, lubrication needs, recalibration requirements, and overall ease of maintenance should be considered during design. | [18] |
Cost and budget | Installation costs, ongoing maintenance, and potential return on investment through increased energy production. | [19] |
LDR, light-dependent resistor.
Factors to be considered . | Description . | References . |
---|---|---|
Tracking method | To determine the tracking method axis or dual axis: • 25%–30% performance gain for a single axis. • Bumps up the performance by another 5%–10% in dual axis. | [10] |
Tracking accuracy | • Determined by energy demands, available solar resources, and precision requirements. • Higher precision ranging from 0.5° to 1° often leads to increased energy output but may increase complexity and cost. | [11] |
Tracking range | • Single axis typically covers 180° from east to west. • Dual axis has a greater range for tracking azimuth and elevation of the sun. • 180°–360° for azimuth, allowing for full east‒west tracking. • The elevation range can span from 0° to 90°. | [12] |
Mounting type | • Fixed axis in which panels are tilted along a fixed axis. • Polar-aligned where panels are aligned parallel to the Earth’s axis. | [13] |
Sensor type | Sensors like LDRs, photodiodes, or digital sun sensors provide feedback for the tracking system to adjust panel position. | [14] |
Control system | The controller and software receive sensor information to adjust panel position using motors or actuators. | [15] |
Power supply | It can be powered by the panels themselves or a separate source like batteries or smaller solar panels. | [16] |
Durability and resistance | Materials and construction should withstand various weather conditions including wind, rain, snow, and temperature fluctuations. | [17] |
Maintenance and servicing | Accessibility to components, lubrication needs, recalibration requirements, and overall ease of maintenance should be considered during design. | [18] |
Cost and budget | Installation costs, ongoing maintenance, and potential return on investment through increased energy production. | [19] |
Factors to be considered . | Description . | References . |
---|---|---|
Tracking method | To determine the tracking method axis or dual axis: • 25%–30% performance gain for a single axis. • Bumps up the performance by another 5%–10% in dual axis. | [10] |
Tracking accuracy | • Determined by energy demands, available solar resources, and precision requirements. • Higher precision ranging from 0.5° to 1° often leads to increased energy output but may increase complexity and cost. | [11] |
Tracking range | • Single axis typically covers 180° from east to west. • Dual axis has a greater range for tracking azimuth and elevation of the sun. • 180°–360° for azimuth, allowing for full east‒west tracking. • The elevation range can span from 0° to 90°. | [12] |
Mounting type | • Fixed axis in which panels are tilted along a fixed axis. • Polar-aligned where panels are aligned parallel to the Earth’s axis. | [13] |
Sensor type | Sensors like LDRs, photodiodes, or digital sun sensors provide feedback for the tracking system to adjust panel position. | [14] |
Control system | The controller and software receive sensor information to adjust panel position using motors or actuators. | [15] |
Power supply | It can be powered by the panels themselves or a separate source like batteries or smaller solar panels. | [16] |
Durability and resistance | Materials and construction should withstand various weather conditions including wind, rain, snow, and temperature fluctuations. | [17] |
Maintenance and servicing | Accessibility to components, lubrication needs, recalibration requirements, and overall ease of maintenance should be considered during design. | [18] |
Cost and budget | Installation costs, ongoing maintenance, and potential return on investment through increased energy production. | [19] |
LDR, light-dependent resistor.
The angle at which the solar panels are inclined about the horizontal plane is known as the tilt angle of a STS. This angle fluctuates based on the latitude of the installation site, the time of day, and the season. This is essential for maximizing the quantity of solar energy captured by the panels throughout the day. The tracking system usually dynamically adjusts the tilt angle to ensure that the panels are oriented to receive the most sunlight possible, which maximizes the energy output. STS can dramatically increase the total efficiency of solar power generation by continuously modifying the tilt angle in response to the position of the sun [20].
The tilt angle is also a mandatory parameter for solar tracking in which the solar panels are inclined about the horizontal plane and is known as the tilt angle of a STS. This angle fluctuates based on the latitude of the installation site, the time of day, and the season. This is essential for maximizing the quantity of solar energy captured by the panels throughout the day. The tracking system usually dynamically adjusts the tilt angle to ensure that the panels are oriented to receive the most sunlight possible, which maximizes the energy output. STS can dramatically increase the total efficiency of solar power generation by continuously modifying the tilt angle in response to the position of the sun [21]. The equations related to STS are as follows.
Single-axis tracking panel tilt angle (θ):
where, θ = tilt angle of the solar panel in degrees (°), φ = latitude of the location in degrees (°), β = slope angle of the panel concerning the horizontal in degrees (°), and ω = hour angle (°)
Dual-axis tracking panel tilt angle (θ):
where θ = tilt angle of the solar panel, Z = solar zenith angle, and A = solar azimuth angle.
Figure 4a shows the periodic variation in the angle of the sun concerning the equatorial plane that occurs periodically throughout the year due to the axial inclination of the Earth. Biannually, during the equinox, the hour angle and solar declination are depicted in the second figure about an equatorial plane. During these specified time intervals, the Sun’s angle to the equatorial plane is precisely zero, indicating that it is situated directly above the equator. The solstices, which occur around June 21 and December 21, designate the moment when the solar angle becomes at its greatest value relative to the equatorial plane. In the Northern Hemisphere, the summer solstice takes place around the 21st of June, when the sun reaches its highest point approximately 23.5° above the equatorial plane [22].
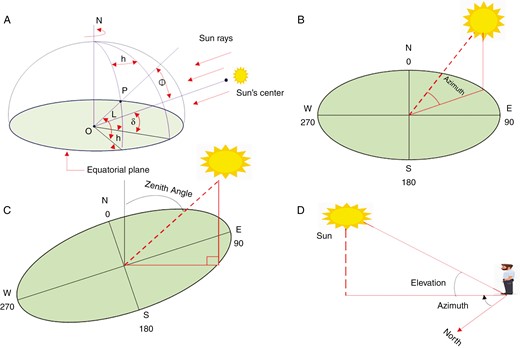
(a) Hour angles and solar declination, (b) azimuth angle, (c) zenith angle, (d) elevation with the position of the observer.
The sun is perceived to be directly overhead at 23.5° in northern latitude, which is the Tropic of Cancer. In contrast, the Tropic of Capricorn, located at 23.5° south latitude, exhibits the sun’s apparent aspect at its minimum. The Sun achieves its minimum angle below the equatorial plane in the Northern Hemisphere on December 21, deviating by approximately 23.5°, in the winter solstice season. As a result, the Sun is positioned perpendicular to the Tropic of Capricorn in the atmosphere, whereas its minimal elevation is perceived from the Tropic of Cancer. The equinoxes, which occur around the 20th of March and the 22nd of September, are distinguished by the Sun’s angle of 0° concerning the equatorial plane. As a result, the Sun is perceived to be positioned directly above the equator [23].
Figure 4b shows that the azimuth angle in an automatic STS is an angle between a solar panel and the position of the sun along the observer’s horizon [24]. An azimuth solar angle for the sun is depicted; it is the horizontal angle that determines the optimal orientation for solar panels to receive maximum sunlight [25].
Solar azimuth angle (A):
where, A = solar azimuth angle, ω = hour angle, φ = latitude of the location, and δ = declination angle [all parameters are in degrees (°)].
The variation in the angle of a sun with an equatorial plane is caused by the axial tilt of the Earth and occurs periodically over a year. Figure 4a shows the hour angle and solar declination about an equatorial plane biannually during the equinox. During these periods, the Sun’s angle to the equatorial plane is nil, indicating that the Sun is positioned above the equator [26]. The solstices, which take place approximately on June 21 and December 21, mark the point at which the angle of a sun concerning the equatorial plane reaches its highest value [27]. In particular, in the Northern Hemisphere, the sun attains its maximum altitude above the equatorial plane, which is approximately 23.5 degrees, during the summer solstice occurring around June 21 [28]. Over the Tropic of Cancer, which is located at 23.5° north latitude, the Sun is observed to be overhead. Conversely, at the Tropic of Capricorn, which is situated at 23.5° south latitude, the Sun is perceived to be at its lowest angle in the sky. By December 21st in the Northern Hemisphere, the Sun reaches its lowest angle below the equatorial plane, which is approximately 23.5° during the winter solstice [29]. Consequently, the Sun is positioned perpendicularly above the Tropic of Capricorn, whereas it is situated at its minimum elevation in the atmosphere when observed from the Tropic of Cancer. The equinoxes, which take place approximately on March 20 and September 22, are characterized by the sun’s angle relative to the equatorial plane being zero. Consequently, the Sun is observed to be directly overhead at the equator [30].
The zenith angle (Fig. 4c) in a solar tracker pertains to the angular measurement between the location of the sun in the sky and a vertical axis. The altitude of the sun above the horizon is determined by the vertical angle [31]. The zenith angle fluctuates throughout the day because of the rotation of the sun across the sky, and the latitude of the installation site of the solar panels is a determining factor in the variability of the zenith angle [32]. Research into tracking systems started immediately after the creation of solar systems in the middle of the 19th century. Different tracking system types, drives, designs, and tracking tactics were defined along with the evolution of tracking systems in this study [33]. This study claims that this survey will help researchers and practicing engineers choose the best control and structure algorithm for real-time applications as a foundation for further advancements in current methods in India, which has broad latitude and longitude and peak climatic and seasonal variations [34].
Solar zenith angle (Z):
where, Z = zenith angle, φ = latitude of the location, δ = declination angle, and ω = hour angle, [all parameters are in degrees (°)].
Figure 4d shows the fluctuation in the altitude angle of the sun during the day, as it traverses from the eastern to the western horizon. During times of sunrise and sunset, the altitude angle of the sun is 0°, indicating that the sun is positioned parallel to the horizon. The sun is at its peak point during the noon hour, creating the day’s greatest height angle. The geographic coordinates of the observer and the data affect this value [35, 36].
1.2 Previous similar works on automatic STS
On the basis of the findings of some studies, a considerable portion of the literature has been devoted to optimizing monitoring algorithms and technologies while neglecting to adequately consider other crucial efficiency-influencing factors. These factors include but are not limited to solar radiation principles, temperature, auxiliary equipment, and the processes employed for power storage and transportation, among others [37]. Compared with open-loop systems, closed-loop systems have been observed to exhibit superior electrical efficacy on average. The tracking mode control systems and the technology used to design PV systems substantially influence the overall system efficiency and cost. Numerous algorithms aimed at enhancing energy efficiency and minimizing power loss have been studied and documented in the scientific literature [38].
Compared with static PV systems, single- and dual-axis PV tracking systems have the potential to increase electrical output by 22%–56% when utilized in conjunction with appropriate control systems. The most prevalent and efficient control strategy combines sensor-based and microprocessor-based control systems. Consequently, it is critical to optimize the energy usage of electrical devices by reducing the motor’s revolution frequency [39]. Active solar trackers outperform alternative trackers, as stated in one review article, owing to their substantial energy gain of 56% and highest panel efficiency of 76%. Chronological tracking, on the other hand, is the best choice when the tracking error is minimized to 0.10°. Among the alternatives of stable, single and dual axes, the dual axes exhibit the highest power gain (56%) and the smallest tracking error (0.11°). As a result, we recommend active dual-axis solar followers in general because of their optimal performance in terms of energy gain and monitoring error [40].
Table 2 presents the recent reviews performed in recent years and, on the basis of this recent research, some of the outcomes, which can be considered gaps or recommendations.
Comparison between previously published review articles on different solar tracking technologies.
