-
PDF
- Split View
-
Views
-
Cite
Cite
Satoru Ato, Hiroshi Matsunami, Riki Ogasawara, Aging Is Associated With Impaired Postprandial Response of Skeletal Muscle Protein Synthesis to High-Intensity Muscle Contraction in Mice, The Journals of Gerontology: Series A, Volume 78, Issue 4, April 2023, Pages 587–595, https://doi.org/10.1093/gerona/glad014
- Share Icon Share
Abstract
The purpose of this study was to investigate whether aging alters the effect of nutritional status on contraction-induced muscle protein metabolism. In an overnight fasted or fed states, the right gastrocnemius muscle of young (3 months) and aged (24 months) male C57BL/6J mice was isometrically contracted via percutaneous electrical stimulation. The left gastrocnemius muscle served as a control. In the fasted state, there were no differences in basal or contraction-induced muscle protein synthesis between young and old mice. However, in the fed state, basal muscle protein synthesis was greater in young mice, and contraction increased muscle protein synthesis only in young mice. In the fed state, although phosphorylation of 4E-BP1 was similarly increased by contraction in both ages, the increase in phosphorylation of p70S6K was greater in young mice. Our results indicate that aging impairs the ability to integrate signals from muscle contraction and nutrition, leading to aging-induced anabolic resistance to muscle contraction in the postprandial state.
Aging is associated with both muscle atrophy, or sarcopenia, and decreased muscle function, or dynapenia. These changes can interfere with daily activities and increase the risk of disease. Thus, maintaining muscle mass and function is critical for healthy aging.
Skeletal muscle mass is maintained by regulating the balance between muscle protein synthesis and degradation. Although basal protein metabolism does not differ between young and old subjects (1), in aged muscle, autophagic regulation is impaired (2), and aged muscle is less sensitive to nutrition (3–5). These features may, in part, contribute to atrophy and decreased function due to a net negative protein balance and/or impaired protein accumulation.
Both muscle contraction and nutrition are important for maintaining homeostasis of muscle protein metabolism and muscle mass. High-intensity muscle contractions, including resistance exercise, increase muscle protein synthesis (6,7) and are the most effective way to increase muscle mass and function, whereas muscle inactivity (eg, limb immobilization) decreases muscle protein synthesis and leads to atrophy (8). Some studies, but not all (9), have reported that aging attenuates anabolic response to muscle contraction (10–12), indicating that aging diminishes anabolic sensitivity to contraction. Therefore, given that the anabolic effect of contraction and nutritional state interact (13), aging likely affects this interaction.
To better understand the aging-related features associated with sarcopenia, we investigated whether aging alters the effect of nutritional status on contraction-induced muscle protein metabolism. Specifically, we investigated the anabolic response by measuring protein synthesis- and ribosome biogenesis-related factors and investigated the catabolic response by measuring autophagy and ubiquitin–proteasome system pathways under both fasted and fed states in young and old mice. We also investigated chaperones that may be involved in a positive adaptive response to contraction by promoting protein folding/myofibrillar formation.
Materials and Methods
Animals
All experimental procedures in this study were approved by the Ethics Committee for Animal Experiments at Nagoya Institute of Technology. Male C57BL/6J mice purchased from CLEA Japan (Tokyo, Japan) were housed in an environment maintained at 22°C with a 12-hour light/dark cycle, with food and water ad libitum.
Experimental Procedures
In the fasted (12-hour overnight fast) and fed (ad libitum feeding) state, under isoflurane anesthesia, the right gastrocnemius muscle was contracted isometrically via percutaneous electrical stimulation (100 Hz, 5 sets of ten 3-second contractions, 7-second rest between contractions, 3-minute rest between sets) as described previously (14). The left gastrocnemius muscle served as a control. Muscle samples were obtained 3 hours after contractions. Tissues were frozen rapidly in liquid nitrogen and stored at −80°C until use.
