-
PDF
- Split View
-
Views
-
Cite
Cite
David C Blackburn, Doug M Boyer, Jaimi A Gray, Julie Winchester, John M Bates, Stephanie L Baumgart, Emily Braker, Daryl Coldren, Kevin W Conway, Alison Davis Rabosky, Noé de la Sancha, Casey B Dillman, Jonathan L Dunnum, Catherine M Early, Benjamin W Frable, Matt W Gage, James Hanken, Jessica A Maisano, Ben D Marks, Katherine P Maslenikov, John E McCormack, Ramon S Nagesan, Gregory G Pandelis, Heather L Prestridge, Daniel L Rabosky, Zachary S Randall, Mark B Robbins, Lauren A Scheinberg, Carol L Spencer, Adam P Summers, Leif Tapanila, Cody W Thompson, Luke Tornabene, Greg J Watkins-Colwell, Luke J Welton, the oVert Project Team, Edward L Stanley, Increasing the impact of vertebrate scientific collections through 3D imaging: The openVertebrate (oVert) Thematic Collections Network, BioScience, Volume 74, Issue 3, March 2024, Pages 169–186, https://doi.org/10.1093/biosci/biad120
- Share Icon Share
Abstract
The impact of preserved museum specimens is transforming and increasing by three-dimensional (3D) imaging that creates high-fidelity online digital specimens. Through examples from the openVertebrate (oVert) Thematic Collections Network, we describe how we created a digitization community dedicated to the shared vision of making 3D data of specimens available and the impact of these data on a broad audience of scientists, students, teachers, artists, and more. High-fidelity digital 3D models allow people from multiple communities to simultaneously access and use scientific specimens. Based on our multiyear, multi-institution project, we identify significant technological and social hurdles that remain for fully realizing the potential impact of digital 3D specimens.
The physical specimens and objects in natural history museums require people to give them meaning. Access to natural history collections has increased over the past few hundred years, from their inception as cabinets of curiosity in aristocratic households to public museums exhibiting subsets of their collection to spark wonder and curiosity. Yet, traditionally, these specimens and objects were collected by specialists and for specialists. Their value has and continues to derive primarily from research conducted using them (Suarez and Tsutsui 2004). Sometimes these specimens were viewed by wider audiences in exhibits, used as referenced by artists, or used in education (Cook et al. 2014), but typically only specialists were granted access to these collections. Because relatively few people—who are not representative of humanity as a whole—have had access to these resources, the potential impacts of these collections have been limited.
Digital representations of physical specimens and objects have dramatically expanded the impact of natural history collections, making them more accessible and transforming research. These representations, even when they are only descriptive text (e.g., metadata), have made research possible at large scales, such as modeling species distributions based on georeferenced locality data from thousands of museum records (Soberón and Peterson 2004). For scientific collections of vertebrates, the first digital representations were largely restricted to the text-based metadata (e.g., locality, date) associated with the specimens, not images or other media files depicting the physical specimen. Projects such as FishNet, HerpNet, the Mammal Networked Information System (MaNIS), Ornithological Information System (ORNIS), and later VertNet, all of which were funded by the US National Science Foundation (NSF), focused on creating and distributing digital specimen metadata online, as well as on geocoding localities (Seltmann et al. 2018). The availability of these data means that a modern researcher can quickly locate specimens in scientific collections around the world. Yet to examine these specimens in detail, a researcher must still visit the collection or receive the specimen on loan. Whereas information about the specimens is accessible via a quick web-based search, access to the physical specimens remains much as it was for early naturalists. Of course, a researcher working remotely can request and receive one or several two-dimensional (2D) images of specimens, but these are often insufficient to address many questions about their biology and reveal little of their internal anatomy, diets, or parasites. In addition to the financial and time-based barriers associated with accessing physical collections, museums are often justifiably protective of rare or important specimens, limiting work that requires extensive loans or destructive sampling to only the most compelling proposals. This can lead to important specimens being among the most difficult to access.
With the advent of new imaging technologies using visible light or X-rays to create digital, high-fidelity, three-dimensional (3D) representations of physical specimens, objects in natural history collections can now be more accessible than ever before to a broad audience. A single object might be examined simultaneously by multiple scientists, working in different countries, in a single day rather than waiting for months (or years) for that specimen to be returned on loan for others to use next. During that same time, educators, students, artists, and the public can work with that same digital representation of the specimen. Rather than replace the need for the physical objects, we anticipate that these digital objects—much like the first digitization of descriptive textual data—will increase the awareness and quality of physical specimens, driving interest in using the physical objects in research. Further, some imaging modalities, such as those based on X-ray or magnetic fields, deepen the exploration of physical objects by providing new data on their internal structures. It is increasingly easy to associate different forms of data—localities, DNA sequences, images, audio recordings, and more—for a single object or preserved scientific specimen. These digital 3D representations play a growing role in understanding the genetic and ecological bases of phenotypes and contribute to fleshing out the Extended Specimen (Webster 2017, Lendemer et al. 2020, Hilton et al. 2021).
Beginning in 2017, the openVertebrate (oVert) Thematic Collections Network, funded by the NSF, set out to create a large collection of digital, high-fidelity 3D representations of vertebrate specimens (figure 1) and to make these data available online for research and education (Cross 2017). oVert was not a research project; it was a digitization community. Inherent in the development and success of oVert has been our shared vision among participants: The museum community can and should provide digital anatomical data of vertebrate diversity to a global community of researchers, educators, students, and the public. Making these data broadly accessible online is of value to both users of the data and to the museums housing the specimens. While many previous independent research projects have generated similar data, these data sets were typically dissociated from the specimen records and not generated with serious involvement or oversight from the staff managing these collections. In many cases, this led to these data becoming dissociated from important metadata related to the collection of those specimens. Further, these data have generally not been made available online in a way that maximizes their reuse (Rowe and Frank 2011, Hipsley and Sherratt 2019). Although we knew the possible negative consequences of sharing data, including commercial uses not approved by institutions, oVert embraced the possibilities for dramatic positive impacts on science, education, art, and more. The oVert approach is clearly appealing to many in the research and museum communities since it is regularly offered as an example of the next wave of museum digitization projects (Nelson and Ellis 2018, Harmon et al. 2019, Hipsley and Sherratt 2019, Beans 2020, Hedrick et al. 2020, Timm et al. 2021, Ford et al. 2023).
![Diversity of extractable data and analyses resulting from traditional computed tomography (CT), diffusible iodine-based contrast-enhanced CT (diceCT), and photogrammetry (https://doi.org/10.17602/M2/M535781) of a formalin-fixed, ethanol-stored lizard specimen. Traditional CT scanning (https://doi.org/10.17602/M2/M359089) generates tomograms (left center) that can be used to reconstruct and isolate (a) the bony skeleton with osteoderms (teal), (b) isolated cranium segmented into individual and labeled bones, including (c) the braincase and (d) endocranial space representing the inner ear (with associated stapes), as well as (e) conducting Finite Element Analyses such as evaluating the distribution of stresses in the lower jaw when forces are applied (the red arrows) or (f) wall thickness analyses such as of cranial osteoderms or (g) to 3D print models. DiceCT scans (https://doi.org/10.17602/M2/M456707) generate tomograms that can be used to evaluate anatomy of soft tissue anatomy such as (h) skeletal muscles, (i) nervous system, (j) cardiovascular system, and (k) gastrointestinal tract, as well as revealing valuable natural history such as (l) gut contents (coleopteran elytra and carapace), (m) glands (such as the femoral glands [blue] and associated pores, in red), and (n) reproductive tract with eggs. Finally, the overlap between CT and diceCT data sets can be directly compared (o). All data sets are based on UF: Herp:191433 of the cordylid lizard Ouroborus cataphractus, except panel (l), which is from CAS: Herp:199384 of the closely related Karusasaurus polyzonus.](https://oup.silverchair-cdn.com/oup/backfile/Content_public/Journal/bioscience/74/3/10.1093_biosci_biad120/1/m_biad120fig1.jpeg?Expires=1750181186&Signature=owaMrUdghbeTF4BvQ7gMZGsfsc~k8nDZRLlzUooImsJxiCZcbwl9rCwePscUiYsF1tsGQMVcjDSGlZfjXEF1MANTN9lLcfVmgnnPPx1TCrORqdK3heqzlH6onJZ97mGpXWEb3BPyqH87L-98q9QAqaluRqdUYuqvlOzmyfJ3VOGJzb0EjPQbxzfobYQYu4L~fbm-mw7j-0tZlbzTFz9uotx4xGTvlFNSWoYP6~HVSxmlL3VfMi90k5-t1EP8nqhXtJ7ii5T8FQ-fxNpDJiJOCA9hA7NBAD4bcYY6yEncwzPp4FOAmu5uYbuf27-gxGNTkxJBxkJYICU3NplScO1AiQ__&Key-Pair-Id=APKAIE5G5CRDK6RD3PGA)
Diversity of extractable data and analyses resulting from traditional computed tomography (CT), diffusible iodine-based contrast-enhanced CT (diceCT), and photogrammetry (https://doi.org/10.17602/M2/M535781) of a formalin-fixed, ethanol-stored lizard specimen. Traditional CT scanning (https://doi.org/10.17602/M2/M359089) generates tomograms (left center) that can be used to reconstruct and isolate (a) the bony skeleton with osteoderms (teal), (b) isolated cranium segmented into individual and labeled bones, including (c) the braincase and (d) endocranial space representing the inner ear (with associated stapes), as well as (e) conducting Finite Element Analyses such as evaluating the distribution of stresses in the lower jaw when forces are applied (the red arrows) or (f) wall thickness analyses such as of cranial osteoderms or (g) to 3D print models. DiceCT scans (https://doi.org/10.17602/M2/M456707) generate tomograms that can be used to evaluate anatomy of soft tissue anatomy such as (h) skeletal muscles, (i) nervous system, (j) cardiovascular system, and (k) gastrointestinal tract, as well as revealing valuable natural history such as (l) gut contents (coleopteran elytra and carapace), (m) glands (such as the femoral glands [blue] and associated pores, in red), and (n) reproductive tract with eggs. Finally, the overlap between CT and diceCT data sets can be directly compared (o). All data sets are based on UF: Herp:191433 of the cordylid lizard Ouroborus cataphractus, except panel (l), which is from CAS: Herp:199384 of the closely related Karusasaurus polyzonus.