Title . | Methodology . | Type of trackers . | Key performance indices . | Gaps/recommendations . | References . |
---|---|---|---|---|---|
Solar tracking systems: technologies and trackers drive types—a review | Investigation of the feasibility of solar tracking devices using multiple axes and geographical locations around the world. | Sequential solar tracking, active solar tracking, semi-passive solar tracking, passive solar tracking, and manual tracking are the different types of tracking. | • Horizontal tracking is used by ~16.67%. • Azimuth tracking by 10%, altitude tracking by 16.67%, and polar solar tracking by 4.44%. • The primary solar tracker has a substantial utilization in the active solar tracking type by 76.42% • A chronological solar tracker has the second-highest influence by 7.55%. | • Lack of a comparative analysis between single-axis and dual-axis STS in terms of their efficiency, cost, and practicality. • The specific needs of different regions and the influence of local climate and sun movement patterns on tracking system choice could be valuable research avenues. | [41] |
Recent advancements and challenges in solar tracking systems (STS): a review | The comparison between the energy exchange between one-axis and two-axis solar trackers, costs, and availability is revealed. | 23 different solar technologies have been compared based on system description, performances, and energy gain. | • When compared to fixed solar collector systems, the electricity return for one axis usually hits 25% and for two axes, it usually goes beyond 40%. • In regard to energy return, double-axis monitoring systems are head and shoulders above the competition. | • A concise discussion of the impact of profitability within the edible oil sector on the functioning of biodiesel production capacity. • One potential constraint is the absence of an in-depth economic analysis, encompassing comprehensive cost‒benefit evaluations, that could offer a more elucidating understanding of the economic obstacles associated with biodiesel production. | [42] |
Deep learning techniques for photovoltaic solar tracking systems: a systematic literature review | Predicting optimal angles, adapting to weather changes, and improving the control algorithms for better efficiency. | Adaptive and intelligent trackers, hybrid trackers, dual-axis trackers, single-axis trackers. | • This review found that many researchers frequently omit preprocessing techniques from their models, potentially limiting the effectiveness of those models. • Additionally, those who have attempted any preprocessing have primarily used the min–max scaling normalization of data technique. | • It does not include an in-depth analysis of the energy efficiency aspects of deep learning-based tracking systems. • Further investigation is required to comprehend and enhance the energy utilization of these systems. | [43] |
Solar photovoltaic tracking systems for electricity generation: a review | This review study looks at the various algorithms and approaches for solar tracking that have greater power generation efficiency and improved accuracy. | Hybrid PV systems include tracker systems, one-axis and two-axis systems with CPV mirrors and PV/T systems. | • According to this review paper, when used in conjunction with the proper control systems, single- and dual-axis solar trackers can boost electrical output by 22%–56%. | • The research emphasizes the significance of control systems in optimizing STS. It mentions the prevalence of microprocessor- and sensor-based control systems and their efficiency. • Research could further explore the development of advanced control methods that minimize energy consumption and improve tracking accuracy. | [44] |
Solar tracker transcript—a review | A comparison of solar tracker data in recent advancements has been done. | Dual axis, horizontal, single, tilt, dual ARNN architecture, parallel mechanism, dual azimuth, ARNN, horizontal, azimuth, and tilt tracking (single and dual). | • 2-, 3-, or 4-point single- or dual-axis active solar trackers consume less electricity than continuous ones. • Studies suggest active tracker with 76.42%, and chronological with 7.55%. • Polar tracking is 4.44%, azimuth is 10%, azimuth and altitude is 16.67%, and horizontal is 16.67%. | There is a lack of detailed analysis considering factors such as cost-effectiveness, maintenance requirements, environmental impact, and applicability in different geographical locations or solar installations. | [45] |
Review of dual axis solar tracking and development of its functional model | Here the different dual-axis solar tracker is compared and analysed on the basis of accuracy and energy generation. | Dual-axis STS and its types | • A dual-tracking arrangement has an average efficiency of 33%–43% during 1997–2017. • In addition, it was shown that active tracking systems are more typically deployed for dual-axis tracking than passive ones. | • The research indicates that active tracking systems prioritize accuracy but consume generated energy, while passive tracking systems are more energy-conservative. • In optimizing the energy efficiency of active dual-axis tracking systems, future studies could focus on enhancing energy conservation in active systems while maintaining accuracy. | [46] |
A comprehensive study of techno-economic and environmental features of different solar tracking systems for residential photovoltaic installations | Considering the environmental, financial, and technological impacts of numerous residential PV STS. | The tracking of the horizontal solar axis, the vertical-axis trackers, and the dual-axis trackers. | • The most efficient tracking method is the dual trackers, which increases power output by an average of 32% compared to the case where there is no tracking. • The most economical solution is the vertical tracking system, which improves power generation by an average of 23%. | • Further exploring the environmental implications and trade-offs of STS in different regions, taking into account not only emissions but also land use and other environmental factors should be considered. | [47] |
Title . | Methodology . | Type of trackers . | Key performance indices . | Gaps/recommendations . | References . |
---|---|---|---|---|---|
Solar tracking systems: technologies and trackers drive types—a review | Investigation of the feasibility of solar tracking devices using multiple axes and geographical locations around the world. | Sequential solar tracking, active solar tracking, semi-passive solar tracking, passive solar tracking, and manual tracking are the different types of tracking. | • Horizontal tracking is used by ~16.67%. • Azimuth tracking by 10%, altitude tracking by 16.67%, and polar solar tracking by 4.44%. • The primary solar tracker has a substantial utilization in the active solar tracking type by 76.42% • A chronological solar tracker has the second-highest influence by 7.55%. | • Lack of a comparative analysis between single-axis and dual-axis STS in terms of their efficiency, cost, and practicality. • The specific needs of different regions and the influence of local climate and sun movement patterns on tracking system choice could be valuable research avenues. | [41] |
Recent advancements and challenges in solar tracking systems (STS): a review | The comparison between the energy exchange between one-axis and two-axis solar trackers, costs, and availability is revealed. | 23 different solar technologies have been compared based on system description, performances, and energy gain. | • When compared to fixed solar collector systems, the electricity return for one axis usually hits 25% and for two axes, it usually goes beyond 40%. • In regard to energy return, double-axis monitoring systems are head and shoulders above the competition. | • A concise discussion of the impact of profitability within the edible oil sector on the functioning of biodiesel production capacity. • One potential constraint is the absence of an in-depth economic analysis, encompassing comprehensive cost‒benefit evaluations, that could offer a more elucidating understanding of the economic obstacles associated with biodiesel production. | [42] |
Deep learning techniques for photovoltaic solar tracking systems: a systematic literature review | Predicting optimal angles, adapting to weather changes, and improving the control algorithms for better efficiency. | Adaptive and intelligent trackers, hybrid trackers, dual-axis trackers, single-axis trackers. | • This review found that many researchers frequently omit preprocessing techniques from their models, potentially limiting the effectiveness of those models. • Additionally, those who have attempted any preprocessing have primarily used the min–max scaling normalization of data technique. | • It does not include an in-depth analysis of the energy efficiency aspects of deep learning-based tracking systems. • Further investigation is required to comprehend and enhance the energy utilization of these systems. | [43] |
Solar photovoltaic tracking systems for electricity generation: a review | This review study looks at the various algorithms and approaches for solar tracking that have greater power generation efficiency and improved accuracy. | Hybrid PV systems include tracker systems, one-axis and two-axis systems with CPV mirrors and PV/T systems. | • According to this review paper, when used in conjunction with the proper control systems, single- and dual-axis solar trackers can boost electrical output by 22%–56%. | • The research emphasizes the significance of control systems in optimizing STS. It mentions the prevalence of microprocessor- and sensor-based control systems and their efficiency. • Research could further explore the development of advanced control methods that minimize energy consumption and improve tracking accuracy. | [44] |
Solar tracker transcript—a review | A comparison of solar tracker data in recent advancements has been done. | Dual axis, horizontal, single, tilt, dual ARNN architecture, parallel mechanism, dual azimuth, ARNN, horizontal, azimuth, and tilt tracking (single and dual). | • 2-, 3-, or 4-point single- or dual-axis active solar trackers consume less electricity than continuous ones. • Studies suggest active tracker with 76.42%, and chronological with 7.55%. • Polar tracking is 4.44%, azimuth is 10%, azimuth and altitude is 16.67%, and horizontal is 16.67%. | There is a lack of detailed analysis considering factors such as cost-effectiveness, maintenance requirements, environmental impact, and applicability in different geographical locations or solar installations. | [45] |
Review of dual axis solar tracking and development of its functional model | Here the different dual-axis solar tracker is compared and analysed on the basis of accuracy and energy generation. | Dual-axis STS and its types | • A dual-tracking arrangement has an average efficiency of 33%–43% during 1997–2017. • In addition, it was shown that active tracking systems are more typically deployed for dual-axis tracking than passive ones. | • The research indicates that active tracking systems prioritize accuracy but consume generated energy, while passive tracking systems are more energy-conservative. • In optimizing the energy efficiency of active dual-axis tracking systems, future studies could focus on enhancing energy conservation in active systems while maintaining accuracy. | [46] |
A comprehensive study of techno-economic and environmental features of different solar tracking systems for residential photovoltaic installations | Considering the environmental, financial, and technological impacts of numerous residential PV STS. | The tracking of the horizontal solar axis, the vertical-axis trackers, and the dual-axis trackers. | • The most efficient tracking method is the dual trackers, which increases power output by an average of 32% compared to the case where there is no tracking. • The most economical solution is the vertical tracking system, which improves power generation by an average of 23%. | • Further exploring the environmental implications and trade-offs of STS in different regions, taking into account not only emissions but also land use and other environmental factors should be considered. | [47] |
CPV, concentrating PV; ARNN, artificial recurrent neural network; PV/T, PV/thermal.
Comparison between previously published review articles on different solar tracking technologies.
Title . | Methodology . | Type of trackers . | Key performance indices . | Gaps/recommendations . | References . |
---|---|---|---|---|---|
Solar tracking systems: technologies and trackers drive types—a review | Investigation of the feasibility of solar tracking devices using multiple axes and geographical locations around the world. | Sequential solar tracking, active solar tracking, semi-passive solar tracking, passive solar tracking, and manual tracking are the different types of tracking. | • Horizontal tracking is used by ~16.67%. • Azimuth tracking by 10%, altitude tracking by 16.67%, and polar solar tracking by 4.44%. • The primary solar tracker has a substantial utilization in the active solar tracking type by 76.42% • A chronological solar tracker has the second-highest influence by 7.55%. | • Lack of a comparative analysis between single-axis and dual-axis STS in terms of their efficiency, cost, and practicality. • The specific needs of different regions and the influence of local climate and sun movement patterns on tracking system choice could be valuable research avenues. | [41] |
Recent advancements and challenges in solar tracking systems (STS): a review | The comparison between the energy exchange between one-axis and two-axis solar trackers, costs, and availability is revealed. | 23 different solar technologies have been compared based on system description, performances, and energy gain. | • When compared to fixed solar collector systems, the electricity return for one axis usually hits 25% and for two axes, it usually goes beyond 40%. • In regard to energy return, double-axis monitoring systems are head and shoulders above the competition. | • A concise discussion of the impact of profitability within the edible oil sector on the functioning of biodiesel production capacity. • One potential constraint is the absence of an in-depth economic analysis, encompassing comprehensive cost‒benefit evaluations, that could offer a more elucidating understanding of the economic obstacles associated with biodiesel production. | [42] |
Deep learning techniques for photovoltaic solar tracking systems: a systematic literature review | Predicting optimal angles, adapting to weather changes, and improving the control algorithms for better efficiency. | Adaptive and intelligent trackers, hybrid trackers, dual-axis trackers, single-axis trackers. | • This review found that many researchers frequently omit preprocessing techniques from their models, potentially limiting the effectiveness of those models. • Additionally, those who have attempted any preprocessing have primarily used the min–max scaling normalization of data technique. | • It does not include an in-depth analysis of the energy efficiency aspects of deep learning-based tracking systems. • Further investigation is required to comprehend and enhance the energy utilization of these systems. | [43] |
Solar photovoltaic tracking systems for electricity generation: a review | This review study looks at the various algorithms and approaches for solar tracking that have greater power generation efficiency and improved accuracy. | Hybrid PV systems include tracker systems, one-axis and two-axis systems with CPV mirrors and PV/T systems. | • According to this review paper, when used in conjunction with the proper control systems, single- and dual-axis solar trackers can boost electrical output by 22%–56%. | • The research emphasizes the significance of control systems in optimizing STS. It mentions the prevalence of microprocessor- and sensor-based control systems and their efficiency. • Research could further explore the development of advanced control methods that minimize energy consumption and improve tracking accuracy. | [44] |
Solar tracker transcript—a review | A comparison of solar tracker data in recent advancements has been done. | Dual axis, horizontal, single, tilt, dual ARNN architecture, parallel mechanism, dual azimuth, ARNN, horizontal, azimuth, and tilt tracking (single and dual). | • 2-, 3-, or 4-point single- or dual-axis active solar trackers consume less electricity than continuous ones. • Studies suggest active tracker with 76.42%, and chronological with 7.55%. • Polar tracking is 4.44%, azimuth is 10%, azimuth and altitude is 16.67%, and horizontal is 16.67%. | There is a lack of detailed analysis considering factors such as cost-effectiveness, maintenance requirements, environmental impact, and applicability in different geographical locations or solar installations. | [45] |
Review of dual axis solar tracking and development of its functional model | Here the different dual-axis solar tracker is compared and analysed on the basis of accuracy and energy generation. | Dual-axis STS and its types | • A dual-tracking arrangement has an average efficiency of 33%–43% during 1997–2017. • In addition, it was shown that active tracking systems are more typically deployed for dual-axis tracking than passive ones. | • The research indicates that active tracking systems prioritize accuracy but consume generated energy, while passive tracking systems are more energy-conservative. • In optimizing the energy efficiency of active dual-axis tracking systems, future studies could focus on enhancing energy conservation in active systems while maintaining accuracy. | [46] |
A comprehensive study of techno-economic and environmental features of different solar tracking systems for residential photovoltaic installations | Considering the environmental, financial, and technological impacts of numerous residential PV STS. | The tracking of the horizontal solar axis, the vertical-axis trackers, and the dual-axis trackers. | • The most efficient tracking method is the dual trackers, which increases power output by an average of 32% compared to the case where there is no tracking. • The most economical solution is the vertical tracking system, which improves power generation by an average of 23%. | • Further exploring the environmental implications and trade-offs of STS in different regions, taking into account not only emissions but also land use and other environmental factors should be considered. | [47] |
Title . | Methodology . | Type of trackers . | Key performance indices . | Gaps/recommendations . | References . |
---|---|---|---|---|---|
Solar tracking systems: technologies and trackers drive types—a review | Investigation of the feasibility of solar tracking devices using multiple axes and geographical locations around the world. | Sequential solar tracking, active solar tracking, semi-passive solar tracking, passive solar tracking, and manual tracking are the different types of tracking. | • Horizontal tracking is used by ~16.67%. • Azimuth tracking by 10%, altitude tracking by 16.67%, and polar solar tracking by 4.44%. • The primary solar tracker has a substantial utilization in the active solar tracking type by 76.42% • A chronological solar tracker has the second-highest influence by 7.55%. | • Lack of a comparative analysis between single-axis and dual-axis STS in terms of their efficiency, cost, and practicality. • The specific needs of different regions and the influence of local climate and sun movement patterns on tracking system choice could be valuable research avenues. | [41] |
Recent advancements and challenges in solar tracking systems (STS): a review | The comparison between the energy exchange between one-axis and two-axis solar trackers, costs, and availability is revealed. | 23 different solar technologies have been compared based on system description, performances, and energy gain. | • When compared to fixed solar collector systems, the electricity return for one axis usually hits 25% and for two axes, it usually goes beyond 40%. • In regard to energy return, double-axis monitoring systems are head and shoulders above the competition. | • A concise discussion of the impact of profitability within the edible oil sector on the functioning of biodiesel production capacity. • One potential constraint is the absence of an in-depth economic analysis, encompassing comprehensive cost‒benefit evaluations, that could offer a more elucidating understanding of the economic obstacles associated with biodiesel production. | [42] |
Deep learning techniques for photovoltaic solar tracking systems: a systematic literature review | Predicting optimal angles, adapting to weather changes, and improving the control algorithms for better efficiency. | Adaptive and intelligent trackers, hybrid trackers, dual-axis trackers, single-axis trackers. | • This review found that many researchers frequently omit preprocessing techniques from their models, potentially limiting the effectiveness of those models. • Additionally, those who have attempted any preprocessing have primarily used the min–max scaling normalization of data technique. | • It does not include an in-depth analysis of the energy efficiency aspects of deep learning-based tracking systems. • Further investigation is required to comprehend and enhance the energy utilization of these systems. | [43] |
Solar photovoltaic tracking systems for electricity generation: a review | This review study looks at the various algorithms and approaches for solar tracking that have greater power generation efficiency and improved accuracy. | Hybrid PV systems include tracker systems, one-axis and two-axis systems with CPV mirrors and PV/T systems. | • According to this review paper, when used in conjunction with the proper control systems, single- and dual-axis solar trackers can boost electrical output by 22%–56%. | • The research emphasizes the significance of control systems in optimizing STS. It mentions the prevalence of microprocessor- and sensor-based control systems and their efficiency. • Research could further explore the development of advanced control methods that minimize energy consumption and improve tracking accuracy. | [44] |
Solar tracker transcript—a review | A comparison of solar tracker data in recent advancements has been done. | Dual axis, horizontal, single, tilt, dual ARNN architecture, parallel mechanism, dual azimuth, ARNN, horizontal, azimuth, and tilt tracking (single and dual). | • 2-, 3-, or 4-point single- or dual-axis active solar trackers consume less electricity than continuous ones. • Studies suggest active tracker with 76.42%, and chronological with 7.55%. • Polar tracking is 4.44%, azimuth is 10%, azimuth and altitude is 16.67%, and horizontal is 16.67%. | There is a lack of detailed analysis considering factors such as cost-effectiveness, maintenance requirements, environmental impact, and applicability in different geographical locations or solar installations. | [45] |
Review of dual axis solar tracking and development of its functional model | Here the different dual-axis solar tracker is compared and analysed on the basis of accuracy and energy generation. | Dual-axis STS and its types | • A dual-tracking arrangement has an average efficiency of 33%–43% during 1997–2017. • In addition, it was shown that active tracking systems are more typically deployed for dual-axis tracking than passive ones. | • The research indicates that active tracking systems prioritize accuracy but consume generated energy, while passive tracking systems are more energy-conservative. • In optimizing the energy efficiency of active dual-axis tracking systems, future studies could focus on enhancing energy conservation in active systems while maintaining accuracy. | [46] |
A comprehensive study of techno-economic and environmental features of different solar tracking systems for residential photovoltaic installations | Considering the environmental, financial, and technological impacts of numerous residential PV STS. | The tracking of the horizontal solar axis, the vertical-axis trackers, and the dual-axis trackers. | • The most efficient tracking method is the dual trackers, which increases power output by an average of 32% compared to the case where there is no tracking. • The most economical solution is the vertical tracking system, which improves power generation by an average of 23%. | • Further exploring the environmental implications and trade-offs of STS in different regions, taking into account not only emissions but also land use and other environmental factors should be considered. | [47] |
CPV, concentrating PV; ARNN, artificial recurrent neural network; PV/T, PV/thermal.
On the basis of previously published research articles in similar areas, the critical unaddressed areas related to similar studies are as follows.
Technoeconomic analysis based on location is lacking for STS across different geographic locations, which limits the understanding of their efficiency in various climates.
Comparative analyses of single-axis and dual-axis STS in terms of their efficiency, cost, and practicality are lacking. Research on the specific needs of different regions and the influence of local climate and sun movement patterns on tracking system choices is needed.
An in-depth economic analysis, including comprehensive cost‒benefit evaluations, to better understand the economic obstacles associated with biodiesel production is lacking. Frequent omission of preprocessing techniques from models by researchers limits the effectiveness of those models.
The use of preprocessing techniques, primarily min–max scaling normalization of data, is limited, and an in-depth analysis of the energy efficiency aspects of deep learning-based tracking systems is lacking. Further investigations are needed to comprehend and enhance the energy utilization of these systems.
Further research on advanced control methods that minimize energy consumption and improve tracking accuracy in STS is needed. A detailed analysis considering factors such as cost-effectiveness, maintenance requirements, environmental impact, and applicability in different geographical locations or solar installations is lacking.