Western Blotting
Powdered frozen muscle samples were homogenized in 10 volumes of buffer containing 20 mM Tris-HCl (pH 7.5), 1% Nonident P-40, 1% sodium deoxycholate, 1 mM EDTA, 1 mM EGTA, 150 mM NaCl, and Halt protease and phosphatase inhibitor cocktail (Thermo Fisher Scientific, Waltham, MA). After centrifugation at 10 000g for 10 minutes at 4°C, the supernatant was collected and the protein concentration of each sample was determined using a Protein Assay Rapid Kit II (FUJIFILM Wako Pure Chemical, Osaka, Japan). Samples were diluted in 3× sample buffer and boiled at 95°C for 5 minutes. Then, using 5%–20% or 10%–20% sodium dodecyl sulfate–polyacrylamide gradient gels, equal amounts of protein were separated using electrophoresis and subsequently transferred to ClearTrans SP polyvinylidene difluoride membranes (Wako). After transfer, the membranes were washed in Tris-buffered saline containing 0.1% Tween-20 (TBST) and blocked with RapidBlock Solution (Amresco, Solon, OH) for 5 minutes at room temperature. Membranes were washed and incubated overnight at 4°C with primary antibodies. Antibodies against phospho-Akt (Thr308, cat# 13038), phospho-Akt (Ser473, cat# 9271), total-Akt (cat# 9272), phospho-mechanistic target of rapamycin (mTOR) (Ser2448, cat# 5536), total-mTOR (cat# 2983), phospho-ULK1 (Ser757, cat# 14202), total-ULK1 (cat# 8054), phospho-AMP-activated protein kinase (AMPK) (Thr172, cat# 2535), total-AMPK (cat# 2532), phospho-p70S6K (Thr389, cat# 9205), phospho-p70S6K (Thr421/Ser424, cat# 9204), total-p70S6K (cat# 2708), phospho-rpS6 (Ser240/244, cat# 2215), total-rpS6 (cat# 2217), phospho-PRAS40 (Ser246, cat# 2640), total-PRAS40 (cat# 2610), phospho-4E-BP1 (Thr37/46, cat# 9459), total-4E-BP1 (cat# 9452), HK2 (cat# 2867), c-Myc (cat# 9402), ubiquitin (cat# 3933), and LC3 (cat# 2775) were obtained from Cell Signaling Technology (Danvers, MA). HSP72 (cat# ADI-SPA-810), HSP73 (cat# ADI-SPA-815), and HSP90 (cat# ADI-SPA-830) were obtained from Enzo Life Sciences (Farmingdale, NY). GLUT1 (cat# 07-1401) and GLUT4 (cat# 07-1404) were obtained from Millipore (Burlington, MA). PFKM (cat# 55028-1-AP) and REDD1 (cat# 10638-1-AP) were obtained from Proteintech (Chicago, IL). PGC-1α (cat# ab54481), HK1 (cat# A1054), p62 (cat# PM045), and UNC45B (cat# sc-162382) were obtained from Abcam (Cambridge, United Kingdom), ABclonal (Wuhan, China), MBL (Nagoya, Japan), and Santa Cruz Biotechnology (Santa Cruz, CA), respectively. The membranes were then washed again in TBST and incubated for 1 hour at room temperature with the appropriate secondary antibody. Membranes were visualized using chemiluminescent reagents, and the bands were detected using the C-DiGit Blot Scanner (LI-COR Biosciences, Lincoln, NE). The membranes were then stained with Coomassie blue, and the intensity of each protein band was normalized to that of the stained blot. Band intensities were quantified using Image Studio (LI-COR Biosciences).
Muscle Protein Synthesis
Muscle protein synthesis was measured by the in vivo SUnSET method (15). Under anesthesia, 0.04 μmol puromycin/g body weight (FUJIFILM Wako Pure Chemical) diluted in a 0.02-M phosphate-buffered saline stock solution was injected intraperitoneally. The gastrocnemius muscle was removed exactly 15 minutes after puromycin administration. Following homogenization as described above, and centrifugation at 2 000g for 3 minutes at 4°C, the supernatant was collected and processed for Western blotting. A mouse monoclonal anti-puromycin antibody (cat# MABE343, Millipore, Billerica, MA) was used to detect puromycin incorporation, which was evaluated as the sum of the intensity of all protein bands in the Western blot.