The focus of oVert was to seed comparative studies of vertebrate diversity with high-resolution X-ray computer tomographic (CT) scans (figure 1) revealing the articulated skeleton, a common currency in studies of functional and comparative morphology, paleontology, systematics, and developmental biology. Rather than aiming to provide the definitive and final set of data for these taxa, our goal was to inspire research at phylogenetic scales that were not previously possible and for parts of the vertebrate Tree of Life that are not already the focus of intensive research (e.g., mice, cichlid fishes, anole lizards). In addition, we aimed to generate data sets of soft-tissue anatomy for a representative of every extant vertebrate family using contrast-enhanced CT scanning (e.g., by staining with contrast agents such as Lugol's iodine or phosphotungstic acid). oVert subsequently expanded to include partner projects that use light-based scanning and photogrammetry.
As part of oVert, we faced challenges in deciding how to select taxa and specimens for imaging, developing best practices for generating comparable CT scan data across institutions, choosing how to best archive and share these data, growing the community that curates and uses 3D data for research and education, and evaluating the impact of these data on a broad audience. In this paper, we outline our approaches to form our digitization community that worked to a common goal of providing high resolution anatomical data sets of vertebrate diversity to be used by a diverse and global audience (our workflow is summarized in figure 2). We also offer our perspective on some of the next steps and challenges for our community. We hope that oVert will inspire other projects across the Tree of Life that will also benefit museums, scientists, educators and students, artists, and the public.
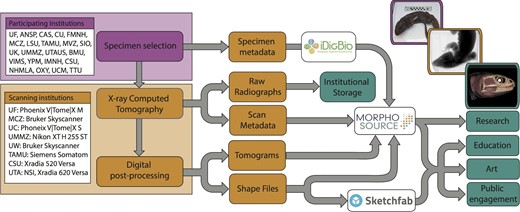
Simplified workflow diagram of the initial oVert project. Following specimen selection at participating institutions, specimens were CT scanned at participating scanning sites, and the resulting data were deposited in institutional repositories and MorphoSource, such that they became widely available for different communities interested in research, education, art, and more. An example of specimens, data, and resulting models is provided as an inset for Sphenodon punctatus (FMNH: Amphibians and Reptiles:11115).
Forming a digitization community
Funded by NSF, oVert focused specifically on specimens in US natural history collections. This effort was supported through the discontinued Advancing Digitization of Biodiversity Collections program, the mission of which is now folded into the Infrastructure Capacity for Biological Research program in the Division of Biological Infrastructure of NSF's Directorate for Biological Sciences. At the outset of the project, we estimated that we could obtain fluid-preserved representatives of more than 80% of extant vertebrate genera based on US collections (more information on specimen selection is provided below).
The initial oVert project involved 16 separate NSF awards supporting work at 18 participating institutions, 16 of which supplied specimens for CT scanning at our scanning facilities. Six institutions acted as centers for CT scanning, including Harvard University (Museum of Comparative Zoology), Texas A&M University (TAMU Institute for Preclinical Studies), University of Chicago (PaleoCT Lab of Dr. Zhe-Xi Luo), University of Florida (Nanoscale Research Facility), University of Michigan (Museum of Zoology), and University of Washington (Karel Liem Bioimaging Facility at Friday Harbor Laboratories). In some cases, large specimens were scanned at locally available facilities, including the Translational Research Imaging Center (Y-TRIC) of Yale University, the University of Chicago Hospital, the Shared Materials Instrumentation Facility of Duke University and veterinary medicine facilities at both Cornell University and Michigan State University. As the network expanded, CT imaging occurred at other institutions, such as the University of Colorado, Boulder, and Sandia National Laboratories. In addition, oVert supported development and growth of the data repository MorphoSource at Duke University (Boyer et al. 2016), also supported by NSF. Specimens from other US natural history collections have also been scanned, including notable partnerships with the American Museum of Natural History, Carnegie Museum of Natural History, and the Smithsonian Institution's National Museum of Natural History. Subsequent to the initial funding of oVert, eight Partner to Existing Network (PEN) grants were funded by NSF (table 1). These PEN awards added seven more funded partner institutions with projects using various imaging modalities, including light-based surface scanning, photogrammetry, and CT scanning. They brought more in-depth focus by imaging specimens ranging from large mammals to small rodents, birds to cryptobenthic reef fishes, and Galápagos tortoises to Mesoamerican salamanders. The oUTCT PEN shepherded data generated at the University of Texas High-Resolution X-ray Computed Tomography Facility (UTCT)—many of which were previously available in some form on the popular DigiMorph website (www.digimorph.org)—to MorphoSource, where these data are now more accessible.
Partner to Existing Network (PEN) projects funded by US National Science Foundation as part of the openVertebrate Thematic Collections Network.
PEN Name . | Lead institution . | Imaging modalities . | Focus . |
---|---|---|---|
Bat PEN! | Texas Tech University | CT scanning | Phyllostomid bats |
CryptoVert | Natural History Museum, Los Angeles | CT scanning | Cryptobenthic fishes |
FuncQEE | Chicago State University | CT scanning | Rodents |
GalapaGateway | California Academy of Sciences | CT scanning, light-based surface scanning | Galápagos vertebrates |
oBird | Occidental College | Photogrammetry | Bird skins |
oMEGA | Idaho State University | Light-based surface scanning | Vertebrates larger than 250 kilograms |
oMeso | University of Colorado, Boulder | CT scanning, 2D photography | Mesoamerican amphibians and reptiles |
oUTCT | University of Texas, Austin | CT scanning | UTCT archive of vertebrates |
PEN Name . | Lead institution . | Imaging modalities . | Focus . |
---|---|---|---|
Bat PEN! | Texas Tech University | CT scanning | Phyllostomid bats |
CryptoVert | Natural History Museum, Los Angeles | CT scanning | Cryptobenthic fishes |
FuncQEE | Chicago State University | CT scanning | Rodents |
GalapaGateway | California Academy of Sciences | CT scanning, light-based surface scanning | Galápagos vertebrates |
oBird | Occidental College | Photogrammetry | Bird skins |
oMEGA | Idaho State University | Light-based surface scanning | Vertebrates larger than 250 kilograms |
oMeso | University of Colorado, Boulder | CT scanning, 2D photography | Mesoamerican amphibians and reptiles |
oUTCT | University of Texas, Austin | CT scanning | UTCT archive of vertebrates |
Partner to Existing Network (PEN) projects funded by US National Science Foundation as part of the openVertebrate Thematic Collections Network.
PEN Name . | Lead institution . | Imaging modalities . | Focus . |
---|---|---|---|
Bat PEN! | Texas Tech University | CT scanning | Phyllostomid bats |
CryptoVert | Natural History Museum, Los Angeles | CT scanning | Cryptobenthic fishes |
FuncQEE | Chicago State University | CT scanning | Rodents |
GalapaGateway | California Academy of Sciences | CT scanning, light-based surface scanning | Galápagos vertebrates |
oBird | Occidental College | Photogrammetry | Bird skins |
oMEGA | Idaho State University | Light-based surface scanning | Vertebrates larger than 250 kilograms |
oMeso | University of Colorado, Boulder | CT scanning, 2D photography | Mesoamerican amphibians and reptiles |
oUTCT | University of Texas, Austin | CT scanning | UTCT archive of vertebrates |
PEN Name . | Lead institution . | Imaging modalities . | Focus . |
---|---|---|---|
Bat PEN! | Texas Tech University | CT scanning | Phyllostomid bats |
CryptoVert | Natural History Museum, Los Angeles | CT scanning | Cryptobenthic fishes |
FuncQEE | Chicago State University | CT scanning | Rodents |
GalapaGateway | California Academy of Sciences | CT scanning, light-based surface scanning | Galápagos vertebrates |
oBird | Occidental College | Photogrammetry | Bird skins |
oMEGA | Idaho State University | Light-based surface scanning | Vertebrates larger than 250 kilograms |
oMeso | University of Colorado, Boulder | CT scanning, 2D photography | Mesoamerican amphibians and reptiles |
oUTCT | University of Texas, Austin | CT scanning | UTCT archive of vertebrates |
The bounds of the oVert community have grown beyond the initial funded institutions. Obviously, some institutions have collections important to the goals of oVert but were not funded through the project. These institutions have become valuable, albeit unfunded, partners that often provide unique collections. The Smithsonian Institution's National Museum of Natural History (NMNH) was not eligible to receive an award from the program that supports oVert but also contains the single largest collection of vertebrates in the world. The fluid-preserved collections of birds at NMNH and the Carnegie Museum of Natural History are both large and relatively unique collections in the United States. We have also worked with many smaller collections (e.g., North Carolina State Museum, Brigham Young University's Monte L. Bean Life Science Museum) to digitize select specimens that fill gaps in our sampling of vertebrate diversity but also bring attention to the unique resources of those institutions.
The oVert project team is composed of approximately 290 people that have been involved in imaging, analysis, curation, education, and more. Together, these individuals represent 48 academic institutions, 29 K–12 schools, and five stand-alone natural history museums in 26 US states, Puerto Rico, and the District of Columbia (see supplemental table S1). These include curators, faculty, collection managers, imaging technicians, informatics specialists, postdoctoral scientists, undergraduate and graduate students, and primary and secondary school (i.e., K–12) teachers. While they were not directly part of the oVert team, the project also benefited from working closely with IT staff and legal experts, as we established workflows for data management and storage, institutional policies for access and use of the data, and more. We have tried to address challenges in communication across our community using online project management websites, as well as disseminating information via social media platforms (e.g., #oVertTCN on Twitter). A team at the University of Florida led coordination across the project, including setting of priorities by developing lists of target taxa (see below), but then different imaging centers worked autonomously as they coordinated with other participating institutions that sent loans of specimens for CT scanning.