The energy efficiency of active dual-axis tracking systems needs to be optimized while maintaining accuracy. Future studies could focus on enhancing energy conservation in active systems. There is a need for further exploration of the environmental implications and trade-offs of STS in different regions while considering emissions, land use, and other environmental factors.
In this study, all the technologies related to the STS are now highlighted with classifications on the basis of the number of axes, the activity of the tracking unit, the control strategies, and the tracking strategies. This literature review will help readers determine the current advancements in STS and their parameter variations. This literature review provides a trace of the path of the sun and reveals how solar insolation changes with time. The solar insolation data can be taken for any latitude and longitude. This literature review compared data based on different criteria, i.e. the axis of rotation, tracking method, accuracy, energy efficiency, space requirement, cost, applicability, weather, wind tolerance, and installation flexibility. This study examines several tracking methodologies employed in different geographic regions. This observation underscores the prevalence of active solar trackers and underscores the importance of conducting comparative evaluations between single-axis and dual-axis systems, taking into account factors such as efficiency and cost. Additionally, a study on the special demands of different regions, which are determined by climate conditions and patterns of solar movement, is proposed.
1.3 Tracing of the sun’s path to develop an automatic STS
Figure 5 shows the solar insolation in kilowatts per square metre (kW/m2) per month in the northern region of India. The graph shows how solar insolation varies over the 12 months of the year. STS have the potential to increase the efficiency and output of solar applications. The principal objective of this research endeavour is to ascertain the viability of STS that incorporate multiple-axis systems and geographic locations [48]. The cost increase associated with increasing power output counterbalances the benefit gained from single-axis tracking compared with dual-axis tracking, rendering the distinction between the two relatively insignificant [49].
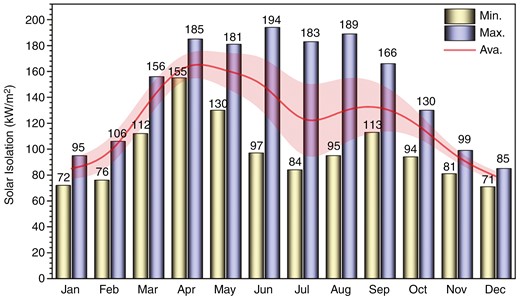
Annual solar insolation in kilowatts per square metre (kW/m2) for the northern region of India.
In regard to increasing the efficiency of an automatic solar tracker, one of the most important aspects to consider is the length of the sunny day as well as the amount of solar insolation (as shown in Fig. 6). The figure shows how the solar insolation changes as the sun travels across the sky. A STS is designed to ensure that solar panels and other equipment continue to be oriented in a direction that is directly toward the sun [50]. The length of a solar day not only changes depending on the season but also changes depending on where an observer is located on the surface of the Earth. Using a method that considers these fluctuations and calculates the location of the sun at various times throughout the day and year, it is possible to compute the day that the sun is visible to the Earth for a STS [51].
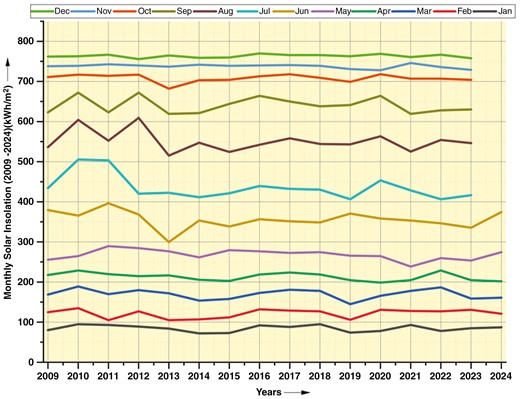
Monthly solar isolation (in kW/m2) throughout the year from 2009 to 2024.
To create a solar heatmap via VEDAS, as shown in Fig. 7, a 3D model of the site was generated via VEDAS modelling tools. The software then uses data on the site’s latitude, longitude, and altitude to calculate the path of the sun over different value times per day and year. Using this information, VEDAS calculates the shading caused by surrounding buildings, trees, and other objects, as well as the shading caused by the building itself. The model includes information about the height and location of buildings, trees, and other objects that could cast shadows; by using this method, a solar heatmap for different latitudes and longitudes for the shadow analysis of any location can be obtained [52, 53].
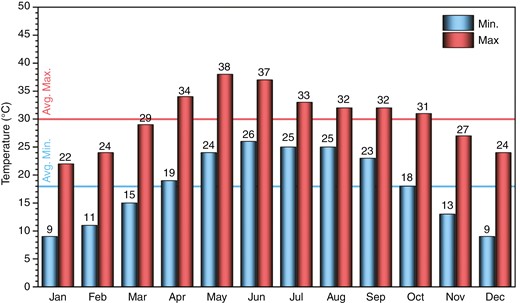
Average minimum and maximum temperatures in degree Celsius per month via Visualisation of Earth Observation Data and Archival System (VEDAS).
2. History of automatic solar trackers
Since the early 20th century, scientists and innovators first realized the potential advantages of using solar energy in automated sun-tracking systems. Shuman created one of the earliest known examples of a sun-tracking system in 1912, as given in Table 3. Using mirrors to focus sunlight onto a boiler, which produces steam to power a pump, he created a solar-powered irrigation system. In the 1950s, researchers began to develop more sophisticated STS that use electronic sensors to follow the path of the sun. After several studies were performed by researchers in this area, a mechanically operated solar tracker was designed by Finster [60], and then, in 1963, A. Saavedra introduced an upgraded model that could track the sun’s location via a rotating pyro heliometer. The first commercial solar tracker was introduced in the 1970s, and these systems were primarily used in the aerospace industry to power satellites. During the 1980s and 1990s, the development of new technologies, such as microprocessors and electronic control systems, made it possible to design STS that were more efficient and reliable. This led to the widespread adoption of solar trackers in commercial and residential solar energy markets. Today, automatic STS are being used in a variety of applications, from large-scale solar power plants to residential solar installations. To adjust the position of solar panels throughout the day, these systems use a combination of sensors, motors, and control systems, increasing the amount of sunlight received and maximizing the overall energy output [61].
Timeline . | Research finding . | References . |
---|---|---|
1912 | Shuman developed a solar-powered irrigation system that used mirrors to concentrate sunlight onto a boiler, which produced steam to power a pump. | [54] |
1950 | Researchers began to frame the STS that used electronic sensors to follow the movement of the sun. | [55] |
1962 | Finster developed the solar tracker which was completely mechanical and not a very efficient system. | [19] |
1970 | The first commercial STS were introduced, primarily for use in the aerospace industry to power satellites. | [56] |
1975 | The sun tracker, created by Raymond H. McFee, is quite accurate, with an inaccuracy of only half a degree to one degree, thanks to the contributions of many individual mirrors. | [57] |
1980 | The first commercially available STS with a limiting position and excess heat switch was created by Dorian and Nelson using an electrically operated mechanism. | [58] |
1980–90 | New technologies, such as microprocessors and electronic control systems, made STS more efficient and dependable. | [59] |
Timeline . | Research finding . | References . |
---|---|---|
1912 | Shuman developed a solar-powered irrigation system that used mirrors to concentrate sunlight onto a boiler, which produced steam to power a pump. | [54] |
1950 | Researchers began to frame the STS that used electronic sensors to follow the movement of the sun. | [55] |
1962 | Finster developed the solar tracker which was completely mechanical and not a very efficient system. | [19] |
1970 | The first commercial STS were introduced, primarily for use in the aerospace industry to power satellites. | [56] |
1975 | The sun tracker, created by Raymond H. McFee, is quite accurate, with an inaccuracy of only half a degree to one degree, thanks to the contributions of many individual mirrors. | [57] |
1980 | The first commercially available STS with a limiting position and excess heat switch was created by Dorian and Nelson using an electrically operated mechanism. | [58] |
1980–90 | New technologies, such as microprocessors and electronic control systems, made STS more efficient and dependable. | [59] |
Timeline . | Research finding . | References . |
---|---|---|
1912 | Shuman developed a solar-powered irrigation system that used mirrors to concentrate sunlight onto a boiler, which produced steam to power a pump. | [54] |
1950 | Researchers began to frame the STS that used electronic sensors to follow the movement of the sun. | [55] |
1962 | Finster developed the solar tracker which was completely mechanical and not a very efficient system. | [19] |
1970 | The first commercial STS were introduced, primarily for use in the aerospace industry to power satellites. | [56] |
1975 | The sun tracker, created by Raymond H. McFee, is quite accurate, with an inaccuracy of only half a degree to one degree, thanks to the contributions of many individual mirrors. | [57] |
1980 | The first commercially available STS with a limiting position and excess heat switch was created by Dorian and Nelson using an electrically operated mechanism. | [58] |
1980–90 | New technologies, such as microprocessors and electronic control systems, made STS more efficient and dependable. | [59] |
Timeline . | Research finding . | References . |
---|---|---|
1912 | Shuman developed a solar-powered irrigation system that used mirrors to concentrate sunlight onto a boiler, which produced steam to power a pump. | [54] |
1950 | Researchers began to frame the STS that used electronic sensors to follow the movement of the sun. | [55] |
1962 | Finster developed the solar tracker which was completely mechanical and not a very efficient system. | [19] |
1970 | The first commercial STS were introduced, primarily for use in the aerospace industry to power satellites. | [56] |
1975 | The sun tracker, created by Raymond H. McFee, is quite accurate, with an inaccuracy of only half a degree to one degree, thanks to the contributions of many individual mirrors. | [57] |
1980 | The first commercially available STS with a limiting position and excess heat switch was created by Dorian and Nelson using an electrically operated mechanism. | [58] |
1980–90 | New technologies, such as microprocessors and electronic control systems, made STS more efficient and dependable. | [59] |
3. Classification of the STS and its comparative analysis based on different parameters
Based on the number of axes, the STS is classified into two types: single-axis trackers and dual-axis trackers. Here, the single-axis trackers are further divided into four parts, i.e. horizontal single-axis trackers (HSATs), vertical single-axis trackers (VSATs), polar-aligned single-axis trackers, and tilted single-axis trackers. The dual-axis trackers can be further divided into two types [62]: tip-tilt dual-axis trackers (TTDATs) and azimuth-altitude dual-axis trackers (AADATs), as shown in Fig. 8.
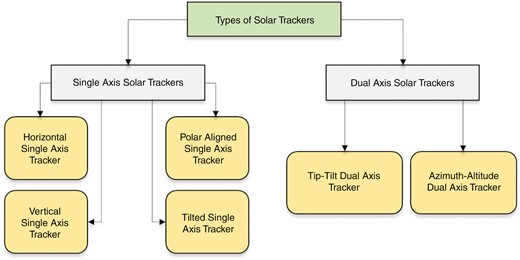
There are two types of STS based on the number of axes: single-axis trackers and dual-axis trackers:
3.1 Single-axis trackers
A single-axis tracker is a form of solar tracker that follows the movement of the sun along a single axis. Depending on the design, the axis of rotation usually aligns with the north‒south or east‒west axis. Single-axis trackers are a common component of solar panel tracking systems and are commonly used in utility-scale solar projects to increase the quantity of energy generated by solar panels [63]. By monitoring the sun’s movement, solar panels can maintain a perpendicular angle with the sun’s rays, maximizing the energy captured. Depending on the design and location, single-axis solar trackers can maximize the generation of energy by up to 25% compared with fixed-tilt solar systems. Nevertheless, single-axis trackers are typically more costly to install and maintain than fixed-tilt systems are; thus, the economic viability of trackers depends on the specific project and location. A single-axis tracker simply refers to the fact that it has a single rotational axis [64].
3.1.1 Horizontal single-axis trackers
An HSAT (Fig. 9a) rotates to track the east‒west path of the sun. Since the rotation axis matches the east‒west axis, the solar panels tilt east‒west as the sun moves. Utility-scale solar projects use HSATs because they can increase energy production by 25% over that of fixed-tilt systems [65]. By monitoring the sun, solar panels can maximize energy collection while facing perpendicularly. HSATs use less acreage than fixed-tilt devices do. The panels can be closer together because they do not cast shadows on each other during the path of the sun. Owing to their simplicity, HSATs require less maintenance than other STS do [66].
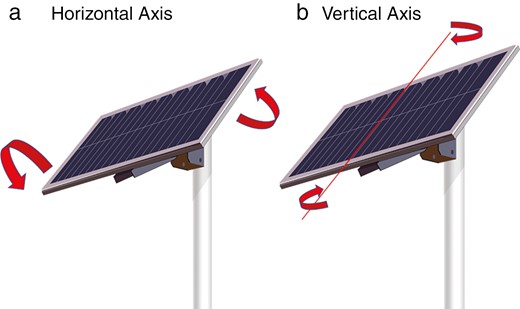
(a) Directional movement of the HSAT and (b) directional movement of the vertical single-axis solar tracker.
3.1.2 Vertical single-axis trackers
A VSAT (Fig. 9b) enables solar panels to rotate on a vertical axis while tracking the path of the sun from east to west. Typically, this form of tracker is made up of several vertically positioned poles or columns that each support a horizontal beam or arm that holds the solar panel array [67, 68]. The involvement of a motor in the vertical single-axis tracking system facilitates the rotation of the array along the vertical axis of the sun, thereby ensuring that the panel remains perpendicular (90°) to the rays of the sun over the course of the day. The implementation of movable solar panels enables the panels to capture a greater amount of sunlight, resulting in increased energy generation in comparison to the stationary PV system. Compared with other tracking systems, the VSAT is a solar panel tracker that boasts a comparatively uncomplicated design and is generally more cost-effective in terms of installation and upkeep [69, 70].
3.1.3 Tilted single-axis trackers
The tilted single-axis tracker is the solar panel mounting system that enables the rotation of the solar panels on a single axis while being inclined at an angle to the ground, as shown in Fig. 10a. This tracking mechanism is typically composed of a sequence of vertical poles or columns that are securely installed into the Earth at a predetermined inclination. Each pole is responsible for sustaining a level, horizontal beam or arm that accommodates the solar panel array [71]. A tilted single-axis tracking system uses a motor to spin the array. This device helps keep panels perpendicular to the light all day. The permanent mounting framework maximizes the angle of contact between the sunbeams and the solar panel array, increasing the energy yield, flexibility, and adaptability, which are advantages of a tilted single-axis tracker over a vertical tracker [72].
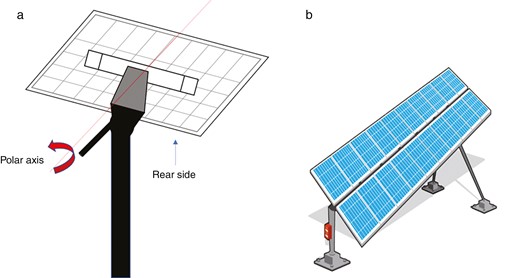
(a) Directional movement of the polar-aligned single-axis tracker. (b) Tilted single-axis tracker.
3.1.4 Polar-aligned single-axis trackers
The polar-aligned single-axis solar tracker (shown in Fig. 10b) is a type of solar tracker that moves solar panels along a single axis of rotation to follow the position of the sun throughout the day. Unlike fixed solar panels, which are stationary, polar-aligned single-axis trackers move in a horizontal or vertical direction, depending upon the orientation of the rotation axis [73]. Polar-aligned single-axis STS are simpler and less expensive than dual-axis trackers, which track the sun horizontally and vertically. Polar-aligned single-axis tracking systems can increase energy production by 25% over that of fixed STS. A well-equipped ascending telescope with a tilted single axis aligns with the polar star’s rotation axis [74, 75].