Real-Time PCR
Total RNA was extracted from 10 mg of powdered frozen muscle tissue using ISOGEN II (NIPPON GENE, Tokyo, Japan). Purity and concentration of RNA were measured using by spectrophotometer (Synergy HT; BioTek, Winooski, VT), and then 500 ng of total RNA was reverse transcribed into cDNA using by PrimeScript RT reagent Kit with gDNA Eraser (Takara Bio, Kusatsu, Japan). Real-time RT-qPCR was performed using appropriate primer and TB Green Premix Ex Taq II (Takara Bio) on Thermal Cycler Dice Real-Time System II (Takara Bio). Gene expression levels were measured by the absolute quantification method and were normalized by rpS18. The housekeeping rpS18 gene was unchanged by the experimental protocol. Primers used in the study were obtained from Thermo Fisher Scientific. Primer sequences were provided in Supplementary Table S1.
Statistical Analyses
Data were analyzed by 2-way analysis of variance. Post hoc analyses were performed using t tests, with a Benjamini and Hochberg false discovery rate correction for multiple comparisons. The level of significance was set at p < .05. The data that support the findings of this study are available from the corresponding author upon reasonable request.
Results
Animal Characteristics
The body weights of old mice were higher than those of young mice (Supplementary Table S2). Also, body weight in the fasted state was lower than in the fed state. Gastrocnemius muscle mass to body weight and maximal tetanic force of old mice were lower than young mice.
Protein Synthesis
Although basal fasted muscle protein synthesis was comparable in the 2 age groups, basal postprandial muscle protein synthesis was higher in young mice than in old mice (Figure 1A).
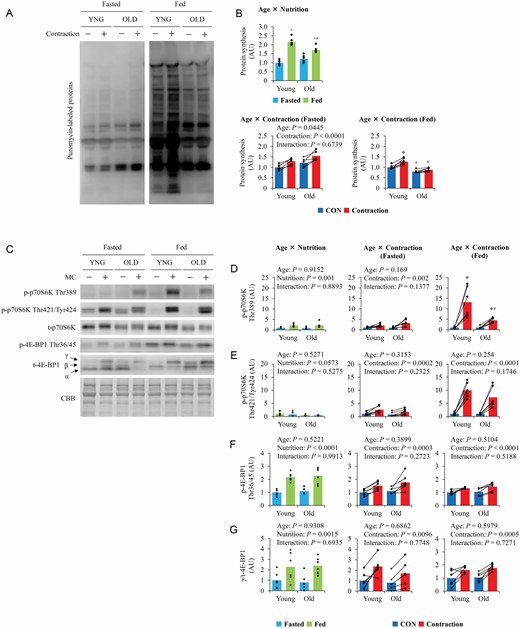
The influence of aging on nutrition- and contraction-induced muscle protein synthesis and mTORC1 activation. (A) Representative blot image of puromycin-labeled nascent polypeptide. (B) Quantitative values of muscle protein synthesis. (C) Representative blot image of mTORC1 substrates. (D–G) Quantitative values of mTORC1 substrates. Values are expressed by MEANS and individual values using bar–dot plot (n = 6 per condition). +p < .05 versus fasted. *p < .05 versus CON. #p < .05 versus similar condition in young.
In the fasted state, muscle contraction similarly increased muscle protein synthesis in the 2 groups (Figure 1B). However, in the fed state, muscle contraction further increased muscle protein synthesis in young mice but not in old mice (Figure 1B).
Downstream of mTOR
Total protein levels did not change with muscle contraction or feeding. Although total p70S6K levels did not significantly differ between the 2 age groups, total 4E-BP1 levels were higher in old mice than in young mice (Supplementary Figure S1A and B). Under fasting conditions, basal phosphorylation of p70S6K at Thr389 (Figure 1D), at Thr421/Tyr424 (Figure 1E), and 4E-BP1 at Thr36/45 (Figure 1F) did not differ between the groups. However, basal phosphorylation levels of p70S6K at Thr389 and 4E-BP1 at Thr36/45 were higher under fed conditions than the corresponding levels under fasting, although there was no significant difference between the age groups. Basal phosphorylation of p70S6K at Thr421/Tyr424 was lower under fed conditions than under fasting in both age groups.