Imaging modalities
CT scanning
The majority of oVert's imaging effort uses CT scanning, because it is a nondestructive X-ray-based imaging technique that facilitates high-resolution 3D reconstructions of nonliving objects and is ideal for studying rare and fragile specimens. The output data from CT represents a 3D high-fidelity facsimile of a scanned object and can be digitally dissected, visualized, and analyzed in many ways (figure 1). With standard CT, we can accurately reconstruct internal and external structures of dense tissues (e.g., bone, teeth), whereas newly developed techniques that use radio-opaque stains allow for visualizing soft tissues, including muscles, vessels, and nerves (Sedlmayr and Witmer 2002, Holliday et al. 2006, Pauwels et al. 2013), including diffusible iodine-based contrast-enhanced CT (diceCT; Gignac and Kley 2014, Gignac et al. 2016). A series of 2D X-ray images is captured in a 360-degree path around the object and then used to create a volumetric density map composed of cuboid voxels (volumetric elements), representing the complete density distribution of the object (in some cases magnified to a submicron-level resolution). This density map can be sliced into 2D sections (tomograms) or rendered in 3D; additional processing allows specific regions of interest to be isolated and visualized separately. Though CT scanners are becoming more common at institutions with natural history collections, at the start of our project, we decided to focus on a core set of institutions that could commit to the amount of time needed to serve as scanning facilities for the oVert network.
Surface scanning
In some cases, the size of a specimen (e.g., whale, giraffe, elephant) prevents its preservation as an intact fluid-preserved specimen, or is simply too large to fit inside conventional CT scanners. To create digital representations of large vertebrates that are often rare in collections, the oMEGA (online Metrology of Extant Giant Animals) project (funded as a Partner to Existing Network to oVert) used surface scanning (laser and structured light) to produce 3D models of skeletons (Pruitt et al. 2023). These 3D surface models lack internal details of the bones, but provide other data, such as mapping color and texture to create photorealistic models. The final models of individual bones require no additional processing for end users and can be digitally assembled to produce articulated skeletons.
Photogrammetry
Photogrammetry is another imaging method added to oVert through partner projects, especially the oBird project (funded as a PEN to oVert and based at Occidental College), that generated colorized 3D models of bird skins. This method captures the outside of a physical specimen hundreds of times from different directions through digital photography, with the resulting photos stitched together computationally from common landmarks to generate a highly detailed 3D model (figure 1). The benefits of photogrammetry include the relatively low startup cost and small footprint, as well as the ability to capture some of the most sought-after phenotypic traits on the outside of specimens, like plumage and pelage color, while retaining scalability to allow for morphometric landmarking. Photogrammetry is an affordable option for creating 3D models of important fossils and cultural heritage artifacts on short term loan to institutions. Molding casts from the meshes can be printed and even painted to replicate repatriated native collections allowing for access and cultural preservation (Deutsch and Hollinger 2017). Photogrammetry has only recently been applied to natural history specimens (Nguyen et al. 2014, Medina et al. 2020) and live organisms (Bot and Irschick 2019), so methods for quantifying features of the resulting models are still in development. However, there are promising applications for quantifying whole-organism, full-spectrum color by analyzing pixel RGB values contained in texture files. For example, this can be performed using the colordistance R package (Weller and Westneat 2019) as with recent work using photogrammetry models of flowers (Leménager et al. 2023). Accessibility is another strength of photogrammetry because only a digital camera, a computer, and software for creating 3D models are needed.
Selecting taxa and specimens
Choosing taxa
An initial challenge for any collaborative project digitizing a large swath of biodiversity spread across collections at different institutions is how to choose the taxa and specimens that will be imaged. Once a list of species was available, it was possible using biodiversity data aggregators to determine which institutions had relevant specimens and to allow participating institutions to begin evaluating the appropriateness of their specimens. As a starting place, oVert made a strategic decision to select specimens representing the type species of each currently recognized genus of living vertebrates. We chose to not prioritize imaging of name-bearing type specimens (e.g., holotypes, syntypes, lectotypes) because in many cases these are not located at US institutions (a necessary focus of our funding). In addition, many older type specimens are in poor condition, sometimes missing limbs or other parts, may not be adult, and may not represent the phenotypes exemplary for and unique to that taxon. Consider, for example, that the type specimen of the anuran genus Ascaphus is an adult female, the most remarkable diagnostic feature of which, to Stejneger (1899), was that it lacked a spade on its hindfoot (hence the genus name from Greek: a-, “without”; skaphos, “boat,” referring to the boat-shaped spade). Stejneger (1899) did not have male specimens available to know that this is the only anuran genus in which males have an intromittent organ, a remarkable and unique phenotype among anurans and clearly one worth prioritizing for imaging. Because not all type species are available in US collections and sometimes the only available specimens were not suitable, we often chose another representative species for the genus. Still, a focus on type species provided a useful starting point and did not require experts with knowledge of every extant genus for choosing which species we should image first. To get this information, we relied on widely used taxonomic resources, including Catalog of Fishes (Fricke et al. 2020), Amphibian Species of the World (Frost 2020), Reptile Database (Uetz et al. 2020), IOC World Birds List (Gill et al. 2020), and Mammal Species of the World (Wilson and Reeder 2005). When possible, we attempted to keep up to date with genus-level revisions, adding specimens to our imaging queues when new genera are recognized. These lists of target species were distributed to the oVert team through online collaborative documents that enabled each institution to identify appropriate specimens (see below) and then loan them to scanning facilities in the oVert network.
Choosing specimens
We also faced a decision as to which single specimen to use to represent each taxon. In addition to the obvious decision to choose an intact fluid-preserved specimen—efficiently containing an entire articulated specimen in one fleshy body—we decided to focus on selecting an adult wild-caught specimen (without specifying the sex), preferably with its mouth closed for a more natural positioning of bones in species with cranial kinesis. Further, building on previous digitization projects for vertebrates (Cook et al. 2014, Nelson and Ellis 2018), such as FishNet (NSF grant no. DBI-415,600,1,202,953), HerpNet (NSF grant no. DBI-0,132,303), MaNIS (NSF grant no. DBI-0,108,161), ORNIS (NSF grant no. DBI-0,345,448), and VertNet (Constable et al. 2010; NSF grant no. DBI-1,062,193), we focused on specimens with associated collections metadata (e.g., locality, date of collection). When possible, we prioritized specimens with other associated resources such as images (especially from the initial collecting event) or tissue samples from which genetic data has been or could be obtained; for fishes, we often scanned an intact specimen from the same lot from which another individual was tissued. We reasoned that focusing on data- and resource-rich specimens creates higher-value digital specimens for further research and aligns with the vision of “extended specimens” with associated geographic, genetic, and phenotypic data (Webster 2017, Lendemer et al. 2020). The project benefited from taxonomic expertise of collection and research staff at participating institutions that helped to identify specimens selected for imaging.
When possible, we selected specimens that were preserved in positions that would maximize the output resolution or otherwise simplify the scanning process. As the CT scanners that generated data during oVert used square, flat panel detectors (with the exception of the medical/veterinary scanners used for oversized specimens; see next section), the optimal specimen shape is a column that is equal in height and diameter. For elongate taxa (e.g., snakes, anguilliform fish), we preferentially selected specimens that had been preserved in a more compact shapes (e.g., coiled) because we could scan these at a much higher resolution than if they had been preserved in a linear position. As these elongate taxa often possess a head that is small in comparison to their total body length, the full-body scans were often followed by a second, higher-resolution scan of the skull region.
We sometimes addressed other challenges with identifying and selecting specimens. Using online data sets for collections to identify fluid-preserved specimens is predicated on these having accurate information on preparation types. For collections in which fluid-preserved specimens are uncommon—especially for collections of birds—details about fluid-preserved preparations were often not stated in consistent ways, leading to additional time spent by curatorial staff in identifying whether they had appropriate specimens. Some institutions, including the Smithsonian Institution's National Museum of Natural History, do not make all of their data available online due to concerns related to the conservation of threatened species, which required specific data requests to the staff of those institutions. Large-bodied fluid-preserved specimens often had the additional complication of containing X-ray dense material that leads to substantial noise in the CT data sets. Before the advent of mist nets, both small and large birds were often collected by shooting them with lead shot, and larger fishes and turtles often contain hooks and other dense materials that led to these data sets derived from these specimens to sometimes be unusable. Because the oVert scanning facilities use industrial CT scanners using a cone-beam X-ray and a square detector, the optimal scanning area was a column. This led to some challenges because fluid-preserved specimens were often not preserved in positions that were optimal for CT scanning, especially for many bird and mammal specimens.
Large specimens
There were other challenges specific to large-bodied vertebrates (>1.5 meter in length). Because of the difficulties of fixing and transporting large specimens, different standards for specimen preparation across subdisciplines of vertebrate zoology (e.g., ichthyology vs. mammalogy), and because of the space constraints that many museums face for storage of specimens, intact and fluid-preserved specimens of large-bodied vertebrates are exceptionally rare in museum collections. When such specimens are available for CT scanning, it can be challenging to transport these heavy specimens to a scanning location, and once there, just as challenging to fit them into an imaging machine. Most oVert partner scanning institutions use CT scanners that can only accommodate specimens up to 50 centimeters (cm) in length and 40 cm in width and depth. One oVert institution (Texas A&M University) houses a wide-bore veterinary CT scanner (Siemens Biograph mCT) with a 200-cm reinforced bed, capable of scanning larger specimens up to 78 cm in width and depth and up to 250 kilograms in weight, and contributed data for a number of larger-bodied vertebrates (>150 cm in length) to the project (>300 media files for >200 specimens; www.morphosource.org/projects/000432051). Even within the United States, large fluid-preserved specimens remain logistically difficult (and expensive) to ship between institutions. Some collections partnered with local medical CT-scanning facilities to get their large specimens scanned (e.g., Yale Peabody Museum Gorilla scanned at Y-TRIC; https://doi.org/10.17602/M2/M56407), but these facilities have limited time to devote to scanning non-patients. Beyond the logistical challenges of scanning large specimens, the low-resolution data sets resulting from medical scanners (typically at a maximum of 0.6 millimeter resolution) represent a scientific hurdle, limiting their utility to coarser investigations. The long time (and moderate chemical expense) needed to prepare larger specimens (i.e., over 500 kilograms) for diceCT (if museum collections are willing to allow this; see below) is another challenge, resulting in only a handful of these data sets being made available as part of oVert (∼20).