3.2 Dual-axis trackers
The dual-axis STS is designed to revolve around a stationary vertical axis, while the panels are affixed to a secondary horizontal axis that synchronizes with the primary axis rotation. This STS is designed to trace the trajectory of the sun by rotating along two axes, thereby enabling the solar panels to remain oriented perpendicular to the sun’s rays for the day [76]. The device monitors the daily progression of the sun’s movement from east to west, as well as its seasonal shift from east to north or south. The phenomenon of moving from east to west is commonly referred to as the zenith angle, whereas the movement from east to north or south that occurs annually is known as the azimuth angle. The solar tracker facilitates the attainment of optimal solar energy by tracking the sun’s movement both vertically and horizontally. The solar output will increase by 40%–45% [77]. They were classified by the orientation of their primary axis about the ground. This tracker has two standard implementations—TTDAT and AADAT—which are detailed in the following sections.
3.2.1 Tip-tilt dual-axis trackers
TTDATs are solar tracking technologies that facilitate the adjustment of solar panels along two axes, enabling them to track the trajectory of the sun throughout the day. The trackers employ a dual-axis rotation mechanism, whereby one axis is utilized for vertical panel tilting and the other for horizontal rotation. The tip-tilt mechanism is employed to maintain the panel’s alignment with the sun throughout the day, despite the movement of the sun across the sky. The sensors of the solar tracker can find the precise position of the sun, and subsequently, the control system of the tracker can make necessary adjustments to the position of the solar panel to optimize the amount of solar radiation it receives. Tip-tilt axis trackers have the potential to increase the efficacy of solar panels by up to 40%, in contrast to stationary installations [78, 79].
3.2.2 Azimuth-altitude dual-axis trackers
AADATs represent a distinct category of STS that enable the repositioning of solar panels along two axes. Azimuth-altitude trackers employ a dual-axis rotation system, whereby one axis facilitates horizontal rotation of the panel, whereas the other axis enables vertical adjustment of its angle. The panel’s orientation is controlled by two axes: the horizontal axis facilitates rotation to track the movement of the sun across the sky, and the vertical axis enables adjustment of the panel’s angle to optimize sunlight absorption over the day [80, 81].
Figure 11 shows how the solar trackers are classified based on two types of control strategies, i.e. open loops and closed loops, which are based on tracking strategies, and unit tracking, which has passive and active solar trackers [82].
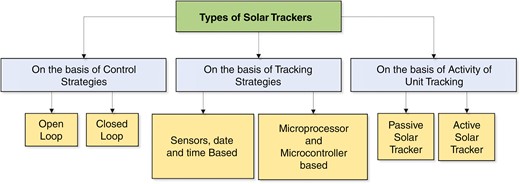
Classification of solar trackers based on different strategies.
3.3 Types of STS based on the activity of the tracking unit
With respect to cost, complexity, efficiency, and upkeep, every variety of STS possesses distinct merits and demerits. Location, available resources, energy output requirements, and the trade-off between initial investment and long-term benefits are frequently determinants in system selection.
3.3.1 Passive solar trackers
To create a passive solar tracker, two identical cylindrical tubes are spaced equally from the centre pivot and positioned on either side of the solar panel. Each tube contains fluid that is under partial pressure. Damping the movement slows it down. This simple setup not only is easy to build but also uses zero power from the PV cell. However, every morning, it sets out with an erroneous orientation, trying to change its position while avoiding the sun. When cylinders are filled with refrigerants, choosing the right cylinder is important. Despite these limitations, the approach is extensively utilized [83]. The energy efficiency of passive sun trackers using holographic gratings was determined theoretically and practically. In central Russia, they improve solar panel signals in ‘smart’ windows by 20%. A 35% signal enhancement is possible by increasing the angular selectivity contour of the gratings while maintaining good diffraction efficiency. This can be accomplished by creating novel materials with refractive index modulations greater than 0.1 and recording layers approximately 3 m thick or by using well-known hybrid diffractive structures that relieve a volume grating [84].
3.3.2 Active solar trackers
STS, known as active solar trackers, employ motors and sensors and control electronics to rotate solar collectors or panels according to the position of the sun in the sky. Although more expensive and complex than passive solar trackers are, active solar trackers can offer greater accuracy and energy output. The tracker controller, the sensors, the actuator, and the solar panel mount are the four essential parts of an active solar tracker. The tracker controller uses data from sensors to determine the best angle for the solar panel by analysing the position and intensity of the sun. The solar panel mount is moved to the desired position by the actuator, which is typically a motor or a linear actuator [85, 86].
Active solar trackers (shown in Fig. 12) optimize the placement of solar panels for optimum energy production through the implementation of a meticulous control process. A sequence of steps is orchestrated by sensors, actuators, and a controller to complete this complex procedure. By incorporating these data in conjunction with the panel orientation, the controller computes the optimal panel alignment. Next, the actuators receive signals that initiate precise movements, which in turn reposition the panels. By consistently monitoring the position of the panels and utilizing feedback control to refine alignment, the controller guarantees that the panels maintain their perpendicular to the sun’s beams. During this dynamic adjustment process, the solar panels produce electrical energy by taking advantage of the most favourable sunlight conditions. The generated energy is either directly used to power a variety of devices or stored in batteries for subsequent utilization [87, 88].
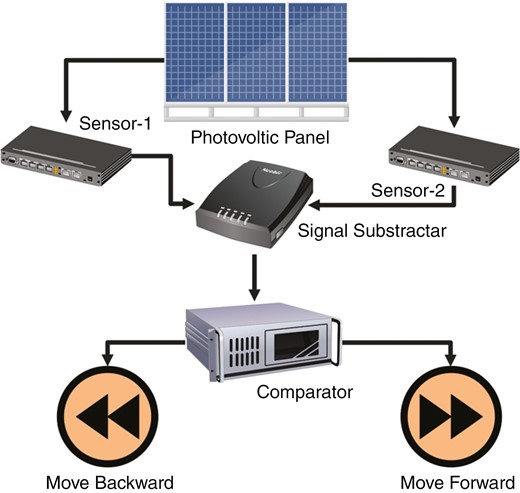
Control process of an active solar tracker using the comparator and signal subtracter.
A common approach is to use several motors linked to an electrical sensor to rotate a PV cell at its core. Figure 13 shows that this is probably the most basic electrical method currently available since it uses a cell to power a motor. The sun’s rays strike the panel at a right angle when this angle reaches zero. In addition, the design’s pivot point was not horizontal; rather, it had one bearing that was lower to the ground than the other, which allowed it to provide the correct elevation for areas beyond the equator. Most solar trackers are electronic, which drains the power from the PV panel for operation and adds extra expenses for installing and maintaining the control system and electric motor [89].
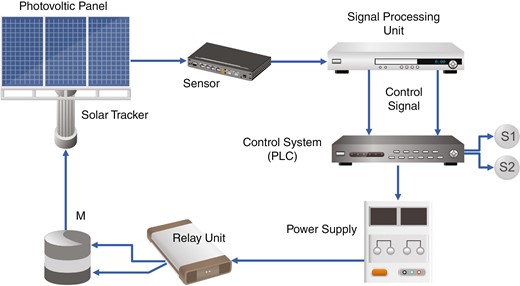
Generic block diagram of the active solar tracker using the programmable logic controller (PLC) and the signal processing unit.
3.4 Types of STS based on control strategies
3.4.1 Closed-loop solar trackers
The feedback control approach underpins the closed-loop solar tracker. A closed-loop tracking system control technique uses light sensors on the solar PV panel to follow the sun at any time of day. If the sun is not directly on the solar panel, the intensities of the light sensors will vary. The tracker’s inclination to face the sun is calculated via this difference [90]. The solar panel has two similar light sensors to track the sun. The east‒west or south‒north orientation helps measure light source strength. For a wide-angle search and quick sun location, an opaque item is placed between the two sensors to isolate light from other orientations. Closed-loop trackers consider two states. The STS is stable if the output signal levels of the two sensors are equal, indicating a zero-output difference and zero motor drive voltage. This shows that the solar PV system followed the sun [91].
3.4.2 Open-loop solar trackers
One type of STS, known as an open-loop solar tracker, operates according to an algorithm or timetable rather than monitoring the real-time location of the sun or any other environmental factors that can affect the efficiency of PV panels. Operating on a timetable or algorithm that considers the time of day, season, and location, an open-loop solar tracker moves the motorized platform that holds the solar panel in line with the sun’s position. To follow the yearly course of the sun, for example, a solar panel can be programmed to travel in an east‒west direction during the day.
Instead of actively seeking the sun, an open-loop solar tracking control approach determines the sun’s position for a certain site. The solar tracker uses time, day, month, and year to locate the sun without feedback. The tracker uses a stepper motor to track the sun without sensors. Therefore, it is called the open-loop tracker, and Fig. 14 shows an open-loop and closed-loop tracker schematic [92].
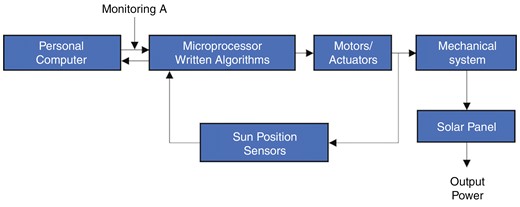
3.5 STS using sensors, time, and date
The initial solar tracking device had a tracking error of 0.14 when it was a sensor-based version, but it only had a tracking error of 0.43 when it was a sensor-less version. During this effort, two innovative STS that make use of two axes were conceived and manufactured. Because of these findings, these tracking errors were far lower than those of modern solar trackers. Compared with a PV module that was stationary, the sensor-based solar tracker resulted in increases of approximately 27.7%, 37.3%, and 42.7% in the average daily obtained solar energy during the winter, spring, autumn, and summer seasons, respectively [93].
3.6 Key advancements, performance analysis, and key findings in the different solar tracking technologies
This study describes the integration of a sun tracker into a solar water heating system to increase thermal efficiency. The transient thermal dynamics of the solar system were studied. This research model can be easily adapted to any solar panel orientation. The model is suitable if the theoretical and experimental results match. This study examined direct and diffuse solar flow as a function of the sun position to estimate solar thermal system operation. This approach optimizes thermal energy use from solar radiation by dynamically altering the solar panel orientation and inclination to track the movement of the sun [94].
The theoretical flux values obtained from both the solar tracker and non-tracker configurations exhibit a high degree of concordance with those derived from the experimental setup. The thermal gain resulting from the use of solar trackers has been assessed in comparison with that of fixed solar panels positioned at varying angles of inclination. The experimental findings are highly consistent with the theoretical model. The analysis of the theoretical and experimental fluxes demonstrated a favourable alignment of the sun’s trajectory with the solar tracker. The present study conducted an experimental comparison between the outcomes obtained for solar panels with fixed inclinations and those obtained with a solar tracker. The results indicate a significant increase of 40% in the overall thermal energy produced. The amount of energy acquired is contingent upon the prevailing season. During summer, low inclinations remain advantageous, whereas during winter, higher inclinations are necessary [95].
A thorough examination of the distinctions between single-axis and dual-axis STS reveals significant variations in tracking capabilities, cost, energy output, ideal applications, and tracking methods, as shown in Table 4. Compared with fixed panels, single-axis tracking systems, which primarily track in one direction and cost between $0.08 and $0.20 per watt for utility-scale initiatives, increase energy production by 20%–30% [102]. These systems are highly suitable for small-scale endeavours that have spatial limitations. On the other hand, dual-axis tracking systems, which are typically priced at $0.18–0.30+ per watt for utility-scale installations, are more complicated and expensive than single-axis systems but provide greater efficiency improvements of 5%–15% [103]. By monitoring both azimuth and elevation, these panels produce 35%–40% more energy than stationary panels do, rendering them well suited for expansive, large-scale endeavours. By utilizing a combination of astronomical data and light intensity, their monitoring method allows them to optimize panel angles for the day [104].
Parameter comparison between the single-axis and dual-axis tracking systems.
Criteria . | Single-axis tracking . | Dual-axis tracking . | References . |
---|---|---|---|
Cost | Range from $0.08 to $0.20 per watt for utility-scale projects | Generally, more expensive due to their increased complexity. Costs can range from $0.18 to $0.30+ per watt for utility-scale installations. | [96] |
Efficiency | Increase energy production by approximately 20%–30% compared to fixed panels | 5%–15% compared to single-axis systems | [97, 98] |
Tracking capability | Limited to one direction | Tracks both azimuth and elevation | |
Ideal application | Small-scale projects with limited space | Large-scale projects with ample space | [99–101] |
Energy output | 20%–25% more energy compared to fixed solar panels | 35%–40% more energy compared to fixed solar panels | |
Tracking method | Tracking based on time or light intensity | Tracking based on a combination of astronomical data and light intensity |
Criteria . | Single-axis tracking . | Dual-axis tracking . | References . |
---|---|---|---|
Cost | Range from $0.08 to $0.20 per watt for utility-scale projects | Generally, more expensive due to their increased complexity. Costs can range from $0.18 to $0.30+ per watt for utility-scale installations. | [96] |
Efficiency | Increase energy production by approximately 20%–30% compared to fixed panels | 5%–15% compared to single-axis systems | [97, 98] |
Tracking capability | Limited to one direction | Tracks both azimuth and elevation | |
Ideal application | Small-scale projects with limited space | Large-scale projects with ample space | [99–101] |
Energy output | 20%–25% more energy compared to fixed solar panels | 35%–40% more energy compared to fixed solar panels | |
Tracking method | Tracking based on time or light intensity | Tracking based on a combination of astronomical data and light intensity |
Parameter comparison between the single-axis and dual-axis tracking systems.
Criteria . | Single-axis tracking . | Dual-axis tracking . | References . |
---|---|---|---|
Cost | Range from $0.08 to $0.20 per watt for utility-scale projects | Generally, more expensive due to their increased complexity. Costs can range from $0.18 to $0.30+ per watt for utility-scale installations. | [96] |
Efficiency | Increase energy production by approximately 20%–30% compared to fixed panels | 5%–15% compared to single-axis systems | [97, 98] |
Tracking capability | Limited to one direction | Tracks both azimuth and elevation | |
Ideal application | Small-scale projects with limited space | Large-scale projects with ample space | [99–101] |
Energy output | 20%–25% more energy compared to fixed solar panels | 35%–40% more energy compared to fixed solar panels | |
Tracking method | Tracking based on time or light intensity | Tracking based on a combination of astronomical data and light intensity |
Criteria . | Single-axis tracking . | Dual-axis tracking . | References . |
---|---|---|---|
Cost | Range from $0.08 to $0.20 per watt for utility-scale projects | Generally, more expensive due to their increased complexity. Costs can range from $0.18 to $0.30+ per watt for utility-scale installations. | [96] |
Efficiency | Increase energy production by approximately 20%–30% compared to fixed panels | 5%–15% compared to single-axis systems | [97, 98] |
Tracking capability | Limited to one direction | Tracks both azimuth and elevation | |
Ideal application | Small-scale projects with limited space | Large-scale projects with ample space | [99–101] |
Energy output | 20%–25% more energy compared to fixed solar panels | 35%–40% more energy compared to fixed solar panels | |
Tracking method | Tracking based on time or light intensity | Tracking based on a combination of astronomical data and light intensity |
The differentiation between the AADAT and the TTDAT in STS is delineated in the comparison in Table 5. Although both tracking methods utilize a two-axis rotation, their axes of rotation are distinct: the azimuth-altitude method is dependent on the azimuth and elevation, whereas tip-tilt method employs tilting and panning motions. Both control mechanisms make use of sensors and feedback circuits. In contrast, the accuracy of the tip-tilt system is 0.2 for a horizontal rotational angle over a 180° range. In contrast, the azimuth-altitude system exhibits exceptional precision without specifying numerical values. In terms of energy efficiency, the tip-tilt sensor exhibits a moderate level of performance in comparison to the azimuth-altitude sensor, which provides a higher degree of efficiency. Additionally, the tip-tilt system has the benefit of requiring less space, accompanied by lower-to-moderate costs and less complicated maintenance requirements. It is more suitable for systems of a modest to medium size. In contrast, the azimuth-altitude tracker is appropriate for utility-scale applications and larger systems despite its increased cost, space requirements, and maintenance complexity. Compared with the tip-tilt tracker, the azimuth-altitude system demonstrates enhanced resistance to adverse weather conditions and wind, as well as greater flexibility in terms of implementation. These differentiations illustrate compromises between the two systems: the tip-tilt system, which is more cost-effective and compact, may be more viable for smaller-scale endeavours. Conversely, the azimuth-altitude system offers advantages for larger installations because of its superior efficiency and adaptability across various conditions.