In the fasted state, muscle contraction increased the phosphorylation of p70S6K at Thr389 and Thr421/Tyr424 and 4E-BP1 at Thr36/45, with no significant difference between the 2 age groups. Under fed conditions, muscle contraction similarly increased the phosphorylation of p70S6K at Thr421/Tyr424 and 4E-BP1 at Thr36/45 in both groups. However, although muscle contraction increased p70S6K Thr389 phosphorylation in both groups, the increase was lower in old mice than in young mice.
Upstream of mTOR
The expression levels of total-Akt and PRAS40 did not differ between the 2 age groups, while total-mTOR was higher in old mice than in young mice (Supplementary Figure S1C and D). Under fasting, phosphorylation of Akt and mTOR were higher in old mice than in young mice (Figure 2B and C). However, in the fed state, Akt and mTOR phosphorylation in old mice were either similar to or lower than that in young mice. In contrast, in both the fasted and fed states, basal phosphorylation of PRAS40 (Figure 2D), a downstream target of Akt, was comparable between the 2 age groups, although phosphorylation was higher in the fed state than in the fasted state in both age groups. Contraction did not affect Akt phosphorylation, although it slightly increased PRAS40 phosphorylation (p = .0686) in both age groups but only in the fasted state.
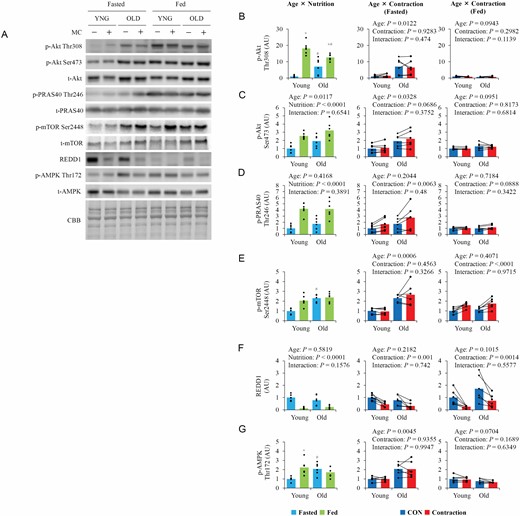
The influence of aging, nutrition, and contraction on upstream regulators of mTOR. (A) Representative blot image of upstream regulators of mTOR. (B–F) Quantitative values of upstream regulators of mTOR. Values are expressed by MEANS and individual values using bar–dot plot (n = 6 per condition). +p < .05 versus fasted. #p < .05 versus similar condition in young.
Basal REDD1 expression levels did not differ between the 2 age groups in either nutritional state but were significantly lower in the fed state than in the fasted state (Figure 2E). Contraction similarly decreased REDD1 expression in young and old mice in both nutritional states. In old mice, total-AMPK expression was lower (Supplementary Figure S1E), but basal phosphorylation of AMPK (Figure 2F) in the fasted state was higher. In young mice, AMPK phosphorylation was higher in the fed state than in the fasted state.
Basal p38MAPK phosphorylation was higher in the fed state than in the fasted state (Supplementary Figure S2A). In the fasted state, contraction increased p38MAPK phosphorylation only in old mice. Conversely, in the fed state, contraction increased p38MAPK phosphorylation in both age groups, but the increase was greater in young mice. Nutritional state did not affect ERK1/2 phosphorylation (Supplementary Figure S2B); however, contraction increased ERK1/2 phosphorylation in all groups except young, fasted mice.
Basal Igf1 mRNA levels in the fasted state were higher in old mice (Supplementary Figure S3B; main effect of age). Although Igf1 mRNA was higher in the fed state than in the fasted state in young mice but not in old mice, contraction did not change Igf1 mRNA levels in either age group. Basal mRNA levels of Ppargc1a4, an upstream regulator of Igf1 expression, did not differ between the 2 age groups in either the fasted or fed state (Supplementary Figure S3B). Contraction increased Ppargc1a4 mRNA levels in both nutritional states in young mice but only in the fed state in old mice.