One way that we created data sets for species of large-bodied vertebrates, at least for mammals and some groups of fishes, was scanning earlier (and thus smaller) developmental stages that can be accommodated by microCT scanners. oVert used this approach for some large bodied fishes, such as an embryo of a whale shark (MorphoSource record, https://doi.org/10.17602/M2/M59110), and mammals, for which fetal or juvenile specimens are available in US collections (e.g., a fetal southern elephant seal, https://doi.org/10.17602/M2/M167666). Though substituting earlier ontogenetic stages for adults allowed us to increase the diversity of large-bodied vertebrates available for inclusion in oVert, there are clear limits to this approach (e.g., adult anatomy is not available for investigation) and earlier ontogenetic material suitable for scanning is also often rare in collections. This “ontogenetic work-around” is still not sufficient to enable oVert scanning of exceptionally large terrestrial or marine mammals. The oMEGA project (Pruitt et al. 2023) partially filled this gap by surface scanning complete skeletal specimens of vertebrate megafauna (www.morphosource.org/projects/00000C960). These scans are useful to the research community, but unlike CT scanning, surface scanning cannot capture the internal structure of bones.
Other sampling strategies
We expanded the primary goal of generating sampling across the vertebrate tree of life with focused efforts on subsets of vertebrate diversity. With more in-depth sampling of particular clades, we aimed to generate data of interest to specific research communities. This included efforts to scan fluid-preserved specimens of species of deer mice (genus Peromyscus) and each species of turtle. We also prioritized scanning of vertebrate species that are considered Extinct or Extinct in the Wild based on the IUCN Red List (IUCN 2020). Because it became clear during the COVID-19 pandemic of 2020 that many educators would benefit from detailed digital data of species used in comparative anatomy courses, we prioritized both normal and contrast-enhanced scans of taxa common to these courses such as lancelets, lampreys, perch, and bullfrogs.
Generating data sets for soft tissue anatomy
Contrast-enhanced CT scans
To extend the diversity of research questions resulting from data generated by oVert, a primary goal was to generate a contrast-enhanced CT data set for as many extant vertebrate families as possible. While generating fewer data sets than by our typical CT-scanning efforts, these family-level data sets enable visualization of soft tissues. Because our sampling was based on specimens for which the tissues had already been fixed—though exactly how is not information typically recorded for vertebrate specimens—it was not feasible to use vascular injection. Further, because we sampled potentially hundreds of specimens and wanted to visualize the soft tissues, we needed a cost-effective method that could be used on specimens varying in both biology and size. We opted to use the diffusible iodine-based contrast-enhanced CT approach (diceCT; Gignac and Kley 2014, Gignac et al. 2016, Callahan et al. 2021) in which already preserved specimens are soaked in (or sometimes injected with) a solution of iodine for a period of days to months. Through this process, the density of the integument, muscles, nervous system, and internal organs is increased, thus enhancing their ability to diffract X-rays during CT scanning.
One advantage of diceCT is that it is both relatively simple and mostly nondestructive for previously fixed specimens stored in alcohol, as are most fluid-preserved vertebrates in museum collections. The ionizing X-ray radiation used in CT can damage DNA (Muller 1927), but CT and iodine staining appear to have a minimal impact on DNA amplification from preserved tissue (Hall et al. 2015). Though there are also concerns about shrinkage (Dawood et al. 2021), decalcification (Early et al. 2020), and changes to coloration (Lanzetti and Ekdale 2021) that can occur during the process of staining a specimen, modified protocols limit these effects (e.g., Dawood et al. 2021) and are not obviously more destructive than traditional dissection. In preparing a specimen for diceCT, it is submerged in an aqueous iodine solution, also known as Lugol's solution, that passively diffuses through the tissues. The length of time required to reach optimal saturation varies depending on the size of the specimen as well as its surface area to volume ratio, density of external tissues (e.g., carapace of turtles; Gray et al. 2024), amount of muscle mass, reproductive stage, pathological issues, and the size of the staining vessel or concentration of staining solution. Although the visual effects of diceCT on a specimen are reversible, the process does alter the specimen at the chemical level. Exactly how and to what extent this occurs is not fully understood, so it is recommended that any specimen chosen to undergo this process has already had tissues sampled for genetic analyses and has been both photographed and CT scanned to visualize the skeleton alone. Because the extent of alteration of the tissues of the specimen are not clear, it is also preferable to not put rare, valuable, or unique specimens through this process.
Assessing quality of diceCT scans
One major drawback to diceCT is the quality of soft tissues in museum specimens. Because we are using museum specimens for oVert, we were unable to adhere to the “fresh is best” approach that is usually recommended for diceCT (Gignac and Kley 2014, Gignac et al. 2016). Sometimes the soft tissue is damaged to the point of being unusable, and this is due to fixation and preservation processes (e.g., Hedrick et al. 2018). Often, we only learn that the tissues are in poor state of preservation or were damaged during collection after staining and scanning what seemed to be a specimen in suitable condition. To try and avoid this issue, we found it is best to choose specimens intentionally, and aim for those where the preservation history is known (or at least reasonably inferred). Preservation with and long-term storage in 70% ethanol can lead to significant dehydration of soft tissues, whereas repeated cycles of freezing and thawing are particularly damaging to neural tissues and specimens. Specimens that were subjected to these are not ideal for diceCT. It is also preferable to choose more recently collected specimens, although good results can still be achieved with older specimens if they were fixed and preserved well.
After scanning, it is advisable to perform quality checks to ensure the resulting diceCT scan is of acceptable quality for use in segmentation and research. Because dehydration and tissue shrinkage during preservation may happen in fluid-preserved specimens, key indicators of the quality of a diceCT scan include the shape and margins of the brain and spinal cord, excess space between muscles, integument, and internal organs, and the directionality of muscle fibers. While there is not yet a measurable standard or threshold that can be recorded for a diceCT scan to indicate how successful post-reconstruction processing stages will be, examining muscle and neural tissues can give a quick indication of general quality.
Forms of data
One of the challenges in using 3D images for science is the complex processing associated with most modalities. Unlike standard photography, audio recordings, or video, the raw data directly captured by sensors is not typically interpretable by a human as a 3D object. Thus, data collection in the form of qualitative trait scoring or quantitative measures of geometry is not possible until the data are processed. Because this requires technicians to make decisions about parameter settings, this leaves open the question about whether processing errors or choices may bias the “final” representation. Related to this, advancing technology in algorithms for data processing means more fidelity might be obtained from the same raw data in the future. This is especially applicable to tomography and photogrammetry. Although these circumstances incentivize storing raw data, this is currently impractical given constraints on data storage costs. However, several oVert institutions and partner projects use in-house data repositories (e.g., Deep Blue Data, at the University of Michigan, https://deepblue.lib.umich.edu/data; the Texas Advanced Computing Center's repository) for storing and making publicly available raw data.
CT data
Most modern microCT scanners capture a series of 2D radiographs on a square panel detector as the specimen is rotated around a fixed axis in line with a cone shaped X-ray beam. These projections are reconstructed as a series of tomographic slices through a specimen, which are typically orthogonal to the axis of rotation. The computed slices can be rendered as an image stack with three dimensions and visualized as a grayscale volume. Though radiographs from CT scans are considered raw data, they have limited utility for most research or education objectives given the limited options for visualizing them. Thus, observations based on projections are not frequently cited directly in research and they are not in demand by researchers or educators. In contrast, cross-sectional CT image series are valuable for research and the most frequently examined and studied result of a specific CT-scanning event. From these image series, anatomical elements may be segmented and saved as mesh files. These resulting surface files are a representation of the surface geometry of a structure. There may be questions about the subjectivity involved in the process by which they were derived from the CT data. However, these surface (or mesh) files are the most familiar format for 3D data and have a large user community for use in 3D printing and shape analyses. Saving the projection series and the CT image series can result in a 20 gigabytes (GB) data package for typical industrial microCT scanners. Omitting the projections and cropping the CT image series to the object of interest can cut the storage footprint while losing little to no data relevant to the majority of use cases. To reduce the storage demands, oVert uses a cropped version of the image series on MorphoSource.
Photogrammetry data
Similar issues with data processing and storage exist with photogrammetry workflows. The raw photograph collection required for a specimen model can easily be 5–20 GB in size. However, when it comes to considering the quality of the final model and its texture, a virtually identical result can be obtained by processing the same photos after first cropping them to the object of interest and then converting them to a lossless compression format such as Jpeg2000. The oBird project saves the photo collections after cropping and conversion and connects these to the final model, along with a set of masked photos (e.g., a greater white-footed goose, https://doi.org/10.17602/M2/M415910). This procedure can reduce data storage needs by an order of magnitude with virtually no loss of potential for later reuse.
Structured light-scanning data
Data from structured light scans are less complicated but also more problematic when it comes to considering raw and derived data. This is because the raw data of structured light scanners are typically inextricably bound to proprietary software designed to walk a user through the processing workflow that generates the final model. In other words, while it might be possible to archive the raw output, it is virtually impossible to meaningfully preserve it due to the requirements of proprietary software. Thus, oVert chose to preserve only the final derivative model from structured light scanning events. Although laser scanning is better in the sense that point clouds can be saved and this format is relatively simple, the additional storage and complexity involved in processing raw point clouds makes it logistically impractical to regularly archive point clouds. For the oMEGA project, what is archived on MorphoSource is the highest resolution version of the final mesh that resulted from processing the point clouds from scanning.
Data archiving
One primary challenge is deciding which resulting data should be archived and in what formats. Some participating institutions opted to archive the X-ray projections generated by CT scanning (the “raw” data) using available institutional data storage resources (e.g., Deep Blue Data at the University of Michigan, https://deepblue.lib.umich.edu/data). In all cases, image stacks and surface files were stored and served via MorphoSource (see the next section below, in Data accessibility). We prioritized a lossless image format such that most series of CT images are available as ZIP files containing 16-bit ushort TIFF images representing the scanning event (figure 2). Most resulting surface files were PLY, STL, or OBJ formats. Image formats used to create a photogrammetry model consist of high-resolution JPEG or converted from RAW format (e.g., CR3, NEF). A resulting photogrammetry model consists of an OBJ surface file, a TIFF texture file, and a MTL sidecar file (used to associate information in the OBJ and TIFF files). Some participating institutions have opted to synchronize a local copy of the image series and surface files with what is online in MorphoSource (e.g., Field Museum of Natural History, Yale Peabody Museum of Natural History).