Criteria . | TTDAT . | AADAT . | References . |
---|---|---|---|
Tracking method | Two-axis rotation | Two-axis rotation | [105–108] |
Axis of rotation | Tilt and pan | Azimuth and elevation | |
Control mechanism | Feedback loop and sensors | Feedback loop and sensors | |
Accuracy | 0.2 horizontal rotating angle: 180° | High as compared to tip-tilted | |
Energy efficiency | 16%–24% when the axis is oriented south‒north | 24%–30% when the axis is oriented south–north | |
Space requirement | Small as compared to fixed tracker | Large as compared to fixed tracker | |
Cost | Low to moderate as compared to fixed trackers | High as compared to tilted trackers | |
Maintenance | Simple as compared to another tracking system | Complex in maintenance | |
Applicability | Small and medium systems | Large systems and utility scale |
Criteria . | TTDAT . | AADAT . | References . |
---|---|---|---|
Tracking method | Two-axis rotation | Two-axis rotation | [105–108] |
Axis of rotation | Tilt and pan | Azimuth and elevation | |
Control mechanism | Feedback loop and sensors | Feedback loop and sensors | |
Accuracy | 0.2 horizontal rotating angle: 180° | High as compared to tip-tilted | |
Energy efficiency | 16%–24% when the axis is oriented south‒north | 24%–30% when the axis is oriented south–north | |
Space requirement | Small as compared to fixed tracker | Large as compared to fixed tracker | |
Cost | Low to moderate as compared to fixed trackers | High as compared to tilted trackers | |
Maintenance | Simple as compared to another tracking system | Complex in maintenance | |
Applicability | Small and medium systems | Large systems and utility scale |
Criteria . | TTDAT . | AADAT . | References . |
---|---|---|---|
Tracking method | Two-axis rotation | Two-axis rotation | [105–108] |
Axis of rotation | Tilt and pan | Azimuth and elevation | |
Control mechanism | Feedback loop and sensors | Feedback loop and sensors | |
Accuracy | 0.2 horizontal rotating angle: 180° | High as compared to tip-tilted | |
Energy efficiency | 16%–24% when the axis is oriented south‒north | 24%–30% when the axis is oriented south–north | |
Space requirement | Small as compared to fixed tracker | Large as compared to fixed tracker | |
Cost | Low to moderate as compared to fixed trackers | High as compared to tilted trackers | |
Maintenance | Simple as compared to another tracking system | Complex in maintenance | |
Applicability | Small and medium systems | Large systems and utility scale |
Criteria . | TTDAT . | AADAT . | References . |
---|---|---|---|
Tracking method | Two-axis rotation | Two-axis rotation | [105–108] |
Axis of rotation | Tilt and pan | Azimuth and elevation | |
Control mechanism | Feedback loop and sensors | Feedback loop and sensors | |
Accuracy | 0.2 horizontal rotating angle: 180° | High as compared to tip-tilted | |
Energy efficiency | 16%–24% when the axis is oriented south‒north | 24%–30% when the axis is oriented south–north | |
Space requirement | Small as compared to fixed tracker | Large as compared to fixed tracker | |
Cost | Low to moderate as compared to fixed trackers | High as compared to tilted trackers | |
Maintenance | Simple as compared to another tracking system | Complex in maintenance | |
Applicability | Small and medium systems | Large systems and utility scale |
The parameter comparisons between the horizontal single axis tracker (HSAT), vertical single axis tracker (VSAT), tilted single-axis tracker, and polar-aligned single-axis tracker are presented in Table 6. Initially, the performance of a PV system was evaluated in a previous study under static conditions. After that, the PV systems were monitored, as they were managed dynamically to follow the path of the sun along two dimensions. Assuming 28 constant tilt angles and an energy rating of 1459 kWh/kWp, the anticipated yearly PV power output is 11.53 MWh. The double-axis sun-tracking system may create 30.79% more solar power than the fixed-latitude tilt method. A solar tracking system that follows the sun’s path along two axes can produce 15.07 MWh per year at an energy rate of 19.08 kWh/kWp [110]. The fixed-tilt PV and STS on the double-axis sun tracker produce 15.98 and 11.53 MWh, respectively. Compared with a fixed PV system inclined at a given latitude, a double-axis sun-tracking system produces 30.79% more electricity. STS employing AS95HPC back-contact monocrystalline silicon PV module systems on a double-axis tracking system have a yearly total energy rating of 1908 kWh/kWp. The fixed PV system should increase by 2.2%, and the double-axis monitoring system should increase by 4.4%.
Parameter comparison between the HSAT, VSAT, tilted single-axis tracker, and polar-aligned single-axis tracker.
Criteria . | HSAT . | VSAT . | Tilted single-axis tracker . | Polar-aligned single-axis tracker . | Reference . |
---|---|---|---|---|---|
Axis of rotation | Rotates around a horizontal axis aligned with the north‒south axis | Rotates around a vertical axis aligned with the east‒west axis | Rotates around a tilted axis aligned with the north‒south axis and oriented at an angle equal to the latitude of the location | Rotates around a polar axis aligned with the Earth’s rotational axis | [109] |
Tracking method | Follows the sun’s daily path from east to west | Follows the sun’s seasonal path from low on the horizon in winter to high on the horizon in summer | Follows both the sun’s daily and seasonal paths by tilting the axis of rotation | Follows both the sun’s daily and seasonal paths by aligning the axis of rotation with the Earth’s rotational axis | |
Applicability | Utility scale | Small and medium systems | Small and medium systems | Small and medium systems |
Criteria . | HSAT . | VSAT . | Tilted single-axis tracker . | Polar-aligned single-axis tracker . | Reference . |
---|---|---|---|---|---|
Axis of rotation | Rotates around a horizontal axis aligned with the north‒south axis | Rotates around a vertical axis aligned with the east‒west axis | Rotates around a tilted axis aligned with the north‒south axis and oriented at an angle equal to the latitude of the location | Rotates around a polar axis aligned with the Earth’s rotational axis | [109] |
Tracking method | Follows the sun’s daily path from east to west | Follows the sun’s seasonal path from low on the horizon in winter to high on the horizon in summer | Follows both the sun’s daily and seasonal paths by tilting the axis of rotation | Follows both the sun’s daily and seasonal paths by aligning the axis of rotation with the Earth’s rotational axis | |
Applicability | Utility scale | Small and medium systems | Small and medium systems | Small and medium systems |
Parameter comparison between the HSAT, VSAT, tilted single-axis tracker, and polar-aligned single-axis tracker.
Criteria . | HSAT . | VSAT . | Tilted single-axis tracker . | Polar-aligned single-axis tracker . | Reference . |
---|---|---|---|---|---|
Axis of rotation | Rotates around a horizontal axis aligned with the north‒south axis | Rotates around a vertical axis aligned with the east‒west axis | Rotates around a tilted axis aligned with the north‒south axis and oriented at an angle equal to the latitude of the location | Rotates around a polar axis aligned with the Earth’s rotational axis | [109] |
Tracking method | Follows the sun’s daily path from east to west | Follows the sun’s seasonal path from low on the horizon in winter to high on the horizon in summer | Follows both the sun’s daily and seasonal paths by tilting the axis of rotation | Follows both the sun’s daily and seasonal paths by aligning the axis of rotation with the Earth’s rotational axis | |
Applicability | Utility scale | Small and medium systems | Small and medium systems | Small and medium systems |
Criteria . | HSAT . | VSAT . | Tilted single-axis tracker . | Polar-aligned single-axis tracker . | Reference . |
---|---|---|---|---|---|
Axis of rotation | Rotates around a horizontal axis aligned with the north‒south axis | Rotates around a vertical axis aligned with the east‒west axis | Rotates around a tilted axis aligned with the north‒south axis and oriented at an angle equal to the latitude of the location | Rotates around a polar axis aligned with the Earth’s rotational axis | [109] |
Tracking method | Follows the sun’s daily path from east to west | Follows the sun’s seasonal path from low on the horizon in winter to high on the horizon in summer | Follows both the sun’s daily and seasonal paths by tilting the axis of rotation | Follows both the sun’s daily and seasonal paths by aligning the axis of rotation with the Earth’s rotational axis | |
Applicability | Utility scale | Small and medium systems | Small and medium systems | Small and medium systems |
The findings of this research indicate that the sensor-based STS outperforms the azimuth-based STS in terms of tracking accuracy and energy efficiency, as it tracks the sun’s rays on the basis of sensor data rather than azimuth measurements. In contrast to sensor-based STS, azimuth-based STS exhibit inferior performance due to their substantial energy consumption and limited tracing precision, as they rely solely on the sun’s theoretical location [111]. The different rotation angles of the single-axis tracking system are shown in Fig. 15.
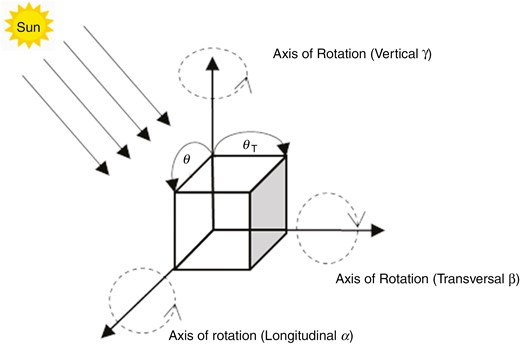
3.6.1 Tracking angle
The tracking angle pertains to the orientation of a solar panel or solar collector with respect to the sun and is aimed at optimizing the quantity of solar radiation it captures. STS employ sensors and motors to modify the alignment of panels or collectors during the day in response to the shifting position of the sun [112].
3.6.2 Collector azimuth
The term ‘collector azimuth’ refers to the directional orientation of the axis of a collector. The angle between the axis of the collector and the direction of the true north is expressed in degrees, with a clockwise orientation from the north.
The inclination of a collector: The collector inclination refers to the angular deviation between the surface of the collector and the horizontal plane. The optimal angle of inclination of the collector is determined by the need to capture the highest possible quantity of solar radiation [113].
3.6.3 Longitudinal angle
The longitudinal angle can be defined as the angular separation between the plane of the collector and the plane that encompasses the projection of the sun’s rays onto the plane of the collector [114].
3.6.4 Transversal angle
The angle of intersection between the plane of the collector and the plane that encompasses the projection of the line that is perpendicular to the axis of the collector onto the plane of the collector is referred to as the transversal angle. The terms ‘azimuth angle’ and ‘orientation angle’ are commonly used to refer to this concept [115].
In Table 7, a concluding table is presented to illustrate the learning outcomes for the different solar tracking technologies. In Table 8, all the key findings of this study are summarized, and concluding remarks are given. The table provides a comprehensive overview of various solar tracking technologies and their respective benefits on the basis of recent research findings.
Type of technology in the system . | Methodology description . | Performance, energy gain, and efficiency . | Key findings . | References . |
---|---|---|---|---|
Fixed- and double-axis STS | Initially, PV systems were of a fixed nature; however, later on, they were regulated to follow the movement of the sun along two axes by making use of solar altitude and azimuth angles. | • The PV system with a fixed tilt generates 11.53 MWh of electricity. • The PV system mounted on a double-axis sun tracker generates 15.98 MWh. | • According to the calculations, the double-axis sun-tracking system generates 30.79% more electricity than the latitude-tilt fixed PV system does. • This difference can be seen when comparing the two systems. | [116] |
Single-axis scheduled STS | This research endeavour is being undertaken to assess the efficiency of a solar system that is stationary, an LDR photo sensor, and a solitary-axis solar tracking mechanism that operates on a programmed schedule under a variety of climatic circumstances. | • The tracker utilized 0.557 Wh of energy during the 5-day experiment, and astronomical estimates put its efficiency at 5.7% when the mechanism that spun the sensor was considered. • On days with cloudy and rainy weather, the planned ST was 4.2% more effective than the LDR ST, but only 1.15% on days with variable cloud cover. | • In diverse weather conditions, the schedule-based STS is 4.2% more efficient than the LDR STS. The proposed tracker was 5.7% more efficient than a fixed PV panel tilted to its ideal angle. • The use of an encoder has resulted in the creation of a method that is capable of accurately determining the azimuth angle of the Sun. | [117] |
Single-axis multiple-position V-trough tracker | PV multiple-position V-trough tracker with reduced reflections and an INSA are theoretically examined for their performance. | When compared to nonconcentrating solar panels of a similar type, concentrating solar panels showed a maximum power point increase of 62%. | • V-trough concentrators are ideal for concentrating sunlight on commercially available solar cells because they are much easier to manufacture than compound concentrators (CPCs). • They have more uniform solar irradiation at their bases, and they are better equipped to disperse excess heat through their sidewalls. | [118] |
Bifacial fixed-tilt single-axis tracking system | • It is possible to model the energy yield of a fixed-tilt bifacial system as a function of the placement height, the number of rows and modules in each row, or all of these factors together. • The data that were measured from a PV system that had constantly shifting tilt angles and the data that were simulated were compared. | In most cases, the GCR for HSAT systems is lower than 35%, but the GCR for fixed systems is often more than 50%. | When comparing monofacial and bifacial tracking gains for a given GCR, the tracking gain for monofacial systems is somewhat higher than that of bifacial systems. | [119] |
One-axis three-position polar-axis-aligned sun tracking | This research proposes and conceptually investigates a new design concept for PV applications that is dubbed one-axis three-position sun-tracking polar-axis-aligned CPCs (3P-CPCs). | The yearly solar gain that was captured by 1P-CPCs was approximately 65%–74% of that which was gathered by fixed EW-aligned CPCs, while the annual solar gain that was obtained by 3P-CPCs was approximately 26%–45% greater. | • The polar-axis single-axis sun-tracking techniques were oriented in the polar-axis direction for effective beam radiation collection practically all day long. | [120] |
Dual-axis PV tracking system | • In this paper, both the design of a dual-axis PV tracking system and the results of experimental testing are discussed. • The output and presentation aspects of the tracking system are handled separately by their respective mechanical and electrical components. | • According to the findings of this investigation, the performance of the dual-axis PV tracking system in comparison to that of the stationary systems was superior by more than 27%. • In regard to solar modules following the course of the sun, the dual-axis tracking system design that is suggested in conjunction with an open-loop control system of electric drives gives outstanding results. | The dual-axis tracking gadget incorporates both mechanical and electronic parts into its construction. The electric circuit of the dual-axis tracking system does a comparison of the resistances of two resistors that depend on the amount of light. (LDR). | [119] |
Azimuth- and sensor-based control strategies for a PV solar tracking application | • Within the scope of this research, a single-axis STS is put into operation, and an azimuth control strategy is developed to improve tracking of the sun. • Real-time calculations of the ideal azimuth angle can be performed by an embedded microprocessor, which takes into account the altitude, date, and time. | Based on the findings, it was determined that the sensor-based STS created 14.8% more net power compared to the azimuth-based STS, whereas the latter generated 7.8% greater power overall. On the other hand, the STS that relied on sensors consumed 192% less power than the latter. | The energy consumption of the STS that was based on sensors was 65% lower than that of the STS that was based on azimuth, which was 150% higher. | [121] |
Filtered sun sensor for solar tracking in HCPV and CSP systems | Infrared and linear polarizing optical filters will be incorporated into a four-quadrant photodiode solar sensor to demonstrate the benefits of doing so. The goal of this effort is to demonstrate such benefits. | • Both indoor and outdoor tracking studies make use of the algorithms found in STs. • These include the PI and PID controllers, as well as a cascade control rule that was recently proposed. | The value of diffuse radiation is rather low when there is no cloud cover, accounting for approximately 7.5% of the total solar radiation. This value in the solar sensor results in low measurement noise levels as compared to other values. | [122] |
Microcontroller-based single-axis PV tracker system on solar panel performance | A solar panel was used in the construction of the system. In addition, two LDR sensors were linked to the north and south sides of the PV, and a servo motor was connected to the Uno board. | The objective of SASTS is to get the most accurate readings possible from the solar panel while the weather is clear and sunny. The precision of this tracking system is 0.85 W/m2, which is rather impressive. | • As a result of the fixed solar panel housing and the high deflection angle, the MPP was significantly less than what was predicted by SASTS. • The mechanism known as SASTS lends a hand in ensuring that the solar panel remains oriented toward the sun and makes full use of the consistent incoming rays, which is the intended result. | [123] |
ST system for solar panels that makes use of the Proteus ISIS 7.6 software | • Proteus 7.6 ISIS was put to use to synchronize an independent STS, and it was successful in doing so. | A STS, utilizing a microcontroller and a low-cost solar sensor, has been developed and simulated. The outcomes of the simulation have been remarkably significant. | The greatest current draw from the sensor is less than 0.5 mA, which demonstrates how little power the sensor consumes while functioning as it is designed. | [124] |