Ribosome Biogenesis
In both the fasted and fed states, basal total RNA content (Figure 3B) did not differ between the 2 age groups and was not altered by contraction. Although basal Myc mRNA decreased with food intake (Figure 3C), Myc protein levels did not change with food intake. In the fed state, contraction increased Myc mRNA and protein levels, and the increase in Myc mRNA was greater in young mice. In the fasted state, contraction also increased Myc mRNA expression but not c-Myc protein expression (Figure 3D). Although basal Polr1b mRNA levels did not differ between the 2 age groups, regardless of nutritional status, contraction increased Polr1b mRNA in only young, fed mice (Figure 3E). Contraction did not affect Ubtf mRNA, but it was lower in old mice (Figure 3F).
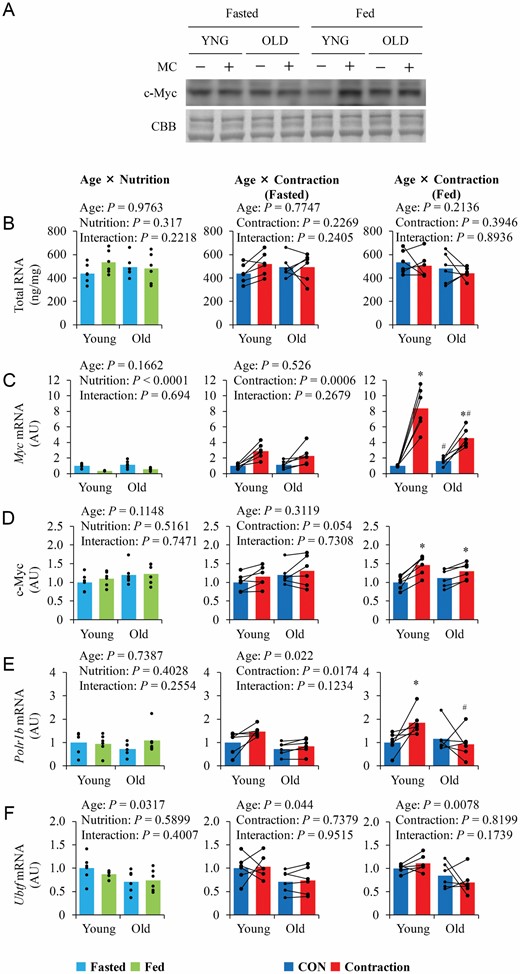
The influence of aging, nutrition, and contraction on ribosome content and expression of upstream regulators of ribosome biogenesis. (A) Representative blot image. (B–F) Quantitative values of total RNA and upstream regulators of ribosome biogenesis. Values are expressed by MEANS and individual values using bar–dot plot (n = 6 per condition). *p < .05 versus CON. #p < .05 versus similar condition in young.
Molecular Chaperones
Although basal HSP70 was higher in old mice, basal HSP73 and HSP90 expression were lower (Figure 4B–D). UNC45B mRNA and protein levels were lower in old mice (Figure 4E and F). Food intake and contraction did not alter the levels of the molecular chaperones measured in this study, except for UNC45B mRNA, which was increased by contraction in the fed state.
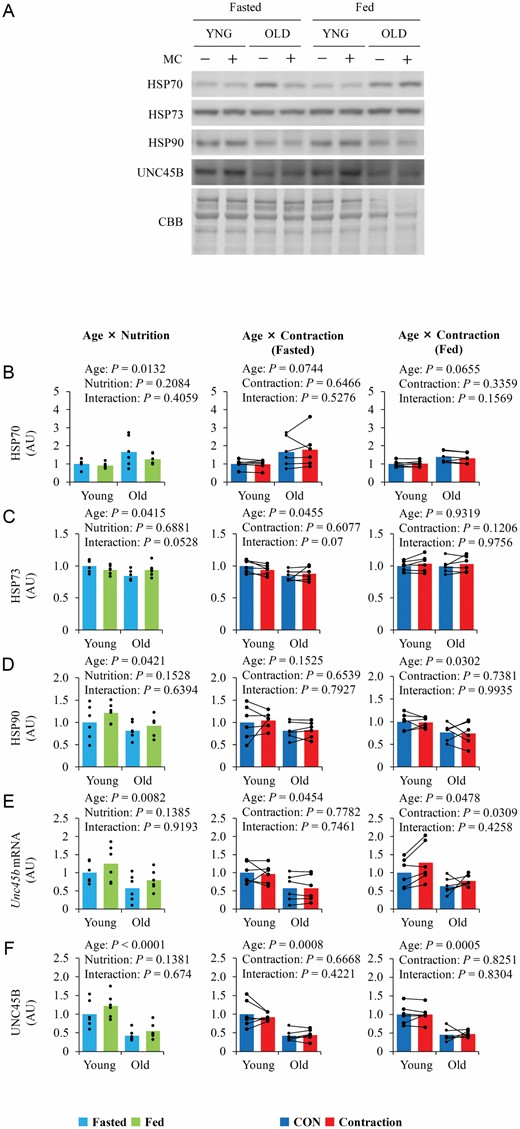
The influence of aging, nutrition, and contraction on myosin chaperones expression. (A) Representative blot image. (B–F) Quantitative values of molecular chaperone of myosin. Values are expressed by MEANS and individual values using bar–dot plot (n = 6 per condition).