Data accessibility
To ensure that data generated by oVert and its partner projects are widely accessible, oVert formed a strong partnership with the online data repository MorphoSource (based at Duke University, in the United States; figure 2). Data aggregators such as iDigBio and GBIF (Global Biodiversity Information Facility) are not currently able to preserve (with appropriate metadata and paradata), display, or make accessible 2D image stacks or 3D meshes or volumes generated from CT scanning and photogrammetry. These aggregators are also not equipped to support nuanced management of image data that have complex intellectual property considerations and various reuse restrictions, which is often the case for high-fidelity 3D data resources. The MorphoSource web archive was designed specifically to excel at archiving non-proprietary 3D image formats of specimens in scientific collections and to support complex management needs. MorphoSource designed routines for in-browser web viewing of 3D mesh files that efficiently translate and load even the largest files for inspection. MorphoSource has demonstrated success in accepting and disseminating raw data sets of large size (>4 GB, with largest files accepted over the web so far being ∼70 GB). With the launch of MorphoSource 2.0, 3D volumes that are automatically derived from CT stacks or MRI image series can be viewed in a web browser. The new web-based viewer also allows display of colorized images and the ability to create annotations and measurements on web assets for which the JSON code can be copied and shared with other users. Because of its ability to support the complex metadata, data format, and rights concerns applicable to museum-sourced 3D data, MorphoSource has been growing rapidly over the past few years; to date, it has received over 161,000 data sets representing 54,000 specimens and surpassed 17,000 users.
Through downloads by registered users, MorphoSource actively collects information on preferred file types and visualization software and has adapted to the user community, including oVert as its needs have crystallized or changed over the course of the project. By centralizing management of the media files for specific institutions through new “organizational teams” collection managers can use MorphoSource as a tool for providing access to digital representations of their specimens, effectively digital loans of specimens.
One significant advance made early in the oVert project was the use of the iDigBio API (Application Programming Interface) to populate DarwinCore-structured metadata (Wieczorek et al. 2012) for specimens added to MorphoSource. We thus capitalized on previous digitization efforts and enabled a system by which we can keep specimen metadata in sync with that published by participating collections. We used the iDigBio API because it makes data available for US institutions, but the same process could be applied to other APIs such as those from GBIF. To facilitate the upload of dozens to hundreds of data sets at a time to MorphoSource, we developed Python and R scripts for batch uploading data sets. These scripts pull specimen data from iDigBio and the metadata from multiple types of files generated by CT scanners (e.g., acquisition log files) to build a file containing metadata for these other files (i.e., a manifest file) that is used to batch upload CT tomogram and shape-file media to MorphoSource. These are freely available online for others to customize and use (https://github.com/nsvitek/CT_tools, https://github.com/drScanley/MorphosourceBatch). By capturing information about the institution and collection from the data published online by museum institutions (e.g., record sets), we can collate information on the specimens and media represented in MorphoSource for each institution. This extends to including details on the usage of these media as captured by the number of views and downloads, the text from any approved download requests, and checked boxes indicating a variety of use intent categories. With these usage data in hand, individual collections can easily determine the impact of the digital representations of their specimens for research and education. Further, because specimens from a given museum might be scanned at different facilities, this process also enables media to be sorted efficiently in MorphoSource into institutional management teams through which institution-specific usage agreements and oversight can be applied.
We strived to make any and all data generated by oVert available for research, education, art, and other noncommercial uses (figure 2). In doing so, we advocate for users to properly attribute museums and their collections such that they receive proper credit for making these data available. By making clear in MorphoSource those institutions that own and manage these digital representations, we make it possible for potential commercial users to know how to begin conversations about making use of these data. MorphoSource includes the ability for institutions to customize user agreements that make explicit their expectations about usage. In some cases, institutions choose to manage their data in a more restricted way, which could be warranted for rare or commercially valuable data sets or culturally sensitive objects or specimens. Data derived from 3D-imaging represent factual measurements (e.g., data) that are not protectable under copyright law in the United States (D'Andrea et al. 2022). Similarly, because CT images are generated by a machine and not a person, they do not qualify as “human authorship” in the United States; “medical imaging produced by X-rays” are specifically mentioned as lacking human authorship (US Copyright Office, Compendium of US Copyright Office Practices § 313, 3D ed. 2021). To be clear, diagrams, illustrations, and figures (such as figure 1) made using these 3D data are created by human authors and are protectable, even though the 3D data themselves or not. For these reasons, MorphoSource provides flexibility such that institutions can manage usage expectations of their 3D data by either applying Creative Commons licenses—which fall under copyright law in the United States—or through the user agreements that allow institutions to manage usage via contract law.
oVert data generated to date
As of November 2023, the oVert project has generated more than 29,000 media files for more than 13,000 specimens, in part through approximately 380 loans of specimens exchanged across 50 US institutions (figure 3). These represent more than 6400 genera in more than 970 families of living vertebrates. We have generated data for more than half of all genera of fishes, amphibians, reptiles, and mammals (figure 3, supplemental figure S1), including most amphibian and reptile genera. We continue working through imaging many genera, especially of fishes. Because of the lack of availability of fluid-preserved specimens, we anticipate not being able to make significantly more progress for bird and mammal genera based on existing collections in the United States.
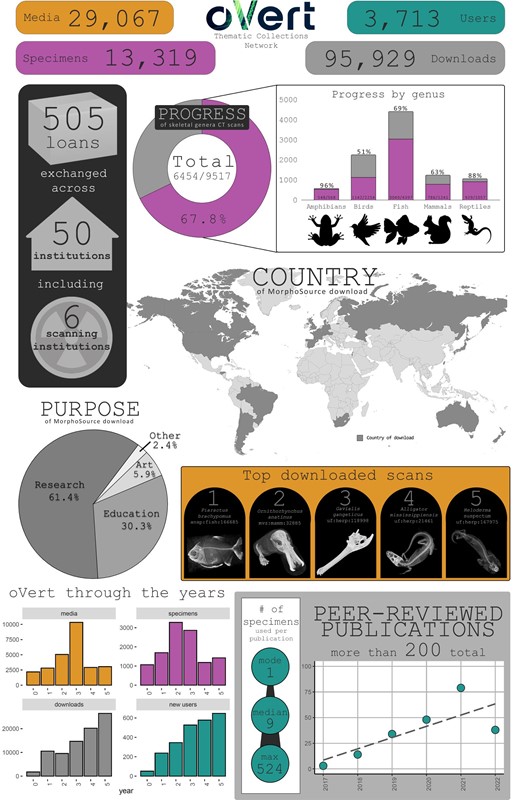
Infographic summarizing key aspects of data generation and use during the oVert Project. The data on downloads and users of media are based on data files generated by MorphoSource for media files hosted in that repository for the oVert project and associated PENs (as of 1 November 2023); we cleaned these data to remove downloads and users that appear to be nonhuman automated requests “bots”. Publication data are based on curated information from Google Scholar. The top downloaded data set of the fish Piaractus (ANSP: Fish:166685, https://doi.org/10.17602/M2/M27533) was suggested as a reference data set for users of 3D Slicer by Buser and colleagues (2020).
Approximately 25% of the specimen data sets from oVert that are now in MorphoSource were generated by partner projects. Of these, the most significant is the oUTCT project that made more than 2,100 CT data sets (representing >1600 specimens) generated at UTCT available in MorphoSource. The oMEGA project generated more than 7500 media files that mostly represent individual bones imaged from approximately 170 large vertebrate skeletons. Several other partner projects remain ongoing at this time and will continue to generate new data sets.
Communities benefiting from oVert data
Natural history collections, researchers, educators, and artists all benefit from the availability of digital 3D representations of specimens on MorphoSource (figures 3 and 4). To date, the data generated by oVert and associated partner projects have been viewed more than one million times, and downloaded approximately 95,000 times by more than 3700 users. Those using oVert data include undergraduate and graduate students, postdoctoral scientists, faculty, collection managers, technicians, K–12 teachers, artists, exhibit staff, and more. This, however, is a conservative estimate of oVert's impact. It does not include the impact of people using the 3D web viewer in MorphoSource 2.0 in which digital specimens can be viewed and measured without downloading the data sets. While these data have been mostly used for research, they are also increasingly popular for education and art.
![Examples of non-research uses of CT scans downloaded from MorphoSource. These data have been used in museum exhibits, including (a) large format prints (from Inner Beauty exhibit at Florida Museum of Natural History [FLMNH], 2021–2023) and (b) manipulatable 3D models on touchscreens (arrow; from Fantastic Fossils exhibit at FLMNH, 2022); for education, including (c) 3D prints used in teaching comparative anatomy (photograph: Christopher Sheil), (d) learning activities posted on QUBESHub, and (e) multipart digital models hosted on Sketchfab; and by artists, such as (f) a sculptural work titled “frog” (sculpture: Margaret Honda, 2019, Carnegie Museum of Art: A. W. Mellon Acquisition Endowment Fund, 2019.71; photograph: Bryan Conley; inspired by Conraua goliath, UF: Herp:64720, https://doi.org/10.17602/M2/M39477, and Pelophylax ridibunda, CAS: Herp:217695, https://doi.org/10.17602/M2/M49916), and (g) digital art of a bushmaster Lachesis muta (art: Erwin van der Minne, based on FMNH: Amphibians and Reptiles:31178, ark:/87,602/m4/M115937).](https://oup.silverchair-cdn.com/oup/backfile/Content_public/Journal/bioscience/74/3/10.1093_biosci_biad120/1/m_biad120fig4.jpeg?Expires=1750181186&Signature=gNm-v2p8xdQmtNlF9SL2LC1iPJI4D5MZZeMaeVIA~Wcnb9isVU3GkPhE04GhCCJtk05ijl6BchK5wgk9avc0bzBIgWoYurH2n75Ec2YCVmoDs5dJj9wSTqlh4k7p7HGLK5SdyB8ekjv5jLPSqW3I9kv6GUp7YznpTJEE~4r72nRLpB4O9G9xBA10XJjqzh518HH~NrV2yayt8aeIBdQAaoSC2m6MppFGDwfoE2UGpOv-XDK9jN4oELo424NjR7~qdNHbUzfKdGovNVEmQ~xuCicxEUQJvYU9VsgVhtGNni94-Bq~nRU7RkNvItbygR6na6KwJrtvqPFQAVXTx7Skbg__&Key-Pair-Id=APKAIE5G5CRDK6RD3PGA)
Examples of non-research uses of CT scans downloaded from MorphoSource. These data have been used in museum exhibits, including (a) large format prints (from Inner Beauty exhibit at Florida Museum of Natural History [FLMNH], 2021–2023) and (b) manipulatable 3D models on touchscreens (arrow; from Fantastic Fossils exhibit at FLMNH, 2022); for education, including (c) 3D prints used in teaching comparative anatomy (photograph: Christopher Sheil), (d) learning activities posted on QUBESHub, and (e) multipart digital models hosted on Sketchfab; and by artists, such as (f) a sculptural work titled “frog” (sculpture: Margaret Honda, 2019, Carnegie Museum of Art: A. W. Mellon Acquisition Endowment Fund, 2019.71; photograph: Bryan Conley; inspired by Conraua goliath, UF: Herp:64720, https://doi.org/10.17602/M2/M39477, and Pelophylax ridibunda, CAS: Herp:217695, https://doi.org/10.17602/M2/M49916), and (g) digital art of a bushmaster Lachesis muta (art: Erwin van der Minne, based on FMNH: Amphibians and Reptiles:31178, ark:/87,602/m4/M115937).