Two-axis STS using flat-mirror reflectors | This study investigated the voltage, electric current, power, and efficiency of a STS equipped with two- and four-sided reflectors positioned at angles of 90°, 120°, and 150° relative to the solar panel. | At a 90° angle, the four-sided flat mirrors were more effective at blocking some sun rays than the two-sided ones; however, at a 150° angle, the rays could not be reflected to the panel. Because of this, two-sided flat mirrors were preferred to four-sided ones. | The fact that the four-sided flat mirrors had an additional two flat mirrors that blocked some of the sun’s rays gave the reflectors with two flat mirrors on each side an advantage when viewed from an angle of 90°. | [125] |
Three-axis STS | In the proposed approach, both the manufacturing and installation of a solar panel mount that is outfitted with a solar tracking controller that has multiple axes are detailed. | • According to this study, the three-axis ST has a high efficiency as compared with the fixed-axis STS. • In comparison, the efficiency of the STS with a fixed axis is just 18%, while the STS with three axes achieves 24.9%. | The concept of the suggested model can be put into action by connecting to the national grid, which can provide exceptional assistance at a time when it is most needed. | [126] |
A multistage hybrid deep learning model for enhanced solar tracking | Through the combination of normalization techniques and the transformation of numerical data into pictures, the suggested model enhances the representation of features in the data. | On a freely accessible dataset, the suggested hybrid model performs better than current approaches, obtaining exceptional results with MAE, MAPE, and RMSE scores of 0.0073, 1.4635, and 0.0097, respectively. | • Approximately 25% of the data acquired over a period of 3 years, or 272 days, were used to train the model. • This could restrict how broadly applicable the model is. Therefore, for better sun tracking, future research should look at training the model on a larger dataset and examining the integration of image and tabular data. | [127] |
Type of technology in the system . | Methodology description . | Performance, energy gain, and efficiency . | Key findings . | References . |
---|---|---|---|---|
Fixed- and double-axis STS | Initially, PV systems were of a fixed nature; however, later on, they were regulated to follow the movement of the sun along two axes by making use of solar altitude and azimuth angles. | • The PV system with a fixed tilt generates 11.53 MWh of electricity. • The PV system mounted on a double-axis sun tracker generates 15.98 MWh. | • According to the calculations, the double-axis sun-tracking system generates 30.79% more electricity than the latitude-tilt fixed PV system does. • This difference can be seen when comparing the two systems. | [116] |
Single-axis scheduled STS | This research endeavour is being undertaken to assess the efficiency of a solar system that is stationary, an LDR photo sensor, and a solitary-axis solar tracking mechanism that operates on a programmed schedule under a variety of climatic circumstances. | • The tracker utilized 0.557 Wh of energy during the 5-day experiment, and astronomical estimates put its efficiency at 5.7% when the mechanism that spun the sensor was considered. • On days with cloudy and rainy weather, the planned ST was 4.2% more effective than the LDR ST, but only 1.15% on days with variable cloud cover. | • In diverse weather conditions, the schedule-based STS is 4.2% more efficient than the LDR STS. The proposed tracker was 5.7% more efficient than a fixed PV panel tilted to its ideal angle. • The use of an encoder has resulted in the creation of a method that is capable of accurately determining the azimuth angle of the Sun. | [117] |
Single-axis multiple-position V-trough tracker | PV multiple-position V-trough tracker with reduced reflections and an INSA are theoretically examined for their performance. | When compared to nonconcentrating solar panels of a similar type, concentrating solar panels showed a maximum power point increase of 62%. | • V-trough concentrators are ideal for concentrating sunlight on commercially available solar cells because they are much easier to manufacture than compound concentrators (CPCs). • They have more uniform solar irradiation at their bases, and they are better equipped to disperse excess heat through their sidewalls. | [118] |
Bifacial fixed-tilt single-axis tracking system | • It is possible to model the energy yield of a fixed-tilt bifacial system as a function of the placement height, the number of rows and modules in each row, or all of these factors together. • The data that were measured from a PV system that had constantly shifting tilt angles and the data that were simulated were compared. | In most cases, the GCR for HSAT systems is lower than 35%, but the GCR for fixed systems is often more than 50%. | When comparing monofacial and bifacial tracking gains for a given GCR, the tracking gain for monofacial systems is somewhat higher than that of bifacial systems. | [119] |
One-axis three-position polar-axis-aligned sun tracking | This research proposes and conceptually investigates a new design concept for PV applications that is dubbed one-axis three-position sun-tracking polar-axis-aligned CPCs (3P-CPCs). | The yearly solar gain that was captured by 1P-CPCs was approximately 65%–74% of that which was gathered by fixed EW-aligned CPCs, while the annual solar gain that was obtained by 3P-CPCs was approximately 26%–45% greater. | • The polar-axis single-axis sun-tracking techniques were oriented in the polar-axis direction for effective beam radiation collection practically all day long. | [120] |
Dual-axis PV tracking system | • In this paper, both the design of a dual-axis PV tracking system and the results of experimental testing are discussed. • The output and presentation aspects of the tracking system are handled separately by their respective mechanical and electrical components. | • According to the findings of this investigation, the performance of the dual-axis PV tracking system in comparison to that of the stationary systems was superior by more than 27%. • In regard to solar modules following the course of the sun, the dual-axis tracking system design that is suggested in conjunction with an open-loop control system of electric drives gives outstanding results. | The dual-axis tracking gadget incorporates both mechanical and electronic parts into its construction. The electric circuit of the dual-axis tracking system does a comparison of the resistances of two resistors that depend on the amount of light. (LDR). | [119] |
Azimuth- and sensor-based control strategies for a PV solar tracking application | • Within the scope of this research, a single-axis STS is put into operation, and an azimuth control strategy is developed to improve tracking of the sun. • Real-time calculations of the ideal azimuth angle can be performed by an embedded microprocessor, which takes into account the altitude, date, and time. | Based on the findings, it was determined that the sensor-based STS created 14.8% more net power compared to the azimuth-based STS, whereas the latter generated 7.8% greater power overall. On the other hand, the STS that relied on sensors consumed 192% less power than the latter. | The energy consumption of the STS that was based on sensors was 65% lower than that of the STS that was based on azimuth, which was 150% higher. | [121] |
Filtered sun sensor for solar tracking in HCPV and CSP systems | Infrared and linear polarizing optical filters will be incorporated into a four-quadrant photodiode solar sensor to demonstrate the benefits of doing so. The goal of this effort is to demonstrate such benefits. | • Both indoor and outdoor tracking studies make use of the algorithms found in STs. • These include the PI and PID controllers, as well as a cascade control rule that was recently proposed. | The value of diffuse radiation is rather low when there is no cloud cover, accounting for approximately 7.5% of the total solar radiation. This value in the solar sensor results in low measurement noise levels as compared to other values. | [122] |
Microcontroller-based single-axis PV tracker system on solar panel performance | A solar panel was used in the construction of the system. In addition, two LDR sensors were linked to the north and south sides of the PV, and a servo motor was connected to the Uno board. | The objective of SASTS is to get the most accurate readings possible from the solar panel while the weather is clear and sunny. The precision of this tracking system is 0.85 W/m2, which is rather impressive. | • As a result of the fixed solar panel housing and the high deflection angle, the MPP was significantly less than what was predicted by SASTS. • The mechanism known as SASTS lends a hand in ensuring that the solar panel remains oriented toward the sun and makes full use of the consistent incoming rays, which is the intended result. | [123] |
ST system for solar panels that makes use of the Proteus ISIS 7.6 software | • Proteus 7.6 ISIS was put to use to synchronize an independent STS, and it was successful in doing so. | A STS, utilizing a microcontroller and a low-cost solar sensor, has been developed and simulated. The outcomes of the simulation have been remarkably significant. | The greatest current draw from the sensor is less than 0.5 mA, which demonstrates how little power the sensor consumes while functioning as it is designed. | [124] |
Two-axis STS using flat-mirror reflectors | This study investigated the voltage, electric current, power, and efficiency of a STS equipped with two- and four-sided reflectors positioned at angles of 90°, 120°, and 150° relative to the solar panel. | At a 90° angle, the four-sided flat mirrors were more effective at blocking some sun rays than the two-sided ones; however, at a 150° angle, the rays could not be reflected to the panel. Because of this, two-sided flat mirrors were preferred to four-sided ones. | The fact that the four-sided flat mirrors had an additional two flat mirrors that blocked some of the sun’s rays gave the reflectors with two flat mirrors on each side an advantage when viewed from an angle of 90°. | [125] |
Three-axis STS | In the proposed approach, both the manufacturing and installation of a solar panel mount that is outfitted with a solar tracking controller that has multiple axes are detailed. | • According to this study, the three-axis ST has a high efficiency as compared with the fixed-axis STS. • In comparison, the efficiency of the STS with a fixed axis is just 18%, while the STS with three axes achieves 24.9%. | The concept of the suggested model can be put into action by connecting to the national grid, which can provide exceptional assistance at a time when it is most needed. | [126] |
A multistage hybrid deep learning model for enhanced solar tracking | Through the combination of normalization techniques and the transformation of numerical data into pictures, the suggested model enhances the representation of features in the data. | On a freely accessible dataset, the suggested hybrid model performs better than current approaches, obtaining exceptional results with MAE, MAPE, and RMSE scores of 0.0073, 1.4635, and 0.0097, respectively. | • Approximately 25% of the data acquired over a period of 3 years, or 272 days, were used to train the model. • This could restrict how broadly applicable the model is. Therefore, for better sun tracking, future research should look at training the model on a larger dataset and examining the integration of image and tabular data. | [127] |
CPC, compound parabolic concentrator; CSP, concentrated solar power; EW, east–west; GCR, ground coverage ratio; HCPV, high-concentration PV; INSA, inclination north‒south axis; LDR, light-dependent resistor; MAE, mean absolute error; MAPE, mean absolute percentage error; MPP, maximum power point; PI, proportional–integral; PID, proportional–integral–derivative; RMSE, root mean square error; SASTS, single-axis solar tracking system; ST, solar tracker.
Type of technology in the system . | Methodology description . | Performance, energy gain, and efficiency . | Key findings . | References . |
---|---|---|---|---|
Fixed- and double-axis STS | Initially, PV systems were of a fixed nature; however, later on, they were regulated to follow the movement of the sun along two axes by making use of solar altitude and azimuth angles. | • The PV system with a fixed tilt generates 11.53 MWh of electricity. • The PV system mounted on a double-axis sun tracker generates 15.98 MWh. | • According to the calculations, the double-axis sun-tracking system generates 30.79% more electricity than the latitude-tilt fixed PV system does. • This difference can be seen when comparing the two systems. | [116] |
Single-axis scheduled STS | This research endeavour is being undertaken to assess the efficiency of a solar system that is stationary, an LDR photo sensor, and a solitary-axis solar tracking mechanism that operates on a programmed schedule under a variety of climatic circumstances. | • The tracker utilized 0.557 Wh of energy during the 5-day experiment, and astronomical estimates put its efficiency at 5.7% when the mechanism that spun the sensor was considered. • On days with cloudy and rainy weather, the planned ST was 4.2% more effective than the LDR ST, but only 1.15% on days with variable cloud cover. | • In diverse weather conditions, the schedule-based STS is 4.2% more efficient than the LDR STS. The proposed tracker was 5.7% more efficient than a fixed PV panel tilted to its ideal angle. • The use of an encoder has resulted in the creation of a method that is capable of accurately determining the azimuth angle of the Sun. | [117] |
Single-axis multiple-position V-trough tracker | PV multiple-position V-trough tracker with reduced reflections and an INSA are theoretically examined for their performance. | When compared to nonconcentrating solar panels of a similar type, concentrating solar panels showed a maximum power point increase of 62%. | • V-trough concentrators are ideal for concentrating sunlight on commercially available solar cells because they are much easier to manufacture than compound concentrators (CPCs). • They have more uniform solar irradiation at their bases, and they are better equipped to disperse excess heat through their sidewalls. | [118] |
Bifacial fixed-tilt single-axis tracking system | • It is possible to model the energy yield of a fixed-tilt bifacial system as a function of the placement height, the number of rows and modules in each row, or all of these factors together. • The data that were measured from a PV system that had constantly shifting tilt angles and the data that were simulated were compared. | In most cases, the GCR for HSAT systems is lower than 35%, but the GCR for fixed systems is often more than 50%. | When comparing monofacial and bifacial tracking gains for a given GCR, the tracking gain for monofacial systems is somewhat higher than that of bifacial systems. | [119] |
One-axis three-position polar-axis-aligned sun tracking | This research proposes and conceptually investigates a new design concept for PV applications that is dubbed one-axis three-position sun-tracking polar-axis-aligned CPCs (3P-CPCs). | The yearly solar gain that was captured by 1P-CPCs was approximately 65%–74% of that which was gathered by fixed EW-aligned CPCs, while the annual solar gain that was obtained by 3P-CPCs was approximately 26%–45% greater. | • The polar-axis single-axis sun-tracking techniques were oriented in the polar-axis direction for effective beam radiation collection practically all day long. | [120] |
Dual-axis PV tracking system | • In this paper, both the design of a dual-axis PV tracking system and the results of experimental testing are discussed. • The output and presentation aspects of the tracking system are handled separately by their respective mechanical and electrical components. | • According to the findings of this investigation, the performance of the dual-axis PV tracking system in comparison to that of the stationary systems was superior by more than 27%. • In regard to solar modules following the course of the sun, the dual-axis tracking system design that is suggested in conjunction with an open-loop control system of electric drives gives outstanding results. | The dual-axis tracking gadget incorporates both mechanical and electronic parts into its construction. The electric circuit of the dual-axis tracking system does a comparison of the resistances of two resistors that depend on the amount of light. (LDR). | [119] |
Azimuth- and sensor-based control strategies for a PV solar tracking application | • Within the scope of this research, a single-axis STS is put into operation, and an azimuth control strategy is developed to improve tracking of the sun. • Real-time calculations of the ideal azimuth angle can be performed by an embedded microprocessor, which takes into account the altitude, date, and time. | Based on the findings, it was determined that the sensor-based STS created 14.8% more net power compared to the azimuth-based STS, whereas the latter generated 7.8% greater power overall. On the other hand, the STS that relied on sensors consumed 192% less power than the latter. | The energy consumption of the STS that was based on sensors was 65% lower than that of the STS that was based on azimuth, which was 150% higher. | [121] |
Filtered sun sensor for solar tracking in HCPV and CSP systems | Infrared and linear polarizing optical filters will be incorporated into a four-quadrant photodiode solar sensor to demonstrate the benefits of doing so. The goal of this effort is to demonstrate such benefits. | • Both indoor and outdoor tracking studies make use of the algorithms found in STs. • These include the PI and PID controllers, as well as a cascade control rule that was recently proposed. | The value of diffuse radiation is rather low when there is no cloud cover, accounting for approximately 7.5% of the total solar radiation. This value in the solar sensor results in low measurement noise levels as compared to other values. | [122] |