Protein Degradation Pathways
Total and phosphorylated ULK1 were higher in old mice than in young mice (Supplementary Figure S1H and Figure 5B), and phosphorylated ULK1 was higher in the fed state than that in the fasted state. Contraction increased ULK1 phosphorylation only in the fed state. LC3-I was higher in the fed state than in the fasted state (Figure 5C), whereas LC3-II was only higher in the fed state in young mice (Figure 5D). Contraction, respectively, increased and decreased LC3-I and LC3-II levels only in the fed state. The levels of p62 were higher in old mice than in young mice (Figure 5E). However, neither nutritional state nor contraction affected p62 expression.
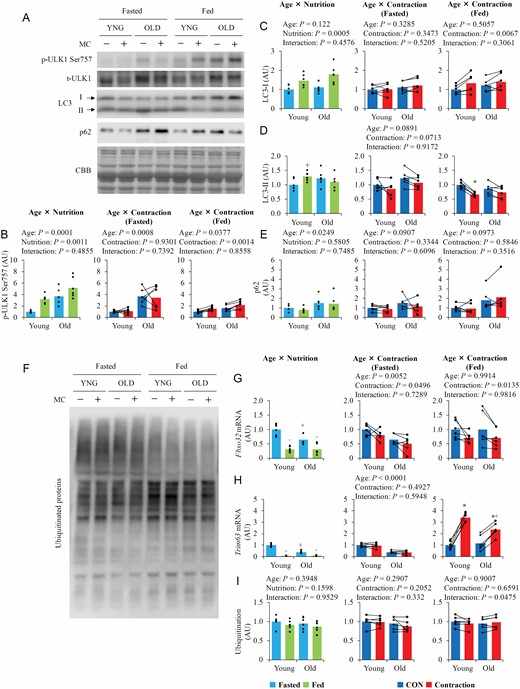
The influence of aging, nutrition, and contraction on the regulators of autophagy–lysosome and ubiquitin–proteasome system. (A) Representative blot images of autophagy–lysosome system regulators. (B–E) Quantitative values of autophagy–lysosome system regulators. (F) Representative blot images of ubiquitinated protein. (G–I) Quantitative values of ubiquitin–proteasome system regulators. Values are expressed by MEANS and individual values using bar–dot plot (n = 6 per condition). +p < .05 versus fasted. *p < .05 versus fasted/CON. #p < .05 versus similar condition in young.
Basal fasted mRNA levels of Fbxo32 and Trim63 were higher in young mice than in old mice (Figure 5G and H), but ubiquitinated protein expression did not differ between the 2 age groups (Figure 5I). Food intake decreased the mRNA levels of both Fbxo32 and Trim63, and contraction decreased Fbxo32 mRNA levels in both the fasted and fed states. Trim63 mRNA levels were not changed following contraction in the fasted state, but increased in the fed state. The increase in Trim63 mRNA levels following contraction was greater in young mice than in old mice.