The availability of digital data took on new importance during the COVID-19 pandemic. In-person work at museums slowed or stopped during the pandemic due to both the inability to travel and many institutions not sending loans of specimens. The digital data generated by oVert provided the capacity for people to continue working, learning, educating, and creating using vertebrate specimens during this time. More than half of all downloads of data from MorphoSource occurred since March 2020.
Benefits to museums
Natural history collections already support scientific research locally, nationally, and globally. Digital 3D representations of specimens allow for the use of collections without the need to examine them in person, through a visit to the collection, or via a loan. These “digital visitors” expand the research impact of specimens in the collection and facilitate use by scientists that would not be able to visit or receive loans. Whereas most loans of specimens relate to research, the digital representations of specimens are also used frequently in education and art (figure 4). Artistic renderings of these 3D data have also been used widely in exhibits (figure 4a,b), including ones at the Florida Museum of Natural History, Texas A&M University (e.g., Revival exhibit, 2021), and the Bruce Museum. A single specimen might be used simultaneously in several research projects as well as in teaching in high school and college classrooms and by artists and museum exhibit designers, though not every specimen will be of such broad interest. The digital representation of specimens online means that museums can satisfy interests in a high-value specimen across disparate user communities without compromising the primary research mission of most natural history collections.
In preparing data on US museum collections as part of designing our sampling approach for oVert, we identified a number of shortcomings of collections of extant vertebrates. In some cases, there are no suitable fluid-preserved specimens in the United States (or elsewhere) representing a particular genus. Many bird and mammal genera have no fluid-preserved specimens in the United States. This is true for several orders of mammals for which adult specimens are too large to be fluid preserved. In the rare cases of existing, large fluid-preserved specimens (e.g., Gorilla gorilla, https://doi.org/10.17602/M2/M56407), these usually must be CT scanned on medical or veterinary scanners. This results in lower-resolution anatomical data (at least a tenth the resolution of other data generated by oVert), limiting some of the downstream research users, such as those focused on internal microstructure. Another challenge is that many fluid-preserved bird specimens were shot during the collecting event and can thus be full of lead birdshot. Bird bones are relatively low density and have limited contrast to the soft tissues as is, and the presence of high density birdshot attenuates and scatters X-rays, resulting in a range of artifacts that further reduce the quality of the tomograms. By highlighting these sampling gaps, oVert has inspired several different collections (especially those of birds) to augment current collection practices with higher-quality intact fluid-preserved specimens.
Last, valuable natural history information is often discovered during CT scanning that enhances data associated with these specimens. This “natural history bycatch” can include information on the number and size of eggs, prey items, pathologies, and parasites, which can be both added to collection databases and published as natural history notes (e.g., Blackburn et al. 2017a, 2017b, Paluh et al. 2019, Weinell et al. 2019, 2021). These data provide potential users of physical specimens with a more targeted approach to selecting specimens for destructive sampling as they can better determine specimens of interest.
Benefits to researchers
To date, more than 200 peer-reviewed scientific publications (with >680 cumulative authors) cite data generated by oVert (figure 3, supplemental table S2). These cover a broad range of topics in vertebrate biology, including descriptions of new species, systematics, comparative anatomy, functional anatomy, paleontology, and trait evolution. In some cases, the data downloaded from MorphoSource are the major or only source of data in a research project. In others, these data supplement or complement existing data sets. Because of the availability of 3D mesh files, some scientists have coded characters for phylogenetic analyses by viewing these data in MorphoSource's in-browser web viewer (e.g., Henrici et al. 2018, Báez and Gómez 2019).
This accessibility of these data removes potential financial and temporal barriers that might otherwise prohibit a researcher from including a taxon or specific specimen in their analyses. Entire research projects can be completed without the need to travel to collections or receive specimens on loan from their home institutions. For example, the trait data scored in Paluh and colleagues (2021) were collected by coauthors all working remotely during the COVID-19 pandemic. In addition, the sample sizes of existing data sets can be increased by using these data, strengthening those studies. Another obvious benefit is that a specimen may be used in different projects by multiple researchers at any given time. For example, during a 3-year period (2020–2022), more than 43 unique users downloaded the same data set of a gharial skull (Gavialis gangeticus; UF: Herp:118,998; https://doi.org/10.17602/M2/M39794), resulting in at least 10 publications from authors in seven countries (Hone et al. 2020, Bowman et al. 2021, Cowgill et al. 2021, 2022, Pochat-Cottilloux et al. 2021, Serrano-Martínez et al. 2021, Iijima et al. 2022, Schwab et al. 2022a, 2022b, Tahara and Larsson 2022). These digital data grow the number of researchers able to interact with specimens, especially people from Low and Middle Income Countries lacking local collections or the resources to travel to other collections. These 3D data do not replace the need for examining physical specimens. Indeed, as members of the oVert community have found, these data have opened doors to research questions that necessitate yet more data collection from specimens in natural history collections.
Benefits to educators and students
Digital 3D representations open the possibility of thousands of people interacting with museum specimens in classrooms and through online learning. From just those institutions funded by the oVert project, more than 2300 students interacted with images, videos, or digital or 3D-printed models of specimens during 2017–2022. The importance of these resources to educators became more acute during the COVID-19 pandemic during which time many were looking for dynamic, accessible, and free resources as they moved classrooms online. Just as many researchers might simultaneously interact with a digital specimen, it is easy for many students to simultaneously manipulate the same 3D representation of a specimen. For example, the gharial skull (Gavialis gangeticus; UF: Herp:118,998) mentioned above has been viewed more than 1500 times on Sketchfab (https://skfb.ly/6SWxU). These 3D representations enable self-guided inquiry into topics such as anatomy, form–function relationships, and evolution. They also create the opportunity to highlight the valuable role of natural history collections in research on these topics, which is often not articulated in K–12 or undergraduate classrooms.
There are many ways to bring these 3D representations of specimens into the classroom. Among the easiest is to have students interact with 3D mesh files through online viewers incorporated into MorphoSource or Sketchfab (figure 2). By incorporating annotations with text and images, Sketchfab provides a particularly useful tool that is directly accessible through a web browser and users do not need any expertise in processing CT data or using a scientific data repository (i.e., MorphoSource). Further, multiple 3D models can be arranged into a single “scene” that, together, convey information, such as the diversity and homology of the tetrapod forelimb (figure 4; https://skfb.ly/6UFqy). 3D mesh files can also be used to 3D print replicas for hands-on lab exercises. Pairing scientific specimens from a teaching collection with 3D prints or digital models on an iPad provides an opportunity for students to easily manipulate internal anatomy (e.g., a skull), including for animals for which dry skeletal material would be extremely small and delicate. A more intensive activity—and most appropriate for courses such as Comparative Vertebrate Anatomy—would be to have students use CT data to create their own models for 3D printing or digital manipulation.
During the oVert project, we worked directly with middle school and high school teachers to develop learning activities for their classrooms involving 3D models of vertebrates. These workshops were led by oVert team members (Julie Bokor and authors DCB, CME, JAG, and ELS) and done in collaboration with other researchers. Teachers worked closely with scientists to develop learning activities for the classroom based on museum specimens and scientific research (supplementary table S3). In some cases, these activities involved simply looking at and interpreting 3D models, whereas others were more involved and required students to collect data from models. For example, an exercise using 3D models on Sketchfab involved students collecting data on the volume of bony armor in different regions of cordylid lizard skeletons so that students could evaluate the relationship between extent of armor and lizard ecology (Broo 2020); this published learning activity on QUBESHub (figure 4) has been used extensively (>1600 views, >480 downloads as of 1 November 2023). Many of the Sketchfab models developed for these activities have been viewed thousands of times online; cumulatively, these models have been viewed more than 100,000 times (as of 1 November 2023; see supplementary table S3).
Benefits to artists
Objects and specimens from natural history museums have a long history of being used by artists as sources of inspiration or the bases for their artwork. Similarly, there is now a global community of artists working with digital anatomical data, some to create digital art but others to use as the basis for sculpture, painting, and more. The diversity of art projects mirrors the diversity of research, including a “wearable sculpture piece,” “animated shadow projections,” “scaled sculpture series,” and “pottery with animal skeletons.” One specific example includes Margaret Honda's large sculpture of the frog that appears in Bramantino's Madonna delle Torri (ca. 1520) painting. Honda's sculpture was exhibited at the Carnegie Museum of Art in 2019 and its internal anatomy is based on CT scans from oVert (figure 4).
What's next?
The oVert project has increased the reach and impact of vertebrate specimens in many scientific collections. We have shown what might be possible through 3D digital imaging of specimens, but there remain a number of obstacles. As our project concluded in August 2023, we use this opportunity to outline what we see as significant challenges going forward for the emerging global audience for these digital scientific specimens.