Microcontroller-based single-axis PV tracker system on solar panel performance | A solar panel was used in the construction of the system. In addition, two LDR sensors were linked to the north and south sides of the PV, and a servo motor was connected to the Uno board. | The objective of SASTS is to get the most accurate readings possible from the solar panel while the weather is clear and sunny. The precision of this tracking system is 0.85 W/m2, which is rather impressive. | • As a result of the fixed solar panel housing and the high deflection angle, the MPP was significantly less than what was predicted by SASTS. • The mechanism known as SASTS lends a hand in ensuring that the solar panel remains oriented toward the sun and makes full use of the consistent incoming rays, which is the intended result. | [123] |
ST system for solar panels that makes use of the Proteus ISIS 7.6 software | • Proteus 7.6 ISIS was put to use to synchronize an independent STS, and it was successful in doing so. | A STS, utilizing a microcontroller and a low-cost solar sensor, has been developed and simulated. The outcomes of the simulation have been remarkably significant. | The greatest current draw from the sensor is less than 0.5 mA, which demonstrates how little power the sensor consumes while functioning as it is designed. | [124] |
Two-axis STS using flat-mirror reflectors | This study investigated the voltage, electric current, power, and efficiency of a STS equipped with two- and four-sided reflectors positioned at angles of 90°, 120°, and 150° relative to the solar panel. | At a 90° angle, the four-sided flat mirrors were more effective at blocking some sun rays than the two-sided ones; however, at a 150° angle, the rays could not be reflected to the panel. Because of this, two-sided flat mirrors were preferred to four-sided ones. | The fact that the four-sided flat mirrors had an additional two flat mirrors that blocked some of the sun’s rays gave the reflectors with two flat mirrors on each side an advantage when viewed from an angle of 90°. | [125] |
Three-axis STS | In the proposed approach, both the manufacturing and installation of a solar panel mount that is outfitted with a solar tracking controller that has multiple axes are detailed. | • According to this study, the three-axis ST has a high efficiency as compared with the fixed-axis STS. • In comparison, the efficiency of the STS with a fixed axis is just 18%, while the STS with three axes achieves 24.9%. | The concept of the suggested model can be put into action by connecting to the national grid, which can provide exceptional assistance at a time when it is most needed. | [126] |
A multistage hybrid deep learning model for enhanced solar tracking | Through the combination of normalization techniques and the transformation of numerical data into pictures, the suggested model enhances the representation of features in the data. | On a freely accessible dataset, the suggested hybrid model performs better than current approaches, obtaining exceptional results with MAE, MAPE, and RMSE scores of 0.0073, 1.4635, and 0.0097, respectively. | • Approximately 25% of the data acquired over a period of 3 years, or 272 days, were used to train the model. • This could restrict how broadly applicable the model is. Therefore, for better sun tracking, future research should look at training the model on a larger dataset and examining the integration of image and tabular data. | [127] |
Type of technology in the system . | Methodology description . | Performance, energy gain, and efficiency . | Key findings . | References . |
---|---|---|---|---|
Fixed- and double-axis STS | Initially, PV systems were of a fixed nature; however, later on, they were regulated to follow the movement of the sun along two axes by making use of solar altitude and azimuth angles. | • The PV system with a fixed tilt generates 11.53 MWh of electricity. • The PV system mounted on a double-axis sun tracker generates 15.98 MWh. | • According to the calculations, the double-axis sun-tracking system generates 30.79% more electricity than the latitude-tilt fixed PV system does. • This difference can be seen when comparing the two systems. | [116] |
Single-axis scheduled STS | This research endeavour is being undertaken to assess the efficiency of a solar system that is stationary, an LDR photo sensor, and a solitary-axis solar tracking mechanism that operates on a programmed schedule under a variety of climatic circumstances. | • The tracker utilized 0.557 Wh of energy during the 5-day experiment, and astronomical estimates put its efficiency at 5.7% when the mechanism that spun the sensor was considered. • On days with cloudy and rainy weather, the planned ST was 4.2% more effective than the LDR ST, but only 1.15% on days with variable cloud cover. | • In diverse weather conditions, the schedule-based STS is 4.2% more efficient than the LDR STS. The proposed tracker was 5.7% more efficient than a fixed PV panel tilted to its ideal angle. • The use of an encoder has resulted in the creation of a method that is capable of accurately determining the azimuth angle of the Sun. | [117] |
Single-axis multiple-position V-trough tracker | PV multiple-position V-trough tracker with reduced reflections and an INSA are theoretically examined for their performance. | When compared to nonconcentrating solar panels of a similar type, concentrating solar panels showed a maximum power point increase of 62%. | • V-trough concentrators are ideal for concentrating sunlight on commercially available solar cells because they are much easier to manufacture than compound concentrators (CPCs). • They have more uniform solar irradiation at their bases, and they are better equipped to disperse excess heat through their sidewalls. | [118] |
Bifacial fixed-tilt single-axis tracking system | • It is possible to model the energy yield of a fixed-tilt bifacial system as a function of the placement height, the number of rows and modules in each row, or all of these factors together. • The data that were measured from a PV system that had constantly shifting tilt angles and the data that were simulated were compared. | In most cases, the GCR for HSAT systems is lower than 35%, but the GCR for fixed systems is often more than 50%. | When comparing monofacial and bifacial tracking gains for a given GCR, the tracking gain for monofacial systems is somewhat higher than that of bifacial systems. | [119] |
One-axis three-position polar-axis-aligned sun tracking | This research proposes and conceptually investigates a new design concept for PV applications that is dubbed one-axis three-position sun-tracking polar-axis-aligned CPCs (3P-CPCs). | The yearly solar gain that was captured by 1P-CPCs was approximately 65%–74% of that which was gathered by fixed EW-aligned CPCs, while the annual solar gain that was obtained by 3P-CPCs was approximately 26%–45% greater. | • The polar-axis single-axis sun-tracking techniques were oriented in the polar-axis direction for effective beam radiation collection practically all day long. | [120] |
Dual-axis PV tracking system | • In this paper, both the design of a dual-axis PV tracking system and the results of experimental testing are discussed. • The output and presentation aspects of the tracking system are handled separately by their respective mechanical and electrical components. | • According to the findings of this investigation, the performance of the dual-axis PV tracking system in comparison to that of the stationary systems was superior by more than 27%. • In regard to solar modules following the course of the sun, the dual-axis tracking system design that is suggested in conjunction with an open-loop control system of electric drives gives outstanding results. | The dual-axis tracking gadget incorporates both mechanical and electronic parts into its construction. The electric circuit of the dual-axis tracking system does a comparison of the resistances of two resistors that depend on the amount of light. (LDR). | [119] |
Azimuth- and sensor-based control strategies for a PV solar tracking application | • Within the scope of this research, a single-axis STS is put into operation, and an azimuth control strategy is developed to improve tracking of the sun. • Real-time calculations of the ideal azimuth angle can be performed by an embedded microprocessor, which takes into account the altitude, date, and time. | Based on the findings, it was determined that the sensor-based STS created 14.8% more net power compared to the azimuth-based STS, whereas the latter generated 7.8% greater power overall. On the other hand, the STS that relied on sensors consumed 192% less power than the latter. | The energy consumption of the STS that was based on sensors was 65% lower than that of the STS that was based on azimuth, which was 150% higher. | [121] |
Filtered sun sensor for solar tracking in HCPV and CSP systems | Infrared and linear polarizing optical filters will be incorporated into a four-quadrant photodiode solar sensor to demonstrate the benefits of doing so. The goal of this effort is to demonstrate such benefits. | • Both indoor and outdoor tracking studies make use of the algorithms found in STs. • These include the PI and PID controllers, as well as a cascade control rule that was recently proposed. | The value of diffuse radiation is rather low when there is no cloud cover, accounting for approximately 7.5% of the total solar radiation. This value in the solar sensor results in low measurement noise levels as compared to other values. | [122] |
Microcontroller-based single-axis PV tracker system on solar panel performance | A solar panel was used in the construction of the system. In addition, two LDR sensors were linked to the north and south sides of the PV, and a servo motor was connected to the Uno board. | The objective of SASTS is to get the most accurate readings possible from the solar panel while the weather is clear and sunny. The precision of this tracking system is 0.85 W/m2, which is rather impressive. | • As a result of the fixed solar panel housing and the high deflection angle, the MPP was significantly less than what was predicted by SASTS. • The mechanism known as SASTS lends a hand in ensuring that the solar panel remains oriented toward the sun and makes full use of the consistent incoming rays, which is the intended result. | [123] |
ST system for solar panels that makes use of the Proteus ISIS 7.6 software | • Proteus 7.6 ISIS was put to use to synchronize an independent STS, and it was successful in doing so. | A STS, utilizing a microcontroller and a low-cost solar sensor, has been developed and simulated. The outcomes of the simulation have been remarkably significant. | The greatest current draw from the sensor is less than 0.5 mA, which demonstrates how little power the sensor consumes while functioning as it is designed. | [124] |
Two-axis STS using flat-mirror reflectors | This study investigated the voltage, electric current, power, and efficiency of a STS equipped with two- and four-sided reflectors positioned at angles of 90°, 120°, and 150° relative to the solar panel. | At a 90° angle, the four-sided flat mirrors were more effective at blocking some sun rays than the two-sided ones; however, at a 150° angle, the rays could not be reflected to the panel. Because of this, two-sided flat mirrors were preferred to four-sided ones. | The fact that the four-sided flat mirrors had an additional two flat mirrors that blocked some of the sun’s rays gave the reflectors with two flat mirrors on each side an advantage when viewed from an angle of 90°. | [125] |
Three-axis STS | In the proposed approach, both the manufacturing and installation of a solar panel mount that is outfitted with a solar tracking controller that has multiple axes are detailed. | • According to this study, the three-axis ST has a high efficiency as compared with the fixed-axis STS. • In comparison, the efficiency of the STS with a fixed axis is just 18%, while the STS with three axes achieves 24.9%. | The concept of the suggested model can be put into action by connecting to the national grid, which can provide exceptional assistance at a time when it is most needed. | [126] |
A multistage hybrid deep learning model for enhanced solar tracking | Through the combination of normalization techniques and the transformation of numerical data into pictures, the suggested model enhances the representation of features in the data. | On a freely accessible dataset, the suggested hybrid model performs better than current approaches, obtaining exceptional results with MAE, MAPE, and RMSE scores of 0.0073, 1.4635, and 0.0097, respectively. | • Approximately 25% of the data acquired over a period of 3 years, or 272 days, were used to train the model. • This could restrict how broadly applicable the model is. Therefore, for better sun tracking, future research should look at training the model on a larger dataset and examining the integration of image and tabular data. | [127] |
CPC, compound parabolic concentrator; CSP, concentrated solar power; EW, east–west; GCR, ground coverage ratio; HCPV, high-concentration PV; INSA, inclination north‒south axis; LDR, light-dependent resistor; MAE, mean absolute error; MAPE, mean absolute percentage error; MPP, maximum power point; PI, proportional–integral; PID, proportional–integral–derivative; RMSE, root mean square error; SASTS, single-axis solar tracking system; ST, solar tracker.
Solar tracking technology . | Concluding remarks and learning outcomes . |
---|---|
Fixed- and double-axis STS | Double-axis sun-tracking system generates 30.79% more electricity than the fixed PV system. |
Single-axis scheduled STS | Schedule-based STS is 4.2% more efficient than LDR-based single-axis solar trackers in diverse weather conditions and 5.7% more efficient than a fixed PV panel tilted to its ideal angle. |
Single-axis multiple-position V-trough tracker | V-trough concentrators are easier to manufacture and better at dispersing excess heat, with a multiple-axis tracking, maximum power point increase of 62% compared to nonconcentrating panels. |
Bifacial fixed-tilt single-axis tracking system | GCR for HSAT systems is generally lower than 35%, while for fixed systems, it is often more than 50%. Monofacial systems show higher tracking gains than bifacial systems. |
One-axis three-position polar-axis-aligned sun tracking | Annual solar gain from 3P-CPCs is approximately 26%–45% greater than fixed EW-CPCs. |
Dual-axis PV tracking system | Dual-axis PV tracking system shows over 27% better performance compared to stationary systems. |
Azimuth- and sensor-based control strategies | Sensor-based STS generated 14.8% more net power and consumed 19% less power than azimuth-based STS. |
Filtered sun sensor for solar tracking in HCPV and CSP system | Low measurement noise levels in solar sensors with polarizing optical filters, leading to better tracking accuracy. |
Microcontroller-based single-axis PV tracker | SASTS ensures accurate solar panel orientation with a precision of 0.85 W/m2, significantly enhancing MPP under clear conditions. |
STS using Proteus ISIS 7.6 software | Low-power STS developed and simulated using Proteus 7.6 ISIS, demonstrating significant energy efficiency. |
Two-axis STS using flat-mirror reflectors | Two-sided flat mirrors at 90° angle are more effective in blocking some sun rays and reflecting them to the panel. |
Three-axis STS | Three-axis solar tracker achieves 24.9% efficiency compared to fixed-axis system, with potential for grid integration. |
Multistage hybrid deep learning model for solar tracking | Hybrid model shows better performance on a dataset with exceptional results, though training on a larger dataset is needed for broader applicability. |
Solar tracking technology . | Concluding remarks and learning outcomes . |
---|---|
Fixed- and double-axis STS | Double-axis sun-tracking system generates 30.79% more electricity than the fixed PV system. |
Single-axis scheduled STS | Schedule-based STS is 4.2% more efficient than LDR-based single-axis solar trackers in diverse weather conditions and 5.7% more efficient than a fixed PV panel tilted to its ideal angle. |
Single-axis multiple-position V-trough tracker | V-trough concentrators are easier to manufacture and better at dispersing excess heat, with a multiple-axis tracking, maximum power point increase of 62% compared to nonconcentrating panels. |
Bifacial fixed-tilt single-axis tracking system | GCR for HSAT systems is generally lower than 35%, while for fixed systems, it is often more than 50%. Monofacial systems show higher tracking gains than bifacial systems. |
One-axis three-position polar-axis-aligned sun tracking | Annual solar gain from 3P-CPCs is approximately 26%–45% greater than fixed EW-CPCs. |
Dual-axis PV tracking system | Dual-axis PV tracking system shows over 27% better performance compared to stationary systems. |
Azimuth- and sensor-based control strategies | Sensor-based STS generated 14.8% more net power and consumed 19% less power than azimuth-based STS. |
Filtered sun sensor for solar tracking in HCPV and CSP system | Low measurement noise levels in solar sensors with polarizing optical filters, leading to better tracking accuracy. |
Microcontroller-based single-axis PV tracker | SASTS ensures accurate solar panel orientation with a precision of 0.85 W/m2, significantly enhancing MPP under clear conditions. |
STS using Proteus ISIS 7.6 software | Low-power STS developed and simulated using Proteus 7.6 ISIS, demonstrating significant energy efficiency. |
Two-axis STS using flat-mirror reflectors | Two-sided flat mirrors at 90° angle are more effective in blocking some sun rays and reflecting them to the panel. |
Three-axis STS | Three-axis solar tracker achieves 24.9% efficiency compared to fixed-axis system, with potential for grid integration. |
Multistage hybrid deep learning model for solar tracking | Hybrid model shows better performance on a dataset with exceptional results, though training on a larger dataset is needed for broader applicability. |
3P-CPC, three-position compound parabolic concentrator; CSP, concentrated solar power; EW-CPC, east–west compound parabolic concentrator; GCR, ground coverage ratio; HCPV, high-concentration PV; LDR, light-dependent resistor; SASTS, single-axis solar tracking system.