Energy Metabolism-Related Factors
Basal Ppargc1a1 mRNA expression did not differ between the 2 age groups under fasting (Supplementary Figure S3D). However, PGC1α protein levels were lower in old mice than in young mice (Supplementary Figure S3E). Basal Ppargc1a1 mRNA levels in the fed state were higher in the fasted state. Ppargc1a1 mRNA levels were increased following contraction only in young mice. Similarly, contraction increased SIRT1 mRNA expression only in young mice (Supplementary Figure S3F).
Hk1 mRNA levels were significantly lower in the muscle of old mice than in young mice (Supplementary Figure S4B). In addition, although the nutritional state and contraction did not affect HK1 expression (Supplementary Figure S4C), it was lower in old mice than in young mice. In contrast, HK2 expression was not affected by age or muscle contraction (Supplementary Figure S4D). Pfkm mRNA expression was lower in old mice and was decreased by contraction in both nutritional states (Supplementary Figure S4E). Although PFKM expression was lower in old mice than in young mice (Supplementary Figure S4F), contraction did not affect protein expression levels. GLUT1 expression was higher in old mice than in young mice (Supplementary Figure S4G), whereas GLUT4 expression was lower in old mice than in young mice (Supplementary Figure S4H).
Discussion
In the present study, anabolic resistance to muscle contraction was observed only in the fed state. Given that the muscle protein synthesis response to muscle contraction in the fasted state was similar between young and old mice, anabolic sensitivity to muscle contraction is intact in old mice, but the interaction between nutrition and muscle contraction is impaired. Moreover, the myosin molecular chaperone UNC45B and its binding partner HSP90 were expressed at lower levels in old mice, and accumulation of p62, a marker of defective protein clearance, was observed, indicating that aging impairs processes related to protein integrity.
Aging Results in Impaired Muscle Protein Synthesis Response to Muscle Contraction in the Fed, But Not Fasted, State
High-intensity muscle contraction stimulates muscle protein synthesis via mTOR signaling (7,16). Nutritional factors, such as protein intake, also stimulate muscle protein synthesis. Protein/amino acid intake enhances muscle contraction-induced muscle protein synthesis (17,18); however, whether energy availability affects the muscle protein synthesis response to muscle contraction is unknown. In this study, both muscle contraction and nutrition status (fed vs fasted) increased muscle protein synthesis in young mice. Muscle contraction increased muscle protein synthesis similarly in the fasted and fed states, indicating that energy availability does not affect muscle contraction-induced muscle protein synthesis, and muscle contraction and nutrition have an additive effect in young mice. Conversely, in old mice, although nutrition and muscle contraction increased muscle protein synthesis in the fasted state, the increase in response to nutrition was lower than that in the young mice, and muscle contraction had no effect on muscle protein synthesis in the fed state. Unfortunately, we did not measure food intake. However, previous studies (19–21) had reported no change in food intake from young (2–3 months old) to old (22–24 months old) in C57BL/6 mice; thus, energy availability would not differ between ages. Therefore, our results support previous results (3,22,23) that aged muscle shows a blunted muscle protein response to nutrition and firstly indicate that aging impaired the integrative ability of muscle protein synthesis to muscle contraction and nutrition. Our result also supports the general agreement in humans that basal fasting muscle protein synthesis is not impaired with aging (1,24).
Nutritional Intake Facilitates Muscle Contraction-Induced Rapamycin-Sensitive mTORC1 Signaling in Young, But Not Old, Mice
mTORC1 has both rapamycin-sensitive and -insensitive signaling pathways. For example, phosphorylation of p70S6K Thr389 is rapamycin-sensitive, whereas phosphorylation of 4E-BP1 Thr36/45 is rapamycin-insensitive (16,25). Rapamycin-sensitive mTORC1 regulates cellular anabolic processes, including protein synthesis. In this study, muscle contraction increased the phosphorylation of p70S6K and 4E-BP1, regardless of age or nutritional status. Interestingly, in young, but not old, mice, p70S6K phosphorylation was increased more in the fed state than in the fasted state, indicating that aging-related anabolic resistance to muscle contraction may be associated with impaired rapamycin-sensitive mTORC1 signaling induced by muscle contraction. However, it should be noted that recent studies indicate that rapamycin-sensitive mTORC1 does not dominantly regulate muscle contraction-induced muscle protein synthesis (6,14,26,27). Therefore, further studies are required to clarify whether rapamycin-sensitive mTORC1 is associated with aging-related anabolic resistance to muscle contraction.