A need for better anatomical specimens
Fluid-preserved specimens have long served as a critical resource for understanding both external and internal anatomy. Yet the sampling of vertebrate specimens available in existing collections has clear biases. Disciplines like ichthyology rely primarily on fluid-preserved specimens, whereas these preparations remain rare in ornithology. Because studies of evolution and ecology using bird specimens have long relied on traditional study skins, many bird species are represented by few—if any—fluid-preserved specimens. There are skeletons of many bird species available in collection, though these are often partial skeletons because they were removed while keeping the skin intact. And, of course, these preparations do not preserve soft tissues useful for comparative morphological studies. In an era where connecting genotype and phenotype becomes both more possible and highly desirable, there is now a strong interest in preparing new bird and mammal specimens to serve as anatomical resources. This will only be possible as the community works to fill in sampling gaps of species and genera not represented in existing fluid collections.
By facilitating greater access, the resources generated by oVert might seem to reduce the need for collecting new specimens from the wild. Yet we still lack high-quality fluid-preserved voucher specimens for many vertebrate genera (let alone species). Moreover, it is increasingly clear that many questions about phenotypes in ecology, evolution, and organismal biology require large intraspecific samples to adequately characterize the population-level variability that fuels evolutionary processes. Consider, for example, testing the linkage between population-level microevolution and phenotypic macroevolution, where dozens to hundreds of individuals per species are necessary to estimate phenotypic covariance structures (e.g., Marroig and Cheverud 2004, Hlusko et al. 2016, Love et al. 2021). High-resolution 3D imaging of newly collected material has tremendous promise for these and related questions, by facilitating high precision, repeatable measurements across a near-infinite set of traits. Further, it is important to have high-quality fluid-preserved specimens associated with other resources, including tissues and other samples for genomic and microbial studies, as well as images, audio, and video of the animals while they were alive. Continuing to build collections of data-rich fluid-preserved specimens will help to fulfill the vision of “extended specimens.”
Because of the constraints of fixation and storage, intact fluid-preserved specimens of large vertebrate species remain rare in collections and often impractical to maintain. However, as many large vertebrates are also faced with extinction (Bennett and Owens 1997, Cardillo et al. 2005, Ripple et al. 2017), it is imperative that institutions coordinate the preservation of intact representatives of large species that can serve future generations of scientists. There are clear ethical challenges with collecting many of these large-bodied species from the wild, but partnerships between museums and wildlife agencies and both zoos and aquaria create opportunities for filling these gaps in existing museum collections (Cook et al. 2017, Poo et al. 2022).
More consistency in citing data sets
Successfully measuring the impact of digital representations of museum specimens and objects depends on users properly reporting information about these data in their works. A first step is to consistently cite specimens using an institutions’ preferred institutional and collection codes (i.e., a Darwin Core triplet such as UF: Herp:1234), but even better is to cite persistent identifiers such as Digital Object Identifiers (DOIs) or Archival Resource (ARK) for specific data (Mabry et al. 2022). While thanking institutions, collection staff, or generators of data in acknowledgments is appreciated, it does not provide easily accessible information about which specimens and data were used in a research study. Online repositories also need to make it easier to provide connections between specimen identifiers and data identifiers. Because we implemented using the iDigBio API within MorphoSource, it is straightforward for connecting new data deposited in MorphoSource to the specimen identifiers used by institutions in the record sets represented in the iDigBio aggregator. But other online resources for digital specimen data (e.g., GenBank, Macaulay Sound Library, Dryad, Open Science Network) do not provide users with the ability to easily connect newly generated data to existing specimen records. Similarly, repositories such as MorphoSource must make it easier for users of digital specimen data to quickly find, collate, and report the persistent identifiers of data sets. We must move from gracious text in acknowledgments to Open Access tables containing identifiers for both specimens and associated data.
Promoting the role of agents in the Extended Specimen Network
oVert has provided a galvanizing model for a functional node within an Extended Specimen Network (Lendemer et al. 2020). Although each resource in the network might be deeply specialized for holding and making discoverable the data it holds, that is not enough. For a tool such as MorphoSource to thrive and provide a useful data resource, it must be designed in a way that creates inclusive, community-wide incentives for building and maintaining its holdings. A narrow perspective, focused only on discovery, access, and reuse of research data can lead to solutions that exclude needs of museums, imaging facilities, the public, and contributors. If the needs for access, management, and attribution by these other communities are not considered and centered, the system cannot thrive. This is not a new revelation, and it is so well understood that best practice standards of digital preservation (PREMIS Data Dictionary for Preservation Metadata) already include both “agents” and “rights” as key elements of their data models. Such data models are silent on how to record and measure access to data. However, if databases are not built from a foundation that explicitly considers unique people and institutions as agents with relationships to objects like specimens and media, it will be impossible to serve diverse and changing needs of multiple communities at larger scales.
MorphoSource is built on a data model that relates “objects” (e.g., specimens, scanners, scans, organizations, projects, teams) to each other and users through “rights” (or roles; e.g., manager, reviewer, depositor) and “events” (e.g., imaging events, processing events). Following best practices for digital preservation is key to MorphoSource's strength in providing deep support for 3D-imaging workflows. But it is also important for efficiently and appropriately displaying and distributing management, attribution, and impact of data to the correct users and user groups. MorphoSource carefully defines its scope and role within the emerging Extended Specimen Network. With that scope intentionally defined, it becomes easier to identify other appropriate and critical nodes for linking. For example, MorphoSource primarily uses both iDigBio (as source of specimen record data) and GBIF (as a comprehensive and updated source of taxonomy information) to complete its data records. New infrastructure tools are needed to integrate with museum repositories and data resources like MorphoSource to build a rich network of data that extends museum specimens and increases their impact. Unique identifiers for individuals (e.g., ORCID; https://orcid.org) or institutions and facilities (e.g., Research Resource Identifiers; www.rrids.org) provide a starting place for keeping track of the many agents working with an Extended Specimen Network. Projects such as oVert are only made possible via individuals and institutions, and exist to serve people across different communities. Critical to the success of oVert and similar future endeavors is explicitly recognizing the needs and rights of contributors, users, and institutions.
New tools for working with rich and diverse 3D data
Our community lacks analytical tools for using hundreds or thousands of 3D data sets for research projects. As of May 2023, MorphoSource contains more than 170,000 media files for more than 60,000 specimens or objects. Despite the more than 13,000 specimens imaged by the oVert project, most studies published using oVert data include only a handful in their analysis. Based on a survey of 195 publications that reported using oVert data, the median number of CT-scanned specimens (derived from oVert or otherwise) is nine and the mode is one (supplemental table S2). Only 22 publications used CT scans of more than 100 specimens in their analyses, only eight publications used more than 200 specimens, and only two publications used more than 500 specimens (Nojiri et al. 2021, Paluh et al. 2021). 3D data for vertebrate specimens are now more accessible than ever, but there are bottlenecks in analyses that prohibit using large numbers of these data sets in a single study. We anticipate that new and better tools for using Artificial Intelligence (AI) will enable working with 3D anatomical data at larger scales (>1000 specimens). These tools include fully or semi-automated segmentation (Rolfe et al. 2021) and geometric morphometrics (Boyer et al. 2015, Vitek et al. 2017), including 3D landmarking (Porto et al. 2021, Zhang et al. 2022). But these tools might also enable using 3D data sets, especially from CT scans, to ask new and different research questions. For example, if we have 10,000 CT scans on hand, can we find those scans in which a vertebrate skeleton is located within the boundaries of another vertebrate (e.g., stomach contents, gravid females)? Without opening TIFF stacks in analytical software used for segmentation, for a set of CT scans can we determine the volumes of the mineralized skeleton, heart, or brain relative to the body volumes for those specimens? Similarly, can we quickly score the presence or absence of a trait (such as teeth on the maxilla) across hundreds or thousands of data sets without segmenting them? The field of medical imaging has developed many AI-based tools (Egger et al. 2013, Yushkevich et al. 2016), especially for radiology (e.g., Diaz-Pinto et al. 2022, Inkeaw et al. 2022), but these benefit from having large numbers of data sets with similar phenotypes (e.g., humans). We now need to leverage those or similar AI-based tools for asking questions of heterogeneous 3D data sets that span the range of vertebrate phenotypes.
More training in using 3D data
The oVert project and similar initiatives are generating thousands of 3D anatomical data sets and these are now accessible through web-based resources such as MorphoSource, MorphoMuseum, Phenome10K, and more (Lebrun and Orliac 2016, Davies et al. 2017). Yet many students, scientists, and museum collection staff remain unfamiliar with generating, analyzing, curating, and preserving these 3D anatomical data sets. Throughout the oVert project, we have hosted workshops at conferences (e.g., American Society of Mammalogists), on site at scanning facilities (e.g., Friday Harbor Laboratories, UTCT, University of Florida's Nanoscale Research Facility), and museums (Florida Museum of Natural History). We have also generated web-based tutorials such as videos on YouTube. To reach more potential users of 3D specimen data, we need more easily accessible online resources aimed at a general audience, not only scientists. The recent NSF-funded Non-Clinical Tomography Users Research Network (NoCTURN, https://nocturnetwork.org) provides a starting place for this work by developing working groups focused on findability, accessibility, interoperability, and reuse of CT data and is working to reach communities in research, education and outreach, and industry.
Information technology (IT) professionals form an important support community within museums and institutions that is often overlooked. We have found that engaging these staff early and often throughout our 3D digitization projects is important to successfully curating and preserving these data into the future. It is important for museum collection staff to gain more familiarity with 3D data, but they cannot be expected to shoulder the burden of supporting digital 3D data alongside the laborious care of physical objects. Museums must invest in growing their IT staff to ensure stability of and access to 3D and other complex digital data. At the beginning of the oVert project, the University of Michigan Museum of Zoology—one of the largest university-based museums in the United States with approximately 15 million specimens—was supported by only one-tenth of one IT staff position, but now has two full-time IT staff to support digital infrastructure, including 3D data.
Bringing 3D data to classrooms
Teachers and students are often excited by the opportunity of interacting with digital or printed 3D models representing museum specimens but lack resources and training to make the most of available data. To fully realize the educational potential of these digital data, scientists and museum staff must partner with educators to develop resources that are useful for their classrooms and to understand the technological limitations that they face. Through workshops with K–12 teachers in the United States, the oVert team learned that many teachers do not have administrative privileges to add software or download data to classroom computers. Thus, we developed online 3D models on Sketchfab rather than developing learning activities that require downloading data locally to manipulate or analyze. Working directly with teachers allowed our team to customize activities to their specific classroom needs and then to share these resources online (e.g., via QUBESHub) so that other teachers could access and remix these for their own classroom activities. Our community needs a richer collection of educational resources for K–12, undergraduate, and graduate students that highlight the variety of vertebrates, the breadth of research questions, and the multitude of scientists asking those questions. As science standards vary by grade level, state, and country, it is important to share these resources online in a way that enables them to be repurposed by educators for their students.