Solar tracking technology . | Concluding remarks and learning outcomes . |
---|---|
Fixed- and double-axis STS | Double-axis sun-tracking system generates 30.79% more electricity than the fixed PV system. |
Single-axis scheduled STS | Schedule-based STS is 4.2% more efficient than LDR-based single-axis solar trackers in diverse weather conditions and 5.7% more efficient than a fixed PV panel tilted to its ideal angle. |
Single-axis multiple-position V-trough tracker | V-trough concentrators are easier to manufacture and better at dispersing excess heat, with a multiple-axis tracking, maximum power point increase of 62% compared to nonconcentrating panels. |
Bifacial fixed-tilt single-axis tracking system | GCR for HSAT systems is generally lower than 35%, while for fixed systems, it is often more than 50%. Monofacial systems show higher tracking gains than bifacial systems. |
One-axis three-position polar-axis-aligned sun tracking | Annual solar gain from 3P-CPCs is approximately 26%–45% greater than fixed EW-CPCs. |
Dual-axis PV tracking system | Dual-axis PV tracking system shows over 27% better performance compared to stationary systems. |
Azimuth- and sensor-based control strategies | Sensor-based STS generated 14.8% more net power and consumed 19% less power than azimuth-based STS. |
Filtered sun sensor for solar tracking in HCPV and CSP system | Low measurement noise levels in solar sensors with polarizing optical filters, leading to better tracking accuracy. |
Microcontroller-based single-axis PV tracker | SASTS ensures accurate solar panel orientation with a precision of 0.85 W/m2, significantly enhancing MPP under clear conditions. |
STS using Proteus ISIS 7.6 software | Low-power STS developed and simulated using Proteus 7.6 ISIS, demonstrating significant energy efficiency. |
Two-axis STS using flat-mirror reflectors | Two-sided flat mirrors at 90° angle are more effective in blocking some sun rays and reflecting them to the panel. |
Three-axis STS | Three-axis solar tracker achieves 24.9% efficiency compared to fixed-axis system, with potential for grid integration. |
Multistage hybrid deep learning model for solar tracking | Hybrid model shows better performance on a dataset with exceptional results, though training on a larger dataset is needed for broader applicability. |
Solar tracking technology . | Concluding remarks and learning outcomes . |
---|---|
Fixed- and double-axis STS | Double-axis sun-tracking system generates 30.79% more electricity than the fixed PV system. |
Single-axis scheduled STS | Schedule-based STS is 4.2% more efficient than LDR-based single-axis solar trackers in diverse weather conditions and 5.7% more efficient than a fixed PV panel tilted to its ideal angle. |
Single-axis multiple-position V-trough tracker | V-trough concentrators are easier to manufacture and better at dispersing excess heat, with a multiple-axis tracking, maximum power point increase of 62% compared to nonconcentrating panels. |
Bifacial fixed-tilt single-axis tracking system | GCR for HSAT systems is generally lower than 35%, while for fixed systems, it is often more than 50%. Monofacial systems show higher tracking gains than bifacial systems. |
One-axis three-position polar-axis-aligned sun tracking | Annual solar gain from 3P-CPCs is approximately 26%–45% greater than fixed EW-CPCs. |
Dual-axis PV tracking system | Dual-axis PV tracking system shows over 27% better performance compared to stationary systems. |
Azimuth- and sensor-based control strategies | Sensor-based STS generated 14.8% more net power and consumed 19% less power than azimuth-based STS. |
Filtered sun sensor for solar tracking in HCPV and CSP system | Low measurement noise levels in solar sensors with polarizing optical filters, leading to better tracking accuracy. |
Microcontroller-based single-axis PV tracker | SASTS ensures accurate solar panel orientation with a precision of 0.85 W/m2, significantly enhancing MPP under clear conditions. |
STS using Proteus ISIS 7.6 software | Low-power STS developed and simulated using Proteus 7.6 ISIS, demonstrating significant energy efficiency. |
Two-axis STS using flat-mirror reflectors | Two-sided flat mirrors at 90° angle are more effective in blocking some sun rays and reflecting them to the panel. |
Three-axis STS | Three-axis solar tracker achieves 24.9% efficiency compared to fixed-axis system, with potential for grid integration. |
Multistage hybrid deep learning model for solar tracking | Hybrid model shows better performance on a dataset with exceptional results, though training on a larger dataset is needed for broader applicability. |
3P-CPC, three-position compound parabolic concentrator; CSP, concentrated solar power; EW-CPC, east–west compound parabolic concentrator; GCR, ground coverage ratio; HCPV, high-concentration PV; LDR, light-dependent resistor; SASTS, single-axis solar tracking system.
4. Results and discussion
Different solar tracking strategies for the same panel capacity have separate energy production and tracking efficiency. Table 8 shows a comparison of the essential parameters for the different solar tracking strategies. If dual-axis tracking is enabled in a solar panel, it will be more efficient in enhancing the ability of the solar panel to generate energy, since it has an efficiency of 27.35% compared with that of a fixed solar panel dual-axis PV tracking system. In one-axis three-position polar-axis-aligned sun tracking, the one-axis three-position polar-axis-aligned sun-tracking strategy was experimentally analysed and compared with the fixed-axis solar tracking strategy, which yielded a lower efficiency of approximately 19.85%, as it was positioned at only three points. Dual-axis PV tracking system, azimuth- and sensor-based control strategies for a PV solar tracking application, and filtered sun sensor for solar tracking in HCPV and CSP system used dual-axis tracking by means of sensor-based control strategies, which increase hardware complexity; a larger number of sensors and control strategies increase hardware complexity, resulting in high cost and maintenance. Figure 16 shows the radar spider 2D chart, which determines the best solar tracking strategy for framing the STS. The datasets for the radar plot are given in Tables 8 and 9, where Table 8 presents the true values of the parameters to be considered for the STS and Table 9 presents their per unit values. The costa and hardware complexities are given numeric values via calibration techniques, where 0.5 denotes very low cost, 1 denotes low cost, 1.5 denotes high cost, and 2 denotes very high cost, which depends on the complexity, i.e. a high complexity results in a high cost. Similarly, a hardware complexity of 1 denotes less complexity, 1.5 denotes medium complexity, and 2 denotes high complexity.
Comparison of essential parameters from the different methodologies used in the STS.
Tracking strategy . | Energy (MWh) . | Efficiency %age . | Hardware complexity . | No. of sensors required . | Cost . |
---|---|---|---|---|---|
Fixed- and double-axis STS | 11.53 | 26.18 | 1 | 4 | 0.5 |
Single-axis scheduled STS | 15.4 | 23.4 | 1 | 2 | 0.5 |
Single-axis multiple-position V-trough tracker | 16.75 | 24.84 | 1.5 | 2 | 1.5 |
Bifacial fixed-tilt single-axis tracking system | 14.12 | 24.23 | 1.5 | 2 | 1 |
One-axis three-position polar-axis-aligned sun tracking | 10.54 | 19.85 | 1.5 | 4 | 1.5 |
Dual-axis PV tracking system | 19.52 | 27.35 | 2 | 4 | 2 |
Azimuth- and sensor-based control strategies for a PV solar tracking application | 13.14 | 23.12 | 2 | 6 | 2 |
Filtered sun sensor for solar tracking in HCPV and CSP system | 17.12 | 24.32 | 2 | 6 | 2 |
Microcontroller-based single-axis PV tracker system on solar panel performance | 11.15 | 21.45 | 2 | 8 | 2 |
STS for solar panels that makes use of the Proteus ISIS 7.6 software | 12.87 | 22.32 | 1.5 | 6 | 1.5 |
Two-axis STS using flat-mirror reflectors | 11.54 | 20.12 | 2 | 4 | 2 |
Three-axis STS | 11.73 | 23.24 | 2 | 6 | 2 |
Tracking strategy . | Energy (MWh) . | Efficiency %age . | Hardware complexity . | No. of sensors required . | Cost . |
---|---|---|---|---|---|
Fixed- and double-axis STS | 11.53 | 26.18 | 1 | 4 | 0.5 |
Single-axis scheduled STS | 15.4 | 23.4 | 1 | 2 | 0.5 |
Single-axis multiple-position V-trough tracker | 16.75 | 24.84 | 1.5 | 2 | 1.5 |
Bifacial fixed-tilt single-axis tracking system | 14.12 | 24.23 | 1.5 | 2 | 1 |
One-axis three-position polar-axis-aligned sun tracking | 10.54 | 19.85 | 1.5 | 4 | 1.5 |
Dual-axis PV tracking system | 19.52 | 27.35 | 2 | 4 | 2 |
Azimuth- and sensor-based control strategies for a PV solar tracking application | 13.14 | 23.12 | 2 | 6 | 2 |
Filtered sun sensor for solar tracking in HCPV and CSP system | 17.12 | 24.32 | 2 | 6 | 2 |
Microcontroller-based single-axis PV tracker system on solar panel performance | 11.15 | 21.45 | 2 | 8 | 2 |
STS for solar panels that makes use of the Proteus ISIS 7.6 software | 12.87 | 22.32 | 1.5 | 6 | 1.5 |
Two-axis STS using flat-mirror reflectors | 11.54 | 20.12 | 2 | 4 | 2 |
Three-axis STS | 11.73 | 23.24 | 2 | 6 | 2 |
CSP, concentrated solar power; HCPV, high-concentration PV.
Comparison of essential parameters from the different methodologies used in the STS.
Tracking strategy . | Energy (MWh) . | Efficiency %age . | Hardware complexity . | No. of sensors required . | Cost . |
---|---|---|---|---|---|
Fixed- and double-axis STS | 11.53 | 26.18 | 1 | 4 | 0.5 |
Single-axis scheduled STS | 15.4 | 23.4 | 1 | 2 | 0.5 |
Single-axis multiple-position V-trough tracker | 16.75 | 24.84 | 1.5 | 2 | 1.5 |
Bifacial fixed-tilt single-axis tracking system | 14.12 | 24.23 | 1.5 | 2 | 1 |
One-axis three-position polar-axis-aligned sun tracking | 10.54 | 19.85 | 1.5 | 4 | 1.5 |
Dual-axis PV tracking system | 19.52 | 27.35 | 2 | 4 | 2 |
Azimuth- and sensor-based control strategies for a PV solar tracking application | 13.14 | 23.12 | 2 | 6 | 2 |
Filtered sun sensor for solar tracking in HCPV and CSP system | 17.12 | 24.32 | 2 | 6 | 2 |
Microcontroller-based single-axis PV tracker system on solar panel performance | 11.15 | 21.45 | 2 | 8 | 2 |
STS for solar panels that makes use of the Proteus ISIS 7.6 software | 12.87 | 22.32 | 1.5 | 6 | 1.5 |
Two-axis STS using flat-mirror reflectors | 11.54 | 20.12 | 2 | 4 | 2 |
Three-axis STS | 11.73 | 23.24 | 2 | 6 | 2 |
Tracking strategy . | Energy (MWh) . | Efficiency %age . | Hardware complexity . | No. of sensors required . | Cost . |
---|---|---|---|---|---|
Fixed- and double-axis STS | 11.53 | 26.18 | 1 | 4 | 0.5 |
Single-axis scheduled STS | 15.4 | 23.4 | 1 | 2 | 0.5 |
Single-axis multiple-position V-trough tracker | 16.75 | 24.84 | 1.5 | 2 | 1.5 |
Bifacial fixed-tilt single-axis tracking system | 14.12 | 24.23 | 1.5 | 2 | 1 |
One-axis three-position polar-axis-aligned sun tracking | 10.54 | 19.85 | 1.5 | 4 | 1.5 |
Dual-axis PV tracking system | 19.52 | 27.35 | 2 | 4 | 2 |
Azimuth- and sensor-based control strategies for a PV solar tracking application | 13.14 | 23.12 | 2 | 6 | 2 |
Filtered sun sensor for solar tracking in HCPV and CSP system | 17.12 | 24.32 | 2 | 6 | 2 |
Microcontroller-based single-axis PV tracker system on solar panel performance | 11.15 | 21.45 | 2 | 8 | 2 |
STS for solar panels that makes use of the Proteus ISIS 7.6 software | 12.87 | 22.32 | 1.5 | 6 | 1.5 |
Two-axis STS using flat-mirror reflectors | 11.54 | 20.12 | 2 | 4 | 2 |
Three-axis STS | 11.73 | 23.24 | 2 | 6 | 2 |
CSP, concentrated solar power; HCPV, high-concentration PV.
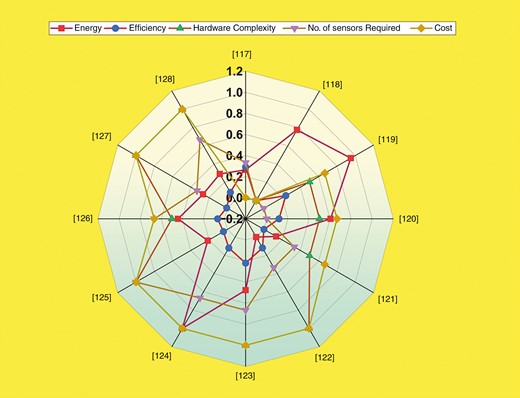
Radar chart for comparative analysis of different STS on the basis of qualitative and quantitative parameters.
5. Conclusion and future scope
Automatic STS have become more efficient because of advancements in sensor technology, control algorithms, and precision mechanics. These systems can optimize the angle and orientation of solar panels to maximize sunlight exposure throughout the day, leading to increased energy production.
Artificial intelligence and machine learning techniques are being integrated into automatic STS to increase their performance. These technologies enable the system to analyse real-time data, predict solar movement patterns, and optimize tracking algorithms accordingly, resulting in improved accuracy and efficiency.
Traditionally, single-axis STS, which track the movement of the sun in only one plane, are prevalent. However, dual-axis STS have gained popularity, as they can track both the azimuth and elevation angles of the sun. This enables the panels to capture sunlight from different angles throughout the day, further maximizing the energy output.
Compared with fixed-tilt systems, implementing automatic STS can be cost intensive. The additional components, sensors, and motors required for tracking increase the overall system cost. However, with advancements in technology and economies of scale, the costs are gradually decreasing, making the systems more accessible.
Automatic STS are made up of moving parts, which may need to be maintained regularly to guarantee that they are operating correctly. Mechanical wear and tear, exposure to weather conditions, and sensor failures can impact system reliability. Ensuring regular inspections, maintenance routines, and robust designs can mitigate these challenges.
Automatic STS rely on accurate sun tracking, which can be affected by environmental factors such as clouds, haze, and shading from nearby structures or vegetation. These factors can impact the system’s ability to track the sun accurately and affect energy generation.
An automatic STS is a promising technology that has undergone significant advancements in recent years. It offers several advantages, including increased energy efficiency and improved power generation from solar panels. This review highlights some of the key advancements and challenges associated with automatic STS in the current scenario. An automatic STS is a promising technology that has undergone significant advancements in recent years. It offers several advantages, including increased energy efficiency and improved power generation from solar panels. This review highlights some of the key advancements and challenges associated with automatic STS in the current scenario.
According to the findings of this review presented in the comparative analysis in Table 7, the performance of the dual-axis PV tracking system was superior to that of the stationary systems by more than 27%. The system efficiency was compared between the two types. Under diverse weather conditions, the schedule-based STS is 4.2% more efficient than the LDR solar trackers. The tracker has 57.4% better efficiency than a fixed solar panel tilted to its ideal angle. This review indicates that the double-axis sun-tracking system generates power at a rate that is 30.79% higher than that of the latitude-tilt fixed PV system. When the two different systems are compared, this distinction becomes clear. The outcome of this review is that V-trough concentrators are more suitable for focusing sunlight on commercially accessible solar cells because they are significantly simpler to produce than CPCs. This is because they have more uniform solar irradiation at their bases and are better fitted to remove surplus heat through the sidewalls of their chambers. CPCs have a greater temperature threshold, which allows them to concentrate sunlight more effectively than V-trough concentrators. If we compare the tracking gains of monofacial and bifacial systems for the same GCR, the tracking gain for monofacial systems is somewhat greater than that of bifacial systems.
Author contributions
Paramjeet Singh Paliyal (Writing—original draft [lead]), Surajit Mondal (Supervision [lead], Writing—review & editing [lead]), Samar Layek (Resources [lead]), Piyush Kuchhal (Conceptualization [equal], Validation [equal]), and Jitendra Kumar Pandey (Methodology [equal], Supervision [equal])
Conflict of interest statement
None declared.
Funding
None declared.
Data availability
No new data were generated or analysed in support of this research.