A previous study reported that nutrition and muscle contraction synergistically stimulate mTORC1 signaling through changes in REDD1 protein expression (28). In the study, REDD1 protein expression was lower in the fed state than in the fasted state and muscle contraction-induced mTORC1 activation was higher in REDD1-knockout mice than in wild-type mice. Therefore, the synergistic effect of nutrition and muscle contraction on muscle contraction-induced p70S6K phosphorylation in young mice may be induced by changes in REDD1 protein expression. However, similar changes in REDD1 were also observed in old mice, indicating that REDD1 is not involved in the mechanisms of age-related anabolic resistance to muscle contraction.
Aging Decreases the Ribosome Biogenesis Response to Muscle Contraction in the Fed State
Similar to previous studies (29,30), the basal total RNA content in our study did not decrease with age. Therefore, our results indicate that ribosome content does not decrease with age; thus, aging does not impair translational capacity. In contrast, previous studies reported that aging decreases the ribosome biogenesis response to muscle contraction (29,30). In this study, although muscle contraction did not significantly alter the total RNA content, it increased the mRNA expression of 2 markers of ribosome biogenesis, Myc and Polr1b, in young mice, especially in the fed state. However, although there was no interaction between age and contraction in the fasted state, there was a significant interaction in the fed state, and the increases in ribosome biogenesis markers were significantly inhibited in old mice. Therefore, our results indicate that energy availability is important for stimulation of ribosome biogenesis by muscle contraction, and aging is associated with a reduced ribosome biogenesis response to muscle contraction in the fed state.
Aging Decreases Myosin Chaperones
In this study, Unc45b mRNA and protein levels were lower in old mice than in young mice. Similarly, protein expression of its binding partner, HSP90, was lower in old mice. In contrast, HSP70 protein expression was higher in old mice. Previous studies reported that aging decreases UNC45B levels in rats and humans (31,32). UNC45B is required for proper myosin assembly (33,34), and HSP90 regulates myosin gene expression and the rate of myosin folding. This suggests impaired myosin folding ability in aged muscle, which may be associated with decreased muscle mass and function, independent of any impairment of muscle protein synthesis.
Aging Does Not Alter Autophagosome Formation and Protein Ubiquitination But Increases Expression of p62 Protein
In aged muscles, autophagic regulation is impaired (2). In this study, in accordance with a previous study (35), p62 protein levels were higher in aged muscle, whereas LC3 levels did not differ between young and old mice. Therefore, although p62 expression may be increased with aging, defective protein clearance is insufficient to meet the requirements of aged muscle.
Summary
We demonstrated that both muscle contraction and feeding increased protein synthesis and that the increase was additive in young mice. In old mice, both muscle contraction and feeding increased protein synthesis, but contraction had no effect on muscle protein synthesis in the fed state. Moreover, although muscle contraction stimulated ribosome biogenesis more in the fed state than in the fasted state in young mice, these effects were attenuated in old mice. Therefore, our results suggest that aging impairs the integrative ability of muscle contraction and nutrition, leading to aging-induced anabolic resistance to muscle contraction in the postprandial state. In addition to the decreased anabolic response to muscle contraction in aged muscle, the levels of myosin chaperones were lower, indicating that aged muscle has reduced myosin folding ability, which is related to functional impairment. Future studies are needed to elucidate why anabolic resistance to muscle contraction is observed in the postprandial state rather than in the postabsorptive state and whether decreased myosin chaperones are associated with muscle atrophy and decreased strength.
Funding
This work was supported by JSPS KAKENHI grant no. 26702028, 19H01085, 19H03999, and 22H03465 (to R.O.).
Conflict of Interest
None declared.
Author Contributions
R.O. conceived and designed research; S.A., H.M., and R.O. performed experiments; S.A. and R.O. analyzed data; S.A. and R.O. interpreted results of experiments; S.A. and R.O. prepared figures; R.O. drafted the manuscript; S.A. and R.O. edited and revised the manuscript; S.A., H.M., and R.O. approved the final version of the manuscript.