Better access for more people
It is enticing to think that a greater diversity of people will encounter and make use of museum specimens because of online digital representations. But it is not sufficient to put data online and simply hope that people find and know how to use these data. We must identify communities that are not using these resources, understand their obstacles to finding, accessing, and using the data, and then design approaches that will promote those communities engaging with our digital collections.
There are many scientific audiences that have traditionally not interacted with natural history collections, including those in medicine, veterinary medicine, and engineering. Details from requests to download data from MorphoSource make clear that at least some people in these communities have already discovered these 3D resources. Examples include using digital or 3D printed models for “veterinary procedure reference,” “teaching undergraduate veterinary students,” “bio-inspired engineering,” “develop[ing] new 3d printing processes and systems,” and “study of the lumbar vertebrae” (text taken from MorphoSource download requests). The museum community would benefit from reaching out to and developing resources for these scientists.
We also must give serious thought to how non-research users can easily share their usage of specimen data. An easy path for researchers is to cite persistent identifiers (e.g., DOI, ARK) in their papers. But how does an artist or a student cite these data to make clear their “use” of specimens? One advantage of using persistent identifiers for data sets is that their impact can be measured through resources such as Altmetric (www.altmetric.com). It is exciting to many in the museum community that artists, educators, and more are using these data. If we want to track their use and impact, then we need better approaches to engage these users and foster best practices in how they reference data so that all appropriate people and institutions receive credit.
oVert is over, but 3D imaging is accelerating and expanding
oVert was a first step in creating a community to digitize, archive, and disseminate 3D digital data sets of vertebrate museum specimens. The genus-level sampling generated from this project serves as a scaffold for others to build upon, adding their data sets to a growing repository (i.e., MorphoSource) and closing gaps in taxonomic sampling. Whereas oVert created a backbone of vertebrate sampling, there are exciting opportunities for 3D imaging of other organisms in natural history collections, such as invertebrates (Nguyen et al. 2014, Ströbel et al. 2018, Semple et al. 2019, Edie et al. 2023), plants (Leménager et al. 2022, Wang et al. 2022), or even living vertebrates (Bot and Irschick 2019, Irschick et al. 2020). New advances in site-based imaging provide opportunity for situating digital versions of specimens within digital representations of their original context, whether excavation sites or coral reefs (Pyenson et al. 2014, Magnani et al. 2020, Bongaerts et al. 2021). As we expand and diversify these digital representations, we must put concerted effort into understanding hurdles to access and use by communities both within and outside of science. By developing new opportunities for engaging wider audiences, we anticipate that the impact of our collective digital 3D data for museum specimens will increase exponentially.
Acknowledgments
We thank NSF—and, specifically, Reed Beaman—for believing in the mission of the oVert project. This work would not have been possible without the substantial funding from awards made across participating institutions (NSF grants no. DBI-1700908, no. 1701402, no. 1701516, no. 1701665, no. 1701713, no. 1701714, no. 1701737, no. 1701769, no. 1701797, no. 1701870, no. 1701932, no. 1701943, no. 1702143, no. 1702263, no. 1702421, no. 1702442, no. 1802491, no. 1902105, no. 1902242, no. 2001435, no. 2001443, no. 2001474, no. 2001652, no. 2101909, and no. RCN-2226185). The iDigBio project (NSF grant no. 1547229) supported a workshop in February 2020 hosted at Duke University titled “Integrating Institutional Archives with Disciplinary Web Repositories” that catalyzed changes in user agreements at institutions, especially through feedback from Kyle K. Courtney (Harvard University). Last, we thank all of the many users of oVert-generated data for sharing information about their work via MorphoSource, their publications, and personal communication. Without that information, demonstrating the impact of digital 3D objects would be remarkably difficult. The entire oVert Project Team would greatly appreciate users citing this article when publishing using data generated by our project.
Author contributions
David C. Blackburn (Conceptualization, Funding acquisition, Methodology, Project administration, Resources, Supervision, Writing – original draft, Writing – review & editing), Doug M. Boyer (Funding acquisition, Project administration, Resources, Software, Writing – review & editing), Jaimi A. Gray (Conceptualization, Data curation, Formal analysis, Methodology, Visualization, Writing – original draft, Writing – review & editing), Julie Winchester (Formal analysis, Methodology, Resources, Writing – review & editing), John M. Bates (Funding acquisition, Project administration, Resources, Writing – review & editing), Stephanie L. Baumgart (Data curation, Formal analysis, Investigation, Writing – review & editing), Emily Braker (Data curation, Formal analysis, Funding acquisition, Investigation, Project administration, Resources, Supervision), Daryl Coldren (Data curation, Resources), Kevin W. Conway (Data curation, Funding acquisition, Investigation, Project administration, Resources), Alison Davis Rabosky (Funding acquisition, Project administration, Resources, Supervision), Noé de la Sancha (Conceptualization, Data curation, Funding acquisition, Project administration, Resources, Supervision), Casey B. Dillman (Data curation, Funding acquisition, Project administration, Resources), Jonathan L. Dunnum (Data curation, Resources), Catherine M. Early (Data curation, Investigation, Project administration, Supervision), Benjamin W. Frable (Data curation, Resources), Matthew W. Gage (Data curation, Formal analysis, Investigation, Resources), James Hanken (Funding acquisition, Project administration, Resources), Jessica A. Maisano (Data curation, Project administration, Resources, Supervision, Validation), Ben D. Marks (Data curation, Resources, Supervision), Katherine P. Maslenikov (Data curation, Resources, Supervision), John E. McCormack (Data curation, Funding acquisition, Investigation, Project administration, Resources, Supervision, Writing – review & editing), Ramon S. Nagesan (Data curation, Formal analysis, Investigation, Resources), Gregory G. Pandelis (Data curation, Formal analysis, Investigation), Heather L. Prestridge (Data curation, Resources, Visualization), Daniel L. Rabosky (Data curation, Funding acquisition, Project administration, Resources, Supervision), Zachary S. Randall (Data curation, Formal analysis, Investigation, Resources, Supervision, Writing – review & editing), Mark B. Robbins (Data curation, Resources), Lauren A. Scheinberg (Data curation, Resources), Carol L. Spencer (Data curation, Funding acquisition, Project administration, Resources, Supervision), Adam P. Summers (Conceptualization, Data curation, Investigation, Resources, Supervision, Visualization, Writing – review & editing), Leif Tapanila (Conceptualization, Data curation, Formal analysis, Funding acquisition, Project administration, Resources, Supervision), Cody W. Thompson (Data curation, Resources, Supervision, Writing – review & editing), Luke Tornabene (Data curation, Funding acquisition, Project administration, Resources, Supervision), Greg J. Watkins-Colwell (Data curation, Project administration, Resources), Luke J. Welton (Data curation, Funding acquisition, Project administration, Resources, Supervision), and Edward L. Stanley (Conceptualization, Data curation, Formal analysis, Funding acquisition, Project administration, Resources, Supervision, Visualization, Writing – original draft, Writing – review & editing).
Author Biography
David C. Blackburn ([email protected]), Jaimi A. Gray, Zachary S. Randall, and Edward L. Stanley are affiliated with the Florida Museum of Natural History (FLMNH), at the University of Florida, in Gainesville, Florida, in the United States; Blackburn served as the lead principal investigator for the oVert Thematic Collections Network. Doug M. Boyer and Julie Winchester are affiliated with Duke University, in Durham, North Carolina, in the United States. John M. Bates, Daryl Coldren, and Ben D. Marks are affiliated with the Field Museum of Natural History, in Chicago, Illinois, in the United States. Stephanie L. Baumgart was affiliated with the University of Chicago and is now affiliated with the University of Florida, in Gainesville, Florida, in the United States. Emily Braker is affiliated with the University of Colorado, in Boulder, Colorado, in the United States. Kevin W. Conway and Heather L. Prestridge are affiliated with Texas A&M University, in College Station, Texas, in the United States. Alison Davis Rabosky, Ramon S. Nagesan, Gregory G. Pandelis, and Daniel L. Rabosky are affiliated with the University of Michigan, in Ann Arbor, Michigan, in the United States. Noé de la Sancha was affiliated with Chicago State University and is now affiliated with DePaul University, in Chicago, Illinois, in the United States. Casey B. Dillman is associated with Cornell University, in Ithaca, New York, in the United States. Jonathan L. Dunnum is affiliated with the Museum of Southwestern Biology, at the University of New Mexico, in Albuquerque, New Mexico, in the United States. Catherine M. Early was affiliated with FLMNH and is now affiliated with the Science Museum of Minnesota, in St. Paul, Minnesota, in the United States. Benjamin W. Frable is affiliated with the Scripps Institute of Oceanography, at the University of California, San Diego, in San Diego, California, in the United States. Matt W. Gage and James Hanken are affiliated with Harvard University, in Cambridge, Massachusetts, in the United States. Jessica A. Maisano is affiliated with the University of Texas at Austin, in Austin, Texas, in the United States. Katherine P. Maslenikov, Adam P. Summers, and Luke Tornabene are affiliated with the University of Washington, in Seattle, Washington, in the United States. John E. McCormack is affiliated with Occidental College, in Los Angeles, California, in the United States. Mark B. Robbins and Luke J. Welton are affiliated with the University of Kansas, in Lawrence, Kansas, in the United States. Lauren A. Scheinberg is affiliated with the California Academy of Sciences, in San Francisco, California, in the United States. Carol L. Spencer is affiliated with the University of California, Berkeley, in Berkeley, California, in the United States. Leif Tapanila is affiliated with Idaho State University, in Pocatello, Idaho, in the United States. Greg J. Watkins-Colwell is affiliated with Yale University, in New Haven, Connecticut, in the United States. The oVert Project Team included many more participants, who are listed in supplemental table 1.
References cited
Author notes
The oVert Project Team included many more participants, who are listed in supplemental table 1.